Barbara Imperiali, Chemical Biologist, Research Leader in Protein Modification, and Builder of Chemical Tools
The creation and flourishing of chemical biology as a new and discrete field rests on two overlapping premises: the study of biological functions offers some of the most impactful and wide-ranging pursuits of knowledge in science, and the chemist's capacity to synthesize molecules expands these possibilities beyond anything possible in traditional biology. As in so many fields, Caltech played a leading role in the development of this new discipline over the past four decades, and Barbara Imperiali, who launched her career at Caltech, has a unique perspective to offer this narrative.
In the discussion below, Imperiali recounts her undergraduate education in medicinal chemistry in London and the opportunity that brought her to organic chemistry as a graduate student at MIT. She reflects on the challenging mentorship of Sat Masamune and Bob Abeles, and navigating the "two body problem" as a young academic. After a false start at Carnegie Mellon, Imperiali recalls with great fondness joining the Caltech faculty, where colleagues such as Dennis Dougherty were pioneering methods in chemical biology and making profound discoveries along the way. In her return to MIT as a professor, Imperiali remarks on her steady path toward studying protein structure and glycoconjugates, and she muses that working at the interface of two disciplines means that colleagues don't know exactly how to place her work: is she a chemist who does biology, or a biologist who does chemistry? And of course, the answer to that questions is, yes!
More recently, Imperiali has embarked on a startup venture to develