Amnon Yariv, Electrical Engineer, Quantum Mechanic, and Pioneer of Optical Communications
At 93 years old and as a full time faculty member who continues to lead a dynamic research group and shows no signs of slowing down, Amnon Yariv has no special secrets to share about the gift of longevity. Certainly, he acknowledges, genetics plays a role. But more compelling is that his mind is constantly engaged in exploring the forces of nature at the intersection of engineering and physics. His sense of wonderment, mixed with a dry sense of humor and a world perspective forged in his Israeli roots, must be the true secret. Born in 1930 in Palestine - 18 years before Israel's founding - Yariv served in the army that ensured the creation of Israel. With interests and talents that far outstripped Israel's educational offerings at the time, Yariv completed his entire higher education at UC Berkeley in electrical engineering.
Working at Bell Labs in its heyday, when the economics of the AT&T monopoly meant that money was essentially no object in pursuit of basic science, Yariv made foundational discoveries and contributions in the related fields of quantum electronics, optics and photonics. Then the West Coast beckoned. Roy Gould succeeded in recruiting him to Caltech in 1964, where Yariv continued his research. His work in semiconductor lasers and the distributed feedback laser had powerful possibilities in the commercial sector, and as fiber optic infrastructure expanded in support of connecting homes and businesses with cable, Yariv co-founded Ortel and subsequently helped to enable high-speed internet with the semiconductor laser.
In a recent development, Yariv recounts below a "eureka" moment he observed while taking a shower one morning: when the water is flowing and the shower wand is hanging detached, the tube moves in a way in the manner of an oscillating pendulum that has never before been scientifically described. With the paper in production, time will tell what comes of this observation - and what societal impact it may confer. Hailed as one of the greatest scientific minds of the last sixty years, Yariv is the recipient of many great awards and recognitions. One highlight is the National Medal of Science, for "scientific and engineering contributions to photonics and quantum electronics that have profoundly impacted lightwave communications and the field of optics as a whole." For that honor he met President Barack Obama, and he hopes one day to reconnect so they can discuss their shared interest in body surfing. For a life spent measuring waves in the laboratory, Yariv delights in noting his love of the ocean is also all about waves.
Interview Transcript
DAVID ZIERLER: This is David Zierler, Director of the Caltech Heritage Project. It is Monday, October 25, 2021. It's my great pleasure to be here Professor Amnon Yariv. Amnon, thank you so much for welcoming me into your office.
AMNON YARIV: My pleasure.
ZIERLER: To start, just to orient our readers, I'd like to point researchers to two previous oral histories with Amnon. The first was with Joan Bromberg, located here at Caltech. This interview day took place on January 28, 1985. And in this interview, you started with your Berkeley years and brought it right up until that present, 1985. The other oral history you did was with Shirley Cohen, also here at Caltech. That one was more of a full-life oral history, where you talked about your family background and your childhood, and you took that right up to the present at that time, which was 1999. So our first effort today, circa October 2021, is to fill in the years from 1999 all the way to our present, 2021.
Amnon, perhaps to start, let's bring it back to the year 1999, the year 2000. You left off with Shirley Cohen talking about all of the work that you had done with your Japanese colleagues, and you mentioned also a certain pendulum shift in terms of Japanese students staying in Japan versus them coming here. Let's start with that. After 1999, in terms of the education and the best research in the fields that were important to you, were the Japanese staying at home, or were they coming here to the United States?
YARIV: The Japanese stayed at home because Japan, at that point, had advanced in my area, both economically and technologically to the point where Japanese students could get first-rate education in Japan, probably most notably at Tokyo Institute of Technology, the University of Tokyo, and maybe the University of Osaka. There were good universities, and the source of the students was mostly leading Japanese companies like Hitachi, Nikon, companies in optics and semiconductors, Toshiba. And at that point, they were probably technologically ahead of the US in terms of making lasers, advanced electronics. Fewer and fewer Japanese students came over. More Koreans, more Israelis, more Chinese. So yes, to answer your question, there was a shift. Probably very few Japanese students today in this area in the US.
ZIERLER: To orient our researchers, what are the industries or the fields of research that were so important to you, but for which the Japanese were leaders at the time?
YARIV: Well, the Japanese were not leaders at the very beginning. we have taught them, for instance, how to make semiconductor distributed feedback lasers that were becoming the main light source for optical fiber communication (they still are). At Caltech in my group we were pursuing a number of problem areas in laser physics and nonlinear optics. The most important of them, in terms of people, resources, were semiconductor lasers and optics. Essentially, a transition, where today, the most daring and exciting experiments in optics are not done with lenses and each component a box on a tabletop, but on crystals. Light is not propagating through air or vacuum, as it does in a camera for instance but in waveguides, which are essentially chemical waveguides on crystals.
And the whole device, which used to occupy a tabletop, will now be about, let's say, a millimeter by a millimeter, compressing many, many components. That was one area. Probably the most important of them, in terms of both intellectually and the impact on technology was integrated optoelectronics. The idea that you could make, on a single crystal, by photo lithographic techniques, essentially, everything you need to perform communication functions. For instance, making, as I've just shown you in the reprint, lasers, modulators, detectors, complete optoelectronic transceivers and receivers, both on a single crystal of Galium Arsenide and related semiconductor alloys. That is becoming the most important communication technology of the 21st century. Joyce Poon described the initial proposal from my group at Caltech in Isfhan (Iran) in a conference in 1971and the first ever demonstration of optoelectronic Integrated circuits in 1978 , in my group at Caltech.
Defining Optoelectronics
ZIERLER: Now, if you can orient yourself back to the year 1999, 2000, what were you working on at that point? The dates are too late. It is important here to get them exact because I find a reluctance on the part of some circles to give Caltech credit for the starting this field. I think it will have major bragging rights and important for Caltech as well as for the people directly involved, including myself.
YARIV: I gave you a bunch of reprints. I haven't done my homework very well over the weekend.
ZIERLER: That's OK.
YARIV: We were at the beginning of integrated optoelectronics. The info I gave you just above and the one shown by Joyce Poon is more accurate. I actually I first proposed IOECs in around 1967-8. We probably could find it in the proceedings of the international semiconductor meeting in Japan in the late 1960s. What's the date on that?
ZIERLER: This is 1984. Were you still working on optoelectronic circuits?
YARIV: I've actually been working on optoelectronic circuits until a few months ago.
ZIERLER: A few months ago.
YARIV: I'm actually still doing it a little bit. The method was bought by industry. And a lot of people are doing it. It's still in the beginning. We'll talk more about it as we go on. And my guess is the next big impact will be on electronics for self-driven automobiles. Although we prefer them starting with the name optoelectronic integrated circuit, the world decided they were photonic integrated circuits, or PICs. We use both names interchangeably.
ZIERLER: Scientifically, is one better or more descriptive than the other?
YARIV: Well, optoelectronic probably describes it better because it's optics and electronics in the title. Photonic integrated circuits don't tell you that it is integrated with what. The word photonic is derived from photons. I prefer the first one, but the world has decided to go one way, and we'll not make a fight about that issue. That reminds me of when I was at Bell Labs, when I joined them in 1959, the term optical masers was used then instead of lasers. And I was the one, I think, at Bell Labs that, in writing a significant paper, used the word lasers. And I got a lot of flak from it at Bell Labs. And I made the same argument. [laugh]
ZIERLER: You should've called it maser, they were saying?
YARIV: They wanted me to call it optical masers, and I called it lasers.
ZIERLER: Did anybody use that term before you?
YARIV: Yes, yes. I did not invent it. But I basically made the point that once the world shows a strong preference, and you are in a small minority, don't make a fight about names.
ZIERLER: What was the distinction in your mind? Why was it important to call it a laser?
YARIV: Because it was one word. [laugh] And shorter. But it was not important for me intellectually to call it a laser. It's just that I could see which way the wind blew. More and more of the papers published, a majority, used the word laser.
ZIERLER: Who was giving you flack at Bell Labs for this?
YARIV: I don't recall their names, but I got flack. It wasn't serious. Bell Labs was a fairly democratic place.
ZIERLER: On the personal side, tell me about the article you wrote in 2000, Catching the Wave. Who asked you to write this autobiographical article?
YARIV: I think the Journal of Quantum Electronics, the leading journal in the field.
ZIERLER: And they wanted you to just write about your life experiences?
YARIV: That's it.
ZIERLER: Why the title, Catching the Wave? What does that refer to?
YARIV: Probably, if you look at my career, almost everything I've done involves waves, optical waves. Probably no exception. The Shower experiment would be the first exception.
ZIERLER: We'll talk about that.
YARIV: And I was a body surfer, which was the main sport activity and I was involved waves… I caught a wave. In Hebrew to surf is translated as wave catching and I was a charter founding member of Agudat Tofsei Hagalim -The Association of Wave Catchers- founded on the beach in Tel Aviv in about 1946. As you'll see from the story, decisions. I went to Bell Labs and I was looking for something to do. Bell gave us nearly complete freedom to do what we wanted as long as we stayed loosely within the field of interest to communication and information technology. The semiconductor laser was invented, demonstrated at IBM and GE Labs. I with my group were the first people at Bell Labs to make a semiconductor laser.
ZIERLER: What was Bell's interest in the semiconductor laser? What was exciting about it?
YARIV: Well, they were not really interested. They didn't invent it, were not involved in its invention but they were intelligent and understood communication i, maybe John Pierce or Rudy Kompfner, thought maybe semiconductors were important. They did not know much about semiconductors but they knew that transistors were important. The labs could afford to have a few scientists play around bit in this area. The transistors were invented at Bell Labs. It was a small thing to ask a scientist at Bell Labs to look into it. I was a new kid on the block, really looking for something significant to do. My first duties at Bell Labs were other things. So I was asked, "Would you be interested?" And I said yes. I forgot why. I was particularly interested. And I made the first semiconductor laser at Bell Labs. And a year later, in the process of making that laser at Bell, I discovered that the laser depended crucially on the phenomenon of optical wave guiding the confined lossless propagation of light inside a channel of a high index of refraction material which is surrounded by material of a lower index. The first lasers were two polished mirrors which enabled an optical wave to bounce back and forth between the two mirrors and be amplified by the gain medium which occupies the volume between the 2 mirrors. prevent the reflected light from escaping by diffraction away from the semiconductor amplifying (gain) medium between the mirrors. My first challenge was to decide what confined the light in the lasers since their GaAs lasers did not have curved end-reflecting mirrors. Just flat parallel polished surfaces.
In the semiconductor laser, the confinement of the light, which was essential to any laser, was done by essentially optical guiding. There was an optical waveguide that confined the light like water in a pipe. With Rogerio Leite we showed that the depletion layer of the p-n junction in the semiconductor laser acted as an optical waveguide and without it the laser would have never lased because the optical losses sue to diffraction would have been too high. Modern optics and lasers as well as integrated optics is based on guiding light on the crystal in those dielectric waveguides. Those are the equivalent of wires in electronic circuits carry which optical energy and signals. In my first experiments, I was trying to understand why the semiconductor worked at all. Because light obviously came out of a very small area of the end reflecting surfaces, and using the height of a simple diffraction loss calculation, I found that the losses would be so eliminating the diffraction loses magnitude. Somebody mentioned to me dielectric waveguiding about which I knew very little at the beginning. As it turned out, the electromagnetic understanding of how to design the confinement and the guiding and the modes of the laser field turned out to be as or even more important than the solid-state physics in designing SC (semiconducting) lasers, and that was new. This was something our world-famous solid-state physicists at the labs did not know much about so it became the domain of a group of us electrical engineers at John Pierce's communication department, which was my home at Bell. That was what gave us at Caltech later on a head start.
ZIERLER: And there's no diffraction with this laser?
YARIV: It loses energy by other mechanism, mostly scattering but a negligible percentage by diffraction as long as the light in the laser is waveguided. It's like a pipe. If you water your lawn with a hose, the light comes to you from wherever, in a pipe. As soon as it gets out, you have a big shpritz of light in all directions. Same with light. But practically all of optics up to that point had to do with free space propagation, and diffracting propagation of light of optics before that is essentially free space propagation of light. The realization that you can create light; i.e., make lasers in III-V semiconductors, guide the light in the dielectric waveguides produced by doping and composition in a single crystal, was a Eureka moment for me. What also helped was that the resulting device could be combined with transistors in the SAME GaAs crystals. This led to the proposal and demonstration later at Caltech of the new technology of Integrated Opto-Electronic Circuits (IOECs)
ZIERLER: What year did you come to Caltech?
YARIV: 1964.
ZIERLER: Were you recruited here out of Bell Labs?
YARIV: No. I joined Bell Labs in 1959, and after maybe three or four years, I decided that although I loved it there–I even enjoyed New Jersey [laugh]–that I did not see it as my long-term place to spend my career. For reasons which were not exactly clear.
ZIERLER: Did you want to teach? Did you want students?
YARIV: No. I hadn't taught before. I did not have a teaching experience, and I did not have a burning desire to teach. But I felt that having been born in the desert, the Middle East, I needed sun. And it's very difficult to get down to Coast around NYC, if you've ever tried it. [laugh] Only in a few months in the summer, you can do that. Yes, but then the one or two highways, the Garden State Freeway, the New Jersey Turnpike, took hours to make the 30, 40 miles from summit. And eventually, I wound up spending the hot summer weekends in a swim club, many were antisemitic [laugh] and would not accept us as members wound up on weekends swimming, driving over to the Delaware river on the border between Jersey and Pennsylvania, and that was nice.
ZIERLER: But you needed more water in your life.
YARIV: So I came back to the dry West Coast. Looking at Bell Labs, there were a whole bunch of very famous people, I decided that it was too confined, maybe intellectually, socially. I didn't see it as a place where I wanted to spend the next 30, 40 years. I didn't go back directly to university. When I left Bell Labs, I had two young children. I resigned from WJ before being offered the summer opportunity at Lockheed.
ZIERLER: What was the problem?
YARIV: Needing to spend my time bringing in money, rather than promising to do something. Making the elecro-optic modulator, as an example.
ZIERLER: Although, ironically, at Bell, you never had to worry about bringing in money.
YARIV: Never. Yes. I was too proud to go back to Bell Labs, though I could've done it. They told me, "You can come back any time." I did well there, they liked me. But I was too proud. And the basic objection to growing old at Bell Labs, it was not an option for me. I liked the West Coast.
ZIERLER: And going back to Israel was out of the question at this point.
YARIV: Out of the question. Don't forget, we're talking about eight years or so after independence. And there was no real research in Israel.
ZIERLER: Nothing was happening at Technion yet?
YARIV: Very little. There was an exception, which I'll talk about later. And I wanted to do research. It was clear that was what I wanted to do. And while at Berkeley, my PhD thesis, which eventually was on lasers, started by work on traveling-wave tubes. A microwave device which is an an amplifier. It's used on communication satellites today. Very good, but very large, and an interesting area of research. My master's thesis, first two years, 1954 through '56, I did a project on traveling-wave tubes with John Whinnery, who was one of the leading experts in the field, wonderful professor. Also my thesis advisor. And I decided it was interesting. Anyhow, that got me interested in waves and so on. And the main center for research on traveling-wave tubes was Caltech.
The Centrality of Roy Gould
ZIERLER: Who was leading it at Caltech at that point?
YARIV: A young professor called Roy Gould, who retired maybe seven, eight years ago, and died a couple of years ago. Wonderful scientist. Wrote a bunch of very influential and insightful papers, which I had read and admired. I knew him. I met him at a meeting or two. And after two years at Bell Labs, maybe '61, '62, I ran into Roy Gould at a meeting and by that time, had written a number of basic papers was known in the new growing field of lasers. And Roy Gould told me, "Look, if you are ever interested in an academic career, call me." And we probably had dinner together, good friends, and I had no desire at that time to go to a university. I was in the thick of making lasers and other things. But of course, I remembered it. And when I became dissatisfied with my job at Watkins-Johnson, I resigned. Th Lockheed research Lab in Palo Alto agreed to offer me a temporary job till the beginning of the academic year in Sept. 1964 since I had already decided to move to Academe.
ZIERLER: Who was your connection at Lockheed? What were they doing that was relevant for you?
YARIV: Lockheed had and has a research lab on Hanover Road in Palo Alto. It's the heart of Silicon Valley, half a mile from Stanford. Lasers were, at that point as important. Not sure why. There were about maybe a dozen people at Lockheed researching them. And I didn't know them before, but they knew of me. I called them, and we agreed to try an idea, a project I wanted to work on. And they agreed to give me a lab and an engineer to work with me. And we did some interesting experiments during that summer. And in the meantime, I had applied to Cornell, Stanford, Berkeley, UC San Diego, and my friend Roy Gould at Caltech.
ZIERLER: Was Gould a research island here? Or were there collaborators he had?
YARIV: He was on his own working on traveling-wave tubes and related issues. And he did some first-rate work.
ZIERLER: What are traveling-wave tubes, and what do they do?
YARIV: A traveling-wave tube (TWT) is an amplifier of high frequency, microwave, signals. It is very wide band so it can amplify microwave signals which is modulated by a large number of tv channels cable tv programming. Like all amplifiers, a microwave signal carrying information, like tv programming, enters the TWT amplifier from one end, comes out amplified maybe 1,000 times or more from the other. Still used today on satellites because it's the best amplifier velocity and then shot through the center of a metallic helix and are moving inside and along the axis of the helix. The microwave signal is applied to the helix and propagates along the helix. which slows it down so it can stay in step the electron beam. And you have to match the velocities.
You can change the voltage on the electron beam and change its velocity until the velocity of the wave projected along the horizontal and the electron beam are nearly in sync. And then, the energy is transferred from the electrons to the wave. And the wave grows -amplified. It comes out of the other side. It's a vacuum envelope, a helix electron gram, and the wave comes out of the other side amplified maybe 1,000 or 10,000 times. For communication satellites, you send a signal, let's say a telephone call, from here to a satellite, obviously by the time it comes to the satellite, it's very weak. But you want to bounce it back from, let's say, the US to a satellite into Asia. You need to amplify it, otherwise the noise will overcome it.
ZIERLER: Now, was Gould already thinking about applications to satellites? Or that's too early?
YARIV: No, Gould was not, I think, thinking of that. The idea of communications satellites started at Bell Labs by my bosses John Pierce and Rudy Kompfner. And they made the world's first communications satellites. And those were big balloons coated with silver. The next big step in the basics of the communications satellites of today came from a Caltech aeronautical engineer, Rosen, then a scientist at Hughes Aircraft synchronous satellites. The first-generation Bell Labs were low-orbit, so they went around every hour or hour and a half because they were low. And then, the idea of having a synchronous satellite, a satellite that would actually, as it revolves around the earth, would revolve at the same speed as the earth rotations, an angular speed.
So the satellite would essentially hover over the same spot on the earth, and that was crucial. But you needed to stabilize it. Those two main ideas came from Rosen, that was his name. His older brother was on the board of trustees of Caltech later. Anyhow, that was the basis of the present-day technology of synchronous communications satellites. Roy Gould was not involved in the satellites, but he was, in my mind, probably the leading theoretician in the exchange of energy in the traveling-wave tube and the noise. Always, noise is the most important, as you'll see in my own work later on the laser.
I called Roy. Roy was on sabbatical, I think in Australia. And Roy called a colleague at Caltech, who invited me to give a seminar. I came down, and maybe a month later, I had an offer from Caltech, so I moved to Pasadena from Palo Alto in 1964. I had offers from all the other universities as well. Cornell, I went to interview with them in April. There was half a foot of snow on the ground so that removed cornel from my list.
ZIERLER: That's even farther from the ocean than Bell Labs.
YARIV: UC San Diego…
ZIERLER: Must've been brand new at that point.
YARIV: Maybe five or six years old. They were too near the ocean. [laugh] Remember, I was a body surfer. You'll read about it in the title of the wave. I was a founding member of Israel's Agudat Tofsei Hagalim (The Wave catchers Association.) There were maybe 20 of us who would go body surfing in the winter, when big waves came.
ZIERLER: Oh, a polar bear.
YARIV: Polar bears. Of course, the Mediterranean doesn't get as cold. [laugh] But still, it was probably 60 degrees or so, and no wetsuit.
ZIERLER: So San Diego, you wouldn't get any work done. You'd be in the ocean.
YARIV: I probably wouldn't get any work done. [laugh] So I had to pass up San Diego. Berkeley was like going back home. Which some people like, some do not.
ZIERLER: You're a kid back home.
YARIV: You're a kid. And so, I passed over Berkeley, although I was very happy at Berkeley as a student but going back seemed like never leaving home. That left Stanford. Stanford offered a position but they already had two laser scientists, good ones, so they wanted me to work on something else.
ZIERLER: What were these jobs you were applying for? Was there an applied physics program at any of these places yet?
YARIV: No.
ZIERLER: Or were you applying in EE?
YARIV: None. I applied to EE and physics. The offer at UC San Diego was in the Physics Department. Because I had already written a paper on quantum theory of nonlinear optics, which was very significant. It gave people the Hamiltonian. [laugh] How to treat problems involving a change of energy between optical waves taking place in crystals.
ZIERLER: So there's no applied physics programs anywhere yet?
YARIV: Departments of applied physics did not exist. There were no faculties or programs anywhere, at least not designated as such. Caltech was the first one to offer it as an academic option. And Roy Gould and I started it.
ZIERLER: Where was Roy? Was he in EE or physics?
YARIV: Roy got his PhD in physics but was in EE or maybe in both. Really, an exceedingly sharp and influential fellow. Jumping ahead a little bit, we came to Caltech, and I started a program. And there were plenty of good students who wanted to join the effort because there was great curiosity. There was actually a professor in EE who had done some early work before me, but he was really an EE., he made an helium-neon laser, which was a replica of what Bell Labs had done. But that was not his main interest or main capability. And there was a small effort here. It was based on physics and EE on crystals, up to date.
ZIERLER: In choosing Caltech, was JPL a factor for you?
YARIV: No. I don't think I was aware of the connection between Caltech and JPL at that time.
ZIERLER: Did you take advantage of that connection later on ever?
YARIV: Not really. What brought me to Caltech was Roy Gould. Roy was so good. I also liked him personally. When I visited here, I became aware of the academic stature of Caltech. Coming here, I realized it was probably a different school from the others that I mentioned. It was small, but with no boundaries. Students worked for which ever professor they wanted to, they didn't have to fill out forms, re-register, or whatever. Also, a center of aerospace, which I realized would be important for me. There was a huge, huge negative factor. Smog. It was the worst period for smog. And you have no idea how bad it was. I almost left Caltech during the first year because of the smog.
ZIERLER: Now, were you aware that there was a professor at Caltech hard at work to fix the problem?
YARIV: No.
ZIERLER: What about Feynman? Was it attractive to you that he was here?
YARIV: I didn't know who Feynman was. [laugh]
The Maser Revolution
ZIERLER: Feynman diagrams though.
YARIV: Yes, I learned that later, even wrote a paper with the words Feynman Diagrams in the title. I actually learned a lot of that in those first few years. I wrote papers. I started teaching a class. Extremely ambitious. But I was young, 34. The physics, the mathematics, the quantum mechanics. At Berkeley, my education was really EE. I arrived at Berkeley in 1951. My plan was to be a power electrical engineer. Transformers, generation, transmission. Work for the power company. After two or three years in Berkeley, my interest shifted slowly but decisively away from power generation to microwaves and radio transmission. And the reason was, basically, a class I'd taken with John Whinnery, the same based on a text that he had written with Si Ramo on radio waves. A beautiful class, rigorous, mathematical. When I graduated in 1954, I joined John Whinnery's research group, that was working on traveling-wave tubes. It was interesting for a year or so, but that field really doesn't have the Maxwell or the Schrödinger equation, the rigorous mathematical foundation. It was more ad hoc.
I became bored with that field, too. And then, in 1956, when I got my master's degree, John Whinnery asked if I would like to join him attending a conference probably because he thought that might help me in finding a new problem to work on for my PhD. The conference was called the Tube Conference, a small, by-invitation-only conference of maybe 30 or 40 people, met every year at a different place. The people who started it, the main movers in that little conference, came from traveling-wave tubes. Caltech, Stanford, and Berkeley. And they were mostly top notch. A wonderful group of people. But they would invite to every meeting annually somebody from a different field. John Whinnery asked me if I would like to join him in going to Boulder, Colorado, the site of that year's Tube Conference–they later changed the name–to The Device Research conference. About the research, which had concluded, looking at electron dynamics and traveling-wave tubes. He thought it would be interesting. John and I drove from Berkeley to Boulder, a two-day drive or so, and there, two scientists from Bell Labs gave a talk about an optical maser. I never heard about it before. And there, he made a maser.
ZIERLER: What was so exciting about the maser at that point? What did they want to convey?
YARIV: They made a microwave maser, essentially a laser but operating at lower, microwave, frequency. It was exciting because it was understood to be potentially a very low-noise amplifier. Amplifiers are used to amplify weak signals, signals that have traveled long distances, from outer space. And to amplify a very weak signal, you need to be very careful not to swamp the signal with noise. And every amplifier adds noise. But classes of amplifiers among the masers or parametric amplifiers add the smallest amount of noise allowed by quantum mechanics. And that was understood. Masers was a very interesting area of research at that point. There were no lasers yet. And I did not understand what they did, the physics. But I could tell the area was elegant, and they gave a good talk. The two scientists were Feher and somebody else. Feher was actually an Israeli who got a PhD from Berkeley about four years before me, working for my thesis professor, John Whinnery.
ZIERLER: Did you know him?
YARIV: Met him in Boulder.
ZIERLER: That was the first time.
YARIV: First time. And I had already decided with John's agreement that for my PhD, I'd do something different. And part of the reason for inviting me to come to the meeting in Boulder was to maybe find another topic, talk to people. And indeed, I did. And of course, there was George Feher. He eventually joined UC San Diego. Very well-known in his own field, which was paramagnetic resonance. And I told him about my problem, and he invited me to come to Bell Labs and work in his lab. And I took him up on his offer, and John Whinnery made it financially possible. Then, I went to Bell Labs and worked with George Feher and learned about his field, the instrumentation, which was quite complicated, mostly microwave. Then, came back to Berkeley and built a system. I didn't have the money to buy the components. Much of it, I had to make myself. That's how I wound up moving from power transmission, generators, which was my aim when I left Israel, to lasers.
ZIERLER: Now, to give a sense of your sensibilities at this point, were you interested in the theory or more the applications of masers?
YARIV: Let me go a step back. When I made the decision, remember, my background was two years of machinery and maybe another year or so working with microwaves, electron beams. Almost no physics, except a one-year, three-semester sequence of physics that all engineers have to take. Essentially, very little physics. And here, I was delving into a new area, which required quantum mechanics, solid-state physics. When I decided to work for my PhD on lasers after my master's degree in '56, I needed to learn graduate level physics. And I worked 20 hours a day. For a learning physics took undergraduate physics, nuclear physics, electromagnetic, quantum mechanics, solid-state physics, and advanced quantum mechanics, all in one year. And it was very interesting. The beauty of electromagnetic theory, Maxwell equations, and quantum mechanics and their coming together and that you start with a well-defined differential equations, Maxwell, Schrödinger. And I think that by my temperament, I needed something I could fall back on. And I did not find it in the other fields that I worked in. There was too much information. "Oh, that's the way it's been done. It works." Here, you could always start from the Maxwell equation. And maybe I'm a Yekke.
ZIERLER: That's a German Jew, who in Israel have a reputation for being very finnicky for our audience, we should say. Somebody who's very precise.
YARIV: Precise. They were much more satisfying. I think by temperament, I enough in my new field so that some of the professors wanted to recruit me and have me switch majors from EE to physics. I didn't do it, mostly, I think, out of loyalty to John Whinnery. And I saw no reason for doing it. I could take the classes. John promised to help me build an experimental system.
ZIERLER: Did you spend any time at the Rad Lab?
YARIV: No. I'm not sure that the Rad Lab was–oh, yes, it was there. It was the only thing that was there. No, I did not. Actually, I've never been there physically. [laugh] A friend of mine ran it for a while. So when you ask me…
ZIERLER: About theory and application.
YARIV: Applications were not even on the horizon. It was just interesting. I'm basically a researcher, I'm curious. But because of my background, had I started in physics as an undergrad, I very well might've wound up being a physics researcher. But I went for an engineering background. John Whinnery and his group. What we were doing was related directly to traveling-wave tubes and communications systems. And I found it very satisfying to work on problems that had both a physics background and application. And the appreciation and feel for waves that I acquired in my engineering classes was very important as well. And the rest of my career, the two are intertwined. I wrote a few papers in pure physics, like the quantum theory for nonlinear optic. The National Medal of Science I got was in physics, not in engineering, which is part of it also. And I was a member of the National Academy of Engineering and Sciences. I'm basically both.
ZIERLER: When you got here in 1964, what department were you hired into?
YARIV: EE.
ZIERLER: Is that when you met Carver Mead?
YARIV: Right away. As a matter of fact, my first work at Caltech was an experiment with Carver Mead, investigating the properties of a crystal, gallium arsenide, to modulate light. To do that, you need to understand the electrooptic effect the physical effect, which is used to modulate light in crystals. It's the change in the optical properties of the crystal when you apply a voltage, a field to it. And you use that to make modulate light. If you're interested, my texts in the laser applications book has maybe two chapters on the topic. That's how you can put information, a Netflix movie for example, to ride piggyback on a laser beam that travel on an optical fiber from new Virginia to LA. For some reason, I don't know how, Carver [Mead] and I met, resonated, became good friends, went to a conference in Europe together. He'll still tell you stories about my talking Yiddish in Denmark, and the waitress understanding my Yiddish. [laugh] And we wound up doing an experiment together. Carver was always curious. We published a paper, the first measurements of the electrooptical coefficient of gallium arsenide. I learned my quantum mechanics in one year from good teachers. Berkeley still had a good fraction of professors who developed the A bomb in Los Alamos. Oppenheimer, Lawrence, Teller, a few others. And it was fascinating to take classes from all of them. Emilio Segré, a whole bunch of Nobel laureates.
ZIERLER: With Gould, why the need to create an applied physics program in and of itself?
YARIV: When I came to Caltech and started teaching classes in laser physics, forming a group to work in this field, the students who came to work for me were mostly physics students. Because the prerequisite, you needed quantum mechanics, and you needed electromagnetic theory. And the EEs who came usually had the electromagnetic Maxwell equations part of it, but most of them did not know quantum mechanics. And they came, and they stayed. The physics students got the degree in physics. There was no problem at Caltech. But at some point, it was clear that we needed a different type of student. A student with mostly a physics background, but interested in applications.
Light modulation, light propagation in crystals requires solid-state physics. We needed a new type of student as a graduate student, even undergraduate, but with a strong background in physics, and not in elementary particle physics but solid-state physics and electrical engineering. But electrical engineering, the John Whinnery type, waves, not transformers. And that did not exist. Roy probably had a similar need, not quite as acute as mine. I don't think he needed quantum mechanics. I came to Roy Gould and told him about my problems. And he agreed to join me. And it didn't take too much problem to start a new option. I think we might have been the first applied physics program in a university. It took other schools years to follow, Stanford maybe 20 years. Eventually, every school had it. I think we were the model. We were very successful.
Joining Caltech
ZIERLER: Did the program grow rapidly?
YARIV: I think there was no time in which professors in applied physics could not get all the students they needed, even today. Yes. And we got some very good students. At some point, we got top students from the Physics Department. There were no boundaries, physics students could work for me without having to change departments.
ZIERLER: In terms of the students that you did get, if they were in physics, why would they have not gone to EE? Why would they have come to applied physics?
YARIV: I don't think you'll find many physicists who will introduce themselves as electrical engineers, [laugh] even if they're doing electrical engineering in their career later on. They're physicists. It carries a bigger cachet, I think.
ZIERLER: Here, in applied physics…
YARIV: Especially at Caltech. The students came here because of names like Feynman. But I think the thing that attracted the students from physics to my group was the intellectual challenge of quantum electronics, as the field was known at the time. Even today, quantum computing, the building blocks are lasers. There was an intellectual challenge there. Some people are attracted to elementary particles, other people to exchange of energy within light waves in crystals. Which requires quantum mechanics as well.
ZIERLER: Were there any computers on campus in 1964?
YARIV: When I came to Caltech, I still have a mental image of people walking around the campus carrying stacks of IBM punch cards. But they came gradually. Maybe not as fast as at other places. Berkeley or University of Illinois.
ZIERLER: In setting up your lab, what were your major funding sources?
YARIV: I got an initial grant and was able to buy some basic pieces of equipment. And then, during my half-year at Watkins-Johnson, before coming to Caltech, I obtained a few contracts from sponsoring agencies. And it was not too difficult. There was a great deal of curiosity in the sponsoring agencies on the Office of Naval Research, National Science Foundation. I had a few research grants, two or three. Roy Gould was very generous and shared with me a research grant he had from the Navy. I was able to set up a lab with two or three students. The number grew. We eventually obtained more support. At the height, we had probably over 20 students here, and five or six post-docs.
ZIERLER: Did you come in with tenure?
YARIV: I came in as an associate professor without tenure, with the promise that my tenure would be considered two years later. Which it was, and I was promoted to professor. While I had offers of tenure and full professorship from Cornell. I came to Caltech, although it was probably the least attractive offer financially. And here was smog.
ZIERLER: When did the smog start getting better?
YARIV: I came here 1964. I know it was more than ten years. Maybe 15, 20 years, when the regulations about not incinerating, unleaded gasoline, eliminating the additive. It was a gradual process, and it took time to see the mountains. You heard the jokes about Caltech, visitors coming here in May and in October when the smog diminished saying, "We didn't realize there are mountains near Pasadena." [laugh]
DARPA and Semiconductor Lasers
ZIERLER: What were the big questions that you were after when you were setting up your lab in Caltech? What were you most curious and excited about doing?
YARIV: I started working right away on semiconductor lasers. I made a decision that these lasers are important and in time will become the most important. There is whole variety of lasers. Gas lasers that took up literally a tabletop like that based on argon, pumped by all kinds of means. And I decided individually, I probably was not alone, but maybe one of the very first to translate it into a career, that semiconductor lasers are going to be the most important lasers in the world. Why? Because they were electronic. Could be made with the technology as transistors. you could make them small and efficient. At Bell Labs, already, I had switched and there was a need for a superior and concentrated on semiconductor lasers. I had made lasers and thought it was a good topic. And the only topic I really understood fairly well.
ZIERLER: And if you can explain the name. There are semiconductors, and there are lasers. This is a semiconductor laser. What does that mean?
YARIV: The lasers are not that different from transistors. Both can be made from layers of semiconductors of different properties. I also realized that you could make lasers, things you need to make a laser, to guide light, to amplify it, in semiconductor crystals. The very same crystals that can make transistors. Semiconductor lasers are driven by current. The idea that you could combine optics and electronics was probably my idea. I proposed it first in a international semiconductor conference in Japan in Japan, maybe 1966, '67, '68 on the feasibility of combining optics and lasers on single semiconductor crystals. A theoretical talk. And an American gentleman, Martin Stickley from DARPA, approached me after my presentation and asked me who supported the work. I said, "Nobody. What you heard is the basic theoretical idea.". He said, "Are you interested in support?" I said, "Of course." [laugh] He wound up supporting my work for maybe 30, 40 years until he retired from DARPA.
ZIERLER: Was he thinking about national security applications?
YARIV: DARPA by definition, the nature of the organization, is research of immediate or potential importance to national security.
ZIERLER: What could it have been? Why would this technology give the United States a military edge, theoretically?
YARIV: DARPA has become more of a bookkeeping operation today. You have to tell them what it is that you will invent how much power, what efficiency, at the beginning. At that time, they were more adventurous. At least in the areas with which I was acquainted.
ZIERLER: Adventurous.
YARIV: –adventurous. And each program manager–and the fellow who came to me over in Japan was a program manager–handles maybe a few million dollars. There were certain limits. You had to stay within the area of interest, to both DARPA and you. This meeting in Japan is a good example of DARPA in those days. Stickley was able to offer me support. I still had to submit a proposal with a budget etc. But he sensed that the idea of combining optics and electronics monolithically is worth supporting.
ZIERLER: Let's go even more fundamental now. Combining optics and electronics, what is optics before electronics? What does the field look like before it's joined with electronics by you?
YARIV: Well, take a camera. In a camera, you have an image. If you take a photo of your daughter, light waves, sunlight, originates by reflection of a light source, sun or a lamp, in her face, reflecting from her face. The image thus formed propagates through the camera to a lens which images it on a film plane. And that was photography. It involves, essentially, propagation of light between optical components, lenses, film plane, light source in vacuum. You don't need air. It's not sound. And here, you're taking all of that and condensing it into a small area of volume. And the need to continue to refocus light if you want to send it from one place to another using lenses, is obviated here because the light is now guided in optical waveguides.
One of the papers that I gave you, the beginning of integrated optoelectronics, has a reprint or a description of a very early experiment at Bell Labs claiming that you could not have a laser without optical waveguiding. That optical waveguiding in the first generation of semiconductor lasers was not there by design and was due to the nature of the p-n junction. A fortunate accident. appreciating its origin and importance was probably the first step in recognizing the potential of wave guiding in what will emerge as the field of integrated optoelectronic circuits (or Integrated Photonics) there and do We perform an experiment that proves it. That was a key experiment. And semiconductor lasers, by then, nature guide light the difference of the index of refraction. There were a lot of physics in understanding the propagation of light and its manipulation in crystals. And then, nonlinear optics comes on the scene a little later, which is all about on exchange of energy between light waves and crystals and exchanging information. Probably the most interesting batch of laser physics is nonlinear optics.
ZIERLER: I'll flip the question. We talked about optics absent electronics. What about electronics absent optics? What did electronics look like before you merged them?
YARIV: Well, electronics are doing fairly well without optics to this day. You hear about the chip shortage nowadays. Well, the chip shortage is a shortage of, essentially, electronic circuits, which do everything. Memory, storage, amplification, switching, logic operations. All using electrons. Electronics was already one of our major industries. No doubt, very important. And supremacy in electronics was considered a key aim of the US. Companies like IBM, GE, Texas Instruments. Electronics was OK. It didn't need optics. But optics needed electronics. I think now, electronics will need optics. It's clear. And what will do it is the ability to manufacture the whole thing, optoelectronics including lasers, lenses, optical components, by the same techniques, photo lithography used to make electronic circuits for mass production. That will happen. It's beginning to happen. And we proposed that in that. Proposed it in Japan, repeated it again in a talk. And, you see all the evidence, and the first demonstrations of that new technology will then appear.
ZIERLER: What year was that, the year of the talk in Japan?
YARIV: Around 1968, plus or minus a year.
ZIERLER: Why was it important to do this in Japan?
YARIV: Because the venue was that of the annual International Semiconductor Conference, which is mostly basic semiconductor physics and a little bit of transistors, and so on. And I was already working on semiconductor lasers here at Caltech at the time, and it was a natural place to present my ideas. I think the problem at that point was mostly semiconductor technology and physics.
ZIERLER: Was this more of a eureka moment for you, a gradual realization that optics and electronics needed to be merged?
YARIV: Probably a eureka moment.
ZIERLER: Do you remember it?
YARIV: I couldn't recreate it. I could fake it. [laugh]
ZIERLER: But it was sudden?
YARIV: It was sudden. Carver Mead might have had something to do with it, but indirectly. I was working very heavily on semiconductor lasers because they were small. And semiconductor lasers are essentially like a transistor in many ways. And you connect a battery to it. And there comes light. And it was already understood that that process converting electrical energy to light energy could be very, very efficient. Non-laser experiments have shown that, like making light emitter diodes, the process was efficient. You could also convert the full energy of the electron. In a semiconductor laser, an electron is fed into a crystal in a high-energetic state in the conduction band and makes the jump across the energy gap, forbidden gap, to the valence band and gives you off the energy as a phot- light particle. I was already working in semiconductors. And the crystal of choice was gallium arsenide. Transistors were made then, and are still today, on silicon. Carver Mead was one of the few who made a transistor in gallium arsenide.
ZIERLER: Why gallium arsenide?
YARIV: People learned how to make gallium arsenide of high quality. It was available up to a point. Not like today. I was working on gallium arsenide and learned that you could make a transistor in semiconductors, and we were already making lasers using them, so the idea of why not put them on the same, GaAs crystal in retrospect, seems an obvious thing to do. But at the time it was a eureka moment.
ZIERLER: In putting these two fields together, obviously, there are numerous challenges. Let's start with the theoretical challenges. There's obviously no theory guiding you, so what are you relying on to give you this intuition that this is going to work?
YARIV: We knew how to make lasers on a semiconductor. This is a single crystal of gallium arsenide. It's not that big. And we knew that we could make a laser here, feed a current into it, and light would come out. We knew and we had already shown we could guide the light in GaAs. Well, a transistor can act as a switch. Why not make a transistor here? And feeding the current into the laser, the current can be turned on and off by an electrical signal in the transistor, and in the process, we modulate the light. Here's a way of putting information from an electrical signal, ones and zeroes, let's say, modulating current directly into a replica of light pulses. That's the essence, basically.
The major building blocks of that whole technology, and I mentioned three of them there, I can add a fourth one. Current comes from the outside. Current comes from electronics. And that will be the way to do it for a long time. Information is carried on a current as modulation of the current, AM or FM or digital and if that current is fed to the laser the information is imposed on the light as light modulation. The major building blocks are the transistor that modulates the current, a laser that emits light as a response to current, and then guiding it, and then maybe one or two others, but those are probably the major building blocks of an optoelectronic technology. And I'd been working on that one for two or three years at that point, and that one, and then I read somewhere that one can make transistors in the same material on which I'd already been working for a few years. That was the moment in which I thought, "Why not try it together?" I wrote a paper or two on that subject and got the money from DARPA.
ZIERLER: I asked you about the theory, now what about the technology? What were those technological challenges?
YARIV: In traveling wave tubes the high frequency electronic wave to be amplified propagates around the helix and is amplified by the energetic electronics moving close to it. And we were probably with lasers in semiconductors because you have to learn how to grow layers high quality layers of controlled chemical composition. Same technology which was used in silicon. We had to learn how to make it in gallium arsenide, control its properties' dimensions. Forming the waveguides in gallium arsenide is difficult. And we had some ideas of properties of gallium arsenide can be used to make–my three or four years preceding coming to Caltech and deciding that was going to be the center of my research here, we acquired basic but early sufficient information to teach us how to make the lasers, how to make the waveguide. And now, I think, Carver must've had some background in making lasers in gallium arsenide. But people were now making them. We were the first university anywhere to make semiconductor lasers. There were very few industrial labs that could make them. We were very early in the game.
And the people who taught us how to make them were Israelis. Of course, we took off from that. In fact, to understand the lasers, we learned how to make them, then understood the importance, and I repeat it all the time, of waveguiding. No waveguiding, no lasers. No nonlinear optics. Light has to be guided. When it's guided, it's also concentrated, so nonlinear effects become large. In one of the papers I gave you, you'll see pictures of those elements, combining a circuit. That's the proof you can guide light. That's Bell Labs, 1961, give or take. And here is the world's first integrated optoelectronic circuit, or photonic. [laugh] Here's the laser. That by itself would've been a good laser of that era. And current would be fed directly into the top. Here, the current comes from a transistor. Here's a transistor, and here's the laser, and everything here's a single crystal. It looks like many. If you look microscopically, the whole thing is a single crystal.
ZIERLER: So that's just a representation. It doesn't look like a bunch of rectangles.
YARIV: Yes.
ZIERLER: Who were these Israelis? What was the connection?
YARIV: RAFAEL is an acronym. It's the major R&D development of the Israeli Army. It's north of Haifa, about 10 miles or so. A few thousand people.
ZIERLER: Were you involved at all with them when you served?
YARIV: No, I was not. I was in my second year at Bell Labs when the announcement of the first laser came out about the ruby laser, demonstrated by Theodore Maiman at the Hughes Research Labs in Malibu. But Bell Labs was very fast in replicating the experiment using a ruby laser. And I got an invitation from the Hebrew University to come and give a talk in Israel on lasers. It was the first lecture in Israel on lasers. I received permission from Bell Labs to carry one of the book-sized ruby lasers to Israel to demonstrate it in my lecture. And there were people there from RAFAEL, of course. It was in an auditorium at the campus of the Hebrew University in Jerusalem. Maybe 1,000 people. Very difficult to get in. They refused to let me in, I looked so young. When I told them I was the lecturer, they didn't believe it. [laugh] Anyhow, my father was there. It was very important. And RAFAEL was there, so they knew about me. But obviously, I couldn't work for them. And then, a couple of years later, they had started an effort to learn how to grow some gallium arsenide lasers, that early, in RAFAEL.
And even made some lasers. And two of those scientists were looking for a place to spend a sabbatical, which they earned. And they wrote me, and it was a match made in heaven. Two of them came to Caltech, spent two years, and taught us how to grow the gallium arsenide and make simple lasers. Today, it's done by other technique. But then, it was the only way of doing it. And our lab was the second university in the world, the first was the Technion in Haifa led by Margalit, to make semiconductor lasers, and only three or four industrial labs.
ZIERLER: What were DARPA's expectations of you? What did they want to see you do?
YARIV: Not much. Really, this was entrepreneurial at that time. They gave the individual program managers so much leeway, and they never asked for anything. Of course, our work started making waves. [laugh] And they were satisfied by being part of it, getting the credit. There was never any expectation. And the program manager supported us throughout his whole career in DARPA. After he left, of course, we had to deal with other people, and DARPA changed. They became more about being bookkeepers and probably less daring or risk taking than they used to be in terms of great ideas like the internet.
ZIERLER: As you say, when your work started to make waves, how so? Who was paying attention? What was interesting?
YARIV: Everybody. At some point, it was clear that lasers would be important for communication. Communication, coast to coast, was still done by means of microwave repeaters, huge parabolic antennas on hilltops spaced some 30 miles apart coast-to-coast, transmitting microwave signals, amplifying them in between using traveling-wave tubes. And that was the first coast-to-coast, long-distance communication. That was the state of affairs in 1960. Then, the first proposal to use lasers, but not semiconductor lasers. They needed a transmission and taking advantage of the shorter wavelength of light. They considered, essentially, long tubes, maybe a few inches in diameter, with lenses, so the light beam would be refocused. These are the tubes, and the conventional lenses, and a light beam will do something like that. Coast to coast. And amplifiers in between.
And then, of course, somebody came up with the idea that you could use optical fibers, A newly graduated PhD, Charles Kao at University College London (who later got a Nobel Prize for it in 2009), and Corning demonstrated optical fibers with a loss of 20 decibels per on kilometer, or in plain English, a loss of 99 percent per kilometer in 1 km which was considered a great step. All the other approaches were abandoned and they started working on fiber communication systems. And it was clear now that the semiconductor laser was the light source for the beam carrying the information. Fibers had the minimum losses, the optimal wavelength at which the absorption in the fiber was the lowest, which enabled you to go longer distances. It was about 1.5 microns, which is infrared. The semiconductor lasers were the best source of light for optical fiber communications. All that information is going, and major labs are beginning to do that. At Bell Labs, the semiconductor laser was becoming very important. And we are, at first, the only university that works on the light source.
But the semiconductor lasers, and we are jumping ahead a bit, emit–if you took the light emitted by a semiconductor laser and passed it through a spectrometer, you'd find five or six different wavelengths. A semiconductor laser is like a piano, where you can hear the first harmonic, second harmonic simultaneously when you strike a chord. Anyhow, you can hear many modes. And at first, that's no problem. you can live with it. But as the transmitted data rate increases from 100 megahertz, to 500, to a gigahertz, the fact that the laser emits simultaneously more than one wavelength rather than a single wavelength puts a limit on how far we can go. Because each one of those wavelengths, propagates at different velocity. Group velocity, the magical word. Remember, you communicate with ones and zeroes each requiring a band of optical frequencies to make it. That's what you are transmitting: groups of frequencies (you will find the basic Fourier math in my texts).
But each one of those pulses is made up of all the different wavelengths, each propagating with its own velocity. The result is that nice rectangular wave shape widens with distance. Eventually, they begin to overlap, and the distinction between ones and zeroes is lost. That becomes the limit on the data rate. And they also understand that that's due to the multi wavelength. The cry goes out from the engineers at Western Electric, which is the arm of AT&T that makes equipment, makes lasers, to the research lab at Murray Hill, "We want lasers of one wavelength." Of course, there's a major effort to make it. And Bell Labs comes up with a solution involving two big boxes full of optics and electronics to solve this problem, and they called a major press conference in New York. And we saw very same semiconductors that we are making, but with a difference in growing the layers.
The whole thing is, you need a magnifying glass. One of the interfaces is wavy. And that forces that laser to emit only one wavelength. And what determines the wavelength is the pitch, the distance here, the period. That's all described in detail in the articles. So we win the race. It's a major race. When Bell Labs invented a two-box solution, tabletop size, they announce and hold a press conference in Manhattan, which is advertised as the first press conference since the invention of the transistor. It's that important. When I saw those two boxes, I said, "That cannot survive."
ZIERLER: Why not?
YARIV: We're talking about electronics. We already have transistors and so on. But independently of that, in addition to making semiconductor lasers, we have a research effort that is theoretical on how light propagates in periodic structures. And this is a periodic structure. And we analyzed purely theoretically what would happen if you took a fiber as an example and made it wavy. Make some property like the index of refraction. As a result, it begins to act as a filter. It will only allow certain frequencies to propagate and not others. Every laser needs a waveguide, a feedback mechanism and gain (amplification of the light). In a way we knew the basic idea of wave propagation in periodic structures already. The mathematics is similar to the electron wave functions in crystals which are periodic structures, and act as filters to the electron wave function. In crystals you grow the layers, interrupted the growth. It's done in a furnace. And using photo lithography to Corrugate the surface, make it wavy, and then put it back in the furnace and finish the growth of the remaining layers. And you've got a semiconductor distributed feedback laser.
We made it right here. We had to go over to an electronic company in east Pasadena, who taught us how to do photo lithography. The period (the distance between two peaks is roughly 0.5 microns, very small). We do photo lithography. We take another laser, emitting short wavelengths, which could be a violet or slightly ultraviolet, and shine it on a thin layer of a photosensitive (light sensitive) which I coated a crystalline surface. And when you have two light waves meeting each other, or two microwaves, you get standing waves. And you can control the period by controlling the angle. So we found a UV laser for that purpose and learned how to corrugate the surface and how to control the wavelength. The lasers emitted one wavelength equal to twice the corrugation peak to peak distance. It's a key technology. Used until today to make lasers for optical we didn't know yet how to fit current, so we pump it, we excite it, and out comes one wave. The same without corrugation has many waves.
That was our solution, and it blew Bell Labs out of the water completely. Got to a point when the researchers know now that you can make a semiconductor laser without mirrors, without boxes. They demanded it. But Bell Labs didn't know how to make it. The team that made it at Caltech was two or three graduate students and a Japanese post-doc. Actually, he was a pre-doc, but a Japanese scientist who was here for a year. And he went back to Japan and convinced his company to make those lasers commercially. The first commercial lasers.
ZIERLER: And what year was that?
YARIV: The talk I gave in Japan was 1971. Active integrated optics, active meaning transistors. Nakamura is the Japanese, Garmire, Somekh, Israel Ury, Yariv and Garvin. Optically pumped gallium arsenide waveguide lasers with a fundamental 0.11 corrugation feedback. September 1973, the first demonstration. Probably I can give you photos of the very first experiment, show you that feedback principle.
Communications Potential in Optics
ZIERLER: When you said that you recognized the possibilities for communications, what was the infrastructure that was available?
YARIV: People at Bell Labs and others recognized the importance of semiconductor lasers for communication, mostly because of size and efficiency. And maybe this leads to modulator.
ZIERLER: My question is, what's the infrastructure? You have the technology, but you need an infrastructure built to support the technology. What was lacking? What needed to be built in terms of infrastructure in society to make this feasible?
YARIV: The ability to grow high-quality, multi-layer semiconductors. And then, using photo lithography, carve out different devices is the key. Even today, the technology is very important, very difficult. To make layered semiconductor structures today, at a university, is a multimillion-dollar effort. And we required special convincing of Caltech to give us the money to do it. The growth of crystals, the optical equipment to measure the properties of the lasers, but I would say the biggest hurdle was semiconductor-controlled crystal growth. Controlled both in dimension and in the properties of the layers, And the ability to then fashion it into devices by photo lithography. Same techniques which are key today to integrated electronics. When you read about the shortages in electronic chips, about one or two bottlenecks, all the advanced electronics in the world, 80% of it is made by a company in Taiwan, and the remaining 20% by Samsung.
And they all depend on photo lithography. And for that, you need short wavelengths with at that time probably the wavelengths in the visible part. And that's all done by one Dutch company. And installation. The Dutch company sells a system maybe for $100 million. You are talking about very expensive, probably the most advanced of our technologies. And we are essentially grappling with trying to build those very simple systems at Caltech in the 70s. And we do.
Science and Shower Thoughts
ZIERLER: We'll return to growing crystals in our next talk. But my last question for today, to bring the conversation into the 21st century, tell me about the epiphany you had in the shower, looking at the way that the water was behaving coming out of your shower head. Tell me about this current research interest of yours.
YARIV: [laugh] Well, it's not a research interest anymore. I think the research is mostly done. Well, at the start of the COVID-19 pandemic, I spent a lot of time at home. And it was also a very warm summer in Pasadena. I was taking a lot of showers to stay cool. [laugh] And the shower that I use at home consists of a shower head, a big circular disc at the end of a long, flexible tube. Flexible both in its ability to vibrate left and right like a pendulum, but also in the ability to twist. If you twist it, it snaps back. It's capable of performing two dances, the pendulum dance and a twist. And it can be fixed to the wall, so it acts as a normal shower, or it can hang loose at the end of the fitting tube. And the day in question, the eureka day, I'm taking a shower, and it's hanging down.
I turn the water on, and the thing begins to dance, go all over the place, hitting the wall. Had to restrain it so it doesn't chip the wall. And then, cutting the water down, and finding out that the process is gradual. If I turned the water on, and I tried to excite the motion, nothing happened. It would vibrate, and then it would die down. But at a certain well-defined threshold of water pressure, water force, the things begin to oscillate, twist, and vibrate slightly. And trying to understand what was going on there, I probably have spent hundreds of hours over the last year and a half or so. That probably took place somewhere in May or June of 2020.
ZIERLER: What was the shower head telling you?
YARIV: The key thing, when I turned the water on, if you imagine your shower head whose surface hangs next to a wall. The wall is maybe five inches away. It's hanging down next to a wall. When water first comes out, the reaction force, water goes forward toward the wall, which is only a few inches away. The reaction force on the shower head pushes it away from the wall. As much as a foot. But at the same time, it also twists a bit. Because I guess the distribution of holes from which water emerges is not uniform, so more water's coming out of the left side. The result is, there's more force on one side. In addition to being forced back by the reaction force of the water, like jet propulsion, it twists. And it'll stay there, with the water on, away from the wall, steady state and twisted. And you increase the water flow even higher and give it a slight nudge left or right, it goes into the joint tango.
ZIERLER: The twist and the pendulum.
YARIV: Exactly. At the time, I was not thinking I'd discovered a new phenomenon, but very early, I learned that the two had to be there together. If I had, with just the touch of a finger, stopped the pendulum oscillation, the twists stopped and vice versa. And I've spent all my scientific life, to a point, learning about waves, resonances, and quantum mechanics. There are two natural modes of vibration here of a system. One is the twisting oscillation, the other is the pendulum. Each one can exist on its own, theoretically. Once you nudge a pendulum, it'll go on for a while. Eventually, the friction or the air resistance will kill it off. Same goes with the twisting. If you had a body at the end of a coiled spring, and you twist it, it will twist back and forth. The mathematics are identical to that of the pendulum, the restoring force.
You have a system now with two natural modes of vibration that theoretically can exist all by themselves. And here, they obviously exist simultaneously and require co-oscillate to exist. And water. The energy source is obviously the water. And it was also clean [laugh]–that each one of the modes excited the other. Well, it was almost immediately obvious why the twisting generates in the presence of the water reaction force excites the pendulum motion. And you will see it in the differential equations. What is not clear is how the pendulum vibration excites the twist – it is not at all obvious. But my crazy mixed background–you'll see in my text, I have a chapter on parametrics. An English physicist, Lord Rayleigh, maybe 150 years ago or so, proposed that if you can take any vibrating system, like the pendulum, or any electronic RLC circuit, or a twist oscillator, and modulate–so you have a system that can do that without friction a long time, and that requires a force.
You always need a force, like a mother in a playground, pushing her son. But if you can modulate a parameter of the system, like in this case, its mass–imagine you can modulate the mass. Or modulate gravity. And modulate at twice the natural frequency than the thing can be set into oscillation as well. And that's another way of exciting oscillation. And that's parametric. And that's Lord Rayleigh. I knew that very well. I have a chapter in my textbook. I found it fascinating. The first question I needed to answer was, how does that motion excite that one? Well, maybe parametrically. Parametrics require that that motion will modulate some parameter involved in the twisting. And that's the twisting spring constant. If you have a spring, and you pull it, the force it tries to bring it back is proportionate to a spring constant times the elongation.
In this situation, there's a torsional spring here. When you turn it, the spring tries to bring it back, and the constant relating the angular displacement to the force is the twist spring constant. The idea is that this oscillator, which depends on the springiness, which returns it back, and that depends on where it is in pendulum cycle. As it oscillates, it does that. But Rayleigh had shown that you needed the modulation to be at twice the frequency. Where's the twice? That is generated by a nonlinearity in the parametric relation, Simple trigonometry. That is the basis of nonlinear optics nonlinear optics. I had already done the quantum analysis of nonlinear optics. Nonlinear, meaning the spring constant depends not only on x but on x squared. And when an optical wave propagates through a crystal with a second optical nonlinear coefficient some of the wave energy is converted to a new wave, which oscillated at twice the frequency. We call this second harmonic generation. The conclusion is that oscillations that cannot be excited on their own can be excited if they are will to collaborate. Like Argentinian tango! If you have a nonlinear element, which responds not only to the first power of an input but to the second power, and if you feed in cosign omega t, you're going to get out a DC term 1 plus cosign 2 omega t. You double the frequency. If the property of this twisting, the decay, depends on displacement, it's a nonlinearity. Usually, the k is a good number. If you do what I've done here, you see that if you now modulate the displacement at omega t, the force will be at twice the frequency. That's the basis of all of nonlinear optics. The twisting oscillation excites the pendulum, and the pendulum excites parametrically the twisting. And the source of the energy, the power, is the water.
ZIERLER: And as far as you know, this has never been observed before?
YARIV: It's probably been observed but not understood before. [laugh]
ZIERLER: Are you going to write this up?
YARIV: Oh, yes. Oh, of course.
ZIERLER: Wonderful.
YARIV: I consider that fundamentally very important. Because nature is full of those frustrated dancers waiting for partners and once they find them go crazy and bring down buildings, bridges, the Tacoma Narrows bridge maybe. It would be too much to ask that the shower head on 480 Madeline in Pasadena is the one I've actually done the theory completely, and I have to write it. I have a big file. [laugh]
ZIERLER: And all it took was a hot summer during the pandemic.
YARIV: Exactly.
ZIERLER: That's a great place to pick up for next time.
YARIV: OK.
[End of Recording]
ZIERLER: OK, this is David Zierler, Director of the Caltech Heritage Project. It's Tuesday, November 2, 2021. It is my great pleasure to be back with Professor Amnon Yariv. Amnon, good to be with you again.
YARIV: Pleasure is mutual.
ZIERLER: Today, I want to discuss the intellectual, technological, and engineering origins of semiconductor integrated optoelectronics. And for that, let's go all the way back to Berkeley, perhaps as far as back as undergraduate or graduate. What is your exposure to the origins of what this field would become while you were a student?
YARIV: Well, Berkeley really enters in a fairly minor way. I probably told you the story about halfway through my graduate research, I stayed in Berkeley, which puts it roughly 1958, give or take. In switching over from EE to physics, I took a lot of courses, and among them, solid-state physics, taught by Charles Kittel. Good class. Studied about Bloch's theorem, solid-state physics, energy gaps, my exposure to semiconductors was simply in the classes I took. I simply had to cover the physics curriculum, which I did in about a year.
ZIERLER: Did you interact with Marvin Cohen at all at Berkeley?
YARIV: No. Mostly with Kittel. Also, an experimentalist, Professor Irwin Hahn working with Kittel. A bunch of solid-state, magnetic resonance types.
ZIERLER: What was Kittel doing at that time?
YARIV: I don't know really because I just took the class from him. I think he was doing maybe his most important achievement. To me, it was known, mostly, to people he wrote a very good book. And the fact that he would come every few years with a new edition, which was worse than the last edition. [laugh] The first one was the best. I was introduced formally to solid-state physics, took the class, but did little or nothing with it for the remainder of my graduate research at Berkeley. What I did was make a maser. And then, a two-level optical laser.
ZIERLER: Two-level means what?
YARIV: A laser works on having two levels, charcoal two and one. Those are two energy levels of an atom, electron, among a lot of other levels, and a ground state, the lowest level. And somehow, you've managed to establish populations N1, N2 and of atoms in the three levels. And if N2 is bigger than N1, and you can achieve it by selective pumping into the levels and taking advantage of relaxation times of the electrons in each level, if this difference is big enough, the material can act as an amplifier. Light propagating through the crystal, if it is a crystal, will be amplified. That's a three-level system. Often, there's another level here, and the pumping mechanism the ground state, zero, to level three, and then they relax very quickly.
It's a way of populating the upper level. two-level. You still have to satisfy the condition N2 bigger than N1. It's called population inversion. That's a condition for gain. I use the word gain and amplification interchangeably. But that's done by a process called adiabatic fast passage. It turns out that if you have a normal state, atoms, electrons, will occupy the lowest available state, ground state. Level zero. But in adiabatic fast passage, for instance, those two levels could be the two split spin states of an electron in a magnetic field. In a magnetic field, and the electrons spin up, spin down, the two states will separate in energy by an amount equal to the magnetron times the magnetic field. You apply a magnetic field, you take a level, which is doubly degenerate, and split it, spin up, spin down, and those become the two laser levels. And of course, the task now is to transfer all the atoms from here to here, and that's done by a process called adiabatic fast passage. Very important process. While keeping the magnetic field down, you vary the microwave field that connects the two quickly, and the result is to exchange population.
ZIERLER: Did you understand all of this as a graduate student? Or what you're telling me now is retrospective, what you came to understand?
YARIV: No, that's my thesis. I have a copy of that somewhere. I did not invent adiabatic fast passage. You actually can read about it in a very good textbook, Schiff's quantum mechanics textbook, which was my textbook. That was known. But applying it to obtain inversion in a laser was done by the two scientists, Feher and another scientist, at Bell Labs who gave the talk about Boulder that essentially converted me.
ZIERLER: When was this talk?
YARIV: 1956.
ZIERLER: Were you there?
YARIV: Yes.
ZIERLER: What was the excitement? What did they have to say?
YARIV: I told you about my graduate advisor, Professor Whinnery, and I driving from Berkeley to Boulder. 1956, I had done my master's thesis on an area related to John Whinnery's research, traveling-wave tubes. I decided that I did not want to continue doing my PhD in that field. It was understood I would do a PhD with John Whinnery, but that was his field. And he suggested that I come with him to an annual meeting, called the Tube Conference at that time, because the people there who started it were the leading experts in traveling-wave tubes. But the meeting was more eclectic. It hardly dealt with traveling wave tubes. All kinds of interesting people, like from Bell Labs, came and gave talks.
ZIERLER: And why Boulder? Was NIST there already?
YARIV: No, they moved around, every year in a different place. NIST wasn't there yet. It was still the Bureau of Standards in Washington. That's where I heard George Feher, the Israeli who got a PhD from Berkeley then went to Bell Labs, and then invited me. I spent a couple of weeks with Feher showing me how to do the basic equipment and experimentation is that of paramagnetic resonance, energy levels of spins, nuclear or electron spins, in magnetic fields. And now, you can do spectroscopy. MRI is based on that process and the laser, which they described. But it was a pulsed laser. You inverted a population, you observed lasing, and had to do it again. But it established the principle.
ZIERLER: Was Norman Ramsey already in on his NMR work at this point?
YARIV: Yes. That was a microwave maser, not a laser. But the principles were the same. The theory of lasers is very much that of microwave resonance.
ZIERLER: Let's focus on that a little bit right now, the mid-1950s. What are the theories that are guiding laser research at this point? What are the big hypotheses or theories?
YARIV: The maser is around already. Meaning like a laser, but oscillating, emitting at microwave frequencies, not optical, which are about a factor of 10,000 smaller than optical frequencies. And the main interest in the maser is low-noise amplification. Amplifiers that add as little noise power as possible to the weak signals which they amplify. In 1968, possibly, there's a paper by Townes and Schawlow about the possibility of obtaining maser action at optical frequencies. Before that, there is no work, really–maybe some in Russia–on the laser, the optical version. The talk I heard was about the maser, the two-level. And the principles were essentially the same as of magnetic resonance, same theory about the difference of N2 is bigger than N1, ordinary resonance, magnetic resonance. MRI, N2 is smaller than N1. So I went to Berkeley and essentially built a system.
ZIERLER: You loved this, this was what you wanted to work on.
YARIV: Well, I made a decision. Yes, I liked it because it used physics. EE as a major didn't satisfy me because I don't have a good memory. Never did. Also, the way you learn, even in high school, was in systems, the principles. And physics was simple. There are the Maxwell Equations for wave propagation and confinement [laugh], the Schrödinger equation for the atoms and that's it. You didn't have to remember too many things. In elementary school, I almost flunked math because I did not get the principle. [laugh] I don't think I'd ever run into anything as elegant as the Maxwell equations, quantum mechanics. It's all a few equations. So yes, I decided to then do my PhD in that field.
Bell Labs and Returning to Berkeley
ZIERLER: Now, the talk, with them coming from Bell Labs, were they thinking about applications at that point? Or this was all basic science?
YARIV: They were in the basic research department. George Feher was in the solid-state research department, working actually on semiconductors. No, they were not thinking about applications, not at all. Oh, yes, the second person was Harold Seidel. A good solid-state guy. And he was more of the engineer of the two. George had graduated from high school in Haifa, Israel, born in Hungary, and when he graduated from high school, he wanted to study at Technion. They wouldn't accept him because his Hebrew wasn't good enough. He flunked it. [laugh] He went to Berkeley. Became world-famous.
ZIERLER: So you come back to Berkeley. What's the plan? First of all, what instruments are off the shelf, and what do you need to build yourself to make this work?
YARIV: There's hardly anything off the shelf. And what's more, things which I could've bought, like a magnet made by Varian Associates in Palo Alto. I think it cost $10,000 even then. Big magnet. We didn't have the money, so I made it.
ZIERLER: What were your funding sources? Who funded the research?
YARIV: John Whinnery.
ZIERLER: He had an NSF grant?
YARIV: I wasn't familiar with his economics or finances, but he had a group of maybe seven, eight graduate students, and that supported the research. He was also very well-connected. He was really prominent in the field of microwave traveling wave tube amplifiers, which was important. John Pierce was deeply interested, and a bunch of other very good people. And they were so prestigious that the annual tube conference attracted some very, very good people. He supported me. I didn't ask him where the money came from. But it meant that a lot of stuff that could've been bought, I built. The sample was in a microwave cavity inside a small metallic box was at liquid helium temperature. I actually built the transfer system. I remember looking up tables of tubes with below thermal conductivity [laugh]. But I had some good support.
ZIERLER: Tell me about building the transfer system. How do you go about doing that?
YARIV: You get the helium. The Physics Department, which was a building away from my building–I was in Cory Hall, which is the western edge of the campus at Berkeley–and they had a setup for making liquid helium. But they did not deliver it. I bought a big one on wheels. They come on wheels because they have to be pushed all around the building. And I had it filled maybe once every two or three weeks in the Physics Department, had to pay for it. And they pushed it, for the space, maybe 200 or 300 yards between the buildings to my lab. It was made by the glass blowers in our building had liquid nitrogen outside as an outer jacket around the liquid helium, and then there was essentially a bottle within the bottle. And the inner bottle had the helium in it.
ZIERLER: Why did you need the outer jacket?
YARIV: To simply keep the liquid helium. If you just poured liquid helium, the heat conductivity through the glass for the top, the air, whatever, will make it evaporate in a few minutes. Natural liquid helium at atmospheric pressure has a boiling point of 4.2 degrees kelvin. The room temperature difference in degrees kelvin was about 300 degrees. And it's expensive. And liquid nitrogen is about 78 or 80 degrees. It pre-cools it. Liquid nitrogen is relatively inexpensive. You pre-cool it, and then it can last maybe a few days. But of course, you have to transfer it from the storage tank on wheels into the inner tube. While the helium is being transferred under pressure, it can also boil off. I had to look up a list of metals or alloys with very low thermal conductivity so that while the helium is transferred, it doesn't get warm. Became an engineer learning about heat transfer, how long helium will last, and so on.
ZIERLER: Were there safety precautions you needed to consider for this research?
YARIV: No, because neither nitrogen nor helium are dangerous. Nitrogen is in the atmosphere, and helium is inert. So no. But there were some experiments later where I used liquid oxygen, and at Caltech when I first arrived, I used liquid oxygen, and that was tricky. Because that's explosive. Out of the two years, 1956 to '58, I probably spent much of it building equipment with help. John Whinnery's group, fortunately, was big enough, and his finances were good enough, that there were two people in the group, one was a microwave engineer type, and the other was a mechanic who could make anything work. He was very crucial. And they helped. It took about a year, year and a half to build the equipment.
ZIERLER: We didn't finish on the helium and the transfer system. What's the next step? You get the helium…
YARIV: Well, I will actually show you my PhD thesis at some point, and you'll see photos I took myself of my equipment. It is essentially two evacuated dewars inside each other the outer containing liquid nitrogen and the inner one liquid helium. The whole contraption rested on wheels which I wheeled every few days to here, you have liquid nitrogen, and in here, liquid helium. And here's the tank with the wheels with liquid helium, which I obtained in the Physics Department. They filled it for me, and I wheeled it over to our building. And the transfer system, simply a transfer tube made of low thermal conductivity metal. Apply pressure on the helium, and helium as liquid will be transferred. Into the inner volume bathing the crystal the laser This was already pre-filled with nitrogen. The liquid helium is contained in a big thermos bottle, a dewar, which as been pumped out (evacuated) glass jacket and is filled with liquid nitrogen, a similar but smaller dewar is placed inside the bigger dewar and the liquid helium is transferred and stored in this second, smaller dewar. It evaporates quickly, in a couple of days, so the experiments have to be performed quickly. That's the cryogenic part of the system. Let's zero in on this part right here. Here, you had essentially a metallic can, and the crystal was here.
ZIERLER: What's the crystal?
YARIVL: The "defect" i.e., the change locally crystal structure in the crystal in that it is s an interruption of the perfectly periodic, repeating structure of the crystal. The defect acts as an atom with a little magnetic dipole which can point up. Or down. I had to buy the crystal, send it to the nuclear facility in Washington state to be irradiated. All the details are in my articles of that period and the thesis, which I'll lend you a copy. Here, there was a whole rack of electronics and other things. The result is, from that box, you got microwave radiation coming out into the metallic waveguide. And this box acted as a microwave oscillator. And in particular, we used a pound-stabilized microwave oscillator. This was a resonator, essentially a metallic can, like a soup can. And it had resonance at the frequency that we were investigating. Like a piano string vibrating in resonance when struck so that there is exactly an integer number of half wavelengths along its length and that determines the frequency of vibration. So the defects are in the Mgo crystal inside the resonator, (can) cooled down to 4.2 degrees Kelvin in a magnetic field. Each defect is like a little magnet pointing against the magnetic field of the big magnet. We used the microwave and extra electronics to perform an adiabatic fast passage which flips the direction of the defect magnets which in the process gains a fixed amount of energy and because they are situated inside the high Q r(low loss) metallic can (resonator) they flip to their original position and in the process give back the excess energy as a pulse of microwave radiation. That what the Maser does. The threshold, the number of defects that you need to invert to get oscillation–the crystal was here, and I told you that we had obtained this condition called population inversion because N2 is bigger than N1. Normally, it'd be the opposite. That's an abnormal condition. To obtain it, we had another box generating, at times, a very magnetic field.
ZIERLER: Why is that an abnormal condition?
YARIV: At thermal equilibrium, low level–t's a Boltzmann distribution. Thermodynamics. In equilibrium, N2 of N1 is equal to E. You have two levels separated by N energy. Delta E. Energy separation. In our case, the separation is brought about by a magnetic field. And it's proportionate to magnetic field. You have two levels, meaning they have the same energy but different function. There are two different energy levels. And they separate in energy when you apply a magnetic field. And that separation is proportional to the magnetic field. And you subject them to a microwave field, the frequency omega. Resonance is when delta E is equal to E2. Those are the energy two levels. E2 minus E1 is equal to H bar omega, where H bar the Planck constant. This is the microwave resonance condition.
You turn on the magnetic field, and then this microwave tube, the high Q, is designed to resonate at a given frequency. And you tune the big box here to emit a frequency equal to that frequency, which resonates. And then, you turn the magnetic field on until this condition is met. Then, you are in resonance. Meaning that the magnetic field separation of the two levels from here to here is equal to H bar omega. Or the microwave photon energy is equal to H bar omega. MRI was called initially nuclear magnetic resonance. People didn't like the way nuclear sounded. It's basically the exact same principle, except that the two levels here are not electronic spin levels but nuclear magnetic normal. But it's the same thing, same theory.
ZIERLER: Was Whinnery involved closely in what you were doing?
YARIV: Essentially, not at all.
ZIERLER: It was beyond his area of expertise in any regard?
YARIV: It was not his area, and he was a pure electrical engineer. He spent the War years in Schenectady at the GE Research Lab with Si Ramo, and he wrote this very famous and good book on microwave engineering. And they were involved in microwave engineering. And then, he came to Berkeley and worked on traveling-wave tubes, which, again, were microwaves. He had basically no experience in this area.
ZIERLER: And were you all by yourself with this? Did you have any graduate students who were helping you?
YARIV: I was in the building called Cory Hall, the main building then, of Electrical Engineering at Berkeley. No one there understood what I was doing. But what's worse, the physics professors didn't know what I was talking about. Lasers, masers, you can amplify light–they were just involved in putting in a magnetic field.
ZIERLER: So you were all by yourself.
YARIV: I was all by myself.
ZIERLER: I'm not sure if you know, but Tom Rosenbaum, for his graduate work at Princeton, he spent nearly all of his time at Bell Labs, even though he was a graduate student at Princeton. Bell Labs was the spot for him. That's where he hung out most of the time.
YARIV: It's only 10 or 20 miles away.
ZIERLER: Of course. That made it easy. My question for you is, did you ever want to just be among people who knew what you were doing, which obviously could not be accomplished at Berkeley? Or was it good for you intellectually to do all of this by yourself?
YARIV: I didn't have a choice. [laugh] So I never considered the alternative. There was a professor at Berkeley, Alan Portis, who actually was also in magnetic resonance who closest by his main interests were elsewhere. It is fair to say that nobody in Berkley understood what I was doing or even the fact that resonance is infinitely narrow. John Whinnery really felt bad for me that I was alone. In retrospect I do not think it was a bad thing, I did not have to "educate" a professor about laser physics which as a discipline did not exist yet. It also made the approval of my thesis easier and faster.
ZIERLER: How long did it take you before you realized you had something that was working?
YARIV: I probably spent a year and a half building equipment and learning how to use it. Every aspect of this problem. I needed to build a magnet. This microwave cavity inside the dewar with the sample is placed between the pole faces in a magnetic field of 10,000 gauss, I designed and built the microwave equipment with the help of George Becker and Fred Clap, (the microwave expert), the microwave system I was a major experimental setup. Far more difficult than anything any of my students ever did. I came from an academic high school with very little experimental background, hands on. Building this system was an experience I will never forget it.
Laser Breakthrough
ZIERLER: What were the goals? Not only at what point did you know that this system was working, but what goals did you have in mind to test if it was success, the research was worth it?
YARIV: I think I got a feeling that if I could just get lasing–lasers and masers were so new, and there was essentially almost zero work at universities all anywhere. One exception was Columbia, where Charlie Townes invented the laser, and a superb student of his, eventually my boss at Bell Labs, Jim Gordon, succeeded in making an ammonia maser, again, at low frequencies. With that exception, making anything of that sort, getting laser action at a university–that's basically what I did.
ZIERLER: So just getting it to work was your goal. How do you know when it works? What does it do?
YARIV: When the populations are inverted, when N2, the population of the centers with their spins pointing up was sufficiently bigger than N1 (spin down population), inside the resonator then an incident microwave radiation is reflected from the resonator bigger than the incident wave .You have made a maser microwave low noise amplifier. The crystals are sitting in a field inside a can. The can is high Q. The microwave field from the oscillator builds up a very strong field inside. The magnetic field is swept through resonance. And that flips the populations N1 and N2 . And that's done on the that basis. You apply a magnetic field, and if your things are right, and that's a big, big if, you succeed in inverting the little defect magnets so that more of the N2 point against the big magnetic field than along it N1 – a crystal in which N2 is bigger than N1. And if that happens inside a high Q resonator that was that applying the pulse at the population [level]. All of a sudden, you get a burst of microwave coming out of the can, which I detected and displayed on an oscilloscope. That was it.
ZIERLER: Was that a eureka moment? Did that happen quickly? Or this was a gradual series of experiment and verification?
YARIV: Everything in that experiment required suppressing the ever-present noise. And you had to verify what you saw. There was not a eureka moment, but there was a moment in which I thought that we probably had it. But you couldn't go and make a statement. You couldn't claim success yet.
ZIERLER: What's the mechanism? What are you looking for to know if you have it or not?
YARIV: You invert the population. Microwave theory. Now, this is the resonator. Let's give it a number. This is three. That's an enlarged version of this can right here. And it's inside the liquid helium in the magnetic field. And you now invert the population by the adiabatic fast passage. You wind up in the sample here, magnesium oxide, irradiated with neutron, I think. That was done in a nuclear facility. You have level two and level one. And the inversion means that you wind up in thermal equilibrium, N1 is bigger than N2. If you apply a microwave field to this sample, because N1 is bigger than N2, the microwave field causes a transition from here to here, which absorbs energy, and from here to here, which emits.
And because N1 is bigger than N2, you absorb more than you emit, and the sample is absorbing. If you can invert the population so that when the microwave comes in, N2 is bigger than N1, more energy is emitted by the sample than is absorbed which is a necessary condition for amplification. And if the Q is high enough, it will oscillate. This box will now emit microwave radiation. And it's that radiation which is the sign. Because this experiment is done on the pulse basis, you invert it transiently because eventually, it relaxes back to N1 bigger than N2, and it absorbs again. There's a distinct microwave there, emission of microwave radiation.
ZIERLER: You said you're building the equipment for a year and a half. What's the timeframe to get to this point here?
YARIV: After that, maybe half a year or so, doing experiments, writing it up. I came to John Whinnery one day and I said, "John, I think I've done enough for a thesis." He said, "If you say so." [laugh]
ZIERLER: Were you writing at all at this point? Did you publish?
YARIV: Probably started writing during the last six months. At some point, I was convinced that I had observed maser oscillation.
ZIERLER: Were you in contact with people at Bell Labs?
YARIV: Yes, George Feher and I. Although he was about four years older, we'd become good friends.
ZIERLER: Did you spend summers at Bell Labs at all?
YARIV: No. I was married. I needed to do the experiment. At some point, John had a new graduate student join me. He was a good "electronic-er" and a good experimentalist. He eventually became a professor at the University of Oregon. And he helped me in the last stages of the experiment, taking data and so on. Good fellow.
ZIERLER: On the personal side, in Berkeley, is there a Jewish community, an Israeli community that you're part of?
YARIV: There were roughly, I would say, 70, 80 Israelis, mostly graduate students. A good Hillel at Berkeley.
ZIERLER: Where were you for chagim [Jewish holidays]?
YARIV: Probably at Hillel. Also, married, and my wife was an American, and her family was in San Francisco. They were not religious but celebrated major holidays. Mostly Hillel.
ZIERLER: That was enough for you.
YARIV: I was not more religious to begin with, but yes. It was more of a social occasion. Lots of Israelis.
ZIERLER: Besides Whinnery, who else was on your thesis committee?
YARIV: Singer, [laugh]. And Allen Portis. It probably says so in the introduction to my thesis.
ZIERLER: Was John Clarke there yet? Was he working on SQUIDs yet?
YARIV: No. That was before John. Because my friends in Berkeley at that time were essentially a bunch of EE and physics graduate students. I still belonged to the EE group that assembled at lunch times. Some of the physics professors, like Kittel and others, tried to pressure me to change majors. They said, "Your field is resonance, you're taking all your classes here. Why don't you move over?"
ZIERLER: But you did not.
YARIV: I did not.
ZIERLER: Just because it was easier to stay where you were?
YARIV: It was easier. And John was supporting me. But I felt loyalty to him. And I saw no reason to switch. I think that was equally strong.
ZIERLER: Were you in a race with anybody? Were you aware of anyone else who was doing this research elsewhere, and you didn't want to get scooped?
YARIV: No. I would say that 70, 80% of my research was replicating what Feher and Seidel already did at Bell Labs about two years earlier.
ZIERLER: What was the value in replicating so much of it? Just to see if you could do it?
YARIV: For me personally, learning what was the mechanism, learning completely a new field, microwave resonance. I needed a thesis. [laugh] I didn't have too many other ideas. And I also analyzed the dynamics of the little spins as there were being flipped . The adiabatic slow passage.
ZIERLER: Now, 70, 80% replicating, what's the 30, 20% that's not replicated and was new?
YARIV: Well, I did theoretical work. It's a transient experiment. You have to solve the Bloch equations in the time domain, not in steady state, which was much more difficult. But I would say probably the fact that nobody understood what I did, and everybody was highly impressed. [laugh] But at that time, my experiment occupied maybe 500 square feet in a corner. And it was kept. About 15 years after my departure from Berkeley, my experiment was still sitting there in the corner.
ZIERLER: So you defended. What was available? Could you stay on at Berkeley? Did they offer you a post-doc?
YARIV: About a year or so before I left, John Whinnery was a high-prestige member of the microwave fraternity in the US, mostly in virtue of his traveling-wave tube work, but he was also a unique individual. Very nice and very logical. At some point, he was, I think, offered to be the provost of Berkeley, which he refused. There was a continuous stream of visitors from Bell Labs who took their sabbaticals in our group. Mostly friends of John Whinnery. And during the first three years, I was working on traveling-wave tubes. I got to know those people. They were interested in what I was doing, and that was what they were doing. And essentially halfway through my thesis research in Berkeley, I had an informal offer from Bell Labs. "If you want to work for us, let us know." I knew I was going to do that, more or less.
ZIERLER: And that was more attractive to you than an academic post-doc?
YARIV: Yes, I wasn't thinking of an academic area. Not at all. I didn't think I was cut out to be a teacher.
ZIERLER: And the research, of course, at Bell Labs. This is the heyday of Bell Labs.
YARIV: Yes. The inventor of the transistor, Shockley, was still there. One or two of the engineers who helped Shockley in his group and experiment helped me later on making a semiconductor laser.
ZIERLER: What about more locally, the origins of Silicon Valley? HP, Fairchild? Did you put out feelers there at all? Was anybody in that world interested in your work?
YARIV: There was no Silicon Valley yet. That came later. Shockley left Bell Labs and started a company, Shockley Semiconductor Laboratory, and hired a bunch of good graduate students who'd recently graduated, mostly from Caltech. And it was dissatisfaction with Shockley as a boss and manager that made them quit and start Intel.
ZIERLER: A rebellion.
YARIV: Yes. He was not a good boss. He also fancied himself as an expert on management, and race superiority as well, and that his company seemed to be a model of management, which was a bad idea. They eventually left and started Intel. All of that took place later. There was no Silicon Valley.
ZIERLER: In terms of industrial research, it's really only Bell Labs for you. There's no other place? I'm thinking RCA, GE, none of them were doing research that would've been right for you?
YARIV: By the time I left Bell Labs, which would be in 1964, give or take, lasers already had been demonstrated. And laser research was going on at IBM Research, RCA.
ZIERLER: I'm saying when you defended your thesis, it was only Bell Labs.
YARIV: Only Bell Labs, and the laser had not been demonstrated anywhere. But I joined a laser group. There were three groups at Bell Labs working on demonstrating a laser, each one following a different class of materials.
ZIERLER: What were the three groups?
YARIV: One under the intellectual leadership of Ali Javan, who later was a professor at MIT, making lasers in gases. He eventually succeeded using neon. And then, two other group. In the group I was associated with, were making lasers from uranium ions embedded in calcium fluoride. And the third group was working on ruby, which is the material that Maiman used and had already demonstrated in California. When I joined Bell Labs and joined one of the groups, we didn't know about Maiman, never heard of him. Maiman's bosses at Hughes didn't know he was working on lasers until he made it. We were competing with each other within the bell groups for who would make the world's first laser. But none of us did. [laugh]
ZIERLER: Who were your cohorts in the group?
YARIV: In my group, the boss was Jim Gordon.
ZIERLER: And you were impressed with Gordon immediately?
YARIV: Oh, Gordon was intellectually a giant. Also, a very good tennis player. [laugh] He was kind of an East Coast aristocrat. The people who played tennis on elevated platforms in the winter, played lacrosse in the summers, sailed [laugh] and did not think about moving to California. And a Caltech PhD who arrived about the same time, Gary Boyd. Very good. Came from EE at Caltech.
ZIERLER: Your age or a little older?
YARIV: About the same age. All things being equal, I was maybe a couple of years older because of my service in the Israeli Army during the war of independence which kept me back for two years. And then, a newly minted EE or physics graduate from Stanford, Kumar Patel, who made the world's first, or second, CO2 laser later on at Bell Labs.
ZIERLER: He was not yet famous.
YARIV: Our uranium calcium fluoride laser was the world's third laser. And so, it was three of us in the group: Gary Boyd, a fellow named Collins. Not Kumar Patel. Kumar had his own experiment.
ZIERLER: What was Kumar like?
YARIV: Kept his cards close to his breast. Smart. And a superb experimentalist. I didn't expect it. I thought India was like Israel, people only did theoretical work, reading Sanskrit. He was practical and very good.
ZIERLER: Now, in this group, did all of you work together?
YARIV: No.
ZIERLER: How did that work? Who did what?
YARIV: Well, I know mostly about my group. In my group, Gary Boyd concentrated mostly on the optical pumping, and we did it by concentrating light from a photo lamp light from an intense light source. His specialty was optics, both theoretically and experimentally. He was a talented fresh PhD from Caltech, an EE graduate. Could always fall back on Maxwell equations. And he was responsible for electromagnetic and optics. The laser is essentially a crystal placed inside a light confining and reflecting enclosure which we call an aresonator in a resonator. This resonator and its design are as important to the laser operation as the atomic medium which emits the laser beam. The two are equally important. If the resonator Q is not high enough, inverting the atomic population, making N2 bigger than N1, I call it inverted population, is a necessary condition for laser action, for laser emission, but not sufficient. Because when N2 is bigger than N1, any micro radiation will be amplified by the medium, but it will also be absorbed simultaneously by the imperfect resonator.
No resonator is perfect. And if the losses are bigger than the gain, then it doesn't lase. The inversion has to be big enough to compensate for losses. For a given resonator, there's a critical inversion that you have to exceed to get light out of it, and the higher the Q of the resonator, the smaller the inversion. If the Q is not very high, meaning losses are high, you need to work on it. Gary Boyd, the Caltech graduate, his main expertise and contribution was the micro theory of what goes on inside there. We didn't choose the same resonator, we used mirrors. And he had developed some of the basic concepts in optical resonators. People don't realize that the optics is as important as the atomic physics to make a laser.
Can be equally important. If you read my book, you'll see big chapters on the optics. And Gary Boyd understood it better than most people at the time. He also was clever in designing our system for exciting, shining light and inverting the population. My contribution was more in the atomic resonance, understanding the physics. More or less, the physicist of the group.
ZIERLER: What were some of Boyd's big insights in optics? What was so important about that?
YARIV: Let's make a slight detour because the optics are very important. Without the optics, there's no laser. As important as the atomic part is. Maybe sometimes more important. Of course, we knew that, but none of us really understood optics. I took optics as an undergraduate in Berkeley for one term, and that's it. Knew there were lenses and ray imaging, that was not enough. It is that our optics people at universities and research labs did not understand the need for a different level of understanding based on Maxwell's Equations and hence including diffraction and optical resonators and waveguiding and modes. That knowledge was generated within our group by people like Gary Boyd, Jim Gordon and a superb Austrian recruit From Vienna to group, Herwig Kogelnik. It required a first principles knowledge of Maxwell while the so-called optics ‘experts ‘kept drawing rays and lenses which was not at all helpful. Gordon and I brought in the quantum mechanics.
And what was lacking was the understanding that you had to solve for the electromagnetic modes of your enclosure, of your system, and design a system. And the optical community of the US, thousands of people with PhDs, companies making equipment, contributed nothing. The knowledge came basically from Gary Boyd with a PhD from Caltech and Jim Gordon. And the idea, basically, that if you have two mirrors, as an example, if you solve Maxwell's Equations, which they did, there is a mode, an electromagnetic field configuration that can oscillate and self-reproduce itself, be stable, and which essentially consists of two beam waves bouncing back between two mirrors, and of course, you have to understand the electromagnetic properties.
If you do it properly, if this distance is related to the radius of the curvature, then very little of the energy spills outside. And you have a high Q resonator. Without it, you can't make a laser. That understanding came from Gary Boyd and Jim Gordon in a few classic papers on this. A little later on, a fellow named Herwig Kogelnik, who was an Austrian with a double PhD from the University of Vienna and from Oxford, and the three of them, essentially, established the electromagnetics of lasers to this day.
ZIERLER: Now, you mentioned there were three groups at Bell Labs. Did they talk to each other?
YARIV: Yes, but not much. In a way, they were competing with each other. Also, the approaches they were following were so different that there was not much they could teach each other.
ZIERLER: How would you distinguish the approach of your group from the others?
YARIV: One group was trying to make lasers in gases. That was so foreign to me.
ZIERLER: Different world.
YARIV: Different world. Spectroscopy of gases. Although that was Charlie Townes's original area of expertise. That's why he got into lasers. They wanted stable sources to do good spectroscopy. We were so busy and competitive, and to some degree, we knew there may be a Nobel Prize in the first group and not the second. [laugh] None of us knew of Maiman yet.
ZIERLER: And what was the third group?
YARIV: The third group essentially reproduced Maiman's experiments using ruby.
ZIERLER: Why ruby?
YARIV: There's nothing magic about ruby. Ruby did not survive as a good laser material. But in the beginning, ruby was a well-understood material. The spectroscopy of ruby. Ruby is essentially trivalent chromium atoms embedded into a crystal. There are impurities in a crystal, and the impurities are the chromium atoms, trivalent. And the crystal is some oxide. Maybe aluminum oxide. That's ruby. The chromium ions in ruby possess two highly energetic levels. And people already were doing experiments on ruby, exciting ruby. And when you excite ruby, you dump atoms into an excited level, not at a concentration high enough to oscillate. Also, you don't have it in a resonator, which was not understood very well. And therefore, it would not have oscillated. But once you excite atoms in a ruby, some of them come down in a characteristic ruby color that's due to that emission. That was a natural candidate. People were desperate.
There was not much knowledge. There were a bunch of fanatic types who had been doing experiments of that sort, looking at energy levels in different crystals, measuring lifetimes, light emitted. But not with the idea of making a laser. We took that base of knowledge. And one of them was ruby. And there were even one or two people at Bell Labs. One of them is the co-inventor of the laser, won a Nobel Prize for it. But those people very quickly decided they had measured the lifetimes involved here, and the excitation rate that ruby is not a good material to make a laser. They gave up on ruby after a while.
ZIERLER: Did that group dissolve or go onto other things?
YARIV: What had happened, though, they were right. But they were thinking of making a continuous laser. And what Maiman eventually did is a pulsed laser. Which was easier to make and therefore the right way to go when you are trying to make the world's first laser. He got outside the box. We were trapped in the box. I was not part of that group. I would've been trapped as well if I were there. Because nobody thought of a pulsed experiment. But the first laser was done on a pulsed basis. The people at Bell Labs were the experts, and ruby (the mineral corundom which contains chromium ions as impurities which give it its color)) is a high-quality crystal, which also is necessary, otherwise the light would be scattered as it traverses the crystal. We understood the spectroscopy, and scientists at Bell measured the lifetime excitation rates etc., found out if the necessary inversion of population could not be achieved. And they were right: not on a continuous basis. Because what happens with ruby, you excite atoms to level two, which is good because you want N2 bigger than N1, but eventually they come down to the ground state. You have to make a laser during the time they remained in the upper level. Three groups following different directions, competitive, working hard, good people, all of them.
Bell Labs as Temple of Basic Science
ZIERLER: Tell me about the funding environment at Bell Labs. Was money no object? Could you ask for anything that you want and get it?
YARIV: Effectively, yes. I don't know if you knew the economic basis of AT&T.
ZIERLER: A monopoly.
YARIV: Monopoly. The way AT&T made money and disseminated some of it to its shareholders is to give the government a list of their expenses for a given year, and allowed, I think, an 8% profit. Allowed expenses were trucks, telephone poles, wires, offices, electricity, and research. Research was an allowed expense. No questions asked. The more research they made, the more money they made because they got eight percent of it.
ZIERLER: That's a great business model. [laugh]
YARIV: Under that business model, the transistor was invented, essentially the laser was invented, a lot of other important stuff.
ZIERLER: And of course, it's in the interest of the US government to fund all of this.
YARIV: Yes. But the amazing thing was, because AT&T was a commercial company eventually run by money people, when they invented the transistor, the most obvious application of it was to automate electronically telephone exchange. What they had were the mechanical clanking boxes with relays going on and off. And a building full of these boxes in every American town and city. And Bell Labs did not develop the transistor-based telephone exchange. And the reason was, they had a business making a profit. Why change? [laugh] It wound up being Texas Instruments, Hitachi, other companies.
ZIERLER: What did that translate to in terms of money being no object with the quality of the instruments?
YARIV: Well, you could buy the best. Whatever you wanted to.
ZIERLER: Did that mean that you didn't really need to build anymore because you could buy the best?
YARIV: You only needed to build things that you couldn't buy. Like building the upper enclosure that Gary Boyd did for illuminating the crystals was a unique, clever, but required machine shop at Bell Labs, which was a very good machine shop. Things we couldn't buy, which were a minority, we would make.
ZIERLER: What were some things you needed to make at Bell Labs?
YARIV: As I said, the enclosure resonator houses the crystals to excite them. We had to make that. But anything that could be bought was bought. My Berkeley experience was magnetic resonance. When I came to Bell Labs for the first year or so, I did magnetic resonance on crystals which were candidates. We had a whole bunch of crystal growers at Bell Labs. And they came to me with a whole bunch of crystals, and I had some idea what crystals were better than others. And they grew them at Bell Labs. And I tested them. I learned about the energy structure by doing conventional electron paramagnetic resonance. I contributed the spectroscopic, atomic aspect to our experiments. When I switched over, and dropped resonance, and concentrated on lasers and pure optics–after about a year at Bell Labs, I had six, seven people working for me, ranging in age.
One or two were crystal growers who worked with me growing crystals. We went through a whole bunch of them. There were a lot of Polish, in that part of New Jersey. And working as technicians at the labs. Very good experimentalists who worked with me. And I was taking mostly data. I designed and built a magnetic resonance system. This time, what took me two years in Berkeley took only a few months at Bell Labs. And I was doing magnetic resonance, mostly. And then, laser experiments. Crystals were grown, I tested them, Gary Boyd built the enclosure, then we did laser experiments, went through many crystals. Uranium turned out to be one of the more interesting ones. We made a uranium laser. And then, came the semiconductor laser. That was the early years at Bell Labs. A fresh PhD after a year, I have six people maybe working under me and a very efficient experimentation, although we've increased the growth to magnetic resonance to trying to make a laser in assembly line.
ZIERLER: Now, was there any pressure from management at Bell Labs or even AT&T to make this research applicable to the corporation?
YARIV: None whatsoever. Bell Labs had a development department, more applied. But we were on the research side. I was in the Electronic Research Department. There was a Solid-state Research Department, Theoretical Physics. There was no pressure whatsoever.
ZIERLER: Were you thinking along these terms yourself? Were you thinking about business, about patents, about applications?
YARIV: Too early. People mentioned all kinds of applications, but the only business you could think of possibly was to start a lab and get government money to do more research. The first time I started thinking that it might be a useful device was when the semiconductor laser appeared.
ZIERLER: Administratively, tell me where it fit overall within Bell. Who did Jim Gordon report to, for example? Would that have been the director of research? Or there was somebody in between?
YARIV: Probably somebody in between. Maybe one or two. Remember, there were maybe 5,000 people, of which 1,000 probably had PhDs at Bell Labs. It was a big operation.
ZIERLER: What about the research culture? Beyond your group, would you find yourself talking to other people in other groups because it was useful?
YARIV: Yes. Only when it was useful. But occasionally, yes. I also did other things. I made a microwave amplifier based on an Esaki diode. Anyhow, I made some microwave amplifiers, not based on laser action, and one year, it may have been 1962 or so, I was selected by Bell Labs to demonstrate my experiment in some National Science Foundation annual event. They took two or three developments they thought were important, and my experiment was one of them. Had nothing to do with lasers.
ZIERLER: Just out of your own interests.
YARIV: What I did in Berkeley is build microwave resonators and put crystals in them. A microwave resonator is a complicated animal. You shine radiation into it, it comes back. A simple microwave resonator can be a can, a microwave waveguide, a hole here. And microwave comes in and is reflected partially, depending on how big the hole. And inside, let's say you have something that can amplify the microwave. Under the right conditions, more light comes out than goes in an amplifier. That's an amplifier. And AT&T was in the business of amplifying signals to transfer information coast to coast or home to home. What I built at Bell Labs was a system like that, and the amplified element was a Schottky diode.
It was a p-n junction using semiconductors, which had some unique characteristics. Voltage, current, and here, the slope is negative. It's a negative differential resistance, the slope. And when this element was used inside here, you had a microwave amplifier. Negative resistance means when the voltage varies, the current flows in the opposite direction to a normal light that generates energy. I made an amplifier based on a Schottky diode, and Bell Labs chose that as one of two or three exhibits. I was kind of a microwave expert., meaning that very few people understood microwave at the labs. That was more a West Coast type of thing; Stanford, Varian, Caltech, Berkley…
ZIERLER: And you saw this as a side project of the laser work?
YARIV: A side project. I was never sure what it was I would do at Bell Labs. When I joined the laser work, I thought it was still a gamble. If it worked, would it be important? Would it last? It took my microwave background from Berkeley to realize that the differential negative resistance inside a box of high Q resonator would make an amplifier. And it did. That was also an experiment. But people realized I was playing around. [laugh] They read about the semiconductor laser demonstrated by GE and IBM. The transistor was invented at Bell Labs. There was a very strong semiconductor culture, and here was a semiconductor laser. Even before that, yes, I was asked if I would be interested in investigating and reporting on semiconductor lasers.
ZIERLER: So, this was in some ways even a hedge. If the laser research did not work out, this was something worth pursuing.
YARIV: Yes.
ZIERLER: What came of the microwave work?
YARIV: It was interesting, but it died off eventually. It wasn't an economic way of doing it. Another class of amplifiers appeared called parametric amplifiers. Which would relate to my later work, which was much simpler and better. So it died off.
ZIERLER: At Bell Labs, how connected were you to the academic world? Would you present papers at conferences, the same places that professors would? Or it was separate?
YARIV: Oh, yes. Gave a number of papers at conferences. Some at universities.
ZIERLER: What kinds of conferences were relevant for to present at? Optics?
YARIV: Optical Society of America. which got very interested but contributed very little. Because they did not understand the idea of optical modes. That's fair to say. That came from Gary Boyd, our group. And that was crucial. The International Laser Conference started as an annual event, which was the main event in the field.
ZIERLER: When did that get started? When you were at Bell Labs?
YARIV: Yes. As a matter of fact, the first International Laser Conference took place even before the laser was demonstrated. People were working on different places, and they got together. And it was done in a lodge in the Catskills. Shawanga Lodge. [laugh] There was a great deal of interest, and it was during those meetings that I met Roy Gould, who eventually was the reason I came to Caltech, so it was important.
ZIERLER: And where was Gould at that point?
YARIV: He was on sabbatical in Australia. But at Caltech.
ZIERLER: Did your wife like New Jersey?
YARIV: The topic didn't come up very often. She had family on the East Coast. And we had a baby. And she was a good wife. If I liked it, she liked it. [laugh] And I was working hard. I remember coming home for dinner, then going back to the labs, working until 11, 12 o'clock. Getting up at 7 or 8, working again.
ZIERLER: You mentioned the Optical Society. What about the IEEE? Was that important?
YARIV: They stepped in later on and started sponsoring a conference on lasers, more on the applied part of it. And I was involved in starting the more physics-oriented, quantum electronic conference, so there was a great deal of interest, not focused because nobody had a good idea what to do with the laser.
ZIERLER: And when you talk about these lasers, just in the pure science perspective, what are they good for in and of themselves? Not applications to other areas of research, but beyond just demonstrating that they work, what are the other goals of the group at Bell Labs? How do you know when you're on the right track?
YARIV: I think there was an idea that we took as axiomatic, that didn't need any proof, that if you could make an oscillator that emits coherent light at optical frequencies–we had oscillators at RF frequencies, at low frequencies. Your television set selects different channels by varying the frequency of a local "oscillator," we call that "tuning," so most sophisticated radio and communication applications all require oscillators. We already had coherent oscillators at radio frequencies, microwave frequencies, and they were the basis of major inventions and applications. And we all assumed that if you could repeat it at optical frequencies, it would be very important. And maybe that was the main driving force. People proposed applications all over the place. [laugh] Death rays, cutting plates of steel. Looking back now, it turns out that the study of the quantum properties of light, quantum optics, One of the most significant things we did and did not fully appreciate at the time – with Bill Louisell at the Labs and Tony Siegman at Stanford, we were the first to apply quantum mechanics to nonlinear optics, which actually started the field of quantum nonlinear optics.
The new theoretical aspects are very deep and very important. The basis of quantum computing, quantum communication to this day are based on understanding the quantum properties of light. And those people did not even ask those questions before lasers were around. The appearance of lasers provided the impetus for asking a new type of questions but also trying out experiments. The branches of optics, nonlinear optics or parametric optics, related to my experiment. [laugh]
ZIERLER: And these questions could not have been raised before this research.
YARIV: Could not. That's right.
ZIERLER: You mentioned as a graduate student, Bell Labs was the only game in town, essentially, in terms of doing this work. But then, later on, GE, RCA. When did it start to become a competition between industrial labs in laser research? Was that during your time at Bell Labs? Or that came later?
YARIV: It did not happen while I was at Bell Labs. Here's an example. The semiconductor laser did not come from Bell Labs. It came from GE and IBM. And that was obviously very, very important. So yes, to be fair, it started while I was at Bell Labs and continued later on. The industrial labs started concentrating on applications of lasers more than basic research with one exception, the Hughes Research Lab in Malibu here. They had some people who asked some very basic questions. And when I came to Caltech, I started consulting with them. And we can go into that in more detail.
ZIERLER: Of course. A technology question, was anybody using computers at Bell Labs? Fortran, Kaypro, the earliest iterations of computers when you were there?
YARIV: I was never a computer person. Not to this day. [laugh] And because of that, I always said when I needed computers, I was at Caltech and had graduate students who understood and used computers far better than I did, so they did it for me. So I can't answer questions about the levels of computers and computer research. I'm sure there was a computer department.
ZIERLER: But it wasn't important for you and your group.
YARIV: No. Even to this day, almost everything I've done, I had a basic mental model always based in fundamentals like Maxwell's Equations or the Schrödinger equation that did not required computers. These came later to work out the details. still claim that you cannot invent new concepts with computers. One day, I'll be proven wrong, probably.
ZIERLER: Maybe with quantum computers. We'll see.
YARIV: You have to think of the problem and realize there is a problem. I will probably be proven wrong. So far, I have not, in my experience. [laugh]
ZIERLER: Last question for today, an easier question. What was it like socially at Bell Labs? Would scientists get together socially? Would you hang out? Would you go on dinner dates with couples?
YARIV: Yes. Murray Hill is in Summit, New Jersey, a small town in Sopranos land. [laugh] Our social life was mostly with Bell Labs people, I was interested in music and ballet, so trips to New York City on weekends, trying to make it to the seashores [laugh] with other hundreds of thousands of cars coming south from New York City on the Garden State Parkway and the New Jersey Turnpike.
ZIERLER: It was good to be close to New York when you had the opportunity.
YARIV: Yes. I enjoyed it very much. But I also knew that I needed to get back to California at some point.
ZIERLER: And on that note, we'll pick up for next time. We'll stay on Bell Labs because there's more to discuss with the origins of semiconductor lasers and of course, the intellectual origins of quantum computing, quantum electronics. We'll pick up on that next time.
YARIV: OK.
[End of Recording]
ZIERLER: OK, this is David Zierler, Director of the Caltech Heritage Project. It's Wednesday, November 10, 2021. It's my great pleasure to be back with Professor Amnon Yariv. Amnon, good morning. As always, it's wonderful to be with you.
YARIV: Thank you. My pleasure, equally or more.
Partnership with Intel
ZIERLER: Today, we're going to take a pause in the narrative trajectory. We'll come back to Bell Labs in a little bit. But even today, you're active in so many fields. Tell me about your work with Intel.
YARIV: Oh, about ten years or so ago, with two ex-students in my group, Naresh Satyan and George Rakuljic, we founded a small Pasadena-based company named Telaris. I don't know what it means, I didn't choose the name. [laugh] My students did. Based on some earlier work at Caltech of electronic control of the frequency of semiconductor lasers, which are an electronic animal and can be therefore driven, we found a way of chirping them. Essentially, varying the frequency output of the laser, linearly, in our case, and with that, a basis for performing imaging. In conventional imaging, you bounce light, you measure the time, LIDAR-type imaging, radar. Ours was based on chirping the frequency. We can go through the details. That method is far superior, but more difficult.
You need to know how to control the laser. Today, it's a major race. Almost every major electronic and automotive company in the world has a substantial effort in autonomously driven vehicles or self-driven cars. And we entered the fray with that new approach. Intel Corporation bought us about three or four years ago. And my two students became employees of Intel, although all of us refused to leave Southern California. Intel–and this is not even known at Caltech–bought us and built us a lab smack in downtown Los Angeles, where we actually continue the development of this technology. In the meantime, Intel already had an effort based on the conventional approach followed by everybody else, and they've essentially dropped it and adopted ours. We have no doubt that the other effort followed by the rest of the world will fail for basic reasons.
ZIERLER: What are those reasons?
YARIV: The main one is the inability to discriminate between your own signal that you are measuring on the highway and that of other cars. To do it really well, the Mickey Mouse method–because the highways are full of hundreds of thousands of cars, and eventually all of them are blasting away with LIDARs. Self-driven or driver-assisted cars. And not to be able to discriminate your target from other objects is fatal. You probably know the Tesla accident, which was an inability to discriminate between the rear of a truck on the highway and the sky.
ZIERLER: Was this the one in Arizona?
YARIV: The one in Arizona. That's probably not something to go into. Ours is based on emitting a continuously chirped signal.
ZIERLER: What does that mean, chirped?
YARIV: It means a signal with varying frequency. Like a bird chirping. Or the way that a bat flies blindly at night.
ZIERLER: Echolocation.
YARIV: Echolocation. But what happens is, if you emit a chirped signal–let's plot frequency as a function of time. As time progresses, the frequency changes, and linearly in this case. That's the idea, though not necessary. That's the transmitted signal. And we keep repeating it. That's a light wave emitted from the car at varying frequency. And we are looking at the signal, which we return. The return signal is delayed, let's say, by some time, tau, which is proportional to the distance. It's the same signal, but delayed. They're transmitted, reflected, and that vertical distance–let's call the slope of this psi. F is psi times T. This is F = psi(T) minus Tau, delay between them. The delay is proportional to the distance. The vertical distance is psi times tau.
At a given time, back at the transmitter, if you can compare the frequency of the emitted wave, which you are emitting all the time, this repeats itself. The vertical difference between the received and the transmitted is proportional to the distance. And it's very easy to measure frequency differences between two optical waves. You're simply multiplying by each other, which is done in an ordinary detector. And the outward current will then oscillate at the different frequency. Let's redo it. What we're doing here is repeating exactly what happened during the World War II, which started with England's desperate to detect German bombers heading toward England. A few hundred bombers would come overnight. The English wouldn't know where they were aiming or going. And they would take their few hundred spitfires and hurricanes, fighter planes, and spread them all over England, but never knowing where they were, they could never concentrate them, so it was not a very effective defense. And the Germans really caused major destruction.
And at some point, the balance of the War was at stake because of that. Eventually, they invented radar. I use the word invention here, but a whole bunch of countries have claims for inventing it. But they have made a major development during the war and invented the magnetron and were able then to bounce powerful pulses of microwaves, radiate them toward France or the direction in which the German bombers would come. They were able to really determine the direction and make an educated guess of where the bombers were and were much better able to anticipate it. And the rate of losses of German bombers was such that eventually, the Germans gave up bombing them. It was a turning point. That and enigma. That was radar. '41, the US entered the War. And a bunch of great scientists who would later win Nobel Prizes, MIT, Harvard types, in a new lab called the Radiation Lab started an American radar effort. And they adopted the chirped approach.
ZIERLER: The Rad Lab at Berkeley?
YARIV: No in Boston, the Rad Lab. The key was the ability to chirp a laser. Our efforts at Caltech, which were not specifically on radar imaging, laid the basis for that. And Caltech patents later were sold to Intel. And we have developed essentially mathematically, intellectually, the equivalent of what happened in World War II. The rest of the world is still using the German radar, and Intel is developing ours.
ZIERLER: What's the timeline on this? How far back does this research effort go?
YARIV: We sold to Intel about three years ago, and the effort at Caltech was ongoing on a small flame for about seven, eight years before that. Two or three students.
ZIERLER: This broader story of Intel losing its footing a little bit in the race for more powerful chips, where does this research play into it?
YARIV: Intel needs a shot in the arm, they're losing their lunch to AMD and Nvidia. If you have access to money, buy their shares. [laugh] And Nvidia, much bigger than AMD, are developing the most advanced electronic chips today, which they develop mostly for the gaming industry. But they're now used by Livermore and Los Alamos to make their biggest computers, supercomputers, and by everybody else. And they signed an original agreement two or three days ago to work with Facebook, now called Meta. And they will exceed Intel and possibly Facebook at the rate they're going. Their technology is kind of the key for this new Meta universe, repeating propaganda. To get back, Intel acquired us, a very smart move.
ZIERLER: Did you need to be acquired to scale up this technology? Or you were fine not being bought?
YARIV: No, we couldn't have done it ourselves. Mobileye is an Israeli company which Intel acquired maybe ten years ago for $20 billion, and it's probably the leading company today in driver-assisted technology by far. Mobileye now is a fully owned division of Intel, and we are part of Mobileye and Intel. Between the two companies, there are probably a few hundred people at Intel working on it. Intellectually, there are two efforts here, the computer part, which is run by mostly Mobileye, the founder of which is Amnon Shashua, a professor at Hebrew University in Jerusalem, and the optic laser part of it is our contribution. And that's run out of Los Angeles in the lab and Santa Clara.
There's a video clip maybe two or three months old in which Amnon Shashua describes where Intel is going. He mentions our chirped laser solution obliquely. We couldn't talk about it until a few months ago. Back of an envelope calculation, there are roughly 100 million cars sold a year. If you assume that each one of those cars will have roughly $2,000 or $3,000 worth of electronics for autonomous driving–which is not a bad estimate because you're already probably spending $2,000 a year on the simple rear camera on your car. This will be far more complicated. You're talking about a market of $100 to $200 billion a year. That might become the major business for Intel.
ZIERLER: Does Moore's Law still apply today? Is that still achievable?
YARIV: It does not. AMD and TSMC essentially have eaten part of Intel's lunch, mostly by their ability to make smaller chips, smaller transistors. They're down now to five nanometers roughly. Future size on the new Amazon, which is made by TSMC, and based on AMD design. Apple is getting their design themselves. TSMC right now and Samsung, roughly 80%/20%, have the whole market. $50 billion of the new infrastructure that Biden just passed will probably go to Intel and a bunch of others, Texas Instruments, to try to catch up with TSMC. But the race there is to make smaller and smaller. To do that, you need the photolithography light sources of shorter and shorter wavelength. And they're down now to five nanometers, 50 angstroms. That's roughly the distance between ten atoms in silicon. They are talking about the next step of three angstroms. Roughly, the distance, I think, in silicon is four angstroms, so you're basically talking about atomic size. You will not be able to make a transistor from a single two or three atoms, so you will have to look for another solution. Therefore, they're running into a brick wall. And the race for the replacement is going to be the race of the century.
ZIERLER: You're saying Moore's Law no longer applies because you can't go smaller than an atom?
YARIV: It was based on a continuous reduction in future size. And the brick wall they're approaching now.
ZIERLER: We have to get smaller than quarks at some point to continue.
YARIV: Yes, you need a paradigm shift of how to switch.
ZIERLER: Is Moore's Law only about size though? Can there be a doubling of however we define progress that's not smaller and smaller?
YARIV: Moore's Law in practice amounted to reducing future size. Philosophically, you would not call it Moore's Law anymore. You may argue that we will continue to increase computing capacity of an iPhone as an example, but it will not be based on future reduction. It may be based on quantum computing, on new programs, new methodology of computing, using the third dimension where Intel is quite strong. The whole chip industry, which is the TSMC in Taiwan, will change.
ZIERLER: What about energy efficiency? Is that part of Moore's Law? I have the new M1 chip on this computer. My battery goes all day now.
YARIV: Yes, because computing is based on switching transistors and capacitors, and memory on, off, on, off. Every time you do that, you dump the charge. You charge capacitors one way or another, and then you dump the charge, throw it away. The charge is proportional to the capacitance times the voltage. The voltage is fixed. Roughly two volts. The capacitance is proportional to the area of the capacitor. As smaller capacitors, effect of two reduction in dimension is effect of four reduction in area, therefore reduction in capacitance. Effect of four reduction in energy loss per switching, and that has gone down with Moore's Law. They became more efficient. The increasing inability to make the future size smaller, that approach is to be gradually given up. Within less than ten years, I would say. There must be an ongoing search. The solution will probably come from a university, as they usually do. [laugh]
The Road to Autonomous Driving
ZIERLER: This research for Intel, when will we see it come to market, and what will the implications be?
YARIV: Self-driven cars. Automobiles. This might be Intel's savior, that little acquisition.
ZIERLER: Is this proprietary in the way that Waymo, Google partnered with Volvo? Is Intel thinking about exclusive rights for a particular car brand?
YARIV: We have, and by we, I mean Intel, the basic patents, but those will expire in probably five to six years. But there's something else going on here. Today, to make a commercial transmitter-receiver based on our technology will take something optimistically the size of a large suitcase, part of a car trunk. Independently of us, Professor John Bowers at UC Santa Barbara invented the technology of fabricating lasers on silicon. Intel has now probably the leading industrial capability of producing what's called silicon photonics, optics on silicon. And that will be crucial. The circuits, which will be the heart of this commercial product, will involve optics and lasers on silicon. And Intel is way ahead of everybody else. Companies like Volvo, GM, are probably not even aware of it. What will probably happen is that Intel will sell the modules, the heart, the swept lasers, the optics as units to everybody else. That's where the money will be. And Mobileye will probably be the vehicle for doing it. You can see it unfolding both scientifically and economically of a major new development.
ZIERLER: Are you now or will you get involved in some of the inevitable ethical or legal implications of self-driving cars when it makes those decisions? "Do I hit the grandmother or the mom with two small kids?" A self-driving car will need to make decisions just like a human driver. In the way that those decisions will have legal, moral or ethical implications for a person, how does it translate to a machine? Or does it not?
YARIV: I'm completely disconnected from those. There are probably batteries of lawyers and moralists consulting. We're solving how can an image, take a picture of what's going on on the highway a few hundred yards ahead of you. The imaging technology.
ZIERLER: But those discussions between the lawyers and the ethicists will result in policies that will need to be embedded in the programming, no?
YARIV: Possibly, yes.
ZIERLER: But that's too far afield, you're saying.
YARIV: It's ahead. We're losing how many people a year in the US to auto accidents? 30 a day? A big number. Probably, they'll decide what is acceptable, how many people can die a year. If it's a fraction of the number of people who die today, then it's a step forward. And I think that technology will actually lead to an improvement in that sense. But you will have to develop it farther. You need a higher degree of discrimination, ability to detect at longer distances. That decision would translate into qualification and specs in the technical realm.
ZIERLER: Will long-haul trucking be an early adopter of this, simply because 99% of the truck route is straightaway highway driving rather than the complexity of driving in a grid, loading, unloading? Would that middle point of the trucking route be an early adopter of this technology?
YARIV: I think so. It's self-driven cars, but more likely, it will be driver-assisting technology. Prevent the truck driver from falling asleep or waking him up when he falls asleep, preventing from changing lanes inadvertently. So yes, I think so.
ZIERLER: And we have technology today, Tesla has the auto assist. Is this different, or is this the beginning, and you're working on the next chapter?
YARIV: Well, this would be based on actually having a good picture of what's going on around you. What's more, having the information in an electronic form can be used immediately to navigate the car, stop, move it away, change lanes, do what's necessary. The fact that the information is obtained electronically is very, very important. You can pre-correlate it with data based on sufficient intelligence to make decisions. Is it a dog crossing the street, or is it an old lady with a cane? [laugh] That's what's going on with Intel. So yes, most lawyers running into a wall. [laugh]
ZIERLER: But for you, this is exciting. This is the future.
YARIV: It's very exciting. I like the competition. We beat Bell Labs and AT&T with the distributed feedback. And of course, with Intel now, we'll give rise to a major new technology.
ZIERLER: Have you ever talked to Gordon Moore himself about these things?
YARIV: No. Is he still around?
ZIERLER: He's alive and well in Hawaii. [Ed. note: Moore passed away in March 2023.]
YARIV: No, he probably doesn't know about it. [laugh]
ZIERLER: He's disconnected from these things.
YARIV: Well, he knows about Carver Mead. I doubt that he is involved in optics.
ZIERLER: Well, let's return back to Bell Labs and start with the semiconductor laser. At a very basic foundational level, what is a semiconductor laser?
YARIV: A semiconductor laser is a laser, the dimensions of which are measured in microns. Think of it as an oblong object, the transverse dimensions of which are roughly on the order of one micron. The length is maybe 100 microns. Remember, 1,000 microns is a millimeter. And then, it's multilayered, and the layered dimensions are fractions of a micron, a tenth, let's say. It's an electronic device made of multilayered semiconductors, mostly III-V type semiconductors, gallium arsenide, indium phosphide mixture. If you choose the right layers and order them properly, choose the right dimensions, when you pass current through them, the device emits light. The light's photon energy, H bar omega, is more or less equal to the energy gap of the active layer of that sandwich. And the conversion is very efficient energetically. It can approach 90% or higher.
The first lasers were the size of this table. The shrunken size are still big and bulky. And here, you have a laser that you need a magnifying glass to see, which directly can be run, excited, fed by electronic, conventional, transistor-based circuitry. And even the voltage and current requirements are compatible, and it converts with near perfect efficiency. It's everything we've dreamt about. And it has the potential of a new generation of technology, in which the electronic part, and the transistor, and the optical part, and other optical components are fabricated using technology, foundries, altogether rather than being connected to each other. And the guiding of the light within that circuit is done by optical waveguides. The basis for my decision when I left Bell Labs to come here to make that a central part of my research was a realization that it was a no-brainer. If you have a laser that can team up with transistors, then you have a winner.
ZIERLER: But you thought of the idea of a semiconductor laser at Bell Labs?
YARIV: Not the semiconductor laser. I did not invent it. I really had no part in the invention of the semiconductor laser.
ZIERLER: Who did invent it?
YARIV: The publication was simultaneous of a paper from the GE Research Lab, and the lead author was Robert Hall, a Caltech PhD. And in parallel, a paper from IBM Research Lab. A bunch of good people there. My contribution to the laser itself was years later, the distributed feedback laser. The laser emits a pure single frequency, which was a prerequisite for optical communication for the fiber technology. Also, the proposal and demonstration of the integration of optics and electronics, which might be the key technology for communication for the 21st century.
ZIERLER: Let's go back to GE Research and Robert Hall. What were the advances, both theoretical and the advances in instrumentation, technology that allowed for the semiconductor laser to be conceived?
YARIV: The early days of the laser, the race was to make lasers, show that lasers were possible in different media. I was involved in the third laser in the world based on uranium doping calcium fluoride. Then, lasers were made in liquids, in gases. "Look, ma, I made a laser." Semiconductors, which were the mother material of electronics, were a natural candidate. There were many papers about the feasibility of making lasers in semiconductors. There were theoretical demonstrations. It turns out, in retrospect, they were barking up the wrong tree. Their approaches were not even scientifically sound. And then, out of the clear blue sky, out of two labs, IBM Lab and GE Lab were working secretly on lasers in gallium arsenide in p-n junction. It was already known before that if you pass current through a p-n junction in gallium arsenide, not silicon–you cannot make p-n diodes in silicon, too–light was emitted and fairly efficiently.
Although the initial generation of scientists probably wasn't aware of how potentially efficient it was. People who were already working with p-n junctions converting current to light said, "Well, let's try our gizmo." They made those p-n junctions, gallium arsenide, single-layer, not multilayer, but the p-n junction was there. Cooled it to liquid nitrogen and ran current through it, and lo and behold, light came out. And they could establish if the light was semi-coherent, and it was a laser, at the threshold which is the signature of a laser.
ZIERLER: And no one was thinking applications at this point, the achievement was just making the laser.
YARIV: Absolutely. I suppose if they were smart, they realized you can make a laser with a semiconductor. That's probably important. But nobody dreamt it. My contribution was, I had already made a laser at Bell Labs, and then when I came to Caltech, we immediately within a few months had simple lasers going, gallium arsenide. And then, through association probably with Carver Mead–Carver Mead and I even wrote a paper together, one paper on a laser-type topic. Carver was working possibly III-V transistors or something. Not even knowing how a transistor worked probably, but the mere fact that one can make transistors in gallium arsenide, and I was already making lasers… "Why not make them together and have the current of the transistor, which I can turn on and off run the laser? And the laser will emit pulses, communication." A mother technology. I call it integrated optoelectronic circuit. IEOC.
ZIERLER: Let's go back. When these papers come out from Robert Hall from GE, you're at Bell Labs. Just to situate us intellectually, what were you working on when these papers came out?
YARIV: Looking for laser action in other materials, mostly solid crystalline, not semiconductors. I didn't know what the semiconductor was.
ZIERLER: You never heard of a semiconductor?
YARIV: I'd heard the name.
ZIERLER: Was there semiconductor research happening at Bell at that time?
YARIV: Oh, major, major. The world's leading center for semiconductor research. The transistor was invented there. But none on light-emitting semiconductors.
ZIERLER: Was Bell aware of the research at IBM and GE?
YARIV: No, not until publication.
ZIERLER: There was no race between Bell and GE and IBM?
YARIV: No. I was called one day by my superior at Bell Labs, shown the article either from GE or IBM, and they also knew that I was kind of at the end of what I was doing, and I was asked politely, "Would you like to explore this? We'd like to start an effort at Bell Labs. Would you like to head it?" And I agreed. I think probably willingly. I knew very little about the semiconductor. But a month later, I gave a talk at the semiconductor physics group at Bell Labs, which was probably the best in the world, on how a laser would work in a semiconductor. I did the theory for gain and lasing in semiconductors. In the meantime, very quickly assembled a team of people who knew how to grow crystals, and we essentially repeated the IBM setup within a month or a little longer and had a laser going. And in describing the laser, it had to be cooled, as I mentioned earlier, liquid nitrogen. That reduces the threshold and therefore, the current required to obtain lasing. Without it, it won't work. In making the laser, trying to understand why it worked, I developed a theory, which I gave in the lion's den at Bell Labs. [laugh] I remember a reaction later, "How would someone at the electronic research lab"–I offered to give a paper, they accepted, and it turned out that although I didn't quite know much about semiconductors, they didn't know anything about lasers so we both came out ahead.
ZIERLER: What is this lion's den?
YARIV: The best people in the world on semiconductor material and theory. And my theory involved the band gap, inversion of population. I learned all of those things very fast. The only thing which really gave me the courage to do it was knowing that they knew much more than I did about semiconductors, but I knew more than they did about laser theory.
ZIERLER: Was there a particular person that you relied on to get you smart on semiconductors at Bell?
YARIV: All I needed was basic textbook knowledge, which wasn't true for them because what they needed, a laser text, was not available. There was only one paper that I'd written with my boss at Bell Labs, Jim Gordon, called The Laser. It's like a small textbook giving laser theory, combining it with optical resonators so that you have to bring in the optical losses, and deriving the threshold condition, and then talking about the communication aspects of it, too. How good was the laser as an amplifier from the point of view of noise? Within a month or so, we had a laser, but then–a semiconductor laser, we made–the material is gallium arsenide. And this is pre-doped, and this is doped. And current, let's say, from a battery.
ZIERLER: And doped means what here?
YARIV: Oh, when you've got a semiconductor like silicon, you make it as pure as possible, the substrate. Doping means that you diffuse into, when you grow the crystal, the silicon an impurity, a foreign atom like calcium, magnesium, arsenic, and that changes–the ideal semiconductor is an insulator at a low temperature. Fairly good insulator. If you dope it, it'll conduct, depending how heavily, and that has to do with the band structure. But that's how you can control the conductivity. You can dope it either with silicon–silicon atoms have four electrons in the outer valance band. If you dope it with arsenic, arsenic has five electrons in the outer orbit. For the arsenic to pretend to be a silicon atom, it has to get rid of this excess electron. That electron conducts. The more arsenic you include–and highly doped will have as much as maybe a few percent of arsenic–the more conducting it is. It'll never reach conductivity of a good metal.
If you do the opposite, if you for instance diffuse indium into silicon–the first type of doping is called n+ doping. n- because you generate free electrons, which are free to move through the crystal. If you dope it with atoms that have less than four electrons, then it's the opposite. They take electrons from the silicon to complete the valance and leave behind holes. And they can conduct electricity as well. A p-n junction is exactly what you have here. You grow layers. One side is diffused with, let's say, indium, which would remove one electron away from the neighboring silicon. Here, the conduction is done by holes. Here, you diffuse arsenic, which has five electrons. I can give you a better diagram in the future. That's a p-n junction. Every p-n junction is doped p type on one side, n type on the other. You connect the voltage to it and look at the current, you got something like that. That's forward bias, that's negative [bias]. If you polish the surfaces, mirror polish them, and you pass current, i, through a power supply, a battery, light would come out with great efficiency.
That was the original IBM/GE lasers, gallium arsenide. A p-n junction, gallium arsenide, polished. We had them going within a few months at Bell Labs. Part of my team had people who actually helped Shockley 15 years earlier to make the first transistor. Probably had one of the best technicians I've ever seen. I gave a talk in the Physics Department at Bell Labs how it worked, and to explain how it works, you have to use semiconductor theory. Not very Pound-stabilized Pound-stabilized deeply, but basic. I taught myself. In trying to understand how it worked, I reached the conclusion that–all lasers worked at that time by light bouncing back and forth and being amplified in transit between mirrors. As it travels back and forth, it gets amplified, bigger, stronger. When it hits the mirrors, some of it gets lost.
Some is getting out, some is lost in transit. If the gain is bigger than the total losses, then in transit, it will increase as a function of time, and that's the laser action. That threshold. When the gain is equal to the loss. In figuring out why it's lasing, I used physics to calculate the gain per one pass. But by photographing when it lased by photographing the output, exit, face of the crystal, we established that light was coming from a narrow region near the center. That was difficult to understand because if light starts traveling from this narrow region, it diffracts, spreads, and yet we saw a narrow stripe so the light did not spread as it bounced between the front and back surfaces of the crystal. By the time it gets to this region, it'll spread, and it'll lose gain because gain is only available here. The light had to be confined to the central region for the laser to work. I reached that conclusion by doing my numbers.
I showed it to friends of mine there at Bell Labs. I said, "Something is keeping the light near the center." And one said, "Have you heard of dielectric waveguide?" Never did before. The whole new electronics and optics, integrated, is guiding. Dielectric waveguide. Index of refraction in a medium, in a material, is the ratio of the velocity of light, c, in vacuum to the velocity of light in material, n. n of glass is roughly 1.5. That means that light propagating in pure, ideal glass is traveling 1.5 slower than it would. Any transparent material, optical material that you would consider is characterized, among other things, with its index of refraction. That number depends also on the wavelength of the light. If you make a sandwich of n1, n2, n2, n1, of two optical materials, and n2 is bigger than n1, meaning if the bread of the sandwich has an index bigger than n1, it means that light propagates more slowly here than here, then light can be trapped.
It essentially does it by zigzagging and total internal reflection. Have you seen light in a swimming pool bouncing off? In a swimming pool, if you have a lightbulb here, and you're out here, light comes like that and doesn't come out, but bounces. It's the same. When you go from a material of a large index, water, to air, which has an index of approximately 1, when light goes from a high index to a low index, it can be totally internally reflected. And that means 100% reflection. It's not silver, that's just an interface. In my first paper, I made a claim that there has to be a dielectric waveguide effect here, meaning that the index of refraction of this layer was bigger–n2 bigger than n1. When that is true, then light can be confined, and that would enable the semiconductor laser to work.
The people who made it at first had no idea about it. They probably had never heard, same as me, of dielectric waveguiding. But being at Bell Labs with so many smart people, when I said, "What makes the light stay in a narrow strip?" they said, "Maybe that is a waveguide." I got a book, an author named Collins, with a chapter on dielectric waveguiding. Very simple mathematics.
ZIERLER: When you agreed to head up this group at Bell Labs, that must've meant you assumed you'd be staying at Bell Labs for a while.
YARIV: Well, that was within a year after coming to Bell Labs. My idea that I would leave was not under consideration by me or anybody else. Most of my colleagues stayed there. My decision to leave was taken maybe three years later and was sudden, not very logical.
ZIERLER: How much of the semiconductor laser research did you do at Bell Labs?
YARIV: After that, most of my work was semiconductor lasers. Most, not all.
ZIERLER: Let's get to that decision point. When do you start thinking about leaving Bell Labs? What year, roughly?
YARIV: I was in Bell Labs from '59 until '63, so roughly early 1963.
ZIERLER: And what were the considerations? The ocean, for one, you wanted to get closer to the ocean.
YARIV: Wanting to get back to California, partially. Another one, wanting to try industry. I went directly from university to a university-like setting.
ZIERLER: Right. Because Bell Labs is academic.
YARIV: Especially the research lab. More academic than a university. We didn't have to worry about money. And I thought, "I'd like to try the real world." Economics was not a consideration to the same degree that choosing to work at Bell Labs rather than other industries where I could've earned twice as much was not a consideration. In retrospect, it was a smart decision. It forced me into learning new things, which was very helpful in coming to Caltech.
ZIERLER: If your interest was in industry, did you think at all about Northern California, HP, the early, early days of Silicon Valley?
YARIV: I interviewed at two or three companies, and the best offer was from a company in Northern California. They also wanted to start a new laser department and gave me a good sales talk. And indeed, I went out and got them a bunch of contracts. But then, they told me to go and get more contracts instead of working.
ZIERLER: You realized you'd be chasing the contracts and not doing the science.
YARIV: Yes. It was obvious, and I didn't like it.
ZIERLER: What's the connecting point to Caltech? Did you know anyone here?
YARIV: Yes. Roy Gould. Roy Gould was very active in the area of traveling-wave tubes and plasma beam interactions in a research program at Caltech. I read his article, and I thought he was one of the best people in the field. I ran into Roy Gould in a number of meetings and introduced myself. I told him I had read one very famous paper of his, so we knew each other. He heard me give talks at those international meetings of the American Physical Society, the annual meetings in Washington DC. On one of those occasions, Roy told me, "If you ever consider an academic career, I'll invite you to come to Caltech." And I remembered it. And our contact continued on the same basis, and then I accepted the job with this company, Watkins-Johnson, in Northern California.
A daughter of mine was born there. And then, I decided that industry wasn't for me. I could have gone back to Bell Labs easily. But one, I really preferred California, and secondly, pride. [laugh] Coming back with my tail between my hind legs. "You were right when you advised me not to go." And I didn't like New Jersey. New Jersey's elegant, the part around Bell Labs was very elegant. But it was difficult to do things, to see theater, go out to the ocean. I was born in the desert. So I didn't go to Bell Labs. I remembered Roy Gould, tried to call him, and was told by his secretary that he was spending a sabbatical in Australia. She gave me his phone number. I reached him, and he said, "Stay where you are. I'm calling a colleague at Caltech, and he will take over." Within a few days, I had an invitation to come and give a talk.
ZIERLER: Who was the colleague?
YARIV: A fellow named Irving Langmuir in the EE department. He died a few years back. Roy Gould was in E-Light. They invited me, I gave a talk, and they made me an offer, which was not the best offer. [laugh] And I accepted it.
ZIERLER: Did you apply to other schools?
YARIV: Yes.
ZIERLER: Where else?
YARIV: I applied to Cornell, UC Santa Barbara, UC San Diego, Stanford. I did not apply to Berkeley, but when Berkeley found out that I was looking, they made me an offer. Because physics professor at Berkeley knew my work, so they didn't need to interview me. San Diego was out because of surfing. I knew I missed surfing. Stanford wanted me to shift directions. They said there were already two laser scientists, and that would be enough. They now have probably 20, 30. [laugh] And I didn't want to do that. I probably would've stayed if they would've allowed me to continue working.
ZIERLER: Were you aware that SLAC was being built up at Stanford? Would that have been interesting for you?
YARIV: I don't think SLAC would've made any difference. SLAC is nuclear physics. Although, the original free electron laser was probably used, but that was before that. The inventor of the free electron laser was a student in my class, and he came to me with the idea and said, "You think it'll work?" [laugh]
ZIERLER: Who was that?
YARIV: John Madey. He's the inventor. He didn't get the credit he deserved because he was not a good politician. That's a side story. He was in my class, a brilliant fellow, but a bit disconnected from reality, which was the source of his problems. He ran into a patent battle with Stanford, which was not a clever thing to do.
ZIERLER: Caltech's offer was not the best.
YARIV: No. Not economically. They offered me associate professor. Cornell and I think San Diego offered me a full professorship. And I was only four or five years out of college. But there's something about Caltech. Roy, the size of the school and the concentration of industry around here, aerospace industry, was attractive. I took the offer. Although, within the first year or so, mostly because of the smog, I came close to regretting it and quitting. It was horrible.
ZIERLER: Now, was what Roy was doing relevant to what you wanted to do? Or you just limited the fact that he was around?
YARIV: The latter. Roy was really brilliant, and a nice fellow to boot. And I liked the atmosphere here. I sensed immediately the freedom of students to move from department to department with minimal red tape. The small size of the school. I liked Los Angeles. The ocean, it's warmer than San Francisco, the mountains are very, very close. In San Francisco, you have to drive 16, 17 miles to get to the mountains.
ZIERLER: How long did it take before you met Carver Mead and saw that you could collaborate?
YARIV: Almost immediately. We were in the same department. He heard me give a talk. We clicked.
ZIERLER: Do you remember what he was working on when you first connected?
YARIV: Things to do with transistors. Not yet on his VLSI. Big, big thing. More tunneling and p-n junctions. Making transistors. Metal semiconductor junctions. You can make a p-n junction, and you can make a metal semiconductor junction as well, and that has applications. Things which I did not understand very well. They're all related to transistors.
ZIERLER: You said that most but not all of your research in semiconductor lasers was at Bell. That begs the question, of course, when you came here to Caltech, what left was there to do in semiconductor lasers? What was the new research for you here?
YARIV: Make semiconductor lasers with different materials. Our first lasers that we made at Caltech, instead of emitting light at the near infrared, as we do today, 1.5 microns, they emitted deep into infrared, indium arsenide and materials of that sort. Did what we could. The field was so young that almost anything you did was publishable. Useful? Question mark. Optical communication was not on the horizon yet.
ZIERLER: Coming from Bell, where money was no object, what was it like setting up a lab here?
YARIV: Well, I got some starting money, which enabled me to buy some basic equipment. And maybe within a year, I made some contacts with the Air Force research establishment, so I got some modest contracts that enabled me to hire two or three graduate students.
ZIERLER: What was the Air Force's interest in your research?
YARIV: The Air Force was interested in laser research, period. They didn't know what it was good for, but everybody was hooked. [laugh]
ZIERLER: For weapons, possibly?
YARIV: The Air Force has research laboratories. The Navy has one. Weapons research is done in other laboratories. Those do more or less basic research. Typically, not the same high quality as top universities, but they have a research lab that keeps tabs on what's going on in the world. Roy Gould was supported here by the Navy, most of his research, and Roy very generously offered to split one of his contracts with me and got me together with a scientist at the Office at Naval Research who agreed to give us the money.
ZIERLER: On the personal front, did you go back to Israel? Did you have family that you would see at least once a year?
YARIV: Yes, more or less, once a year. Went back to Israel with my family, which consisted at that point of a wife and two children, daughters. We went every year.
ZIERLER: Did your kids pick up Hebrew? Did you speak to them in Hebrew?
YARIV: No. I was working hard.
ZIERLER: Your wife was American, so you communicated in English, of course.
YARIV: Right, the children only heard us speak English. It may be true that it's almost impossible for the children to pick it up if only one parent speaks a language. They have to hear it more naturally. Also, I was working for the first few years, starting a new research lab, worrying about my tenure. It was not automatic.
ZIERLER: You were associate, though? Or was it assistant with a rapid review?
YARIV: I was hired with tenure–
ZIERLER: You must've been if you were offered full professor elsewhere.
YARIV: –and told that I probably would be promoted to full professorship in two years. But I taught two courses, which were new in the world. There were no textbooks on lasers and laser physics. I started teaching those, write the books, consulted for an outside university because I needed some extra money, so I was working late every night, which ate into my time I had to play with my children. I made up for it on the weekend.
ZIERLER: Where was your first house?
YARIV: We rented a house in Altadena, not far from the mountains.
ZIERLER: Near Carver?
YARIV: No, I know exactly where he lived. On the border of Sierra Madre, on a street that starts in Pasadena, runs north-south. When it hits the mountain, it becomes a canyon, a very beautiful area. Linus Pauling and a bunch of professors lived there as well. But very prone to floods and fires. I lived maybe two or three miles west of him, roughly the same latitude, at the foothill of the mountains.
ZIERLER: You must've loved being by the mountains.
YARIV: Even now, I love it. But it's a 15- or 20-minute drive from here. I found myself on average making maybe two trips a day between Caltech and home, and therefore, spending over an hour or more. How much of my life would be taken up driving back and forth? When we looked for a home a year or so later–well, we bought a home in Altadena, too. I take it back. It was a little further down, but a normal home this time.
Staying Connected to Israel
ZIERLER: And you said you still had family in Israel, where were they?
YARIV: Most of them in Tel Aviv.
ZIERLER: Parents, siblings?
YARIV: Parents, yes. Stayed with my parents on my trips. Very nice.
ZIERLER: What was the '67 War like for you? When did you first get concerned that there was going to be a war?
YARIV: It was a shock. It came suddenly. I remember I went to the Israeli consulate, informed them I would like to go back, and they said, "We may not need you."
ZIERLER: Were you still in the reserves? How does that work?
YARIV: I was in the reserves until I was 50 years old. And we are talking about when I was 34, 35, 36. That meant that every time I went to Israel, before I could leave Israel, I had to first get a letter from the Army saying that I was not violating anything by leaving. In addition, I had to have a medical checkup, verify I was still in fighting condition. Every time before coming back, I went to the major recruiting center of the Israeli Army near Tel Aviv and stood in line in my underwear only, waiting for the medical exam, with kids who were roughly half my age. [laugh] They asked, "What are you doing here?"
ZIERLER: When the Six-Day War broke out, you went to the consulate and said, "I want to go home."
YARIV: Yes.
ZIERLER: How did your wife feel about that?
YARIV: Oh, she was OK. They said, "We probably won't need you."
ZIERLER: They knew it was going to be so fast. You must've been nervous.
YARIV: Very, very nervous. The next war, '73, Yom Kippur, I was in Russia. I remember that day because I had gone to the synagogue, which is not easy, it's a story by itself. I had to find out where the synagogue was in the morning. In the afternoon, we went to a Russian space exhibit, where they were showing Sputnik and the rest of the paraphernalia. My Russian escort who stayed with me the whole time, a Russian scientist, said, "There's a war between Israel and Egypt." I said, "Is Israel winning, as usual?" He said, "I think they're in some trouble." I got nervous. Two or three days later, we were in Kiev, and I got a chance to call my wife. And she got on the phone, and I remember saying to her, "I may be disconnected at any moment. People are probably listening to our conversation. Tell me how the War is going with Egypt." And she said, "Not too well. They're making gains." I became very nervous. I got to her again a day or two later, and she said, "Things are better."
ZIERLER: And you were in synagogue because it was Yom Kippur, obviously.
YARIV: Yes.
ZIERLER: What unit would you have reported to?
YARIV: I was in artillery. But I think before I left the Army the last few months, I was transferred at my request to the Air Force because the Air Force had a possibility of going to the United States and getting a degree in aeronautical engineering. You had to take an exam. That appealed to me. I was not yet sold on lasers or optics. I took the exam, and I didn't pass. They said, "You can go and study airplane maintenance, be an aircraft mechanic, but not an aeronautical engineer."
ZIERLER: Why? What did they find?
YARIV: It was a difficult exam. It involved mathematics, but also psychiatric, and I didn't do well.
ZIERLER: Maybe they realized you were a crazy professor. [laugh]
YARIV: I had no chance to talk, I just got the results and the decision. Thank God. [laugh]
ZIERLER: You don't have to share any secrets that we still don't know about, but did you know about Dimona?
YARIV: No, I don't think so.
ZIERLER: That was all secret, beyond you? You had no idea?
YARIV: People did not write about it. And later, people referred to Dimona by a name. Maybe the Fertilizer Factory, or some name, which was a euphemism, not a true thing.
ZIERLER: Did you hear about Golda Meir and the Shimshon threat?
YARIV: What about it?
ZIERLER: That when Nixon and Kissinger were deciding about whether or not they were going to airlift the tanks and weapons, Golda Meir intimated that if they didn't support Israel while the Egyptians and Syrians were moving in, that she might resort to the Shimshon factor, meaning nuclear weapons. I was curious if you ever heard of that.
YARIV: No. There was a unit in the Israeli Army called the Forces of Samson. They were basically a Jeep commando unit.
ZIERLER: To think of the person who shook the walls down–you use nuclear weapons, there are no winners. Everybody loses. That's Shimshon. It's interesting.
YARIV: Referring to the bomb?
ZIERLER: Nuclear, yeah.
YARIV: Did they have it already?
ZIERLER: Israel has never publicly confirmed nor denied.
YARIV: When I was at Berkeley, there was a graduate student, a group of two or three very smart Israeli kids roughly my age came to study nuclear engineering, and they all went back to the army. They were there for a year or two.
ZIERLER: And then, the last topic I want to cover in today's discussion, the one thing to pick up on from last time, we started talking about the early, early origins of quantum computing at Bell Labs. Quantum science, quantum light. What were you doing in that realm at all at Bell?
YARIV: When I was at Bell, there was no quantum computing.
ZIERLER: Quantum optics, quantum light? Was anyone working on this?
YARIV: Well, light is a quantum object. I may have been the first one. [laugh] Did I mention the quantum theory of nonlinear optics?
ZIERLER: A little bit, but that's what we should go more into.
YARIV: I went up, spending part of my time coming up with a model about light through the quantum object. And the best work on the quantum nature of light wasn't done at Bell Labs. Professor Glauber at Harvard got the Nobel Prize for that. My contribution, which turned out to be important, was to look at nonlinear optics. Nonlinear optics is the area of optics and laser physics where you shine light, as an example, green light, into a crystal, and out comes infrared light or red light. Well, light can change color. We can amplify one light beam with another light beam. It involves a change of energy. Most of linear optics is where if you shine two light beams into a material, say, crystal, they ignore each other. Each one behaves as if it's all by itself. Nonlinear optics, one beam can affect the other. It can exchange information. The field of nonlinear optics deals with that.
And there are two or three Nobel Prizes, including Nico Bloembergen of Harvard. It turns out that the most beautiful aspects of nonlinear optics are the quantum aspects, and that can only be treated by quantizing. And I did it with a colleague who wrote the first paper on it. And among things, in the process, made a prediction of a new physical phenomenon called spontaneous parametric fluorescence, that if you have a crystal–special crystals, not every crystal. Had to do with the symmetry of the crystal. Crystals are all described by the symmetry properties. Crystals are self-repeating, and there's a symmetry, like a salt crystal, it has a four-four symmetry. That means if you are inside the crystal, and you turn around by 90 degrees, and you close your eyes and open them, you will not be aware that you actually turned around because you will see the same thing. It's self-repeating.
There are something like 20 categories of symmetry, which crystallographers defined, and only some of them have the properties which make possible nonlinear optical interaction. You shine light, fairly intense, let's say, blue. That's the frequency. Out of the crystal, this could be blue, and this could be red. I'll call it omega 2. And this could be omega 1. And it could be maybe green. And omega 3, the frequency of the incoming beam, is equal to omega 1, and that's omega 2. Conservation of energy. Well, it turns out that the quantum picture, which was not known–we predicted it–is what happens here. This photon is annihilated and essentially absorbed by atoms, and instantaneously, two new photons are emitted, and the energy is conserved. That's why this relationship. There is an umbilical connection. Those two photons are entangled. If this is a beam with a phase, then those two have phases which are related to that phase.
What we have developed is a quantum theory, meaning you need to quantize the electromagnetic field. That's a formalism, which maintains–that's the most basic way of looking at light. Classical light cannot describe–and we predicted a new phenomenon called spontaneous parametric fluorescence, the spontaneous emitting of photons when you shine high-frequency light at the crystal. What we did actually try was to quantize the optical parametric amplifiers. The optical parametric amplifier, again, a crystal, and you shine an intense laser beam, let's say, blue, into the crystal. And you shine a weak, let's say, red, beam, and what happens is that this weak red beam, energy is transferred, if you orient the crystal in the right direction–there are chapters on that in my book–and the weak beam is amplified and emerges more intense than it came in. And that's the optical parametric amplifier. Microwave parametric amplifiers already existed before that. The field of nonlinear optics is undoubtedly the most interesting and exciting part of optics.
ZIERLER: And you started this at Bell Labs?
YARIV: Yes. Big thing. There's a story. I went to a meeting, the first laser meeting in the world, held in Shawanga Lodge in the Catskills.
ZIERLER: How many people roughly were there?
YARIV: Maybe 150. A good electrical engineer, Heffner, gave a talk on the noise analysis of the microwave parametric amplifier. People had already derived the theoretical limit of the noise of a laser amplifier. Every good amplifier today, among the specs is the noise figure. Essentially, a measure of how much noise will be added to an amplified signal. And you want it to be as low as possible. You don't want to degrade the signal-to-noise ratio. It limits information rate. You want little noise, and people understood the noise limitation of the laser. A professor from Stanford was present at the meeting at Shawanga, gave a talk on a microwave–the microwave parametric amplifiers have a noise figure even better than a quantum limit of the laser amplifier.
The limit is a quantum limit consistent with the uncertainty principle. And of course, that surprised people. But he was a good man. I knew he didn't know quantum mechanics, so I raised my hand and just said, "Is it possible that this result is because the laser result, the limit derived, was based on quantum mechanics and he used a classical analysis?" And then, I went to Bell Labs and tried to solve the problem. And I had to teach myself quantum optics. Even the language is exotic annihilation, creator operators. The treatment is very elegant and the concepts even more so. Very abstract, but at some point very straightforward once you learned and internalized it. So I applied it, came up immediately showing that the two were equal in the limit. They are both quantum limited devices. But in writing the paper, which was published in the Bell System Technical Journal, I needed mathematical help and collaborated with a colleague, Bill Louisell.
We wrote the first paper, which was the basis of a whole bunch of things. It, among other things, predicted the physical phenomenon, which today is used for quantum communication. Coming to Caltech, at Bell Labs, I wrote a paper, which was a path opener, started a new direction. But other, better mathematicians at Bell Labs took over. I had other things to do, so beyond that original paper with Bill Louisell, I went back to semiconductor lasers. But coming to Caltech, I now had very good students, who were very good mathematicians. We got involved again.
ZIERLER: Amnon, I think that's a great place to pick up for next time, when you start getting involved with your students here. Perfect.
[End of Recording]
ZIERLER: OK, this is David Zierler, Director of the Caltech Heritage Project. It's Friday, November 12, 2021. Once again, it's my great pleasure to be back with Professor Amnon Yariv. Amnon, as always, a pleasure.
YARIV: My pleasure as well.
ZIERLER: Today, we're going to pick up on the transition from Bell to Caltech, and I want to develop further this story about at Bell, there were mathematicians at work who were more skilled than you, and you left the project sort of on the side. But when you got to Caltech, you connected with graduate students who were pretty advanced in mathematics, and you picked the story back up. Tell me about that transition. Who were these mathematical graduate students that allowed for you to pick this research back up?
YARIV: Actually, not quite. Maybe I can modify it a little bit. We're now talking about an aspect of my work, which is quantum optics, which is different from the semiconductor laser or from phase conjugate optics.
ZIERLER: Let's start there, with phase conjugate optics.
YARIV: Phase conjugate optics is a phase of my research here and probably a field of which I'm considered a cofounder. It'll take us probably a meeting or half a meeting to talk about it, so it'll be a major diversion from the direction you were heading a few minutes ago.
The Origins of Quantum Electronics
ZIERLER: Chronologically, does this come after? Because I want to start chronologically right when you got to Caltech and connected with these graduate students.
YARIV: That started maybe ten years or so after I came to Caltech.
ZIERLER: So we'll come back to that.
YARIV: But it's important and very elegant. Involves Feynman even a little bit.
ZIERLER: Wonderful. I never tire of Feynman stories, so we'll get there. Who were these graduate students?
YARIV: Well, the correction was, my involvement with quantum optics was in my first year at Bell Labs, so the year would be maybe 1960, give or take. Right now, any time I give you a date, it's plus or minus a year. Events, though, will be in the correct sequence. I went to the first international conference on lasers, or quantum electronics, as the field was known at the beginning.
ZIERLER: This was in the Catskills?
YARIV: In the Shawanga Lodge in the Catskills. I heard a talk given by Hugh Heffner, a professor at Stanford, on the microwave parametric oscillator. Now, parametric amplifier oscillators were, at that time, microwave devices, in which you modulated by applying an external alternating field of voltage to a resonant circuit, electrical circuit, RLC. You can make an optic resonator, if you have a resistance, a capacitor, and an inductance in parallel. If you excite it with a voltage and let go, this will keep ringing, oscillating. Half the time, the energy will be in the inductance, and the other half in the capacitance and go back and forth. If not for the resistance, it would do so forever. In the microwave parametric amplifier, this capacitance is a p-n junction, and the capacitance depends on the voltage.
It's not a number. If you plot capacitance as a function of voltage, it's something like that. It's nonlinear. If you apply a voltage of, let's say, cosine omega T or V, V of T–this circuit resonates at a resonance frequency, which is equal to one over the square root of LC. And if the applied voltage now is alternating, then the nonlinearity means that this capacitance is modulated at twice the frequency as well as the fundamental. Because the nonlinearity, if you do Taylor expansion, you get squared, cubed, and the square will mean there will be a component now of the voltage at twice the frequency, and that's the criterion for closing the circuit. That's what parametric oscillators and amplifiers do.
Lord Rayleigh had shown that if you modulate an energy storage parameter of a circuit, in this case, the capacitance, at twice the resonant frequency of the circuit–the applied voltage here has to be at twice the resonant frequency, which is twice–Hugh Heffner then gives a talk on this amplifier resonance principle and microwave amplifier and claims that it is a better amplifier than the maser, before lasers. But the meeting was on both, really. And he presented the theory for explaining it. And it did follow from his theory that this amplifier would be quieter, would add less noise, which is a figure of merit of a good low-noise amplifier to an amplified signal.
ZIERLER: Is it a theory because it's not yet built, we're not testing yet?
YARIV: No, it was built and tested. But it wasn't pushed to the limit where it could be compared to a laser. But the theory was more or less considered correct, so it was a quandary. And I, a first-year at Bell Labs, raised my hand. And I had taken a graduate course in quantum mechanics and quantum optics in Berkeley but never used it. I said, "Is it possible that you obtained your result because you analyzed it classically while the laser is essentially a quantum device?"
ZIERLER: What's the distinction, classic and quantum, in this context?
YARIV: The quantum description of light uses a formalism, one of the most elegant you can imagine. That formalism predicts, without going too deeply into it, a range of new phenomena that cannot be explained classically. They drop out of the mathematics of the quantized treatment using annihilation and creation operators. You basically use a theory which incorporates the uncertainty principle into it. The result is that no device can be an ideal noiseless amplifier. That will contradict the uncertainty principle, and that's the mistake that Hugh Heffner made. Hugh Heffner didn't know quantum mechanics. I knew him very well. He was a genius, but in microwaves. And if you don't take quantum mechanics, there's no way in the world you will ever get the result.
And I went back to Bell Labs, dusted off my classes from Berkeley, started this quantized field theory. Glauber gets a Nobel Prize later on, not for that work, but for the whole idea of quantizing the electromagnetic field. And one consequence is that you cannot have a perfect amplifier. I was able, within a week or two at Bell Labs, to get the correct result for the correct reason. To first order, you can do it very easily, and I did, and got immediately the residual noise of Hugh Heffner's amplifier, which he analyzed classically. If you did it quantum mechanically, it would be identical to that of the laser. Both of them were limiting cases of ideal amplifiers, limited only by the uncertainty principle. In the process, I needed to quantize to get the Hamiltonian of the electromagnetic field. And that treatment is, I think, the first ever quantizing of nonlinear optics and the starting point for a lot of other things.
ZIERLER: What was it in Heffner's presentation that made you say, "A-ha, there needs to be a quantum approach"? What was missing as he presented it?
YARIV: His analysis was a classical electromagnetic analysis that any good circuit expert could have done. He applied it to the parametric amplifier. Nobody else had done it before. Given that you have the power of quantum mechanics, after Hugh Heffner, that field–Hugh Heffner came from Stanford. The field I'm not sure started at Stanford, but they had a strong effort. But even Bell Labs had a strong effort done by electrical engineers. And there's a paper by Weiss and company, two or three people, where after ten pages of mathematics, they derive some of the basic features of a microwave parametric amplifier. Using the quantized approach, which I applied you get them in two lines. The power of quantum mechanics. One result of the analysis is the prediction of spontaneous parametric fluorescence, which is a new physical phenomenon. [laugh]
If you take classes of crystal–the crystal's to be non-central symmetric. Every crystal in the world has a rigorous description of its internal symmetry. And that means there are operations that you can perform on the crystal and return it to its original state. Or in plain English, if a crystal has cubic symmetry, then there are axes normal to the faces of the cube, where if you turn the crystal by 90 degrees, you cannot tell that the new crystal, having been rotated, is different to the original one. Those are called central symmetry crystals. The first cut in crystallography of crystals into central symmetric and non-central symmetric crystals. And nonlinear optics is essentially based on non-central symmetric. The effect for mathematical reasons, a simple one, doesn't exist. One such example is potassium dihydrogen phosphate, a non-central symmetric crystal. There are hundreds of others, thousands.
And if you shine light into the crystal–and this is light now. When you know the amplitude of the light, that's optical now. It's very large. It turns light at a frequency omega 3. That's the applied optical field. If you do that, out of that crystal will come pairs of photons, where omega 1 plus omega 2 is equal to the applied frequency. The quantum mechanical description of that is that photons at the input frequency, omega 3, which is the high frequency because it's equal to the sum, get annihilated, and instantaneously, two new photons are born, the frequencies of which add up to the original. That's conservation of energy. That took the people, the two or three pages of mathematics, directly [into] conservation of photon energy. That's all. The other relationship even has a name. That's a new physical phenomenon, and that dropped out of nonlinear analysis. You want me to write the Hamiltonian down?
ZIERLER: Sure. But speak it out so we capture it.
YARIV: The Hamiltonian can be described as the product of the creation operator times the annihilation operator and some bunch of constants, which includes the nonlinearity, obviously. That only happens because the lack of central symmetry of the crystal, some number, which we can measure, and that Hamiltonian plus other terms, which are non-resonant, predicts just that. Quantum mechanically, this photon is annihilated, so the quantum state, omega 3, omega 1, omega 2–the initial quantum state has N3 photons. The field is quantized. It's characterized by how many photons it has in it. It has N3 photons at omega 3, N2 photons at omega 2, and N1 photons at omega 1. That's an eigenfunction of the original electromagnetic state.
ZIERLER: What's an eigenfunction?
YARIV: Quantum mechanics is all about eigenfunctions. If you investigate a state, could be an electron, an atom, a mode of the electromagnetic field, according to quantum mechanics, you can only find it in prescribed states and no other states, and in each state, it can have definite energy. Those are the eigenstates. Quantum mechanics is essentially solving for the eigenstate. They can be time independent or time-varying, and each eigenstate has its eigenvalue, the allowed energy. So the quantization of quantum mechanics is, if you measure the energies at which you can find the electron of the hydrogen atom, it's a single proton surrounded by one electron, if you analyze it using quantum mechanics, solve the Schrödinger equation, what comes out of it is the eigenfunction, meaning a description of the state in which the electron can be found, the description in space and time.
And as a byproduct, every eigenfunction has associated with it an eigenvalue. That's a mathematical statement. That's very much part of linear algebra. Each eigenstate gives you mathematically the description of the electron in space, which you use to calculate properties of the electron. In addition, it gives you the eigenvalue. Each eigenfunction is such a number, which drops out in mathematical analysis. If you look at my book, you'll see all of that. And those are the allowed energies, the quantized energy that Bohm tried to explain. Indeed, to some degree. This process is described as a transition brought about by the nonlinearity of the crystal, in which a photon at omega 3 is annihilated, and since the energy is conserved, two new photons are generated.
The transition from the initial state, the interaction Hamiltonian part of the–A3, A1 bigger. In the new state, the initial mode of the photon lost one unit–a photon is annihilated, so the number of photons drops by one. At the same time, two new photons are generated, so N1 increases by–that's a quantum mechanical description of the transition. That ports directly out of quantum mechanics, and that's the so-called matrix element. And it tells you, by looking at it, right away that you can annihilate a photon because the A3 here doesn't have a dagger. That's the annihilation operator. It means a photon is annihilated, taken out. And those are, with the daggers, creation operators. What they do is, they connect the state, which contains, as an example, N3 photons at omega 3, the pump, and N1 photons at omega 1, N2 at omega 2. Annihilates one, so the final initial state has one photon missing from the strong pump laser that's a source of energy.
And simultaneously, the number of photons in the two other frequencies increase. And the beautiful thing of that is, if you take an initial state in which N1 and N2 are zero, meaning the fields at omega 1 and omega 2 are not excited, classically, zero energy, this is a number you can calculate that is nonzero, meaning you are creating new photons, even if there are none there to begin with. Well, that, by definition, is a noise. If you create electromagnetic field energies, which are not related to an input, an amplifier has to replicate the input, that's a noise. That's the fundamental look at quantum mechanical noise and how it limits amplification. And we wrote the first paper on that.
And the result is that Heffner's classical microwave amplifier, in the limit, was as good as a laser. Though, it took a lot more work to get it to reach it. Never really reached it for practical reasons. That phenomenon, in which you get two new photons, by turning the crystal around, omega 1 and omega 2 change. They always satisfy this condition. You can tune the output here because momentum has to be conserved as well as energy, and that determines what photons are generated. That's elegant, that point of view and that language.
ZIERLER: What made you think of the uncertainty principle at this juncture?
YARIV: I didn't think of that. The uncertainty principle is built into quantum mechanics. If you apply quantum mechanics correctly, solve the Schrödinger equation in a given situation, then the results are consistent with the uncertainty principle if you made no mistakes. In this case, it's probably, I would say, the most dramatic case you can imagine, or one of them, where it just drops out. This was a new physical phenomenon, playing a key role in modern optics and quantum communication today. I have strong evidence, including one of the foremost professors in the field, giving us credit for that. I did that with two other people.
ZIERLER: Who were the other people?
YARIV: Essentially, what I've done here, I'd done within a week of coming back to Bell Labs. I was still relatively fresh on quantum field theory, although I've never used it. I also read up on Glauber.
ZIERLER: Did you talk to Glauber at all?
YARIV: Yes, we're good friends. The paper is a few pages with mathematics, but the crux is here, and I had that result. At that point, I sought the help of a very good mathematical physicist at Bell Labs, Bill Louisell, later a professor at USC. And he added and polished that into the paper.
ZIERLER: What were you lacking in terms of the math that you needed his help?
YARIV: My analysis here essentially looks at the first order. It's a good analysis of how much noise power is generated. But if you want to ask a more difficult question involving correlations and so on, it's not powerful enough. You have to go into extension. And my friend was conversant and familiar with the mathematics. He was a good mathematician to begin with.
ZIERLER: Was Glauber at the conference in the Catskills?
YARIV: No, but he started showing up later.
ZIERLER: What about Charlie Townes? Was he there?
YARIV: Yes.
ZIERLER: Was he in the audience during your exchange with Heffner?
YARIV: Probably, yes. But probably didn't pay much attention. Charlie Townes never got involved in nonlinear optics.
ZIERLER: It was outside his area.
YARIV: Yes, probably never quite understood it. To understand it, you really have to be immersed in quantum–and he was in spectroscopy.
ZIERLER: And for you, you could go back to graduate school for your education, for your background in this.
YARIV: Well, I was lucky, and I showed up in Berkeley…
ZIERLER: At the right time.
YARIV: Out of Tel Aviv to Berkeley with half the professors in the physics department having just left Los Alamos or Lawrence Livermore. They were all there teaching.
ZIERLER: That was the place to be.
YARIV: Yes. But the truth is, I learned quantum field theory mostly from Bill Louisell. He really taught me. I knew that much, but that was good enough to write a good letter. But we went a step further.
ZIERLER: Now, when you challenged Heffner in this moment, because he didn't have the quantum background, was he in a position to say, "Oh, of course, you're right," or not?
YARIV: I didn't challenge him saying, "You're wrong," it was really more of a question. "Is it possible that because you're analysis"–and it's difficult to say no. Because again, a typical electrical engineer didn't know quantum field theory. Even a typical physicist didn't know quantum field theory. It's not needed to do spectroscopy. When I did my PhD thesis on a maser in Berkeley, my physics professors, all of them, didn't really know what I was talking about. Later on, they would, but not at that point.
ZIERLER: Now, to come back to this idea in quantum optics, you left this at Bell Labs, but then you picked it back up at Caltech.
YARIV: I left it at Bell Labs because Bill Louisell was a mathematical physicist, of which we had a group at Bell Labs who were very good. The kernel, the basic idea of this project, the idea that there was a problem here, and there was a solution, was mine. But I think our first paper got 90% of the fame and explanation, but there was a lot of room there for mathematical refinement, but I realized I was out of my league, and secondly, I was truly not that interested.
ZIERLER: Out of your league mathematically?
YARIV: Mathematically. I'm not a mathematical physicist, and I was more interested in new phenomena, not in refining the old one. I dropped it and moved onto other things. Bill Louisell stayed with it. He eventually got a job at USC, where he stayed until he died a few years later.
ZIERLER: I'm curious–it would've been a while later–if you ever connected with Barry Simon on this.
YARIV: Oh, I did connect with Barry Simon when he first came to Caltech. And we didn't really find any common ground. It was too esoteric.
ZIERLER: Yeah, very theoretical.
YARIV: Almost everything I've done, we went back into the lab and demonstrated it. And if not, we dropped it.
ZIERLER: Besides Gould, when you came to Caltech, were you looking for mathematicians so that you could pick up the quantum optics work?
YARIV: No, no. It was my experience, and it might be somewhat arrogant, that in many of those problems, if you have a visible math background, and you understand the math, you can get 90% of the problem and the meat of it with what you have. And there are problems which I have initiated that mathematicians are still working on 20 years later. [laugh] And I lose interest.
ZIERLER: Because you can't hold it, you can't work with it.
YARIV: No, no, because the novelty's, to me–this problem, I felt I understood at that stage, in which I brought in Louisell, who was a pure mathematician, to help me.
ZIERLER: Where did quantum optics go absent your more direct involvement? Who picked it up?
YARIV: Quantum optics is arguably the most exciting part of laser physics. Have you heard about frequency combs?
ZIERLER: Yes.
YARIV: Mode-locking. Physical phenomena in which photons interact with and influence each other. And frequency combs. The German physicist Ted Hansch who was at Stanford as a graduate student, and as a postdoc. But you can now have an instrument, which will emit frequencies, starting for my microwave frequency standard locked to an atomic transition in multiples. You can generate within a wide band, any desired frequency with ions determined by near immutable atomic energy in atoms such as iridium or sodium. The atomic transition locks an oscillator, and that's the time standard, it's locked to an optical transition, maybe iridium or sodium. And then, a device which gives you integral multiples, one, two, three, all the way to 10,000, hold that frequency. You can now choose an optical frequency tied to the time standard, frequency standard, by dialing it up. Wonderful concept. It's based on mode-locking. A laser, in principle, can emit many, many frequencies.
You need two conditions for a laser, an atomic transition between two energetic states of the laser atom or ion which amplifies the wave intensity as it bounces back and forth in a resonator structure for a wave to resonate. Remember, we already talked about resonators above, in a microwave, where it can bounce back and forth. A laser resonator made of two curved mirrors can have thousands. Those are equivalent completely mathematically to the modes of a violin or piano string. First harmony, second, third, fourth, etc. They correspond to the number of half wavelengths between the two ends if you clamp the string of a piano. Those are the modes. And then, you need a gain medium to amplify that. In a semiconductor laser, you can have thousands of those modes being amplified, so the laser, in principle, can oscillate simultaneously all of the modes. For communication purposes, it seems to be undesirable because you want a pure frequency, in which you can put your information, let's say, and have it travel from Los Angeles to New York, then retrieve it.
Many frequencies just confuse the issue. Remember, the distributed feedback laser holds emits because it emitted a single frequency. Here, you have the opposite. The semiconductor laser oscillating at thousands of frequencies, but they're equally spaced more or less because the resonance condition for the resonator makes them equally spaced in frequency. And there's a mode in which they oscillate and a medium in which they phase lock. Not only do they oscillate independently, each one with its own frequency, equal spacing, their phases are locked because of the interaction between them. And that's a nonlinear optic interaction. And that is the basis of the frequency comb or optical parametric amplifiers and oscillators, which are very important. You can see the mathematics and phenomena here are all new, and every one of the analyses will start with the Hamiltonian. I will give you a Bell Labs paper if you remind me. I think I have copies here. That's nonlinear optics.
At some point, it became highly mathematical. And I dropped it. When I came to Caltech, my first two or three students started working–maybe student one and two–on semiconductor lasers. But students four, five, and six, I put to work on nonlinear optics because it was clear at that point that there were other things we could do, which we did. It's not that I sought help from the students. A good student who works for me, at least in my group, all of them, after half a year here, you have to teach me. You should be the world expert in that particular area. Some of them were superb mathematicians, much better than I was. And given a new area, a new problem, they did very interesting things. And that led to phase conjugate optics.
ZIERLER: Did you stay with semiconductor lasers?
YARIV: Until today. Actually, probably the tape in there is, but still, my post-doc next door is.
ZIERLER: Phase conjugate optics, tell me the origins.
YARIV: It's a branch of optics today.
ZIERLER: Did it exist before you came to Caltech? Is this a brand-new field?
YARIV: Brand-new field.
ZIERLER: Let's start at the beginning. Where does it come from?
YARIV: I created the name. The name is mine. I'll give you a paper calling maybe the beginning of phase conjugate optics.
ZIERLER: Let's start with the name. Why did you chose the name phase conjugate optics? What's the phase, what's the conjugate?
YARIV: You're getting into a deep and exciting area of optics. [laugh] It's a field depending completely on nonlinear optics.
ZIERLER: This is intellectually a direct outgrowth of your previous work.
YARIV: Yes.
ZIERLER: It was the next logical place to go.
YARIV: Well, as you see from the history, the field probably, to be accurate, is triggered by work in Russia and maybe even Germany. The typical experiment is, you shine light, a coherent laser light, ideally monofrequency, coming out of a laser. And you allow it to move through an atmosphere. The light, to begin with, is a pure, beautiful beam coming out of a laser, well-defined spatially and temporally, highly coherent both in space and time. You can describe it mathematically as cosine omega T, signal frequency, times the function of space. Because it's a resonator.
Dialing in Coherence for Lasers
ZIERLER: What does coherence in time mean for a laser?
YARIV: Coherence in time, I will go to the extreme. People will quarrel about that, Talmudic scholars, forever, but it really means it approaches a pure sine wave or cosine wave. You can write it as a field that exists over a region of space, the output of a laser, and described everywhere by some function of space. OK, coherent field. Would you like that I take a little more time and…
ZIERLER: Absolutely.
YARIV: OK. A coherent optical field, the field is a function of space. R means position, and time is some E naught. The field is a product of what we'll call the modal function, F of R, which describes its region in space, R, and the time dependent is cosine omega T. At any one location, let's say, inside a resonator of a laser, R is determined fixed-rate. R is the address. It's a pure harmonic so that Fourier spectrum, E is the electric field of the laser. It's Fourier spectrum, which I will write like that, or Fourier integral. Any mathematical function can be described either in space or in time. And the two are related by a Fourier integral transformation. And communication theory is based essentially on behavior in the space domain. If you make a filter, by definition, only allow certain frequencies to propagate.
You are not thinking of the time behavior, but on the frequency content of the wave. That's E. The Fourier spectrum of this wave is a delta function, a function of narrow width at the frequency, omega naught. Meaning, the wave, to begin with, as it exits from the laser, all its energy is at that single frequency, the frequency omega naught. Uncertainty principle says that that's not really true. There's a little width here, which the uncertainty principle–you asked what I mean by coherence. A wave should be a pure cosine where all the energy is emitted as a wave of single frequency. Quantum mechanics doesn't allow it, but it allows you to approach that pure state. You can approach perfect coherence but never reach it. I have spent much of my career looking for more efficient ways of approaching it. Today's semiconductors are largely based on the work of my group at Caltech. To the degree that you deviate from the condition, meaning that the Fourier spectrum is no longer a single delta function but now has a width, it becomes–now, that width can be due–that omega, finite bandwidth of a wave can be due to presence of information, to noise, including the uncertainty principle noise.
I'm moving you into quantum optics. Even before lasers, if you took a classical field, which allows you to put all the field energies at a pure single frequency, perfectly coherent, and you modulate it with information, the spectrum broadens. The information modulates it, and therefore, throws the energy away from here to the side. And this is that finite width, delta omega, due to either information or noise. Long answer to question about coherence, a coherent field is a field that at a given point, is a pure sine wave. Or if you do things to it, what you do to it is known completely classically, and therefore, you can correct for it. Coherent. But I think we started with phase conjugate optics.
ZIERLER: The name, where did the name come from?
YARIV: At this point, it'll make more sense to pull out the paper. Let's see if you can dig on the very bottom and see if you can find what says The Beginning of Phase Conjugate Optics. It'll be a reprint. Here is Phase Conjugate Optics and Real-Time Holography. Keep that, and I'll give you the quick and dirty. This is a digression, a detour. What's the basic laws of optics that you probably know? That you have a perfect mirror…
ZIERLER: And a perfect mirror is a physical reality? Or it's a concept?
YARIV: It's mathematical, but you can approach it.
ZIERLER: Physically.
YARIV: Physically, making a very good mirror, shining it well. If a wave is incident on it, denoting by an arrow–an arrow, in optics, means a wave. It is reflected, and the angle of incidence, theta incidence, is equal to theta reflection. The law of reflection, which you probably had in high school demonstrated with arrows like here. This angle is equal to this angle. Imagine what will happen if you have a mirror where an incident wave like that is reflected like that. That's radical. Talking about optics. The whole field of optics will stand on its head. New physical phenomenon. And the question is, how do you get that? The field that resulted from that question, and you'll see how we got to it, is known as phase conjugate optics. Why phase conjugate? This is a phase conjugate optics or PCO. This is a perfect mirror, classical. In a classical mirror, an incident field is reflected, law of reflection.
In this hypothetical phase conjugate mirror, the mathematical description of this field is some amplitude E to the I omega 2 minus K dot R. This is how you describe a plain wave. The simplest description of a traveling optical wave is that term right here. In phase conjugate optics, the arrow description here corresponds to the wave returning exactly back. It's like playing a movie reel backwards. In nonlinear optics, or in specifically phase conjugate, the incident wave, call it one, is E naught E to the I omega 2 minus K dot R. And the reflected field is E naught E to the I omega T. This minus becomes plus. That's a pure description, accurate, of the reflected field. This is the incident, this is E reflected. This is one, and this is two. This two is this two here. The field coming back. One can be rewritten as E to the I omega T, E to the minus I K dot R. I simply multiply out the exponent.
That's the incident. E reflected is E to the I omega T, E to the plus I K dot R. This is the phase. That's a number, which changes position. That's what makes it a wave. This combination gives you a traveling wave, the simplest description of a wave anywhere. Notice that this part is the same, omega is the frequency. This number here is the negative of that one. If you give a mathematician two numbers, in which a difference is–that's the phase. The complex part of it, one is negative. The other, they call it the complex conjugate. Pure mathematics, lesson in complex algebra. In mathematics, the second year, of course, in complex numbers, a number can be written as A naught cosine omega T plus phi, frequency phase. And the number A naught cosine omega T minus phi is the complex conjugate. A mirror, which retro reflects rays rather than–we didn't have a mirror.
We now have it due to our field. But before phase conjugate optics, this was considered a dream. People didn't even dream that you could make a mirror like that. But if you could make a mirror like that, then the description of this mirror would be that a reflected field mathematically is the complex conjugate of the incident field. That opens up a new field in optics. And I'll show you one example. If you just accept that you have a mirror–you see, if I have a complex field and not a simple plain wave, a field with information, meaning that the field, if you mathematically analyze it due to Fourier now, it has wavelets going in all possible directions. And the field is the sum of all of them. Each one of those wavelets, you can break any complex field into a collection of plain waves. That's Fourier analysis. Probably the most powerful mathematical tool in physics. A genius. Any wave is a combination of waves. Even a wave of a pure single frequency.
The spatial part of it gives it an assembly of directions, and each one of those directions, when incident on that magic mirror, soon will be reflected, obeying the law of reflections, back. And an immediate consequence of that is that if you have an ideal plain wave, incident on incident, a mess. A bottle of Coca-Cola, the effect would be–this is E to the I omega T minus K dot R. A pure plain wave going forward. When it comes out of it, the frequency doesn't change because that's an inert frequency. But the direction will change. If you can now have a phase conjugate mirror, so coming out, still propagating forward, but it's not an ideal plain wave, so describe it like that. If this wave carries information, pictorial information, as an example, by having it pass through a transparency, as an example, up here, you could put a camera, and re-image it, and see the picture. If you did it here, you'll get a mess.
ZIERLER: Why is that such a mess?
YARIV: Because each direction, it will give you a dot. And pure imaging means that you can re-image it. That essentially scatters the light into infinite directions, and each direction like that is imaged into a different spot on a film. Therefore, you get white noise. The coarser the bottle, the worse the picture. But if you now take that wave and reflect it from a phase conjugate mirror, that hypothetical mirror that has that retro reflection property, that wave will go back, and regerminate itself, and come out ideal, virgin. Anyhow, that's the barest, most fundamental–the component plain wave of a complex picture. The mathematics are in the paper. One example, high-power lasers, especially for the military, and industry, welding and so on, are a very desired commodity. Like welding, you want to take the output of a laser and concentrate it into a small spot. Let's say, a welding laser. This is the laser, and here's a lens. And you take the outward field of the laser and focus it down, and this is where you do the welding. Here's a gain medium, the medium that provides the gain.
A laser requires a resonator, that's the mirror. Two of them, here and there. That establishes the mode of every laser. And then, the medium here, which is pumped with energy to supply the gain. We know now that a laser has to have gain. And then, you image it down, and you do with the welding, or you do so in enemy missile. High-power. But this medium now gets very hot because you're dumping energy into it, and the result, instead of being an ideal medium, it becomes more like a Coca-Cola bottle. And the result is, you will not get a spot like that, but maybe actual spot due to thermal instabilities. You dump energy into this medium, it's going to boil. [laugh] That's an extreme. A medium, if you should dump a lot of it, is not going to be an optically good material.
Parts of the medium will be hotter than others, distort the wave. High-powered laser, the medium here gets heated up, and the result is that this ideal picture of a wave bouncing back and forth, the result is that the optical quality of the laser beam coming out is distorted. One manifestation of this degradation, for example, is that and instead of being able to focus the light into a small ideal spot, it is now into a much larger spot, which is undesirable and in many cases it is unacceptable. If it's an enemy missile, then the energy density which burns a hole is reduced, may not do the job, or you will not get a good weld. If I replace one of the mirrors with a phase conjugate mirror, I already talked about how it will repair the damage, and that laser will give you an ideal spot, even when the junky medium inside will try to otherwise spoil it. There are many others. You see details, you'll probably ask me more questions. Now this is a major branch of optics, of sessions in phase conjugate optics.
ZIERLER: So far, this is all equations on the paper. How does your lab change when you move into this field?
YARIV: I have students, actually, working on lasers, and those are the two main areas. In the lab, we learned how to make semiconductor lasers, and we did. We were probably the first university in the world to make semiconductor lasers. And we were taught that by a bunch of Israeli engineers, the initial step, then we went on from that. Other students are doing phase conjugate optics. At some point, we brought them together for a while, and that's important.
ZIERLER: What was the benefit of bringing them together?
YARIV: It was clear at some point later on that the most important lasers are semiconductor lasers. Every other laser has a specialized purpose, one for removing spots from your skin by dermatologists, for welding cars, but there was one laser, which was a communication, effective like a transistor in its generic, universal importance in semiconductor lasers. Because it can be run by electronics, and it's small, and it's efficient. And it can be made part of a bigger picture in integrated optoelectronics, which we'll probably have to talk about sooner or later. Therefore, we already had a lab where we were making semiconductor lasers and the application of semiconductor lasers, which eventually led to mode-locking and frequency combs. Basically, when you take a field like optics, which at that point is an "inspectable" field with universities like the Optical Science Center at Tucson or the School of Optics based on the conventional law of reflection, and now say, "We are changing that law. Instead of going like that, it's retro-reflected," you are going to shake the foundations of the field, and that's what we did.
Bringing Lasers to Business
ZIERLER: When did you start to get involved with startups? When did people start to see the commercial viability of these lasers?
YARIV: I told you the story that I gave my first theoretical paper on the feasibility of a new technology in which you combine optics and lasers on a single crystal in a conference in Japan. The exact year, probably the late 1960s, '68, '69, maybe early 70s, and was approached by somebody from DARPA who asked if they could support the work, and then they supported us for 30, 40 years continuously, very generously and consistently. That led, at some point, to the fact, if you fast forward another probably 30 years, the fact that in my group, we had the best lasers in the world, meaning the fastest. At that point, we understood that semiconductor lasers would be used primarily for optical communication. Optical communication, you put information on a laser beam, you modulate them, in an electrooptical nonlinear crystal. The faster you can turn the laser on and off–every time you turn lasers on and off, you create a one or a zero–you have more information, obviously, more bits per second. Fast lasers became the important thing to do. And we have learned here in my group to make lasers that could be turned on and off at the highest rate. We have lasers that could be turned on and off, 30, 40 years ago, at a rate of ten-billion times per second.
ZIERLER: Is that what it means to have a fast laser, how fast it turns on and off?
YARIV: Yes, exactly. As a matter of fact, in the first days, the laser was turned on and off literally to generate the pulses. But the data rate has become–the appetite came with eating, and communication has moved, Netflix movies, data centers, to a point where they couldn't turn the laser on and off fast enough, even a small, miniature semiconductor laser. And the way it is done today is, you let the laser oscillate, emitting a steady beam, and it moves through a nonlinear crystal, and the signal bearing the information is applied to the crystal, so the modulation of the laser beam is done in the external crystal after it exits. But you want to make sure that the light coming out of the laser is as pure as it can be. Because otherwise, modulating a laser beam takes the initial laser beam, the Fourier spectrum of which is a delta function, meaning all of the optical energy is concentrated in one frequency, and the information spreads it. Noise will also spread it, so you have to–signal-to-noise ratio, which is a figure of merit of a good amplifier, is degraded.
A good optical communications system consists of a laser emitting a pure wave, a wave which has a single-frequency output, or its equivalent, and then sending it through a crystal, which is being modulated with the information. At that point, you needed quick lasers. And a simple calculation had shown that one of those lasers could handle all the telephone traffic of Los Angeles. And we had them here. My students made them. A little story, there were a lot of theoretical papers at that point, not from us, as to how quickly you can turn a semiconductor laser on and off. And one paper from Bell Labs made the prediction, gave a formula that there would be a limit to how fast you can do it. And one of my graduate students here, Kam Lao, a talented student–and then, Bell Labs described experimental papers, in which they showed that prediction was right, that lasers could be modulated up to, more or less, that frequency. Well, Kam Lao discovered that the mathematics were wrong.
We can go into details. Actually, they didn't make a mistake, but the theory it predicted gave an expression that had two terms for the limit of the noisiness or the speed at which you can modulate the laser. And they claimed that one term was much, much smaller than the other, and they threw it away. And they threw away the baby with the water. And the other term is the one that predicted a limit. And lo and behold, they found that limit. Well, it turned out that the term they threw away was the one to keep, and that predicted a limit ten times higher. And we made that limit. The question is, why didn't they find that in their experiments? The point is, they had a number they were looking for. Once they found it, they stopped looking. [laugh] It's natural. They were not cheating or anything. They had a result that agreed with their experiments. Their experiment was not careful. They had parasitic distorting–and it happened by accident to fall within the realm of the prediction, so they declared victory.
And so, we had a laser for four or five years, which was the fastest the laser acknowledged to be. And at that point, two or three students whose doctorates were based on that research were about to graduate. And with it, we would've lost a major capability, although other students were stepping in. And that work was supported by DARPA. And when those two students were about to graduate, maybe a year before, DARPA came to us, the fellow who supported our work, and said, "That is an important capability, and I don't want it to get lost. What would you people think if we, DARPA, gave you a basic support to start a new company making a product?"
And that was the basis of Ortel. One of the two fellows was an ex-captain in the Israeli Army who came to me as a post-doc, graduate of Tel Aviv University, very good, very careful, very organized. And the other one was a son of a Los Angeles rabbi. Nadav Bar-Chaim and Israel Yuri. Nadav and I met each other in Israel. There's a university, we find ourselves with the fastest laser in the world, acknowledged now. Big embarrassment at Bell Labs. [laugh] They should've done it.
ZIERLER: Was DARPA more interested from the communications side? Because of course, they were fundamental to the development of the internet. Or were they more interested in the weapons aspects of lasers?
YARIV: I think our semiconductor lasers did not, at the time, look like they could have–the power levels we were working with was a milliwatt. To get down to military applications, you are probably talking about kilowatts, meaning six orders of magnitude or more power, which needs to be concentrated on the target or whatever. But the fellow supported us almost blindly for 30, 40 years. Because they sensed we were doing important work. The power level was such that probably the application was mostly communication. At that point, I feel, it was clear.
ZIERLER: Could you see, in their interest in the development of the internet, the value of this research to what DARPA was doing?
YARIV: DARPA has good scientists working in the government, supervising projects. And we were way beyond them. Obviously, they knew all of a sudden that we had a small communication-based laser, which was a factor of ten at least better than anything else. A factor of ten more in data capacity. Maybe a second for downloading a Netflix movie instead of a minute or whatever. We're not talking about DARPA the monolithic organization. We're talking about one person at DARPA. And at that time, those people had a great deal of independence. And they could more or less support a project, not over budget, but pick one or two projects they thought had viability. And they've lost that ability, I think, since then.
ZIERLER: When did Ortel start to catch the attention of the big companies?
YARIV: I forget the exact timing, but basically, what was happening in the meantime was that the field of optical communication was growing by leaps and bounds, and now there were major conferences, and it was understood that it was the future of optical communication. The optical fibers were invented in the meantime–not by us, we had nothing to do with it–as the means for transporting information long distances, under the ocean. I think Ortel probably started 1980, give or take. Our first clients were the Department of Energy, Los Alamos. Because there was a treaty then that banned atmospheric testing of atomic weapons, so they started doing testing underground. Typically, you will have a nuclear explosion with a whole room full of instrumentation right next to it to measure pressure, temperature, whatever. But the equipment would be destroyed. You needed to get it out before the soundwave, or the earthquake destroyed the instrument. That meant a quick laser, a laser that could convert the pressure wave to an electrical signal and send it down a fiber.
ZIERLER: You mean from the sensors that would get destroyed, to transfer what the sensors were?
YARIV: If you measured pressure, your pressure sensors at the site of the explosion or a few feet away.
ZIERLER: They'll get pulverized.
YARIV: Which will transfer the pressure wave into an electrical signal, current, which has the pressure information on it, calibrated, which is sent out to a laboratory a mile away or whatever. And we had the only lasers that were fast enough. You see here that the property of the laser that you needed is speed because you're talking about nanoseconds maybe.
ZIERLER: But again, speed is defined by how fast the laser turns on and off.
YARIV: Exactly.
ZIERLER: So distance is not a consideration?
YARIV: No, because optical communication already consisted of sending information in fiber, or without fibers, distances of tens of miles. Distance was not a consideration. Speed. Get it out of there. We made our living there for maybe–it was a gradual process. That was our main client, but enough to keep 10, 12, 13 people busy. Very good people, the best of the best. And one day, one of our engineers in the company, one of my best ever graduate students from my group, Hank Blauvelt, who's probably, as we speak, two doors away, working not with me but with a fellow professor. Oh, before Hank, we realized that we couldn't make a living supplying the Atomic Energy Commission with lasers which self-destruct. We looked around for other things to do, and one day, one of our engineers, I forget who, had the brilliant idea of applying it to cable TV.
Since you're interested in the story of Ortel, that's important. And that time, and I'm talking maybe in 1985, if you subscribed to cable TV, you probably had an offering of less than 50 channels, carrying information, movies, gardening, golf, whatever. And the way the programming arrived at your home, there was a communication satellite somewhere, South Pasadena, Alhambra, literally, a big parking lot with maybe half a dozen huge parabolic antennas aimed at different satellites in spaces, beaming down different programming from communication satellites, which already were used for that purpose. And the channels they were offering, channels 1 through 100, or 1 through 50, were assembled there on the site, made to ride piggyback on a microwave, which was sent in a co-ax cable from South Pasadena to Pasadena, maybe seven or eight miles' distance. Along the route, you needed to amplify it maybe ten times because it would die.
It was carried on maybe a wave of 100-megahertz carrier wave. All the movie information was riding on a microwave signal, piggybacked, and it decayed, so you had to amplify it. It was clumsy, coax cable. Amplifiers every half a mile or so. Noisy. And the engineer at Ortel had the idea, "Let's do it with a laser beam. We can probably get that distance without any amplification because optical amplifiers were only used every 20, 30 miles." The signal can go a distance of, let's say, ten miles with hardly any need for amplification. Optical fibers were that good. We tried it in Alhambra, where the company was located, and it worked. We put the receiver on the roof, brought down the conventional signal. We took it out of microwave cable. We subscribed to one of the channel companies and displayed their program on a TV set, and then we picked it directly from the sky, took the same programming, and sent it through a fiber. And it looked equally good.
We took a conventional picture that we got as a subscriber, and one in which we sent information through a fiber. And it looked good. We showed it in the next annual cable TV show, which was done in Anaheim, next to Disneyland, where there was maybe 20,000 people. It was a huge show by that time. Cable TV is a big business. A lot of scantily clad ladies sitting on new cars. [Laugh] You know the typical picture in one of those. Our exhibit, though, had two TV monitors, one conventional showing the picture, one where the picture is 10, 12 miles in a fiber, 20 miles. We had a line of engineers there all day long who couldn't believe it. To convince them it was optical fiber, they could actually take the fiber link next to the microwave link, separate it, and put it back together. And you could see the excitement on their faces.
ZIERLER: This was a game-changer.
YARIV: A game-changer. But then, at the end of the day, a good microwave engineer from one of the companies said, "This is game-changing, but there's a slight problem with your system." He showed us that on the screen, every now and then, there was a fleeting shadow moving by on the other screen, which we ignored. He said, "That is because your transduction, your modulation of the microwave information you get from the satellite, in modulating the laser, is not perfectly linear." And he could tell immediately that that shadow was a result of that nonlinearity. I said, "I thought our laser was very linear." He said, "Yes, but not quite enough." I said, "How linear does it have to be?" He said, "A part in a million."
ZIERLER: And where were you?
YARIV: The deviation from a straight line of the conversion of microwave to optics, transferring the information, had to be good to a part in a million. We were maybe off by an order of magnitude, which would've made little difference, but that made it unacceptable to two microwave engineers.
ZIERLER: You could fix it, though?
YARIV: Well, here's the story. In the meantime, the idea spread immediately. We still were a bit short of money. A microwave company, General Instrument, I think, was the name, came to us, and we wound up signing a contract with them. They gave us half a million dollars, and we gave them a monopoly on the product for a year or two. And they agreed to give us the money. With that money, we plugged it back. By that time, other companies, including AT&T, the Bell system, understood what was going on. Their whole industry was depending on a linear laser, so the word came to Bell Labs, and I had half a dozen students at Bell Labs already, to make a linear laser. Bell Labs can do anything.
But to make something so complicated as a semiconductor laser, linear to a part in a million, is impossible. Because you don't understand the physics well enough, and you can't control it well enough. At Bell Labs, something like thirty PhDs were working on it, and the way they got the linear lasers was, they would make 10,000 of them, and check every one of them, and one or two of the 10,000 would happen to be linear. But that's no way to run a business.
ZIERLER: Because you can't replicate it.
YARIV: That's right. And it's so expensive. You cannot have enough. One of the engineers, Hank Blauvelt, who's sitting two doors down, said, "Well, the lasers are nonlinear, but it's probably logical, reasonable, that the nonlinearity is fixed, doesn't vary randomly. Let's correct for it." If a system is linear, that means that the output, whatever, and input is a perfect straight line. The two are fairly obvious. We are talking now about–I'll exaggerate–if a system is nonlinear, there may be slight–and that deviation here, remember, has to be down to one part in a million to be acceptable cable TV. How do you detect this nonlinearity? In this case, if you modulate the input with information, let's say–let's take it as a pure sine wave. And you detect it here with a detector. If you detect that light with a good detector, you will get back the pure sine wave, or Beethoven's 5th Symphony, if that is what you used to modulate the laser beam instead of the pure sine wave. This is nowhere written, but I don't want to go into more. If it's nonlinearity, it stands to reason that if you now apply a pure sine wave–in the case of a nonlinear system, which was our laser, if you apply a pure sine wave, and you detect it, in addition to the original pure sine wave that you detect, you will now also measure a very slight second and third harmonic. If you use a Fourier analysis, that's a consequence. The difference between a perfect linear system and one with a little nonlinearity, here, the modulation generates new frequencies. We took each laser, and modulated with a pure sine wave, and measured how much second harmonic, twice the frequency was generated. That should not be there in an ideal laser. We measured it and then applied to the input an equal amount out of phase to cancel it out.
At that point, we generated a code name, and we threatened everybody with–we never used the word linearity anymore. And then, there was a major conference, and that was one of the side stories. Bell Labs became very conscious of the importance, and a whole number of people at the lab were told to make the world's most linear laser. And there was no reason why they couldn't. It was the best lab in the world. They were trying to learn the physics of the semiconductor laser and make it linear, which is impossible. It's not been done, even today.
ZIERLER: It's theoretically impossible? They're still trying?
YARIV: Theoretically, everything is possible. Practically, one laser in a million may turn out to be linear, but you cannot build a business on that. We could make every laser perfect with a $1 expenditure. There was a conference at that time where a Bell Labs scientist gave a talk on why you could not linearize a laser. And the following paper was from a commercial tv company actually using an Ortel laser in their system, showing that the laser was perfect.
ZIERLER: What was the cable companies' response?
YARIV: Cable TVs switched within two or three years, 100%. We could now transmit a perfect single from Alhambra to your home in Pasadena with all the needed fidelity, which exceeded that of the microwave system, with no need for re-amplification en route. And that changed the whole field. Today, it's actually done digitally, so linearity's no longer that critical. Things have changed. That was the beginning of Ortel. Oh, the reason that we were so secretive about it, a year or so before that happened, we got a letter from the Bell Labs patent department. Nothing to revert. At that time, they realized we became a legit company, and the letter said, "It's come to our knowledge that you people have been violating our patents, so you're being put on notice. Cease and desist." Something like that. We wrote back and said, "What patents are we violating, so we can avoid it?" They said, "It's for you to find out." It was essentially a hostile move trying to get access to our patent portfolio. Before they realized the importance of the laser. They became persona non grata to us independently of this development, which I've described. A year later, when they were trying desperately to find out what we were doing to make a perfect laser, we had a secrecy code. Eventually, they bought us.
ZIERLER: Bell Labs bought Ortel.
YARIV: AT&T. Bell Labs is just the research arm of it. Yes. At that time, they had loosened. That was after Judge Greene broke them up. That business part became Lucent.
ZIERLER: Last topic for today, you said you had a Feynman story with quantum optics.
Neighbors with Feynman
YARIV: Couple of Feynman stories. Feynman and I were neighbors, lived within a block or so of each other in Altadena.
ZIERLER: Oh, really? What was his house like?
YARIV: Oh, it's a nice part of Altadena. A lot of old Spanish houses. Old meaning 20, 30, 40 years. Red roofs, mountains nearby. Kind of an ideal location. A very nice house. Not ostentatious, but very comfortable. Kind of house you'd expect from upper-middle income. Not top income, but a good doctor. [Laugh] We lived a block away in a house not quite as nice. I was a young, new professor. I remember one day, there was a coffeeshop in Altadena that was very popular. And occasionally, Feynman would be there, often with his son. And the signature experiment in those days in nonlinear optics was one I already described to you, where you shine a blue beam into a nonlinear crystal, a crystal lacking symmetry, and in the crystal, part of the light will be converted from the input blue beam into a red or infrared beam. And the convergence is lossless, incidentally. The mathematics which best describes it uses "creation" and "annihilation" operators and is super elegant.
Feynman and I ran into each other, he with his son, maybe 7 or 8 years old, I with my daughter. And Feynman said, "That gentleman at Caltech converts blue light into red light." [Laugh] The other story is, a few years later, at that time we were already considered one of the cofounders of the field Phase Conjugate Optics. And Feynman saw a short description of phase conjugate optics. The idea that you can, in real time, reflect a wave and making it retrace its path in two ways. Mathematically, you change the sign of the phase. In reality, it's like playing a movie backwards. The wave that went through the Coca-Cola bottle and as a consequence became highly distorted, now goes back and regains its original virginal condition, including information. You can take an image, lose it completely on its original passage through the Coca-Cola bottle, and regain it coming back.
Phase conjugate optics, in description, on the basis I just gave to you, started spreading, and Feynman heard about it. He came to me one day and said, "Explain it to me." I gave him a ten-minute description, and he understood it mathematically in no time at all. But while I explained it to him, I made a condition, I said, "I am now approaching in my class a point where I will talk about phase conjugation objects. Why don't you teach my class for me?" He accepted. He gave a lecture on phase conjugated optics.
ZIERLER: Did he ever incorporate that into this own research, what he was working on?
YARIV: I don't think it lent itself to his work. There are probably mathematical operations in quantum field theory and so on that involve phase conjugation, but not for that purpose. And the answer is probably not.
ZIERLER: Did you ever engage with Feynman in some of his early ideas that would go on to inform quantum computing?
YARIV: No. I really was never involved in quantum computing. More in generating maybe the infrastructure, the detectors, the lasers, the modulators, that would be needed, but not in the actual computation. You're aware of work going on right now here, yes?
ZIERLER: Yeah.
YARIV: Is it an Apple or Amazon center?
ZIERLER: It's an Amazon center. This is what John Preskill is leading right now.
YARIV: Preskill? I thought my colleague here…
ZIERLER: Well, it's a big operation.
ZIERLER: Well, next time, we'll pick up on integrated optoelectronic circuits.
YARIV: OK.
ZIERLER: Excellent.
[End of Recording]
ZIERLER: OK, this is David Zierler, Director of the Caltech Heritage Project. It's Wednesday, November 17, 2021. Once again, I'm delighted to be back with Professor Amnon Yariv. Amnon, as always, great to be with you.
YARIV: I will use a Spanish trick and say igualmente, which means, "Equally so."
ZIERLER: OK! Today, we're going to discuss the origins of optoelectronic integrated circuits. The first question there, at least intellectually, if not technologically, can you trace the origins of this work back to Bell Labs? Or is this purely a Caltech story?
YARIV: It's both. The proposal for integrated optoelectronic circuits–we need a short name for them. Should we call them OEICs?
ZIERLER: OEICs.
YARIV: We'll call them OEICs. The proposal and demonstration of OEICs was done completely at Caltech. But the theoretical, intellectual origin, the understanding of the possibility, was obviously connected with my work on semiconductor lasers. As you'll see later, we are combining semiconductor lasers with transistors, electronics. And my work on semiconductor lasers, the role of waveguiding, started at Bell Labs. Caltech can claim, essentially, most of the credit. But everything's connected. Everything we do today is related to classes I took in Berkeley I took as a graduate student. So do I give Berkeley credit? Yes, I do. [Laugh]
ZIERLER: Just mechanistically, then, tell me what is the physical relationship between OEICs and semiconductor lasers?
YARIV: OEICs are integrated optoelectronic circuits. We go back to Bell Labs in '62, when I basically begin to work on semiconductor lasers. And semiconductor lasers, at that time– and you remember, those were not invented at Bell Labs. GE and IBM get credit. That's the gallium arsenide semiconductor laser. And it's made of three layers of gallium arsenide are pre-doped. Crucial to every transistor. Everything done today is doping. That's what makes a transistor. Current from a battery, I, and ground, and light comes out. That's the semiconductor laser. It's made of a semiconductor, gallium arsenide. At the same time, transistors are made by doping, mixing in small concentrations of foreign atoms with the GaAs crystal which modifies its electrical characteristics. Those are transistors. This is electronics, and this is lasers. Call it optics. They put out light. That's state of the art. What I did at Bell Labs when I was asked to look into semiconductor lasers, we made those lasers, followed the recipe of IBM and GE, and within a month or two, we had lasers.
We caught up. But we went a step further. Working at Bell Labs, I realized that the laser would not have worked if the light was not confined, guided, in the crystal. I think we talked about it last time. What confined it was an accident. It wasn't planned, but the act of doping this area with a P, and doping this N with makes a p-n junction. And there's a region here, the transition region, in which the index of refraction in the middle here is bigger than on either side and that is a necessary condition to guide light with dielectric waveguiding is a material, let's say a tube or wire made of whatever material, transparent to light, with an index of refraction larger than the surrounding material. And that gives rise, as you recall, to essentially, light that–this is the big index, and this is the small index.
And a condition for total internal reflection is that a light moves from a high index (where light propagates more slowly) region towards a low index region, which here, is fulfilled on both sides. See a total internal reflection here and here. The light is confined to the high index region, guided, that's not a rigorous way of looking at it, but that's the was a good high school graduate can understand it. That's why if you look in a swimming pool, if you have a light at the bottom of the pool, a bulb, the light doesn't come out. You can see it reflected at the interface of water air. Water has a higher index of refraction for light with air, and therefore, it can be totally internally reflected.
If you had water on both sides, it will zig-zag. This is a demonstration I did at Caltech in a Watson lecture some years back, showing light guided by a water jet, water has a higher index of refraction than air and thus can act as dielectric waveguide. Water is coming from here into a cup. That's a water jet. And I injected the laser beam from here, which enters the water. And here, you see it zig-zag. This is a flowing water jet. And you can see the light zig-zagging inside that jet. That was a tremendous demonstration.
ZIERLER: You said that was not the rigorous way of looking at it. What is the rigorous way of looking at it?
YARIV: The rigorous way is to solve Maxwell's Equations, very simply. Again, it is in the book, the whole page details–a simple example, a sandwich of three layers. The salami is a high-index material.
ZIERLER: The salami's the middle stuff.
YARIV: The middle. It's a sandwich where you have low index on either side and a high index in the middle. And you solve Maxwell's Equation rigorously for that. It would not be very difficult. Wave equation, second-order differential equation, two or three lines. And you find out that a mode of the structure, meaning a propagating field, electromagnetic field configuration, which remains unchanged as it propagates, ideally, ad infinitum, has this form. That's the intensity. Here is a cosine, H(X), this is X, and here's exponential, e to the minus alpha X, e to the plus–plus because X here is negative. A wave outside the salami, the inner layer, X is part of a cosine wave, and it connects you to an exponential, so that essentially, if you go a short distance away, there's no light.
And all the optical energy moves forward. You know pointing power? If you have an electromagnetic wave, at any point of the wave, you can calculate how much power, energy per unit time, flows through a unit area. That's the actual power in watts of the field. Poynting theorem. This wave, electromagnetic, that's a low-order mode. There's also a mode that does like a string of a piano, first harmonic, second, modes. We usually use the lowest-order mode. And this is the rigorous way. Here, you can solve for everything you need. The zig-zag picture is the ray optics taught to opticians and the way you teach it in high schools. We will never use it. But to explain it to a non-scientist who probably may have heard of total internal reflection, the fact that light can be totally reflected, 100%, at the interface between a high-index and a low-index if it comes from the high-index, like in a swimming pool.
When I talk about the high index and the low index, the difference can be very small. The difference between n-sub 2 and n-sub 1 minus N1 over N2 could be 1%. That's enough to guide. In an optical fiber, both the inner core, which is the high index, and the cladding around it, silica, both of them only differ in index of refraction, all glass, all silica, by a few percent. And that's why in page one there at Bell Labs, we observed guiding. The difference in index was very small just because of the doping, I think my main contribution was pointing out the role of waveguiding in SC lasers and that without the guiding wbe no lasers,
ZIERLER: Are you thinking, at least theoretically at this point, about what would become OEICs?
YARIV: Not yet.
ZIERLER: What's the connecting point? Where do you have to go to get there?
YARIV: I come to Caltech. We have described the process. I get two or three students working on semiconductor lasers. I begin to think about it. I meet Carver Mead, and I learn from him that one can make transistors in gallium arsenide.
ZIERLER: He taught you that?
YARIV: Yes, we were talking. He was working on it. "What are you doing?" I discovered, I think it was in talking to Carver, but I don't recall the details, that one could make transistors in gallium arsenide. I told myself, "Look, I am already making lasers in gallium arsenide. Can we make the lasers and transistors on the same GaAs?" But the key, of course, if you have a big gallium arsenide crystal, and they're going to make various devices on a same crystal, laser modulator, transistor, which is supplied the–OK, here is a transmitter as an example. Here's a source of light. That's all gallium arsenide. One crystal. Single crystal. I decide, "Here, I will make a laser." And I know how to make it. Here, I will have a box that modulates a light. We know how to do that. The book has two chapters on it. The electrooptic effect. Gallium arsenide has an electrooptic effect. It belongs to the crystal classes, which can modulate light. And here, a transistor applies the current to the laser and maybe transistor circuitry. They have to be connected. Light has to come from here to here. To the outside world, light. Optics, up to that point, is mostly in propagation through air. Lenses, prisms. But the vision here is that we are doing it in a crystal, and the light is taken from one point to another, from the laser, to the modulator, to other components, and then out to a fiber, as an example. But that's before fibers. Today, by waveguides. Waveguides are the light pipes. And they are done by selective doping. I was working with lasers, I find out you can make transistors on the same material I am already using. Put them together and write a paper suggesting they could be done.
ZIERLER: Let's back up a little bit. Gallium arsenide. It's so important, it's so amazing. Explain a little what gallium arsenide is and why you can do these things with it.
YARIV: Gallium arsenide is a semiconductor.
ZIERLER: Is it an element? Is it a chemical compound? What does it look and feel like?
YARIV: The name, gallium arsenide, means it's a crystal. Salt is sodium chloride. Its arrangement of atoms of sodium and chlorine are perfectly cubic. It's like two intertwined, interlaced cubic crystals, in GaAs it is one of gallium, one of arsenic. Just like in salt
ZIERLER: And these are two elements.
YARIV: Two elements. Salt is sodium chloride. Gallium arsenide is like salt. It has the same basic crystal structure. One is the element gallium. The other is arsenic. The two, when molten, probably, grow as a crystal. Why exactly they choose to form a crystal, not my field. That's well-known by the time.
ZIERLER: But this is naturally occurring? Or it has to be cultured in a lab?
YARIV: In a lab. You melt the–crystal-growing is a different world. But it's done in the lab. I don't think we have gallium arsenide crystals occurring naturally, unlike salt. I think the temperatures needed are probably higher. But people knew how to make gallium arsenide crystals before the lasers. People already made p-n junctions in gallium arsenide, which emitted light when you passed it through them, and fairly efficiently. That was the motivation for GE and IBM to try to make a laser of gallium arsenide. They brute forced it. They shot a current. They were not aware that it took waveguiding in the junction region to make it possible. Nature provided it for them. The word waveguide is never mentioned in any of their work. It was the understanding that you could also make a waveguide in addition to the transistor because the waveguide is the connecting wire that takes light from one location to another. I proposed a new technology, in which we grow optical components, both active and passive. A prism will be a passive component. A laser, where you provide current which you can turn on and off thus modulate the light is an active device. You can make use it to manipulate information and to do calculations. I was at Bell Labs in an area that was concerned with the basic questions of how to do it.
The Wonders of Gallium Arsenide
ZIERLER: You were working with gallium arsenide at Bell Labs already?
YARIV: Oh, remember, when I was asked to make a laser following the publication by IBM and GE, their laser was gallium arsenide. And I'd never heard of gallium arsenide until I was asked by my boss. And it turned out that there were people at Bell Labs who knew how to grow gallium arsenide. I got together with two or three of them, and within weeks, maybe, we had a gallium arsenide very much like the one that GE and IMB made. Remember, I was at Bell Labs, I told you the story, giving a talk in the physics department–trying to understand why it worked, and essentially decided that there had to be a waveguide in the laser. The light bounces back and forth. It doesn't propagate through the volume of the crystal.
It's confined to a narrow stripe straddling the interface between the p and the n. to make a laser, you start with a crystal of gallium arsenide, and you grow a layer doped p type and then n type and in the interface you have a p-n junction. If you apply voltage to it, look at the current as a function of the voltage you get the junction characteristic. Flattish on one side and climbing steeply on the other. Here, you get a lot of current for a little voltage, and here, you get very little. Anyhow, that is a p-n junction. And the IBM, GE people simply applied current to it and got light out. First IBM, GE gallium arsenide laser. I did that at Bell Labs. Wrote a paper on the need for the requirement to have a waveguiding action in a laser. Today, people don't even think about it. But it was a revelation at the time. And provided the experimental evidence for that. I think the stuff I gave you has a reprint of that original paper.
ZIERLER: Gallium arsenide, by the time you get to Caltech, is obviously not new to you. What was Carver Mead doing where you connected with him, and then gallium arsenide was different for you?
YARIV: Gallium arsenide is a semiconductor. Semiconductors come, like germanium silicon, which are a Monel element. A semiconductor is a material that has an energy gap, in which no electron can exist. This means that you can control the conductivity of a semiconductor of with an applied voltage (electric field) or permanently ,by doping n type where the electrons carry the current or p type where the "holes' (missing electrons) carry it.
ZIERLER: No energy is lost?
YARIV: No. An atom could be a hydrogen atom, one electron around a nucleus, and you solve the Schrödinger equation for the allowed energy levels. The energetic states in which that configuration can be found are quantized, meaning the hydrogen atom cannot possess any arbitrary energy that you want to. It has only distinct energy levels in which it can be found and you can get their values by solving the Schrödinger equation, and they are near perfectly with you measure. When the atom drops from a high energy state to one of less energy the difference in energy is carried off as light, if you excite a hydrogen atom to a high level the atom cascades back down to the lowest ground state, it emits photons of light at each of the steps whose energy is that of the difference in energy between the two states. Every basic configuration has allowed energy levels, which are a solution of the Schrödinger equation. In semiconductors, if you solve for the energy level, you find out there's a continuum of level as you go up in energy.
Remember, every line here is an allowed energetic state of the whole crystal. And that's the valance band. Then, the region, the energy gap, in which no allowed states can exist. That's due mathematically to the periodicity of the crystal. And then, another region, the conduction band, which energy states–those are allowed energy states. It doesn't say that they're occupied, but that's where electrons can reside. I'm simplifying things. An ordinary semiconductor will have–those are electrons. In a valance band, up to the edge of the energy levels, occupied are full of electrons which are free to roam throughout the whole volume crystal while retaining their total energy. Each one of the electrons roams throughout the whole crystal. And then, a region which–this is energy. No electron can be found with energies in this region. And then, here, the energy levels are occupied. Two electrons, spin up, spin down. And you cannot put more electrons into here. If you are going to shove more electrons into it, they'll go here.
A fairly pure semiconductor–now, a full band, a valance band where all the levels are occupied, cannot conduct electricity. Here, there are no electrons, so it cannot conduct electricity. A good semiconductor doesn't conduct electricity very well. Now, that situation, where all the levels here are full and all those are empty, only exists at very low temperature. Ordinary temperature, there will be some electrons here. And of the few electrons here, billions will conduct current. Levels here which are not occupied can also conduct electricity. We call them holes. In other words, electron holes are unoccupied energy levels, which can conduct–a fully occupied level like the valance band cannot conduct electricity. In a metal, there's no energy gap. So a metal will conduct electricity–if you take a semiconductor, and you cool it, the few electrons up here, which are excited thermally, at low temperature, will drop field of valance band, and the semiconductor will act like an insulator.
If you increase the temperature, electrons will be excited from here to here, and both the holes and the electrons will conduct electricity. And a p-n junction uses those properties. In a metal, there's no energy gap. A metal can continue to conduct electricity as you cool it down. The only reason that stops conducting, the electron drops down and fills the band here. And a full band doesn't conduct. This band is completely empty. There's nothing to conduct either. Gallium arsenide has those properties the same way that silicon does. But there's a difference between gallium arsenide and silicon. They belong to two different crystal structures. In a gallium arsenide laser, you dump current, which shoves electrons up here, and those electrons then fall down and fill an unoccupied state–so in a p-n junction, electrons dropping down from the conduction band to the valance band emit light.
They are losing energy. Remember, this is energy. And the energy's given as light. In silicon, the process is very, very inefficient, so you don't get much light, if any. Maybe a millionth that you get–in gallium arsenide, almost every electron dumped in the conduction band drops across and gives you light at a higher efficiency, exceeding 90%. In ordinary gallium arsenide, the light given out is random. But if you make a laser, it provides feedback, and shove enough current, the light begins to be amplified as it goes across, back and forth, and it lases.
ZIERLER: Again, to go back to Carver Mead, what was he doing that helped put this all together for you?
YARIV: Carver Mead was playing with transistors when I came here. You will have to talk to Carver Mead.
ZIERLER: I have. I want to hear from your perspective. He was on the faculty at this point, or he was still a graduate student?
YARIV: No, no, he was on the faculty. He may have been even a full professor. Maybe associate.
ZIERLER: And probably, what, 25 or 26 years old?
YARIV: Well, he wasn't full when he was 25 or 26. Probably got his PhD around that time. By the time I came here, maybe an associate professor.
ZIERLER: But he was already a dynamo on campus.
YARIV: Oh, he was a dynamo. He hasn't slowed down, but he was a dynamo then. And clearly, at that he hasn't yet done the work for which he's most famous. He did good work.
ZIERLER: You mean VLSI?
YARIV: VLSI, yes.
ZIERLER: But this is foundational.
YARIV: But people who knew him were impressed, but he was still groping around for his big purpose. We collaborated for a while, wrote a paper together. He was interested in optics. He got back to it more recently after retirement. And among the things I was interested in, when you evaporate a metal on top of a semiconductor, the junction acts like a p-n junction. It's called a metal semiconductor junction. And Carver was very heavy into that as well. And he looked at the other materials. The details, I'm not sure. But I think the knowledge that one could make transistors from gallium arsenide came to me from Carver. I don't know whether he was a pioneer or was just teaching me something known in his field. But that was all I needed, so I started reading.
ZIERLER: Now, let's break down the name like we've done for all the others. We call it OEICs for short, but optoelectronic integrated circuits. Let's start first with optoelectronic. What does that mean? Simply, it's the combination of optics and electronics?
YARIV: That's all. Opto means light. Electronics means electronics, which means, really, transistors and junctions. And later on, anything involving the flow of electrons. No light involved. That's electronics.
ZIERLER: And then, how well-developed by itself is the field of integrated circuits by 1964, '65?
YARIV: Very, very developed. They have not yet achieved the technical control, which enables them to pack 10 billion transistors today into an iPhone. But the basic notions, the CMOS transistors, the ability to pack large numbers of transistors and make integrated circuits, was well-known. I think intellectually, there are no new ideas, basically, since then in terms of physics and how it works. Just the ability to make them smaller and more efficient. You know about the competition now between Intel and TSMC?
ZIERLER: Yes. Intellectually, you're now able to merge these fields.
YARIV: Yes.
ZIERLER: Technologically, what does it look like to go about building these OEICs?
YARIV: Well, do you have the material I gave you?
ZIERLER: I have it pulled up.
YARIV: You'll see the first two or three integrated circuits. You are looking here at the world's first integrated optoelectronic circuit.
ZIERLER: In your own voice, let's walk through it.
YARIV: Take this one as an example. The whole thing you see is a single crystal. Meaning all the atoms are arranged in a perfect order. And it's gallium arsenide. And over here, you have a laser exactly like this one. This was one we started at Caltech, and we finished, working with Hitachi. Here, on a single crystal, on the left side, you have a laser. And here, you have a transistor. I think the kind that Carver Mead was playing with. And they're made by diffusion, and etching, and so on, using photolithographic techniques. And in practice, this is the beginning of a transmitter. An electronic signal, current, carrying information, music, computer data, etc., is fed into the transistor. The transistor amplifies the current and feeds it into the laser which then emits aa laser beam whose intensity mimics the current thus carrying piggy back the information as light modulation. ready to enter an optical fiber and carries the information to, say, New York City
It's all done by the same CMOS techniques used to make transistors, etching and doping no wires and in many orders of magnitudes less volume.
ZIERLER: Now, did you pull your original graduate students off semiconductor lasers to start on this? Or this is a new crop of graduate students who work on OEICs? I'm thinking of David Hall, for example.
YARIV: No, David Hall essentially demonstrated the first active component on a laser, which was a simple modulator and the all-important guiding.
ZIERLER: What do you mean, active component?
YARIV: Yes, it was a modulator. You can turn the light of the laser on and off by modulating the equal current.
ZIERLER: Is this chirping or something else?
YARIV: No, chirping comes later. In the David Hall experiment, essentially, you have a laser here–I take it back. This simply demonstrates that you can use gallium arsenide as a modulator. This is figure three of the beginning of integrated optoelectronic circuits. And it demonstrates that gallium arsenide–you already know there's a waveguide and a p-n junction in gallium arsenide. There's p-n junction here, the doping. And that waveguide simply guides light think of it as a pipe. And we can essentially modulate the waveguide, making it conduct light or stop it by modulating the electric in the volume of the crystal in which the light propagates. This is called the electro-optic effect. The point is, we can make an electrooptic modulator, which is known how to make an electrooptic modulator in materials with certain symmetry properties. Gallium arsenide has those properties.
And here, we show how it's done. And once you can make a modulator here, we can now make exactly what we do here in the integrated circuit. We can add the modulator, which is a key component. That's how you put information on a laser beam. We know how to put a transistor there. We can take the current out with the transistor and apply it to a different region on the same crystal being modulated with modulator light coming from a laser on the same circuit. We show here you can begin to make and interconnect both electronically so current can flow from one place to another and also optically by waveguiding. And that basically illustrates the feasibility of this new technology. Once we could connect, and turn on and off, and interrupt–it's really a marriage of electronics and optics.
ZIERLER: How long until proof of concept? Just the timeline. You get to Caltech in 1964. How long does it take before you're demonstrating viability?
YARIV: Well, remember, I mentioned to you that part of our team at Bell Labs made the laser in a few weeks. Walter Bond was part of the team that helped Shockley and Bardeen to make that transistor. One of the best technologists I've ever seen. My first proposal, active integrated optics, the idea that it can be done, not demonstration, is published in the Fundamental and Application Laser Physics, proceedings of the Isfahan 1971 symposium. I have a copy here. The first theoretical proposal is in 1971. I came to Caltech in 1964, so about seven years later.
ZIERLER: Seven years is demonstration of feasibility?
YARIV: Yes, and a proposal.
ZIERLER: So this is really a long gestation period.
YARIV: This is the actual publication. The idea, probably, was maybe a year before that. But this is the first publication. Actually, I gave a talk earlier in Japan, maybe a year or two earlier. But there was a conference in Iran, Persia still, the Shah was there, and I wanted to go. I thought I'd talk about it. When I first came up with the idea that you could integrate the two, it wasn't a eureka. I wasn't struck by lightning. I didn't realize how important it was. I knew it was interesting, and the notion you could combine two different fields together at random on a crystal–but gradually, later, I realized it was an important idea.
ZIERLER: If 1971 is the demonstration…
YARIV: No, that's the proposal. The demonstration–let's go down here. The demonstration is later. This is '73, and the demonstration is 1978. 1978, and maybe a year or two. It wasn't easy to acquire all this working with students.
ZIERLER: At what point are the majority of your graduate students working on OEICs? How long does that take? Does that roughly accord with proposal in 1971, so it's the 1970s?
YARIV: Actually, a majority of my graduate students never worked on OEICs. There was an intense period there, which we have slotted a few years, which we demonstrated basically, I think, all the basic ideas to convince us, and later, the world, that indeed, there were here the basics for new technology. integrated optoelectronic circuits. Whether it would be competitive or not–if you combined electronics and optics–everybody, of course, understood that electronics were very important. But I think the understanding that optics would play almost an equal role in importance as electronics time did not exist as yet. The importance wasn't immediately obvious to us.
ZIERLER: In the way you set up your research agenda, intellectually, administratively, is semiconductor lasers always in its own lane? Or at some point does it merge into the OEICs work?
YARIV: No, semiconductor is the main river. And I had students working on semiconductor lasers almost from day one. I still have a post-doc across the wall here…That main river kept flowing. The proposal and demonstration of an integrated optoelectronic technology was a big detour that lasted a few years. We knew it was important, but not how important. And then, we returned to the main river. We basically realized that the next step wasn't up to us. We'd shown it could be done. To make it practical, you have to show you can make them reliably, efficiently, in big numbers, yield, and it took maybe another 20, 30 years for that to happen in industry. I think we smartly realized that we probably would get the credit for proposing it, and the rest is an industrial issue. We were not in the best position to do it. We withdrew from it. We stopped working on the integration. There were other basic problems that seemed more interesting. Integration is essentially photolithography. That's what TSMC's doing now and Intel less well..
ZIERLER: If semiconductor lasers is the main river, and let's say, from 1964 all the way until viability of OEICs in 1978, at what point does the field of semiconductor lasers reach maturity?
YARIV: Well, you recall that the distributed feedback semiconductor laser, which is probably to the world our main claim to fame–this should be, actually, and it will be one day–at that point had not happened yet. That was later. The history of the Semi Cond, Distributed Feedback laser. The first publication, Yen, Nakamura, Garmire, Somekh, Yariv, and Garvin optically pumped gallium arsenide waveguided lasers with a fundamental 0.11 micron corrugation feedback. A laser with a wavy interface. We call it corrugated. That's the first demonstration of a distributed feedback laser in gallium arsenide.
ZIERLER: And what year is that?
YARIV: The year is September 1973.
ZIERLER: With the demonstration…
YARIV: That started the race worldwide to make such lasers. Those are the lasers that emitted a single frequency in response to the need by industry for long-distance optical communication, which was a necessity.
ZIERLER: So this was an industrial race, It should have been industrial but we had, as it turned out the winning solution, so we demonstrated it and then left it to industry to develop a competing solution from Bell labs turned out to be non-competitive and was abandoned by them quietly.
YARIV: What industry did was put out the word, "We need a laser that emits a single tone or frequency."
Remaking the Cable Industry
ZIERLER: And up to that point, they were all multifrequency.
YARIV: Multifrequency. And that was OK for reasons I can spell out the physics of. Initially, when lasers entered service supplying the light source for optical fiber communications systems, the data rate was very small.
ZIERLER: This is the cable story we talked about previously.
YARIV: Yes. The data rate was small, and it was not a problem if the light consisted of multi-wavelength. Later on, the data rate increased, appetite for optical communication for bandwidth. That became the main obstacle for further increase of bandwidth, data rate. The multi-tone, multi-wavelength. And the reason is simple. Here's the laser. Gallium arsenide. And here's the current. And the current is made up, let's say, of ones and zeroes. That's the electronic data that you want to transmit as an optical wave. You need to replicate the electric current temporal variation with the variation of the intensity of the emitted light. Ideally, you would like the light intensity to be a bunch of ones and zeroes. Because the laser emits more than one wavelength each one of those light pulses consists of three or four wavelengths. Spectrally, this is the current, this is time. This is light, this is light intensity. The laser ideally emits light pulses, which are a replica of the electronic current feeding it. This is the information, the music, Beethoven's 9th Symphony, and here, it's transformed into light pulses, which replicate it exactly.
If you look at the spectrum, of one of those pulses, it will consist of lambda one, two, three, four, five, six. This is a case where there are six wavelengths present. Here, I'm showing the time profile of the intensity. If you take it into a spectrometer, you'll see that. Each one of those propagates with its own group velocity. The result is that this pulse will broaden. Because remember, each one of those has a different velocity, and therefore, the pulse will become wider as it propagates. Because they will not stay in the same location in the fiber. And eventually, the pulse becomes wider and wider, and the point where this pulse and this pulse begin to overlap, you are beginning to lose the identity of the ones and zeroes, and therefore, the information. That's the limiting factor. If you have a single wavelength, it doesn't happen. The call comes out from the engineers for a single-frequency laser, but of course, those are the good electrical engineers at Western Electric.
They don't know how to do it, but they know what they need. They understand pulse spreading. That's basic electronics. The solution for making that one came from us, the idea that a periodic interface will do it, was due to a basic understanding of light propagation. And people here were getting PhDs, post-docs from Japan just on studying how light propagates in a periodic structure. The idea that we can take that knowledge and apply it to solving that unique problem, then, was applying a basic theoretical understanding to a need of industry, the connection, that was mine. And that's because I'd developed, at just about the same time–during a summer in Israel, I published a paper I considered one of the more important. Coupled wave theory for guided wave optics. It showed how you can reflect light, which is what you do in a laser, and modulate it, transform it, by the theory that connects the exchange of energy between different light modalities in crystals. Which was the key here.
ZIERLER: Did you work with your fellow Amnon on this?
YARIV: I think that Amnon is about 30 years younger than I am.
ZIERLER: He would've been too young then.
YARIV: He may have been barely born. [Laugh] And he was a professor of computer science. Going back, we were working on light propagation in periodic structures, structures which repeat themselves. And the reason I did it was because, as I said, my traveling-wave tube in Berkeley, my master's thesis–a traveling-wave tube is a helix, an electron beam through it, and the theory–a helix is periodic. As you move through the center of a helix, you look around you, it repeats itself. It's a periodic structure. And there's something very basic. What distinguishes a metal or an alloy from a crystal in a unique property that makes crystals important is that the crystals are periodic. And all of a sudden, I realized that a periodic interface–all of the laser didn't have to be periodic. I could introduce artificial periodicity, which I could control, that's the waviness or the corrugation, in one interface.
And that acts as if the whole crystal was periodic. That's in the mathematics. Suppose you built a multilayered, one, two, one, two, one, two. Could be glass. A multilayered sandwich, many, many, many layers. And light comes here. This light can be almost totally reflected. By adding more layers, you can make it approach 100% reflection. Light incident, light reflected, and you can reflect almost 100% by adding layers. This is essentially the Bragg reflection. And if you come at the right angle, the extra is reflected. It's reflected from here, from all the layers, and when the Bragg condition is satisfied, if this angle is theta, then 2D sine theta is equal to some integer, one, two, three, that was the order of the wavelength, that's a Bragg condition. X-rays reflected. In optics, you can make a multilayer, and they are used, actually, as mirrors.
And what I realized was, if I made the laser and had a periodic interface in gallium arsenide, the first experiment, the laser prefers to emit light of only one wavelength, the one where the wavelength is equal to twice the corrugation period. The distributed feedback laser's essentially a gallium arsenide laser with a periodic interface, like a washboard. Although it's limited, the corrugation or waviness, to a very narrow region, it reflects the whole way. Why? intuition cannot tell you. But it dropped out of my mathematics. Actually, out of perturbation theory and eigen modes in quantum mechanics
And the paper was, I think, coupled mode theory and its reference. And I think if I haven't given you a copy, I can. "Coupled mode theory for guided wave optics". The distributed feedback laser's just a laser with–and that's a laser that oscillated at a single wavelength, which met the requirements of the Bell system for a single wavelength, and made it possible to increase the bandwidth. It's still the main source of light, the only source for the whole optical fiber communication.
ZIERLER: To this day, you mean.
YARIV: To this day. Difficult to see anything–nothing can be simpler than that. Same structure, very simple. And this waviness–we also incidentally proposed how to do it. I told you the story. No idea how to do it. But we basically learned how to do photolithography from an electronic company on Foothill Boulevard near the Panda Express in Pasadena. [Laugh] They showed us how to use photo resist, etc. How do you make a periodic interface? It's a DFB laser, distributed feedback. People refer to it as a DFB laser. Suppose you want to make the surface periodic. Step one, coat a gallium arsenide crystal with photoresist layer with properties that are changed chemically when light is shone on it. And you can get two versions. If you have a photoresist layer, and you shiny a light on part of it, and you then put it in acid, the area which exposed to light will be etched out. That's the basis of photolithography. The light changes the atomic bonding so that the subsequent immersion in an acid will eat it out. By projecting a pattern on the photoresist, then etching it, you can replicate it as a surface relief. You can make channels or whatever you want. That's the basis of photolithography. That's how you pack five billion transistors. Into an iPhone. You make the pattern, then you project it on a photoresist, on silicon. You demagnify it, of course.
You have to pack five billion of them. A lot of good engineering there. And then, you submerge it in acid, then you diffuse to make P and N and all of that. That takes maybe a few weeks, as the crystals, the silicon wafers, are moving slowly. At Intel or TSMC, moving in a vacuum from one station to another. Each one of those operations. And eventually, come up with those almost defect free. It's a miracle of technology. But at the front edge, TSMC and ASML are the leaders.
ZIERLER: What exactly were the techniques you had to learn at this little shop at the Foothill Boulevard? What did they teach you?
YARIV: Everything here. The stuff they taught us was very basic, A, B, C for people in semiconductor manufacturing. But we didn't need very much. You take the crystal–everything I'm talking now is something they taught us. Here's a polished crystal. Spin photoresist onto it. And then, next step, we take this crystal, and we shine a laser beam coming from lasers divided in two. Lambda is a wavelength. It's a single source of laser divided with mirrors, so you wind up with two beams more or less propagating opposite to each other forming a standing wave patten on top of the photoresist. You can control this angle between them. What you can control is this angle, theta, with simple mirrors. And of course, the wavelength of the laser. That's a UV laser. The result is that the light intensity–you know what standing waves are? If you have two beams of the same frequency, coherent, "colliding" with each other, the intensity of the light is going to be periodic like a sine wave, but stationary in time, non-varying, along a direction of propagation. This patter is called "standing waves."
ZIERLER: At the moment they collide?
YARIV: By colliding, those are continuous waves. If you take a single wave and measure its intensity along the direction of propagation here, you'll get a constant. A single wave propagating has a constant intensity. Intensity means power per square area. As long as the wave is on, if you measure how much energy moves through a unit area, that's the, it's a number. That's how you measure how powerful the wave is. If you take the same wave light here, divide it, and shine it from two directions, then the intensity of the pattern, instead of being a constant, will be a sine squared, periodic standing wave. This, we did not learn from those people there because they probably didn't understand that. We understood that. But they taught us how to do the spin, what photoresist that we needed.
And what we did with it, then, was do that, and the result here, that we now illuminate, expose the photoresist with this intensity. Intensity. We called it standing wave. This wave doesn't propagate. It stands there. Mathematics. This is a traveling wave, this is a standing wave. Basic optics. And the distance here, call it period, if this is lambda, is the wavelength over 2 the sine theta. Theta is not this angle, but this angle. The wavelength is fixed, comes from a laser, lambda. We can change theta, and therefore, the period here, distance between two adjacent intensity peaks [which] depends on theta. Theta is 90 degrees, meaning the two waves propagate opposite to each other; you get half a wavelength. You can expose the photoresist with a repeating pattern of intensity, and it can be as small, the repetition distance, half a wavelength of the light. We can make very, very small–after exposure, we immerse in acid, which washes away the exposed high-intensity region, and we wind up with–that's the pattern of exposure, and the area which received lots of light are etched preferentially.
And you wind up, after watching the wave, periodic structure. And this is P. We do that to the laser, and then take it back and continue to grow the necessary layers. Remember, the lasers are multi-structure layer. And the result is that now, gallium arsenide, p-n, so you have a junction. Remember, light now propagates–that laser doesn't require a mirror because the feedback is done by–remember, this is the light profile. And light is reflected. And at the right wavelength, all those reflections add up in phase. Light, which normally would've gone straight through that light pipe, the waveguide, now is reflected partially at the interface, and at the right wavelength, all of them add up together. The result is that you can trap light in, and you don't need mirrors. And that's the DFB laser. This should help you understand when you read. All of that is the material I gave you. And that's the laser, which is still that today. Variations, improvements.
ZIERLER: Last topic for today, to go back to September 1973, you talk about the launch of this worldwide industrial race. Who were the players? Who was doing the race?
YARIV: Oh, the race to make a laser that…
ZIERLER: My question is, who were the participants in the race?
YARIV: Maybe I should go back. I probably exaggerated. It was not a worldwide race. The story was that before 1973, Bell Labs was making lasers for their own increasing needs of lasers for optical communication.
ZIERLER: For AT&T's own communication systems.
YARIV: Yeah, AT&T was the sole supplier until 1984 or so, when Judge Greene–GTE had 5%, including Santa Monica, and 95% was the Bell system. And they were making lasers for their own needs. Lasers were gallium arsenide lasers emitting many wavelengths, five, six, seven. And at some point, that became the limiting factor on the data rate they could supply in the new optical fiber communications. And they understood that the reason for that was the multi-wavelength. In the book, there's a title of group velocity, dispersion, all of that, shows why that happened. They said, "We need lasers of a single wavelength."
ZIERLER: Bell said this, AT&T?
YARIV: The Western Electric engineers. And then, of course, that request went to Bell Labs. And the Bell Labs bosses told the physicists there, "Why can't you make a perfect laser?" That means a single wavelength. They didn't understand that a laser doesn't like to oscillate at a single frequency. If you have gain that can amplify different wavelengths and feedback, they will oscillate. So you have to actually choose conditions that only favor one wavelength. And they came up with a solution, I told you this story, which consisted of complicated electronics, lenses, mirrors, detectors, which could detect when their laser oscillated on more than one wavelength and then apply currents. It was a complicated laser of many parts. Without going into details, the solution required a trunk full of electronics and optics. And that laser emitted a single wavelength.
And they announced in a meeting, I think, of the American Physical Society, a press conferences, thousands of people in industry and science reporters, that they'd solved the problem. My reaction, I saw it was that it was a kluge. [Laugh] Anyhow, that's really true. I looked at it with a bunch of students, and we were laughing. It took a while when we realized that we really–as I said, we were already working. A few students obtained PhDs unrelated to this story before simply trying to understand better how light propagates in periodic structures. But what we visualized the periodic structure is some crystal where the whole crystal is periodic. Wherever you go, the fact that you can only make a layer of a waveguide periodic and still reflect a wave, that was unique to us.
Because that dropped out of my theoretical paper, analysis of coupled mode theory. The fact that you are working with modes, not plain waves, which are localized. And the theory says that by making part of the structure periodic, if that structure can hold a wave, waveguide it to the region near the periodic structure or encompassing it, that total mode will be reflected, although you are only physically affecting part of it. A mode is an integral animal in mathematics. We realized that as a result of our involvement theoretically with periodic structures for years before that unrelated to the AT&T need contained the solution, so we made one. And I referred a little earlier to the first demonstration. We simply took a gallium arsenide laser, and we learned how to apply photoresist to interrupt the growth process.
The standing wave illumination, photoresist the etching, and then going back and continuing the growth. The semiconductor people, the experts told us it would not work because they told us that, "You'll make your periodic interface, but when you put it back to grow"–we used liquid-phase epitaxy–"you bring back the surface in contact with molted solution of gallium and arsenide. It will melt back and smooth the corrugation." Well, it didn't happen. [Laugh]
ZIERLER: What was the theory that suggested that it would?
YARIV: Well, take any structured surface. Take salt and carve with a knife a little structure. Put it in warm water, in five minutes, it's gone. Because the areas which stick out get attacked by the water more intensely, and eventually you get a smooth surface.
ZIERLER: So why didn't this happen here? It seems like it would.
YARIV: Basically, what we were growing, when you put it back in contact with the hot solution, we didn't let it be there too long. It turned out, and we never planned on it, that the time it took to grow the necessary next layer, which was smooth, wasn't long enough to melt, so we lost maybe 10, 20% of the height of the wave. We could plan on it. After we grew it and had a corrugated periodic interface, and we put it back to grow the next layers, which we needed, we only needed maybe half a minute or so to grow the next layer. And half a minute wasn't enough to melt it. To melt something takes time. We were lucky.
ZIERLER: So these naysayers figured you were going to leave it in for longer when they said, "It's going to dissolve"?
YARIV: Yeah. And we believe they were right, but I tell my students in classes, "Much of what you see in books is wrong. Any book. And the trouble is that you don't know what is wrong. Is it Gödel's theorem or something like that? There are theorems which cannot be proved, but the trouble is, you don't know which one are and which one are not they are, and that drives you crazy." I said, "Let's try it." And then, the expert, the metallurgists could explain to us why it happened. So we did. That's how the DFB lasers–the results were published, and that was the death knell for the Bell Labs solution. It died within a week after that. They never held another press conference to announce the death of their great idea. [Laugh]
ZIERLER: They let that go out.
YARIV: We had a post-doc here from Japan at the time, Nakamura, who went back to Japan. He came from Hitachi. And he convinced management to make a product of that laser. They were the first one. And we collaborated with them. The picture here of–this is the commercial laser sold by Hitachi. And AT&T bought the lasers from Hitachi. They requested that the boxes not say Hitachi on them. Because the Bell system, the world's largest communication provider, was buying the key component from a Japanese company.
ZIERLER: And the Japanese electronics industry is not well-respected at this point.
YARIV: Respected. Hitachi, Toshiba, I think they were respected. Their optics and electronics were respected. They were already beginning to dominate. That's before the Koreans took it away from them. The idea was not that the Japanese were not respected, it was that Bell could not do it themselves, a key component. And they were the best communication company in the world. For a few years, they bought it from Hitachi. And then, one of my students, who is a member of the initial team, goes to work with them and teaches them how to make them.
ZIERLER: Not Nakamura, someone else?
YARIV: Nakamura went back to Japan. One of my students, Somekh, Israeli, got a PhD in the group. A good student. He was recruited by Bell Labs and taught them how to make gratings on the laser.
ZIERLER: Even if it was not a worldwide race, I still want to know, who were the participants? Was it Hitachi and Bell? The industrial people or companies involved in this race from the demonstration in 1973.
YARIV: The race was to demonstrate it, to find a solution. We found the solution at Caltech. There was no race. After that, essentially, if you fast-forward a few years, every major optical company in the world and a lot of smaller companies are making DFB lasers.
ZIERLER: And chronologically, this transition where there's widespread application in industry, this is the late 1970s by this point?
YARIV: Yes.
ZIERLER: That's a perfect place to stop. We'll pick up next time in the 1980s and large-scale industrial applications. Good.
[End of Recording]
ZIERLER: OK, this is David Zierler, Director of the Caltech Heritage Project. It's Tuesday, November 23, 2021. Once again, it's my great pleasure to be back with Professor Amnon Yariv. Amnon, now I know why last time, you responded, "Igualmente," because you speak Spanish. We talked a little bit before I hit record about your love of languages. The first question there, obviously, would be, has your mastery or familiarity with other languages, besides Hebrew and English, ever been advantageous for your science?
YARIV: In Berkeley, it made it easier for me to fulfill the foreign language requirement, which is part of the PhD requirement. And I took it in French. Maybe a minute or two on how I learned French. War of Independence in Israel, 1948, the initial fighting, two or three months into it.
Recollections on the Founding of Israel
ZIERLER: And you're 18, you're in the Tzahal [Irsaeli Army] at this point.
YARIV: Yes, 18 in a few months. Over, and we have a ceasefire. During the ceasefire, there's a large influx of young Jews from all over to join the Israeli Army. North-African Jews, European from displaced person camps. And one day, I was an artillery in the first Israeli artillery school. My commanding officer in charge comes over and says, "Amnon, we have a big influx of Moroccan young men arriving tonight, and we have to turn them from a mob to Israeli soldiers in two or three weeks." The result was that that evening, I had a barrack with 100 young unruly Jewish kids from Morocco between 18 and 22. They were pushed out by the Arabs, and they spoke no Hebrew.
They only spoke bad French and the local Arabic dialect. I knew a little Arabic, but not enough, and I was in charge of them. Had a long barrack with 100 cots and my little room at the end where. They really were not well-disciplined. [Laugh] I needed to be able to talk to them. To cut a long story short, the first occasion I had to go back home to Tel Aviv, which wasn't far, I went to a used bookstore on Allenby Street, the main street in Tel Aviv, and found a Scottish text for teaching French in Scottish high schools. It was a three-year course. I finished it roughly in two or three weeks. [Laugh] It was important.
ZIERLER: What language was primarily spoken among Israeli soldiers in '48? Was it Hebrew or Yiddish?
YARIV: Oh, absolutely, Hebrew. I would say we spoke Hebrew the way that an American would speak English. It seemed to us that the whole world spoke Hebrew. We hadn't met many people who did not. And that language was already good enough to do everything. And there only 600,000 of us.
ZIERLER: And that's your first language, Hebrew.
YARIV: Yes.
ZIERLER: Your parents spoke Yiddish to each other?
YARIV: Yes, at first. But within a few years, they switched over to, in my presence, only Hebrew. And occasionally, Polish, when they didn't want me to understand what they were saying. I never learned Polish, really.
ZIERLER: But they pushed to speak Hebrew as Zionists, to teach you Hebrew?
YARIV: Yes. And Yiddish was the only language spoken by my grandparents, so I heard a lot of Yiddish and some German. I had a couple of uncles who came from Germany. Ich sprechen some Deutsche. [Laugh]
ZIERLER: Were there Israeli Arabs who were loyal to the Jewish forces, to the Tzahal, in 1948?
YARIV: No.
ZIERLER: No such thing?
YARIV: I mean, some may have existed, but there was no organized framework.
ZIERLER: There were no Israeli Arabs you could have turned to for their Arabic language abilities to help speak to the Moroccan Jews?
YARIV: No, it was a war, and even many of the non-belligerent Arabs left what today is Israel, for reasons which are mixed. You probably know the story. My father was a painting contractor, small scale, and had a few Arabs working for him before the trouble started in the late 30s and early 40s. Occasionally, I spoke with them. English was mandatory in school from the age of 10, so by the time I graduated from elementary school, I had four years of English. Intense. They did a good job. I could read. Ellery Queen, Earl Stanley Gardner, mostly what I read. And in high school, after the second year, we had to add another foreign language. And the choice was French, Latin, or Arabic, and I chose Arabic, since I planned on staying in Israel, Arabic would be useful. And I enjoyed it, too. I always liked languages.
ZIERLER: When you were serving as an 18-year-old, did you already have scientific inclinations that were relevant in the War? Were you doing technical work?
YARIV: I mentioned that I was in the artillery. And the reason I was in the artillery, the high school I went to, we had to choose one of three directions, and that's the only choice we ever had. One was business, one was humanistic, and one was scientific. I chose scientific. I took calculus for about two years out of the four, and chemistry, physics, a lot of it. Geometry. Matter of fact, when I arrived at Berkeley, I was told that I could skip the whole first year. Our high school was on the roster among a few other high schools throughout the world, and they considered the scientific background we had equivalent to the first year in Berkeley. I did not take advantage of it, so I repeated the first year because I had a war in between, and I thought it would be a good idea to repeat my math and physics.
ZIERLER: Were there any religious soldiers in the Tzahal in 1948?
YARIV: The Hebrew word for it is Tsahal. It's spelled in English with a Z, but a better choice would've been T-S. English has no tsa. It's Ts'va Haganah l'YIisrael. But yes, there were. Not orthodox Jews, ultra-orthodox. Observant, today by our term.
ZIERLER: But minyan, tallit and tefillin in the morning?
YARIV: Eh, maybe one or two. But they were not segregated or put in special groups as they would be today.
ZIERLER: No payot, no haredim [Sidecurls; religious Jews]?
YARIV: No, no payot.
ZIERLER: Where were you in 1948? Where did you see the War?
YARIV: A number of places. The British left Israel, I think, if I'm not mistaken, the 25th of May in 1948, and the War started at midnight with about five or six surrounding Arab countries. I was in the artillery unit. We had trained for a month or so before that while the British were still around us, looking for us. And our weapons were [mostly], 50-millimeter. Essentially, a heavy machine gun, or a light–actually, gun is a better word for that. It required five people to get it going. And we used anti-tank, anti-aircraft, anti-ship, whatever they needed. We were sent immediately to Gush Etzion, an enclave four or five kibbutzim about ten miles southeast of Jerusalem. Embedded completely in a pure Arab countryside farming villages.
Bethlehem is another ten miles, which is probably the most fanatic, even today. When the War started, that whole enclave was immediately surrounded by Arabs, villagers. It was clear that that place would fall to the Arabs. There were only maybe a few hundred men in the group, surrounded by tens of thousands of Arabs. With the help of the Arab Legion, which was officered by English officers, if you read the history, and our job was to make it to Jerusalem, which was not easy because the road to Jerusalem was and besieged, essentially, and then break out of Jerusalem and reinforce Gush Etzion. Gush in Hebrew is an enclave. We never made it. We were met at the entrance to the valley outside of Jerusalem. And that had Arab villagers, steep banks on both sides, and even before the War of Independence, Jerusalem was besieged. Our job was to enter that valley to Jerusalem and reinforce Gush Etzion.
At the entrance, we were met by English armored cars with two-pounder guns and essentially blasted us, didn't allow us to get in. A few of my friends were killed in that encounter. So the British physically helped the Arabs in the War of Independence. We never made it there. That night, the Arabs overran Gush Etzion, and we saw the flames. We were maybe 30 miles away as the crow flies, where we spent the night, and we could see the burning. That's where I was at first. After that, I was in a number of places. They put us on a truck, and that made us an armored unit. [Laugh] Then, they put us on an ex-American icebreaker, and that was the first Israeli warship. Needed a gun -just call. [Laugh] For a while, we actually even guarded what today is the Weizmann Institute. It had a different name then.
Machon Ziv Institute, Z-I-V, and it was the nucleus of the Weizmann Institute. I guess it was the sum total of Israel's scientific ability, that group of buildings. And we were guarding them from Arab bombardment. But now, we were fighting, and the Egyptians were bombing Tel Aviv using English planes, American planes. The Israeli pilots who were fighting the Egyptian bombers flew Messerschmitts. [Laugh] German.
ZIERLER: How did they get them?
YARIV: The story started, a bunch of American Jews who decided they needed to help Israel, whether they were Zionists or not. Some of them were ex-pilots or served in the US fair Force during World War II, and the planes were brought to Glendaleorf Burbank. Ex-Lockheed. And they bought out-of-commission flying fortresses, loaded them here with ammunition, rifles, machine guns, and then flew them over to New York, and then to Prague, Czechoslovakia. During the War, the famous machine group in Germany, and maybe GM here, were making Messerschmitts for the Germans, who were under occupation in Czechoslovakia. Czechs were good at making machines. And so, they loaned a number of Messerschmitts and other stuff in Prague and then flew to Israel.
ZIERLER: Wow. Where were your guns made? Did Israel have any native armament industry?
YARIV: Zero. Oh, they could make bullets. The guns were named Hispano-Suiza. I don't know if you're interested in antique cars. If you look at the first cars that are probably worth a million dollars apiece, among them, the old Mercedes-Benz, like the one that Hitler rode around in, convertibles, and Hispano-Suizas. Beautiful cars made in Spain and Switzerland. They also made armament, mostly armament. After the fighting during the ceasefire, and after that, too, I was an instructor in the artillery school. The long answer to your question: why were we in the artillery? Because we went to scientific high school. And we knew trig. And they figured that would be important. Probably wasn't so important to zero in on a target, figure out the inclination. [Laugh] Some of that. But we knew numbers. We could add and subtract. That's why almost my whole class was put into artillery.
ZIERLER: How close were you to getting killed? Were you ever in real danger in '48?
YARIV: Yes. Yes. That takes us back to the Spitfires. Guarding the Weizmann Institute–the Weizmann Institute was a group of buildings, maybe a few-hundred people at that time. Today, it's a beautiful park-like setting. And we were outside a few hundred yards in a field, just one gun. A crew of five altogether. I was in charge of the group. I was a sergeant. I became a sergeant later on. At that point, there were no ranks. None. After we won the War, we were given ranks. But I was in charge of the five. One day, an Egyptian Spitfire dove at the Institute, probably to drop a few bombs, but he noticed us, so he kind of changed course and dove at the five of us. And the way they do it, there's this empty field, and he's diving at us. He cannot aim at us directly, so he begins to strafe before he gets to us. And we're in an open sandy field. You can see the sand jumping when the bullets hit the sand, and it moves forward. What I see is the plane on top and two lines of jumping sand advancing towards me. You know when it gets to you, it's over. I was shooting at him.
ZIERLER: With what? You had anti-aircraft guns?
YARIV: Our gun was also an anti-aircraft gun. You could put it down on a base. There was procedure for doing it, and you'll see the routine in my iPhone. Had he continued that, he would've killed us all probably. But as he was doing it, he was losing altitude because he was coming down. And he was too low, so he took off. And I got him from behind as he was rising again, probably getting ready for another run at us, in which he would've done a better job. And he crashed in that huge, open field. But there were two of us. There was a heavy machine gun on a building maybe a quarter-mile away shooting at him, we learned later. And there was us, so we split the credit. [Laugh]
ZIERLER: You shot him out of the sky?
YARIV: Yes. It was a Spitfire, a British plane. English. And other occasions, we came close. But that was the closest.
ZIERLER: Where were you when the ceasefire was announced?
YARIV: I was south of that, near the Egyptian border. There's an Arab town called Yavne, a large Israeli town today. Maybe 20 miles north of the border. And the Egyptians who were advancing towards Yavne. And the Israeli Army essentially captured it. And with an officer, myself and an officer alone in that area, where we didn't know where the Egyptians were who were advancing. The officer and I drove into deserted Yavne. The population had left. The eerie feeling of this town, which was alive a day earlier, and was now completely abandoned. And I was there when we heard over his radio there was a ceasefire.
ZIERLER: What did that feel like?
YARIV: I forgot. [Laugh] The thing is, we were kids out of high school. And I remember a little later, we put our gun and another gun on Israel's first warship, which was an American icebreaker called the Northern or Northerner. And we actually had an encounter with an Egyptian warship, probably a frigate or light destroyer. And they were shooting at us with their heavy guns, and we were shooting back with our essentially heavy machine gun, the Hispano-Suiza. We probably didn't even reach them. One of the shells hit our deck, and a friend of mine was injured gravely but was saved by an American surgeon who volunteered. He survived.
ZIERLER: How did it feel?
YARIV: Oh, that's a story. We almost lost our lives a number of times, but while the fighting was still on, they founded the Israeli artillery military school, and I was asked–I had good grades–to be among the first instructors. I didn't like it. I didn't want it. But I had no choice. But I remember sitting there first week, second week, every night, writing a letter asking to rejoin my group. It wasn't patriotism. That was maybe taken for granted. I wanted to be with my friends, my buddies. [Laugh] And eventually, they granted my request. I was put back on a ship. We didn't feel for a moment that we would lose that war.
ZIERLER: Because Israel always had the advantage?
YARIV: No, it was irrational. If you look at the numbers, what advantage did we have? The British weren't helping us. As you can tell, they were doing the opposite. They made up all they could, searching and expropriating every piece of munitions or arms before they left to make sure we would not be able to defend ourselves. The theory is that they were hoping that we would ask them to come back and save us, and then they would stay long term. They were actually physically fighting alongside the Arab Legion around Jerusalem against us, so they were doing anything but. We were cocky, I guess. First generation. Converting our names from Polish names to Hebrew. [Laugh] I guess it must've been a big thing. When I came to Berkeley, which was maybe a year later, I was in great demand by Jewish synagogues all around the Bay Area. There was a veteran of the Israeli War for Independence who could speak English. And I could've made a living on giving talks to Jewish synagogues. I never did it. I didn't like it. I should've. Serving the cause.
ZIERLER: I'm not sure how spiritually oriented you were as an 18-year-old, but given that you never felt that Israel was in danger of losing the war, even though the odds were overwhelmingly against, were there any nissim [miracles]? Did you feel yad Hashem [the hand of God]? Any of those things?
YARIV: No, no, I was not religious. Not at all, ever. We knew the Arabs a little bit, and we didn't have too much respect for their fighting ability.
ZIERLER: It could be understood in purely secular terms, as far as you're concerned. [Laugh]
YARIV: Or maybe God made them! [Laugh]
ZIERLER: Well, let's fast-forward all the way back to the 1980s, if you will. We left off last time where, by the end of the 1970s, there's a maturity in the field at this point. Ortel, high-speed lasers. More and more companies are recognizing the commercial possibilities. Just to set the stage scientifically, you have your work here. Are you already starting to produce graduate students who are going into industry, and they're starting their own laboratories, their own commercial interests? Or this is still early in the process? For example, Nadav Bar-Chaim, where is he at this point?
YARIV: Even maybe one step backwards. I probably told you, or should've told you, that when we made the first DFB laser that essentially became the solution for a single-frequency, pure-tone laser, AT&T, at the behest of Western Electric engineers, bought their lasers from Hitachi. Hitachi was involved with us in inventing and developing it. One of my students, an Israeli student, called Sasson Somekh, joined Bell Labs and basically took our technology to AT&T and taught them how to make them.
ZIERLER: Was this before or after the lawyers sent you, from AT&T, the cease and desist letter?
YARIV: That was after. They were unable and didn't know how we were making our single-frequency lasers until Sasson Somekh, who was in the group, went to Bell Labs and taught them how to make single-tone lasers, how to make gratings. Or the idea of gratings, period.
ZIERLER: And was this the same technology that AT&T the lawyers on you to tell you to tell you to stop your work? Or this was different?
YARIV: AT&T wasn't linked to any particular device or product. The letter they sent us just said, "We are conscious of work that you are violating our patents, so the only way that we will allow you to do it is for you and us to sign a mutual patent agreement." I think that was even before the issue of the single-tone laser and our solution at Ortel. Just a shot across the bow. Maybe it's routine for them to do it to any technical company. Arrogant.
ZIERLER: How did you feel when your student took this technology to AT&T? Were you upset? Did you understand? What was your reaction?
YARIV: I don't recall being very upset about it.
ZIERLER: Should you have been?
YARIV: Well, I'm not even sure we even asked him to keep it a secret or anything like that. But I remember I wasn't very upset. And I kept interacting with the student later on.
ZIERLER: Maybe we can back up. With your students, your role as a mentor, what agreements did you reach formally and informally about what they can take with them in their own careers?
YARIV: It was understood in spirit and practice that everything they do here is open property at Caltech. Because asking them to keep it confidential would've benefitted Ortel, not Caltech.
ZIERLER: Which is a great explanation for how important it is to keep those administrative divides between the university and the business, and not to have graduate students work at Ortel.
YARIV: None did. Some joined after they finished, but not at the same time.
ZIERLER: Now, if an employee at Ortel took this technology to Bell Labs, that's a different story.
YARIV: That's a different story altogether.
ZIERLER: Did you have any of those issues, given how valuable the research at Ortel was? People defecting, leaving, leaking?
YARIV: I don't think we ever expected that that would remain a secret forever. We understood that during a given window of time–what made Ortel a success at first financially was the linear laser. The ability to make a laser with a light output directly proportional to the current input, which enabled you then to take electronic information, digital or analogue, and piggyback it on the back of a light beam. It's that linearization. When you detect the light, you get back the original electronic current. The Bell Labs hostile letter taught us that we are better off at Ortel, keep the ideas internal for a few years. After that, they'll leak out one way or another, via employees or what have you, and do the best we can.
ZIERLER: And keeping a lid on it for one or two years, what are the benefits scientifically and financially?
YARIV: Remember, Ortel had no scientific interest. It was a company to make a living, be profitable.
ZIERLER: Because all of the technology came from Caltech, all of the science. It can't be purely a business.
YARIV: It came from Caltech, but it was also published work. And the main development that made Ortel a success was the linearization of a laser by an ex-student of ours, who was an Ortel employee at that point, which was the key. I told you the story probably why it was important, the trade show and all of that. But that was Ortel. Ortel was really never interested in publications. It's interesting, the people who were here, students, some of them, the good ones, were all for publishing over there changed the color of their skin. What's good for the company. And it paid off. Most of the students became very wealthy. And Sass gave Caltech a big gift later on.
ZIERLER: Yeah, it works out.
YARIV: Spread your burn on the water, huh? [Laugh]
ZIERLER: There you go. When your student went to Bell, what did AT&T do with this technology? How did that change where they were headed?
YARIV: Well, they started making these lasers and used them in the system.
ZIERLER: How did it improve the system? Just give a sense of the infrastructure there.
YARIV: Now, we are getting into second-order details, which are probably not aware of it, but not in a fundamental way. There was a significant improvement that came later on from a combination of Bell Labs and an MIT professor, which is still used today. And what that did is that, with our lasers, the DFB laser, maybe 20, 30% of the laser, or 50, were not usable. To do that, we had to essentially reflect it from the end. And some small variation in the way you make the grating was invented by Bell Labs together with MIT, Hermann Haus, a very, very good professor. And that also was incorporated into the way they're made. But the basic idea was the grating. It's an internal filter built in, and that was elegant.
ZIERLER: The DFB laser, just to give a sense, where is AT&T deploying this technology? What technologies are being used?
YARIV: The whole optical fiber communication internet is conducted by light beams, infrared, moving inside fibers, which are the optical waveguides we talked about, and the source, to take advantage of the properties of the fiber, you need pure-tone lasers at a very specific wavelength. That's the wavelength at which the group velocity dispersion is minimal. Group velocity dispersion is what causes it to spread as they propagate. And the properties of the material, of fibers, at 1.5 micron, that dispersion is minimal, and you can go farther before you need to reform, reshape the pulses. At that point, even the patent expired. It's becoming worldwide. Many companies make it all over the world. And they haven't changed much the basic design from the one we made first with Hitachi.
ZIERLER: Now, when you're talking about the internet, fiber optics, this goes all the way back to the 1980s? AT&T was thinking about the internet that early?
YARIV: No, they were thinking about optical communication on fibers. The internet, the protocol, which enables you to send stuff, remember, that came from joint DARPA–had a different name in the beginning. Actually, professors at UCLA, I think, played a key role there. And it was developed in order to enable universities to exchange data. I don't think they envisioned it as the main means of communication in the world. The internet protocol came later.
Nationalizing Fiber Optic Technology
ZIERLER: What does the infrastructure look like in the 1980s for fiber optics? What are AT&T and other companies trying to build? What does it look like?
YARIV: Well, we know that what you need for a communication network, the basic components are the fiber, which is the waveguide, the pipe that carries the light from here to there–and by that time, it's clear that you can go across the continent and across the oceans with optical fibers, with light being re-amplified every 30, 40 miles. The invention of optical fiber amplifiers was a huge invention. That's English, University of Southampton. That means that light propagating in a fiber and diminishes, decays in intensity can be re-amplified by a factor of 1,000 in power, and do it every 30, 40 miles. And without leaving the fiber. There's a section of the fiber, maybe ten feet long, which is pumped from the outside and acts to amplify the propagating beam, including the information.
And that hasn't changed. The key components are the optical fiber, the semiconductor laser supplying the proper wavelength and single pure tone to take advantage, a modulator to put information on the laser beam, and detectors. The two breakthrough devices here are the laser and the amplifier. The others are good electronics that any good company could make. The first telephone exchanges were probably rather simple, including basic components. AT&T had to learn how to take all the telephone companies–oh, to take advantage of the bandwidth of the lasers, you had to learn to interleave. I mentioned that probably a fiber can carry on one laser beam thousands of phone conversations, if not tens of thousands.
And that means that your string of ones and zeroes representing your speech are then separated and combined with thousands of other speakers, and then separated again to reform your speech on the receiving side. That's electronics. I don't understand how it works. Never tried to. It's a sophisticated specialty, but a knowledge that was widespread, worldwide. Takes good engineers.
ZIERLER: To go back to your sensibilities, when you're developing these lasers, and you're strictly in a basic science mode, just figuring out how to make them work, how far afield are you thinking in terms of infrastructure, applications? And are you specifically thinking that you're going to design a laser in a particular way so that it can be used in fiber optic communications, for example? How did these things work?
YARIV: They were not planned and directed logically. If I were to rewrite history Russian-style…
ZIERLER: You would say you had this grand vision.
YARIV: Great vision one day. Epiphany, eureka, or whatever.
ZIERLER: You're not even thinking necessarily of communications when you're building these lasers.
YARIV: Not at first. Not at all. Briefly speaking, I came to Bell Labs, I did other things. Asked to look for new lasers, regardless of whether they walk, jump, sing. Lasers. And I wind up on a team that invents the world's number-three laser using uranium and calcium fluoride. Then, I am asked politely, but I understand I probably cannot refuse, to work on semiconductor lasers, which I invented elsewhere. Become very interested in them. The challenge is just to make them at first. Bell Labs didn't know how to make semiconductor lasers, so we made them. We now could make lasers that way. And even at Bell Labs, at AT&T, the idea that those lasers would be foundational in optical fiber communications systems–I'm not sure whether optical fiber was even invented at that time. No, it was not because it was a parallel effort at AT&T in New Jersey–lasers would be used for transcontinental communication. What will they really be like? And the main candidate was a sequence of evacuated tubes. Laser beams would be shot down the tubes, and there would be lenses every 100 feet or whatever to refocus them. A metallic tube, lenses, and the laser beam will–that's the optical beam. In my book, you will see a description of such a system. It's a lens relay. The lenses were not going to be glass, they were going to be gas lenses.
ZIERLER: What is a gas lens?
YARIV: You'll admit some gas, CO2, and the gas paper can tell you that if I make a nozzle of a certain shape, the gas can do something equivalent to a lens. It'll be denser in the middle, and it'll be a weak lens. And that was what they were working on until the news broke about a Chinese scientist working in England who got a Nobel Prize for realizing that silica could be made very pure, and therefore, very, very low losses. Optical fibers were known before but were used for Christmas, Hanukkah decoration. You shone light into that, and the light came out sparkling from the other side, maybe a meter long. They were a curiosity. And the reason was that the light decayed almost to nothing in a few feet. Nobody paid much attention to fibers as long-distance optical waveguides.
This Chinese fellow, Kao, got a Nobel Prize as a graduate student in London. He did some experiment and said the reason that optical fibers absorb light so intensely is iron impurities or other impurities, and you have to learn to make a pure silica to make the fibers out of. And he did some experiments that demonstrated it was true. He could maybe transmit light in his fibers ten meters instead of one meter. And then, Konig, who was the world expert on manipulating glass, managed to get the losses down to 20 dB per kilometer, meaning the light would be down to 1% of its initial intensity after one kilometer, and that was enough to convince AT&T engineers it could be the basis of a transcontinental transmission of light, so they abandoned the pipes and all of that. To go back, the reason I was working on semiconductor lasers at Caltech with a group of students was, I was asked to work on it at Bell Labs. Before I left Bell Labs, I probably spent two years or so.
Once I started working on it, I became absolutely drawn by trying to understand why the laser lased and then the role of optical waveguiding. I cannot over-emphasize it. Without optical waveguiding, you have no laser, and you don't integrated optoelectronics either because light has to be taken from one location to another on the devices to be manipulated, reshaped, put information on it. You have to guide it. The guides are like wires in the original TV set. And then, I come to Caltech. In spite of the fact that I loved what I was doing, my problem was, I had that interim working for a company up north. But I was at Caltech, and I had to decide what to do. And then, I made a key decision, to work on semiconductor lasers. At that time, the reasoning I recall, I said, "Look, you have a laser." Lasers at the time were tabletop. The argon laser, which was one of the first lasers emitting blue light and was used for maybe ten years at the main lab, was that big.
Required kilowatts of power to run it, water to cool it. Here's a laser, which is the size of a grain of salt. The actual working part is much smaller than that. It's electronic. It's very efficient. And it was the statement [that] it's going to be important. And I don't think many people understood that. That was the reason that I decided to stake my initial career, and it was, in retrospect, a very [good bet]. Starting to work on the lasers, in parallel, because I had maybe a group of ten people, let's say, after three or four years at Caltech, including some good students, one or two post-docs, one from Japan, and I had an abiding interest that started earlier, completely unrelated to lasers, related to my master's thesis, of how electromagnetic waves propagate in periodic structures.
This was also related to physics. The electrons are waves, and an electron propagating in a crystal, as it does in a semiconductor, if the crystal is a periodic structure, it turns out that basic notions in math of electrons, solid-state physics, work by Felix Bloch, Nobel Prize, Bloch waves, how they propagate, and how microwaves propagated in a traveling-wave tube in a helix are basically the same. I had a strong interest in periodic structures. But instead of working on microwaves, I started working on semiconductor lasers, but also, for instance, I wrote a paper or two on X-rays, short-wavelength light. And X-rays and crystals, Bragg reflection, is again, radiation propagating in periodic structures. And that was the reason that eventually, when the demand from AT&T engineers came for pure lasers, our solution of the distributed feedback laser was related to that work.
There were other proposals for DFB, UV radiation of big crystals. Our solution of corrugating an interface was due to my background from Berkeley. We were doing all of that, never thinking about optical communication. It was a gradual process. The role of lasers and semiconductor lasers in optical communication was a gradual process. And we learned as the world did, really. We didn't invent that. But we had a solution for the DFB laser.
ZIERLER: Now, AT&T, of course, is still a monopoly at this point, before Judge Greene. Were there other communication businesses that were in the game at this point? Or it was only AT&T?
YARIV: Other companies were getting commercial companies interested in making DFB lasers. But AT&T, I would say, is the only company probably at first in the world, maybe a Japanese company, NTNT, because they were closer to the source of good lasers, Hitachi.
ZIERLER: Yeah. AT&T is an American monopoly. They don't have a stranglehold in Japan or Europe.
YARIV: NTNT is the AT&T of Japan. And they're still the monopoly.
ZIERLER: No Judge Greene in Japan.
YARIV: No, [Laugh] no Judge Greene.
ZIERLER: What about in Europe? Any parallel companies in Europe doing this, what AT&T was doing?
YARIV: No.
ZIERLER: They're behind?
YARIV: They're behind. Later on, especially the English, the invention of the optical fiber, optical amplifier is a huge invention. Without it, there would be no optical fiber communication. And that came from the University of Southampton, not Cambridge, not Oxford. A red-brick university, as they call it.
No End in Laser Applications
ZIERLER: In talking about industry, our conversation appropriately is focused on communications. Where else do we see applications for this technology from Caltech? What other industries? Military, medicine, optical? Where else do you see at least the ideas germinating from Caltech from your work transfer to other industries?
YARIV: Well, the laser is gradually becoming the transistor of light. Every optical electronic company in the world is working on lasers and semiconductor lasers. Even the original light sources that took a tabletop to operate are being abandoned in favor of little devices, where light from a semiconductor laser, which typically is in the infrared, is doubled and tripled in frequency by nonlinear optical tricks. Today, you can make and buy sources of blue light, which instead of that whole table, are maybe a small box of some electronics emitting a watt of power. The laser was becoming so widespread and ubiquitous. Everybody was doing it for different reasons. For cutting, for defense, anti-missile defense probably. All over the place. Laser RAs to get out of power, metal cutting, fabric cutting, medicine. But we stayed with the communication aspect of the laser all along. Other applications were to solve a problem, how to cut, how to drill. Communication was kind of endless. Bandwidth, noise. And the center of gravity of our work was shifting to making the laser purer and purer. And that's an endless game. We made, I'd say, the important contribution, even until a year or two ago, last papers on the subject.
ZIERLER: To stay on the military track for a second, when Ronald Reagan was talking about Star Wars in '82, '83, lasers in space, were you following this at all?
YARIV: More than following. There was a committee of the American Physical Society at some point to investigate the feasibility of Star Wars, and there were maybe 20, and I was one of them. We were constituted by the APS, and we spent a few months with full collaboration with the DOD, who told us about the plans and so on.
ZIERLER: Did you have to get a clearance for this work?
YARIV: I had a clearance. All of us were cleared. All got a clearance for the occasion. And we wrote a report. I can give you a copy of it. It had magic, a large aura on the left side, in which we basically were very critical. Claimed it probably would not work. For many reasons.
ZIERLER: Who was the key booster in Star Wars? Who gave a scientific imprimatur to this?
YARIV: Edward Teller. The Teller connection–remember, Teller eventually had a falling out with Oppenheimer. As a matter of fact, he later on helped make Oppenheimer a persona non grata under security clearance and so on. But Oppenheimer had Los Alamos. Teller, now, is very important, father of the H-bomb, which isn't true either.
ZIERLER: Dick Garwin, you're thinking of?
YARIV: No, no, not at all. A Jewish mathematician from the University of Krakow, who winds up a superb mathematician during the War, working in the theory group in Los Alamos. He's considered the father of game theory, who had the idea to make an H-bomb. Anyhow, he reports to Teller, and Teller essentially shoved him aside. But Teller, then, could not work with Oppenheimer, so DOD and Department of Energy built Teller–you'll have to check my facts here because I'm not a historian [Laugh]–Livermore to develop the H-bomb. So Teller has Livermore. 1,000 PhDs. He also starts an Applied Physics Department at UC Davis. And then, there is an X-ray project at Livermore.
There's another scientist who's much younger who's in charge of that effort. And among them, the key scientific person in that effort is my student, a very brilliant fellow who went to work at Livermore. This group of hot-shot PhDs, and my student is doing the basic X-ray physics work, to this day, probably the world's leading expert. And he has Teller and the other redhead, slightly fanatical, peaking over his shoulder every day. And one day, they decided, not my student, that it was good enough to base an anti-missile defense. The X-ray laser is a stage where they can base an anti-missile defense system. The information of my student is not available because my student used to come and visit here and tell him what's going on. Teller uses that knowledge, saying, "We now have an X-ray laser capable of shooting down intercontinental ballistic missiles," and that's how Star Wars starts.
ZIERLER: Did Teller oversell it, or did he not understand the science himself?
YARIV: He probably did not understand it on the level needed to really do that. The X-ray spectroscopy of what goes on there. But he understood the basic concept. He looked at the data, probably did not understand it. Not that he didn't have the intellectual power to understand it, but probably never took the time.
ZIERLER: And how did the APS committee form? Did the DOD commission it?
YARIV: No, no, the American Physical Society decided that they ought to look into it. Who else would do it? They didn't trust the Army or DOD. They said they needed an independent feasibility, and they formed their own committee.
ZIERLER: And who chaired it, or who asked you to join?
YARIV: It was chaired by Nico Bloembergen. A professor of applied physics at Harvard. Considered more or less to be the father of nonlinear optics, got a Nobel Prize for that. I think he chaired it. Maybe co-chaired by a Bell Labs scientist named Kumar Patel. Los Angeles-based right now. Key role in developing, not inventing, the CO2 laser.
ZIERLER: What were the conclusions of the committee?
YARIV: That it wasn't ready.
ZIERLER: It wasn't ready then, or it would never be ready because the physics didn't make sense?
YARIV: It was implied that the gap between what we had and what we needed was so large, it wasn't likely.
ZIERLER: Technological gap?
YARIV: Technological gap.
ZIERLER: Not a theoretical gap, though?
YARIV: No.
ZIERLER: What technology was missing?
YARIV: Remember, the idea of shooting down a launched intercontinental ballistic missile, at first, the assumption was it would be through the atmosphere. And the conclusion was that we probably could not destroy them as long as they were in the atmosphere because destroying them with X-rays or laser beams, they would be absorbed in the atmosphere or distorted, so they would not be able to focus them down to the intensity level needed to destroy an incoming missile. Once they were in the atmosphere, they were moving fast, and there was a question of acquisition, tracking, destroying them in a limited time. There was an issue of decoys. The enemy can launch a large number of decoys that, once in the atmosphere, will open and launch the decoys outside the atmosphere. And then, with no air resistance, they will move and look like the ordinary missiles. So the defense system would have to discriminate between the real missile, the warhead, and the decoys. Those are, for instance, the two main problems. And the laser it would have, to avoid the atmosphere, would have to probably be on satellite or outside the atmosphere as well. And there's a question of intensity and power. The numbers didn't agree.
ZIERLER: Do you know if Teller saw the report or if he responded to it?
YARIV: He obviously saw it. There was no way he couldn't see it. No, he didn't respond to it. Not that we are aware of. He must've responded to it probably to the Army officers in charge of the program. But not officially. I don't think he had the background.
ZIERLER: What about the Reagan Administration or anybody in Washington? What did they think about this?
YARIV: Well, I know the program eventually was curtailed or killed. I think we had a part in doing it. But it had one effect. It ended the Cold War.
ZIERLER: Because the Soviets got so freaked out, they upped their military spending.
YARIV: That's right. they couldn't afford to keep up the competition. And the leader said, "Look, if it's such a bad idea, how come the Americans are doing it? [Laugh] They have good scientists." But they have stupid leaders, like us. [Laugh]
ZIERLER: And then, you mentioned satellites. In the focus of communications, when did you really start to pay attention to lasers and satellites for communication?
YARIV: The truth is, apart from being called to serve on a committee, we were not so much interested in optical communication, the whole surrounding technology that has to accompany a viable communication network, the electronics, the fibers. We concentrated on the laser. A parallel effort here all along was nonlinear optics. And with some fundamental issues with the laser, and that's Caltech, and especially coherence. What's the limit to the laser coherence? And how can we reach it? I think we may be partly responsible for the fundamental theoretical published analysis that showed which way to go, and most of them were within the last ten years or so. And the idea is basically that the key to make lasers spectrally purer and purer–to give you an idea, at the time that the explosion in optical communication takes place, the laser lined with the spectral width of the laser beam put out by the semiconductor laser is roughly half a megahertz.
Five times ten to the five Hertz. We had brought it down to less than one Hertz, meaning almost six orders of magnitude improvement. And even pointed out that we can go even farther. And that's important for things like GPS, atomic pumping, quantum computing, pure light. And what we pointed out, which is now accepted, is that the key is to make the laser resonator. A laser is a gain medium inside a resonator. Initially, just two mirrors. Our grating acts as a resonator, too. The DFB laser. Make it have very, very small optical losses. Those optical losses eventually limit coherence. Loss and coherence always go together. And the reason, in the laser, if you have losses, you have to compensate from them. Even small, you still need gain to make up for them. To make an oscillator, you need to have gain equal to losses, and then light can bounce back and forth, get purer, and purer, and purer. A simple way of looking at it, the more times it bounces, the purer it gets.
Every time, it sheds off some noise. How many times it can go around depends on the loss of the resonator. The Q of the resonator is related to the number of bounces. You cannot work in optics today, or lasers, without understanding Q. Q is a measure of the loss, essentially. We started with Qs of 10,000. Now, we have Qs of billions. Q is the symbol. It's a figure of merit of an optical resonator. Actually, any resonator, mechanical, optical. Any resonator could be a mechanical resonator. The frequency, omega, times the stored energy–a child on a swing has a certain amount of kinetic energy–divided by the power lost to anything. If you excite a resonator, put a child on a swing, give it a good push, and if you look at its oscillation, the oscillation amplitude decays as E to the -T, time. That's the height of the swing. You push the child initially and let it go. Of course, you don't push it anymore, so the oscillation will decrease every time. And the oscillation gross is over tau.
Tau is equal to Q over omega. If you have a large Q, this number turns to zero, and therefore, the oscillation doesn't decay. Q is a measure of the losses. But that particular definition is consistent with this, and that's very important. Any resonator is measured by Q. If you remove the losses, Q gets higher, and higher, and higher. And the line width of the laser is related to the Q. The key of more recent work, both theoretically and in the lab, is how to make semiconductor lasers with high Q with small losses, so that once excited, light can bounce many, many times. And our latest work has stopped. I still have a post-doc here cleaning up the mess. We could see our way, if we had the money, to go into sub-hertz line width, which probably translates to a GPS with an accuracy of a few millimeters. [Laugh]
ZIERLER: Is there a Moore's Law equivalent here?
YARIV: Things get harder. Moore's Law essentially says that you can make the transistors smaller by a factor of two, and therefore, pack twice as many of them every couple of years or so. And Moore's Law is running into a brick wall, as we talked about, mostly because the latest transistors by TSMC and ASML, the Dutch company that makes the lithography equipment, is down to the–we're talking about two nanometers, which is 20 angstroms, which is five atoms. The next step will be making a transistor of two or one atom. Nobody knows how to do that. We are limited by the purity of the materials we can get and how well we can fabricate them to make a grating. And the best people who are doing it now, not us, can make resonators with Qs of ten-billion. We did our work here with resonators of up to ten-million. And we actually made lasers which were 10,000 times purer than commercial lasers, even today, give or take.
ZIERLER: To go back to satellites for a second, was JPL ever an asset for you? Were they doing things that were important for you? Did they care about what you were doing?
YARIV: Yes, they were. Maybe with a slight exception, we never worked together. A few of my students went to JPL and got involved in laser projects, and important ones. One of them is how to synchronize receiving antennas, antenna arrays. To make them into one huge antenna, to increase the resolution, you have to control the phase of the microwave. The synchronization of antennas, which was important for operating antenna arrays, and therefore, optical communication with space vehicles, satellites, Mars, is done by means of optical fibers. We interacted with them, students, but we didn't work together. I don't think they had the quality of people I would've required, of our students. And the students who went to work for them were good. And they did important work. But work that was no longer of interest to me.
ZIERLER: When you talk about the explosion in fiber optics, when does this happen? Are we getting into the mid-1990s now, when we see the internet coming into people's houses? When do we see this?
The Corning Connection
YARIV: That explosion owes a total debt to Kao, who showed the way that optical fiber could be the means of distributing information on earth. And I'm not exactly sure when he did his work. It must've been early. Probably the 80s. Charles Kao. Optical fiber. Can you look it up?
ZIERLER: In 1966, he laid the groundwork for fiber optics and communication. Oh, he passed away. It's sad. 2018, he died. He was vice chancellor of Chinese University of Hong Kong from '87 to '96. This is all administrative work, though. In the mid-1970s, he did seminal work on glass fiber fatigue strength. "During this period, this is the mid-1960s, Kao pointed out that the high purity of fused silica made it an ideal candidate for optical communication. Kao also stated that the impurity of glass material is the main cause for the dramatic decay of light transmission inside glass fiber rather than fundamental physical effects, such as scattering, as many physicists thought at that time, and such impurity could be removed. This led to a worldwide study in production of high-purity glass fibers. When Kao first proposed that such glass fiber could be used for long-distance information transfer and could replace copper wires, which were used for telecommunication during that era, his ideas were widely disbelieved. Later, people realized that Kao's ideas revolutionized the whole communication technology and industry."
YARIV: The people who took the next big step and actually made fibers, the main name is Snitzer at Corning.
ZIERLER: Yeah, Elias Snitzer.
YARIV: That will give you the time. It was after that, what you call the explosion in optical fiber communication.
ZIERLER: "Snitzer was hired by American Optical, where he began his work in optical fibers and lasers. In 1961, he demonstrated the first optical fiber laser and the first fiber amplifier. Snitzer remained at AO as Director of Corporate Research until 1977. He then moved to United Technologies Research Center. From '84 to '88, he worked at Polaroid Corporation, where he directed research and development of fiber and integrated optics programs for communication, sensors, and photographic instrumentation. At Polaroid, Snitzer and his team first demonstrated the double-clad fiber laser in 1988. In 1989, he joined the Ceramic Science and Engineering faculty at Rutgers, where he continued to teach and research fiber laser amplifiers, glass, and fiber Bragg gratings until his retirement in 2001."
YARIV: Then, I might be wrong. They don't mention at all Corning. Corning Labs, Corning, New York.
ZIERLER: Corning comes into the role after learning about what Kao does in 1970. It's Robert Maurer, Donald Keck, an experimental physicist, and Peter Schultz, a glass chemist. "The team had fabricated yet another new variety of fiber, and late on a Friday afternoon in August of 1970, Keck inserted this latest sample into the team's equipment for testing. Keck was eager to start his weekend but wanted to measure this latest attempt before going home. Bending over his microscope, he was astonished by a bright pinpoint of light. Later, Keck described it as, 'The most glorious thing I'd ever seen.' Light lost is measured in decibels." So it's Keck.
YARIV: Over what distance was it? I think 20 dB per kilometer, something like that.
ZIERLER: Yes. "The light-carrying ability of glass needed to exhibit this new fiber measured between 16 and 17 dB. It was as if, Keck said, he could just about feel the spirit of Edison in his lab. In his lab notebook, he wrote, 'Whoopee.'" [Laugh] Did you know Keck? Were you following these things?
YARIV: Yes, of course. It was a big thing. We understood that meant optical communication was a reality.
ZIERLER: Now, to go back to what made you think of Kao and to research for Keck, the original question was the explosion of fiber optics and the timing of this, when it goes from this early research in 1970 to fiber optics everywhere. When does that really start to happen?
YARIV: It was a gradual process. As the fibers got better and better, you got to a point where the losses were maybe a dB per kilometer now, something like that. Really reaching the basic–the Q number goes up. They mentioned, actually, that Maurer made the first optical fiber amplifier. He's usually not give the credit for inventing it. At least, the invention of the version that turned out to be practical was done by the English at Southampton. And without the ability to amplify the light in the fiber, without taking it out of it, we would not have the optical fiber communication. That means that every 10, 20 miles, you will have a little building, maybe a pillbox of concrete, full of electronic equipment to remove the information from the laser beam, amplify it, reshape it, and relaunch it. You couldn't easily do it underwater. [Laugh] And here, basically, every 20 miles or so, a section of the fiber is treated a little differently during the manufacturing, and essentially serves as the amplifier. Light goes in, comes out. I have a chapter in my book, one little thing I did, basically, how far you need the amplifier to get the best results, etc. I have a chapter talking about optical amplifiers and the noise consideration. You can get an idea of what's involved. It was a big invention.
ZIERLER: Last question for today, we'll get into more details in the 1990s and the turn of the century. The quest to make lasers purer and purer, to what extent was that motivated by the opportunities that the fiber optic infrastructure provided? Or did you see these developments separately?
YARIV: The optical fiber has reached such a degree of perfection, and that must've happened even 20 years ago, that it's no longer really in our circle an issue of conversation even. We take it for granted that the optical fiber technology is much closer to the unattainable perfection than anything else we have. We don't spend much time talking about fibers anymore.
ZIERLER: The idea is that the fiber optic technology provides a perfect tunnel, and it's on you to make the laser as perfect as possible to travel through it?
YARIV: That's right.
ZIERLER: We'll pick up for next time.
[End of Recording]
ZIERLER: OK, this is David Zierler, Director of the Caltech Heritage Project. It's Tuesday, November 30, 2021. Once again, it's my great pleasure to be back with Professor Amnon Yariv. Amnon, as always, great to be with you.
YARIV: Thank you. And now that you're here in California, you need to learn a little Spanish, so igualmente.
ZIERLER: Igualmente, again.
YARIV: Equally so on my part.
ZIERLER: Por supuesto! Amnon, today, we're turning to the late 1990s, turn of the century. When the dot-com boom met the fiber optic infrastructure that was already being built at that point, what did that mean for you and the research you were doing at the time?
YARIV: You know the Ortel story, we don't need to talk about that anymore. That, of course, benefitted hugely from the dot-com, right in there with converting cable television from cables to optical fibers. We at Caltech, any university, we don't look at the global picture. We have our local problem and interest. It's only in retrospect that you're trying to see big trends, pictures. With the semiconductor laser, which we'd been working on, we'd developed a commercial version called the distributed feedback laser, the DFB, and it became clear at that point that it was a very important laser.
ZIERLER: How did it have to change for commercial use?
YARIV: Well, let's take the DFB laser as an example. At Caltech we were just making a different type semiconductor laser that was radically different. That provided the necessary feedback by corrugations -waviness- in a thin layer next to the waveguiding from the bulk stored optical energy of the laser mode. (the understanding and the confidence that it could work that should its feasibility came from an analysis I published around that time "Coupled Mode Theory for Guided Wave Optics" which I had done about that time. This is not a world changing but it was important in the world of optical resonator theory. So, we had this basic understanding and it came out of pure curiosity. Like, "if electrons can propagate in a periodic crystal-why cannot guided light waves propagate in an artificially periodic waveguide with aa surface (grating rather than volume)?" The mathematics and basic principles in both cases are very similar. And the when the Engineers at Western Electric started crying out for a single frequency laser we thought we might have a solution- and we did. It worked beyond all expectations and even today some 40 years later the DFB SC laser is the sole light source for optical communication. All over the world. Normally we would have written a couple of papers, gave a couple of talks and gone on. Commercially, that's not enough. If you invented it in industry, that would be maybe 20% of the work. The packaging, making it reliable, inexpensive is probably the bigger engineering challenge. But the basic idea required the fundament al understanding and the curiosity and, believe, was only possible at a university setting with a modicum of financial support and of students Not as creative, but as important commercially. We didn't bother about all that. That was done in industry at Ortel, Hitachi. But to us at Caltech, we kept on going. The Ortel effort was separate. I was involved personally through consulting. At Caltech, we started shifting emphasis into other aspects of the semiconductor lasers that were interesting fundamentally and even practically, further on. The semiconductor laser, any laser, was sold initially–the main excitement was coherence. Here, you had a source of coherent light.
And it became clear that coherence was a limiting factor on how much data you could transmit in optical fiber communication speed. And the distributed feedback principle, the DFB, was a partial solution to a coherence problem. Going from a laser that emitted many wavelengths simultaneously, which was not desirable and a limiting factor, to a laser emitting a single tone was a big step toward a more coherent laser. But even if you had a single-frequency laser, which we had then, the question is, how pure is that one single frequency? And there will probably be a push to make the laser more coherent forever.
ZIERLER: There's no limit to pushing on the coherence?
YARIV: There is a limit, but we'll probably never quite approach it for practical reasons. Part of the effort here at Caltech moved in that direction, and, I would say, went on until maybe a year, half a year ago. We basically showed the recipe in a series of papers from here of what was limiting the semiconductor laser. Once we solve the problem of multi-moding (many wavelengths, what can we do with a single? Our DFB laser had a line width of with roughly half a megahertz. And we have pushed it within the period of starting in the turn of the century until maybe a year ago. We managed to reduce it from 500,000 Hertz, half a megahertz, to essentially one Hertz, talking about five to six orders of magnitude, by a fundamental understanding of what causes that line width. Essentially, spontaneous emission.
The fact that in any laser, the basic process for generating light is stimulated emission. Light stimulates atoms or electrons to drop to lower levels by induced emission. But at the same time, in parallel, there is a process where some of the transitions are spontaneous, and that's noise. Meaning they're not related at all to the presence of radiation. And that's noise. It's a round process. And understanding the theoretical mathematical basis, we essentially invented a new genre of lasers. And the main recipe was a drastic reduction of losses.
ZIERLER: Technically, how do you achieve that?
YARIV: Paper?
ZIERLER: Of course. Always.
YARIV: The laser of, let's say, the year 2000–this was P gallium aluminum arsenide. P-type. N-type gallium aluminum arsenide. Then, went to a battery. Power supply, current. Gallium arsenide. And light. That's a laser. And of course, we had the DFB. The correct way of looking how light propagates here, the profile of the light intensity, the light that propagates back and forth is like that. And that light is moving like that. Most of the light is in that inner layer. The inner layer's gallium arsenide. Those are gallium aluminum arsenide. Remember, a semiconductor laser might have guiding. Light must be trapped. And the way you trap it is by making a dielectric waveguide. N equal to N2, N1. If N2 is bigger than N1, you have guiding. That means the light is concentrated to the inner layer. But concentrated means that theoretically, not only is it concentrated here, all of the energy propagates in that direction. Light doesn't go out to the side. That was the laser then. We have a line width of half a megahertz. I call it delta nu laser. If you took the light of the laser and analyzed its coherence, you'd find out that it wasn't a single frequency, but a single frequency smeared over half a megahertz.
ZIERLER: A single frequency smeared over half a megahertz?
YARIV: To understand this concept you need to into Fourier analysis -the most important mathematical tool for communication theory and for much else.
YARIV: Let's talk a little bit about line width. Every physical phenomenon can be described either in the time domain–let's say you have music, or pressure wave, or anything. And you put it in an oscilloscope, you're going to see–that's in the time domain. And that, we'll say, could be the voltage out of a speaker. You have a microphone over an orchestra playing, and the output of the microphone is a current, a voltage, and it looks like that, a function of time. And that's Beethoven's Fifth Symphony. You can equivalently mathematically also look at it at the frequency domain. Same signal. The phenomenon in the time domain. Knowing that, you can derive that. As a matter of fact, F omega mathematically is minus the integral from the infinity of F of T, E to the I omega T, DT. And F of omega is the integral minus infinity to infinity F of T, E to the I plus omega T, D. F of T, F of omega, D omega. Given F of T, you can derive F omega and vice versa, given F omega, you can derive F of T. Simple integrals. Fourier integral pair. To form a pair, it's enough to know one of them. Fourier integral. Probably the most important mathematical relationship in communication.
And probably in physics. That's the real phenomenon, the analogue. That's an exact mathematical action. You need nothing more. In practical terms, that tells you this signal is made up of many frequencies. And that's how they're distributed. A pure tone only has a single frequency. For pure tone, I'll give you one example. That will be your lesson in Fourier. Pure tone, which is the mathematical idealization, a pure sine wave, or cosine. Could be, for instance, A cosine omega T. F of T, in the time domain, a pure tone is a pure wave. If you do this mathematically, you find that F omega is equal to O. Let's call it omega naught. This is the distance between two peaks, the period, is 2 pi over omega naught. This distance. If you take the Fourier transform of that, you get function in omega space. It's now a function of omega. This omega. And it's delta omega naught. The same physical phenomenon in the time domain is this. In the frequency domain, all the energy is at one frequency. Beautiful thing is that if you, for instance, built a filter that eliminates this frequency, you can block it completely.
The whole idea of filtering is done conceptually in the frequency domain because until maybe 30, 40 years ago, the idea of building a filter to shape or eliminate frequencies had to do with basically working in the frequency domain. You make a filter that eliminates a given frequency or allows it. In communication, engineers actually work bandwidth–let's go back to this one. What you are saying, for practical purposes, the energy of this wave is concentrated in this frequency band. There's no energy outside here. This is the bandwidth of F of T. The time signal, the voice, the music, the singing, is made up of frequencies. When I tell you, for instance, that we can only hear, and our speech contains, frequencies roughly between 0 and 5,000 or 10,000. That means if you took our speech and fully analyzed it, you'd find out that human speech F omega, omega–and this would be roughly, let's say, 10,000 Hertz.
When you talk you produce a mixture of frequencies between 0 and 10,000 Hz. Communication engineers really talk mostly in the Fourier domain. Bandwidth. If you buy memory, so many gigabytes–recently, the lines have been blurred, and now people do filtering instead of making actual filters. The two fields are kind of getting mixed. But basically, communication, bandwidth, all of that is in the Fourier domain. One, two, three. The laser now is a single tone, the DFB. But if you Fourier analyze it, and the instruments to do it, you can buy from Hewlett-Packard or whatever, so DFB laser, you took that light from the laser and shone it into this instrument, which is a spectrum analyzer–spectrum is another way of talking about…here–you'll find out that our laser, which ideally should have all its energy at one frequency, a pure sine wave, actually has line width of DFB. The laser puts out a line that has frequencies centered on the ideal frequency, omega naught, but spread over–now, that's not due to imperfection, that's a reflection of the uncertainty principle.
According to the uncertainty principle, there are pairs of variables that cannot be simultaneously measured with arbitrary accuracy, like position and momentum of an electron. If you want to measure position accurately, you have to give up information about speed and velocity. Frequency and time are similarly conjugate variables, variables that cannot be measured simultaneously. And that is a direct mathematical consequence of the fact that they are related by a Fourier relationship. That's a consequence of Fourier. But the quantum limit really says that we can fundamentally make the spectrum narrower, and narrower, and narrower, purer and purer, if we are giving up information about timing. The point is, we can make it purer. This is not a limit due to what we call the schmutz effect. Schmutz, dirt trapped in a highway. It's a quantum limit. But a quantum limit is flexible.
You can massage it. We spent over ten years developing the theory. And why is it important? Because that line width, the fact that it's not a delta function, that if you looked at the laser output in the time domain, instead of being the ideal–this is a pure laser. Ideal laser. The consequence of the fact that the spectrum of the laser is not a delta function, but it has a finite width, which is quantum limited, is that instead of looking like a pure sine wave, you will see a little deviation. And that limits the amount of information that you can carry on the laser for fundamental reasons. We undertook a project that involved maybe ten doctoral students to understand. What do we do about it? That was your original question. Still, commercial lasers are essentially mostly of this variety. And what characterizes it is that the modal energy of the laser–this is the intensity plot. X, this is F of X.
Light goes like that. The great majority of the light is in gallium arsenide, and gallium arsenide is lossy. A high density of carriers. And losses force the laser to increase the line width. Direct relationship. Remember, we're talking about Q. Our solution was to design a new laser with the modal energy now, add essentially a low-loss transparent layer, redesign the modal energy of the laser, DFB laser, as before. The only difference between this and this, our new laser, we simply redesigned it optically using the Maxwell equation so that the mode, instead of residing in the gallium arsenide, is shifted into a transparent layer, which is actually silicon dioxide. We grew out the layer, which is essentially transparent. We reduced the losses by a factor of 10,000 or more. And that reduces the line width.
The theoretical understanding of what it takes to make a narrow line width laser, and then making it in the lab, which wasn't trivial, in our new laser has delta nu–remember, delta nu is the width. Delta nu laser. The width of the spectrum of the laser has been reduced by about 100,000 times narrower. That's important for communication, for atomic clocks, for navigation, a whole bunch of other things. Atomic clocks on a chip would require such lasers. And a whole slew of other applications, mostly in navigation, measurements, spectroscopy, GPS. That was a major work. Actually, to some degree, still going on. If I were not 91 years old, it would've been a major effort. I would have a big lab doing it.
ZIERLER: Who's picking it up? Students?
YARIV: To some degree, Kerry Vahala here at Caltech, my ex-student who is a professor here. A fellow named John Bowers at UC Santa Barbara. He's exceedingly talented, probably one of the main actors right now. Kerry and John are working together on some project with DARPA support. I was involved until a year or so ago, but pulling out slowly. Coherence.
Lasers Make the Modern Internet
ZIERLER: How has coherence made the internet faster or better?
YARIV: The amount of information that you can transmit on an optical beam in a fiber is inversely proportional to the line width. More coherence, more information. Beats per second. If you took two minutes to download a Netflix movie, you'd be able to download it 1,000 times faster, carry more information, more telephone conversation, more data, more anything. The name of the game today in communication is bandwidth, speed, accuracy. Also leads to errors. Ready for a two-minute lesson in communication theory?
ZIERLER: Please.
YARIV: Remember, the biggest geniuses of the 20th century are not all the names that you hear. [Laugh] A fellow named Shannon. [Laugh] Ideal communication theory for data transmission. After all, most of communication is to take data from here and transmit it to somewhere else. Call it data transmission. Fibers. You start with a pure source of radiation, pure laser, pure microwave signal. And remember, pure means that in the time domain, it is a pure sine wave. Pure harmonic. When I say sine, could be cosine, could be any phrasing that has a pure harmonic wave. That's your basis. That carries no information. Why? Because it's predictable. Information means unpredictability. And then, you have your information. Which is electronic signal, current, coming out of a microphone or data out of memory. Let's say music. Data. Movie. Actually, this should be a sine wave, which is much denser than that. This is the pure ideal light wave. A naught cosine omega naught T. Pure cosine.
My drawing is not very good. Now, you want to use this wave as a carrier to piggyback the information. This is time. We're in the time domain. You make a modulator that's an instrument. One of the main elements of fiber communication, the internet, is modulators where you take the information source that you want to transmit, data, music, and modulate the pure laser beam. Here's a pure laser idealized. And it's this way, pure, ideal. It enters a box, which we call a modulator. Two or three chapters in the book of how to use the electrooptic effect to make modulators. The modulator has the information signal applied to it. It's a current. This is, let's say, an electrooptic modulator. And the pure sine wave now is modulated, meaning turned on and off, modified in some way by the information, comes out no longer a pure sine wave. It's been affected by the information. And instead of looking like a pure sine wave, it might be a laser beam modulated by information.
To summarize in a few sentences, a pure laser beam, ideally, enters a crystal. That's an instrument you buy, a modulator. The information coming from storage, Netflix, whatever, is applied as an electronic current voltage to the crystal. And the effect is the act of modulating the laser beam by the information signal is to modify it from a pure sine wave to something, which now is modified by the information. And if you look at this phenomenon in the Fourier domain, if you take the signal coming out, this is now F of omega of pure laser. The idea is that all the information is at one frequency. Fourier domain. We are talking like a communication engineer. What happens if you now take the laser that has been modulated with information? Remember, we have a laser beam at output of the modulator, carrying information in it. We take the laser beam now coming out of the modulator, out here, and Fourier analyze it, the instrument for you. You'll find out that instead of all the frequency, all the energy of the wave at a single frequency, it's been spread a bit.
Delta omega of the modulated laser beam. This is now the laser beam carrying information. Matter of fact, if the information is the speech of one person, then already the width here is roughly 20 kilobytes. All of that was ideal, meaning no noise, pure laser. Remember, putting in information takes a delta function, a pure wave in the Fourier domain, and broadens it. This broadening now is due to information. It's good. That's what you want. But what if the laser to begin with wasn't pure? If instead of that, the real laser, real world–this is ideal–no information, before you modulate it with information–we don't have ideal lasers. I've shown before that the laser will have an inherent width, which we call delta omega of the laser. And now, we use that laser and put information on it.
The information also causes broadening. Remember, we've shown that the process of modulating the laser is to broaden the spectrum a little bit. And now, you have the actual bandwidth of the laser, half a megahertz commercial, the one that we brought down, and the line width, due to the music occupying the same spectrum, you cannot separate them, limits how much information it can carry. If somebody next to you is shouting, a drunkard, then people will not be able to understand you very well.
ZIERLER: Given these capabilities, did this outpace all of the dot-com companies and what they wanted to do? What was the timing?
YARIV: This work took place at Caltech. You probably will not grasp everything I said today. There's too much to follow. That's basically the contents of a semester course in communication theory, and modulation, and all of that. Exciting topic. When I came to Caltech, I wrote within the first seven, eight years two books. One, on the quantum mechanics of the laser, the other one, how to apply it. Modulation, bandwidth, all of that. We had all of that down. We did it not because there was any great pressure, but because we knew the laser needed to be purer and purer. And why settle? It turned out that the laser design still, most of the commercial lasers you'll buy, uses that wrong resonator, where energy is concentrated in lossy material. That's a no-no.
You look back now, and you wonder why we've tolerated it so long. Well, nobody did the actual basic until we did the simple analysis. We'll give you preprints. They're universal. They don't apply to our laser, but any laser. The first thing you should do is minimize losses. Any time you have losses, you also have noise. I think there's a theorem in thermodynamics or statistical mechanics, dissipation fluctuation theorem. It says that any time you have dissipation, losses, you have fluctuations, which is noise. The two go together. Our lasers now have losses reduced by over effect of 10,000, and in the future, probably even higher. It probably will not be done by us. And that will translate directly to the ability to carry more information. Because information takes a pure sine wave and makes it less pure. The nature of carrying information. So does noise. Once the two intermingle, there's no way to separate them. We want a laser to begin with that has less noise.
It turns out, for instance, that now only does industry wake up to the need for these very pure lasers. As a matter of fact, Intel, which bought Telaris two or three years ago, insisted on buying the Caltech patents that were generated as a result of our work. And in their case, a purer laser, a laser that has an inherent narrow line width, will enable them to see farther on the freeway. Because your ability to see a longer distance always depends on how pure the signal that comes back is, and any noise limits your range. And that's key. Or ability to discriminate against other cars on the highway. The low-noise laser, the pure laser, will be a key element in the next generation of optoelectronics and optics for self-driven automobiles. The difference between the ability to determine where you are within a foot, an inch, a quarter of an inch. It doesn't sound important when you're driving home, but the other applications, it is important. If you want to navigate to a far enough planet, it might make the difference whether you miss it by a mile or by a million miles.
ZIERLER: It'll come in handy when we first meet the aliens.
YARIV: Yes. [Laugh]
ZIERLER: We'll see. Totally different topic. What was it like winning the National Medal of Science and going to the White House? First of all, who gave you this information? Who called you?
YARIV: Oh, I got a call one day by the Science Advisor.
ZIERLER: John Holdren.
YARIV: He said that I was a serious candidate. They probably already knew and wanted to make sure I would come. I said I would come but on one condition. [Laugh] He asked me what. I said, "Obama and I share a hobby. We are both body surfers. We both body surf in Hawaii." That was known. "And so, I would like an autographed photograph showing Obama body surfing." He said, "No problem." Fast forward another half a year or so during the ceremony, Holdren comes over, introduces himself, we shake hands. He says, "I'm sorry, I won't be able to give you the autographed picture of Obama body surfing." I said, "Why not?" What had happened was–that was maybe November–in April of that year, the LA Times had a full-page color picture of Obama body surfing. And you can tell from the picture that he was a good body surfer. There's a technique out on a wave, lifting yourself. And that appeared in the LA Times and caused a whole bunch of negative reaction, mostly among Republicans, that, "Our soldiers are dying in Afghanistan, and our president is frolicking in the surf." It's OK to play golf, mind you, but not to surf. They decided not to get those pictures out anymore, so I couldn't get one.
ZIERLER: That's too bad.
YARIV: I said, "Well, you know what? I'll get even with it." I was going to give him a picture of me body surfing at Hawaii. [Laugh] And I didn't give it to him. [Laugh]
ZIERLER: What was it like meeting the president? Did you schmooze with him? Did you talk science?
YARIV: No. First, he met all of us in one room. And yes, as I shook his hand, he said, "Nice meeting you. I appreciate your work." That lasted 30 seconds. And then, I had maybe two or three minutes with him afterwards in the meeting reception. Probably no more than a few minutes. And we agreed to go body surfing together. [Laugh] In Shipwreck. It's a beach in Hawaii, very good for body surfing. I never tried, I knew he would be too busy. [Laugh]
ZIERLER: What do you mean he's too busy? You're the one who's not retired. He's retired.
YARIV: He and I will never be retired. [Laugh]
ZIERLER: Right. [Laugh]
YARIV: But it was a lot of fun. He's very personable, friendly. Somebody you would like to play basketball or body surf with. [Laugh]
ZIERLER: Is your sense that this award was like a lifetime achievement award, or was it recognizing a specific aspect of your work?
YARIV: The citation mentions specifically the distributed feedback laser. I think it talks about an award for foundational contributions to laser theory and optics, and specifically the invention and development of the distributed feedback laser, which is used in optical fiber communication. Something like that. It was both.
ZIERLER: You've won a lot of awards. Where does that one rank in terms of being most special to you?
YARIV: It's the only one I have on the wall at home. People know I have won awards. They take wall space. [Laugh] But I have that one at home. But in my study, not in the entrance hallway.
ZIERLER: Coming closer to the present, when you're starting to just think about maybe retiring at some point, maybe at 120, you'll retire, how is that end game affecting the research questions or agenda, the kinds of things where you can say, "Here's what I can accomplish in one year, five years, ten years"?
YARIV: I think to do the kind of research I have done over the years, I probably required a group of people working with me, which were mostly students and post-docs. That's the way I work. Most of the things we did, we were involved in the original idea. It wasn't always born here, but we usually were at the early stage. Many of them were born here with our group. And we did essentially the basic theory relevant to a given project and the demonstration. For some reason, that was very important to me, to do both. I think the importance of doing it has proven itself through the years. It's nice to do something, it's five times nicer to come up with a theory and a demonstration. As in my recent shower experiment and theory. The satisfaction of explaining something is immense. It's clear now that I cannot put in the effort required to run both an experimental and theoretical group. I have essentially wound down my experimental to almost nothing, and in a year from now, I will have probably given back all the space. I'm not looking for new money anymore.
ZIERLER: Because the experimental work is physically demanding?
YARIV: I don't do it myself. The students did the work. I'm involved in it intellectually. And getting the money has always been a negative aspect, but maybe not. But time-consuming and sometimes unpleasant. Trying to convince people in government of the importance, you have to educate them, and they don't always understand what you're talking about. Some do, some don't.
ZIERLER: But because of the applications in technology, why would you ever need to go to the government if Netflix, Microsoft, Amazon are interested in what you're doing?
YARIV: No, they're not. Netflix, Amazon, and so on, with few exceptions, and the exceptions are recent, were not developing technology. Apple developed the iPhone, but it's essentially software. They didn't spend money on making smaller and better transistors. Which is the key for making our iPhone. They assume that that will be done by other people, and they'll buy the best product. And that's still where they are today.
ZIERLER: So there's no analogue of Bell Labs today, there's no place where there's industrial research that's really focused…
YARIV: What made it possible for Bell Labs is the fact that it was a monopoly. Research didn't cost them anything. Bell Labs was not always the first in. They were among the last to understand that the transistor was important for switching. They hung onto the mechanical switches until the very end. They wanted to amortize the investment. So no, industry is not–especially today, where industry has been moving more toward–take the latest example in the chip shortage. The electronic chip, which is the key to computation, data transmission, everything you want to do. And yet, we, today, don't have the best technology. Holland and Taiwan do. We're trying to change it, but it may not happen. Intel no longer makes the best electronics. It's a fact. And that's a reflection of the fact that Amazon and Facebook, as a matter of principle or a business decision, spend most of their money on programs, on networking, and then get the best technology available. It's only now, the next generation, or maybe the one after that, of Apple iPhones will have their own circuits made in Taiwan. They are not investing in making that. That's a fact.
ZIERLER: And this gets back to your answer why government support of the research is always primary.
YARIV: Absolutely. Bell Labs was the fount of much of the important ideas. Bell Labs isn't there. The other researchers, IBM, GE are not in the picture. They're too small and not don't even have the talent to do those things. We depend today for the new generation of hardware on universities. And I'd say, in our field, Santa Barbara and Caltech are the leaders. Schools like MIT, Stanford, Berkeley are the second rank of pushing optics forward. You may not want to say all of that, but between us.
ZIERLER: We talked in the very first discussion about what your current work is. Now that we've worked right up to the question, some big retrospective questions to wrap it all up. The research that you've done, where you've attained the satisfaction of coming up with the theory and demonstrating or applying it, just in a general chapter-heading format, what are those different areas of research where you've both done the theoretical work and seen it to application? If we could list them chronologically, where would we start?
YARIV: The laser. We are responsible for not all but a major, sometimes the most important, part of the theoretical development. For instance, the development of the semiconductor distributed feedback laser. A step further, the demonstration of the need for optical waveguiding as for the new generation of integrated optoelectronic circuits. Or for the laser itself. The semiconductor laser would not have worked without guiding. That understanding, which seems simple at the time, is key. Everything we are talking about is done with waveguiding. The fiber is a waveguide. Has a dense, high-index core and a low-index cladding. Same principle we discussed, except it's in a fiber. To go back, in the laser, I think we were the first ones to claim you must have waveguiding, to document it. And then, eventually, going forward, making the distributed feedback laser. The idea of distributed feedback was not ours. Realizing it in a semiconductor laser by means of wavy interfaces was our idea. And that was the key to making it.
Both theory and experiment came from here. Later on, moving forward to what we talked about here, the recipe for making the next generation of extremely high-coherence laser is ours. How to do it and the demonstration. In the meantime, we also worked in parallel in another area altogether, and we didn't speak much about it, and that's phase conjugate optics. It doesn't have the same commercial impact as a semiconductor. The semiconductor is one of the lynchpins, along with fiber, of optical communication. But intellectually, it was and is very, very interesting. Here, we were major contributors to the theory and demonstration. We mentioned the high-coherence laser, the recent work still going on, but essentially over. The shower experiment. [Laugh]
A Eureka Moment and Never Stopping
ZIERLER: The shower experiment. We'll have to wait and see what the applications of this are. Any ideas? Where might we see this research?
YARIV: Well, if you asked Charles Townes, the inventor of the laser, "Will the laser be good for optical communication?" he very likely would've said, "I don't know. I don't see how. Maybe." If he were cautious. I would say I think the main application of the ideas generated here is that I don't think God chose me like Moses in the shower to demonstrate this. That phenomenon exists as we speak in other contexts, locales. Like an old building in a hurricane. [Laugh] Subject to, instead of water pressure, wind pressure. The building can twist, can creak, can move, and it's completely possible that under the right circumstances, two of those modes of the building, let's say twisting, can get together, and that [affects] the energy. Remember, we now understand the phenomenon can be described by the spectrum.
Cause the building to amplify, convert wave pressure, which is uniform in time, into a pure sine wave, which would bring the building down. It's like when soldiers march over a beach fall out of step. When they walk in step, it's like a pure sine wave. It repeats itself. And if you find a resonance and excite it at the right frequency, you can cause large amplitude oscillation will bring it down. I think they are structural, meaning where the source of energy is wind, water, earthquake. It's a new phenomenon. New like discovering America. Columbus did not discover America. He found it.
ZIERLER: A new phenomenon is going to require a new name. Any contenders?
YARIV: The paper will be called something like Eureka in the Shower/A Class of Volume-Modeled Parametric Instabilities. I think Lord Rayleigh, who first came up with the principle of parametric excitation called it on a class of vibration or something like that. Here's one mode, we now have two modes. And I'm beginning to wake up at night and formulate the language, how to describe it. One idea was to start by today, kind of link, show the phenomenon. There I am in the shower, bare feet, turning on the water. There's a shower head hanging down. Show it. Show the threshold. And what happens when you exceed the water flow rate exceeds the threshold, [it] goes into the vibration. And then, go back to Lord Rayleigh, go briefly through his mathematics, which is very simple, and the class of–oscillations can be either like a mother pushing a child, you push it at the frequency of oscillation, or the boy's older, he stands up and can pump himself.
Doesn't need a mother, it's parametric. But he does actually modulate when he does that. He modulates the earth gravity. By lifting himself ever so slightly as far as the seat, the seat thinks that he's lighter because he's not pulling himself up, he's modulating the gravitational constant. Or he is modulating mathematically the mass of the earth. It would be the same equation. That's where the word parametric came from. You modulate the parameter. The gravity, mass of the earth. And that [is] a class of phenomena. But that requires that you modulate at twice the frequency, the parametric. The boy pumping himself on a swing does modulate up and down at twice the rate that the mother would do, pushing the child. In my case, that requirement is thrown away. The source of energy is a constant, time in varying water pressure, as an example. But it requires two modes, so it's a new class. Mathematics are more advanced and complicated than Lord Rayleigh. Also, more elegant. But Lord Rayleigh is the genius who opened up the door. It's a step forward.
ZIERLER: In all of the successes and satisfactions you've achieved in connecting the theory to the demonstration, are there any failures in there? Any times where you had the theory but just couldn't get it to application?
YARIV: Oh, there were many, many failures over the years. You can't avoid it. We don't write papers about the failures, usually. That's where we retract something. [Laugh] We've been very careful, yes. We bury the failures. You don't talk about them. If somebody invited me, I would. But it's the nature of research. It's mostly failure.
ZIERLER: But are the failures useful? Do they teach you things that success does not?
YARIV: Mostly, humility. [Laugh] And to be very careful in claiming victory. There are so many pitfalls.
ZIERLER: The gamble you took when you joined the Caltech faculty so many years ago, what is most obvious in the payoff from that decision? What have you been able to accomplish here that might not have been the case elsewhere?
YARIV: I ask myself the same question, and what you call success in life is often chance. Accidents. You have to be prepared to take advantage of those. I remember one movie where a young man enters a main headquarters building in Manhattan, about to enter the elevator to go up to his office, and in the elevator, as the door opened, there's a very beautiful woman. He looks at her, she looks at him, and there's maybe a little rapport, and the door closes. He rushes, doesn't make it. The movie is, what about the parallel story? If the door had closed a second later, he would've gone into it. And I think the question it asks is something like that. My thinking is, if I had wound up at Stanford or MIT as an example, the chances are it certainly would not be this story, but I would maybe have done something that would've been OK. But I would've never known. But sidestepping slightly, I think Caltech was a good choice.
The structure of Caltech, the fact that it's very informal–I can pick up the phone now and talk to our president if I have a problem. Or go tonight and meet him at a candle-lighting. The ability of students to move freely without the need for any agreements by professors or filling out forms is a key. And of course, the very, very good students that we get here. But Stanford and MIT probably get equally good students on the average. Although, I said at some point, the reputation of our work here brought students that specifically came to work with me. And that was key. I have maybe ten students who were just as good as you can get. And they were key. I, myself, at some point, stopped doing experiments, and I was never a good experimenter, even when I did experiments. I like Los Angeles, I like the mountains, I like the ocean. It's the nearest to Israel that I could find. It was a good choice.
ZIERLER: On that point with your students, at a very broad level, of all the students you've interacted with, in what ways are you teaching them, and in what ways are they teaching you?
YARIV: A speech I give to all my students, not a ritual, but de facto at some point, after a year, if you join my group and spend maybe the first year learning the field, what's going on, reading, you have to teach me. You have to be the best-informed person in the world on that narrow subject. Because you'll do nothing else but that. And I do other things. Besides, you are probably a better mathematician than I am. And then, of course, you will learn. I think I was able until the very end to come up with most, but not all, of the ideas. They started with me in some way. Because I had a more general background than most of my students in physics, engineering, communication, surfing, waves.
ZIERLER: Quantum mechanics.
YARIV: Yeah, physics. I would've never done any of the things I've done without students, even if they were theoretical. Phase conjugate optics arose–I taught a class, the class that I started at Caltech, on the optics of lasers, optical fibers. In an optical fiber, simply make it bigger. Today, an optical fiber has an inner core of maybe 100 microns, like a hair. But if you make it bigger, 400 microns, it can propagate lots of modes. A thin fiber, the light is like that. If this instance, call it T, is small, only one mode can propagate. That's what you want. If you make T bigger, then there are higher-order modes. Optical communication wants a pure tone and a single mode. If the mode is not single, then less data. Because every Fourier component will propagate at a different velocity. Pulses will spread, the ones and zeroes will emerge. Fiber is big, you can have many of them, hundreds of them, depending on how big it is. Optical communication makes it ten.
The question was, if there's a tree outside in a garden, and a window, and you look at the tree, then the pictorial information has traveled from the tree. Light from the sun falls on the tree, it's reflected, gets into your retina, image. That's how you see the world. Going back to the tree, the pictorial information traveled from the tree through the window into your eye. Now, take the window, and begin to shrink its transverse dimension. Make it smaller, and smaller, and smaller. Eventually, you have a fiber. Are we able to see the tree looking at one end of the fiber? How would the pictorial information travel now? When you think about the fiber, it's just a limiting case of a window. That's a deep fundamental question. It turns out that to carry the pictorial information of the tree, you need a fiber of many modes.
Each mode can carry a pixel, essentially. And a rich picture has many pixels. You need many modes. But most propagate at a different velocity. And therefore, the picture will get smeared. If you look at it after a few meters, you will see it looks like noise. But it's not noise because what happened here is reversible. It's predictable. Could be a perfect fiber mode. The idea of sending pictures in multimode fibers–I taught light propagation in fiber as part of a class. Important. And then, that question, I gave it to the class. I said, "Here's what happens. The picture will be smeared because of group velocity dispersion. In other words, the different pixels, now carried by different modes, will get out of step. But we know how they get out of step, and we can reverse it. It's like playing a movie backwards.
I first gave it to the class and said, "All we need to do is follow the recipe. Separate the picture, be able to take each mode, reverse its phrase, and put them back together, and you will get the picture back. Try and think of ways of doing it." Obviously, it's a nonlinear process. Well, they didn't. [Laugh] I came up with a solution, which was OK, but not practical. My colleague, Bob Hellwarth at USC came up with a solution that we are using to this day. Both using nonlinearity. He went an order higher. [Laugh] Meaning third-order nonlinearity. I stopped at second. Anyhow, that would not have happened if I hadn't taught the class. Working with other people, especially smart people, is a prerequisite for creativity, I think. Very few people like Einstein do it in an office in Basel, working for the Swiss–well, we know he spoke to colleagues like Schwarzschild, Minkowski, and others.
Don't quote me, but probably the smartest of all my students was a fellow named Tony Kewitsch. He and I cofounded a company called Telescent. The company will probably be an important company. It makes robotic cross-connects for optical communication. Tony can do anything under the sun. He can do quantum mechanics at the highest level if he wants to, but he's an engineer. That's why Tony did not go to university, although he was probably my number-one student. But in coming up with the solution–the problem with cross-connect switching, today, with so many fibers entering, needing to be connected and reconnected, especially in data centers, it's a big mess. Engineers dive into this tangle of fibers, finding what's connected to what, and he has done it all with robotic, computer-controlled switching, and developed it theoretically, the mathematics. Probably the single most important criteria for my work at Caltech is the good graduate students.
But good grad students would not come here if the school didn't have a prestige value to attract them, good programs to attract them, and good facilities and support. And Caltech provided that ambience. And very few places can do that. My guess is that the top two or three universities I mentioned–the amazing thing is John Barrows at Santa Barbara, I would say, is probably the most dynamic–and Caltech. Kerry Vahala's doing a very good job. The two of them are the main contributors to the new generation of technology.
ZIERLER: As you well know, there's the satisfaction in discovery, the basic science. There's the satisfaction in the applications, putting it to some real-world use. And there's the satisfaction of success in business, of selling products, seeing them adopted. How do you compare these satisfactions?
YARIV: I never try to compare them. I enjoy all of them. But I'd say if I had to give up one of them, it would probably be the business part. I was talking about my shower experiment. Very exciting because I think I've discovered something new. The discovery is, to me, personally, more exciting. But it's also very exciting, of course, to demonstrate an idea. The three go together. To me, doing experiments about theory or theory about experiments is not very satisfactory. Business, less so, but still very satisfying. The ability to be financially independent is also very, very important. The lady who just called me is my wife's nurse, and the ability to keep my wife at home–and she's functioning but has a memory span of maybe a few minutes. She needs help. The ability to provide the help and keep her in a comfortable home, perfectly happy, I could not have done it, for instance, if I weren't financially independent. It's expensive. Anyhow, Caltech was a good place to be. And I love Pasadena. Wonderful mountains here. Too bad you weren't here 20 years earlier, I would've taken you to a very exciting place, a little climbing. Not today. [Laugh]
ZIERLER: Finally, looking to the future, just start at the broad scale. What are some of the developments on the horizon that are going to be real game-changers, just like 50 years ago, the things you were involved with and what they did?
YARIV: I think integrated optoelectronics. That hasn't happened yet. People are working on it. Actually, it's getting very, very close. My consulting at Intel involves actually–between us, I think Intel will dominate electronics for self-driven automobiles, mostly by virtue of having acquired us. And the main contributions are not mine. My ex-students, working with Israel, Mobileye, will restore their glory days, which they have lost. Papers are full of articles about companies called AMD and Nvidia eating Intel's lunch. It's been relegated to number two or three.
ZIERLER: The focus is on self-driving cars. Where else might we see this technology applied?
YARIV: Oh, everywhere you do optical communication or use lasers. Lasers by themselves just generate light. You need to manipulate it, put information in, retrieve it, compute with it. Quantum computing will probably be one place. It's the main means of communication. And communication is becoming the area of human endeavor that's getting the big investment today. You can only drive so many cars, have so many homes. But there seems to be no limit to how much we want to communicate. I think communication will use for sure integrated optoelectronic technologies with both the optical components, the control, the modulated detectors, and the computation on single crystals. Optical and electronic components embedded in each other, connected by waveguides. And I am a cofounder of a company, Telescent focused on next-generation networking.
ZIERLER: And to get there, what are the breakthroughs that are needed? Are they theoretical? Are they technological?
YARIV: I think they're technological now. I think the idea that you can do it is something I did 1970s, early 80s. But it took all those years to develop the technology to get there. The materials technology and so on. Things take a long time.
ZIERLER: And they happen gradually.
YARIV: Yes. Also, industry wasn't–there wasn't enough money invested in it. There's only one commercial company today, called Infinera, that actually makes mostly products for optical communication using everything on one crystal. But Intel is already doing it and not advertising it. [Laugh] Because to make the control, the circuitry and the devices needed for self-driven cars, it might take a trunk full of equipment. And the idea is to shrink it down to a cubic centimeter. It will be done.
ZIERLER: Finally, last question, it's not so far off, it's amazing to think, on your 100th birthday, what do you want to be doing? What would that day look like for you?
YARIV: Body surfing. [Laugh] Or climbing up a mountain.
ZIERLER: Now, that's a picture you can send to Obama. You at 100, body surfing. That would be pretty great. You think you'll still be coming into the office? Would you want to?
YARIV: Actually, due to COVID, I found that I came less, and it was a productive year. I probably would come less. I can see my deterioration physically. I gave up tennis 20 years ago. Body surfing, probably ten years ago on the big waves. Things change.
ZIERLER: But being intellectually engaged, being involved, that's certainly a secret. That's one of the explanations of how well you're doing at age 91.
YARIV: Oh, absolutely.
ZIERLER: Genetics, of course.
YARIV: Genetics, exercise. It's very, very important. And intellectual involvement, being involved.
ZIERLER: Amnon, thank you so much for spending all of this time with me. It's been so much fun. It's been a great pleasure learning about everything in your own voice, and I'm so excited for what we do with this next, so thank you so much.
[End]
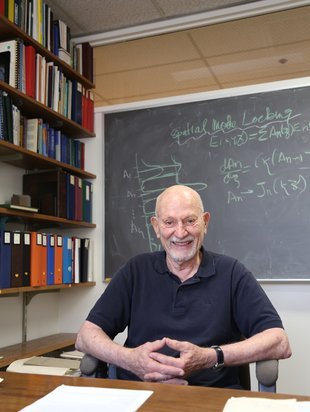
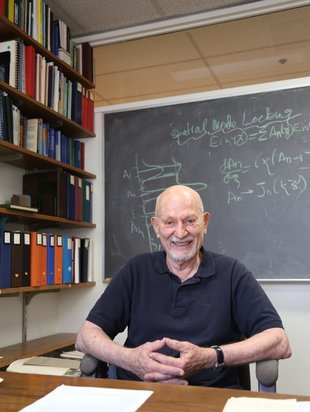
Interview Highlights
- Defining Optoelectronics
- The Centrality of Roy Gould
- The Maser Revolution
- Joining Caltech
- DARPA and Semiconductor Lasers
- Communications Potential in Optics
- Science and Shower Thoughts
- Bell Labs and Returning to Berkeley
- Bell Labs as Temple of Basic Science
- Laser Breakthrough
- Partnership with Intel
- The Road to Autonomous Driving
- Staying Connected to Israel
- The Origins of Quantum Electronics
- Dialing in Coherence for Lasers
- Bringing Lasers to Business
- Neighbors with Feynman
- The Wonders of Gallium Arsenide
- Remaking the Cable Industry
- Recollections on the Founding of Israel
- Nationalizing Fiber Optic Technology
- No End in Laser Applications
- The Corning Connection
- Lasers Make the Modern Internet
- A Eureka Moment and Never Stopping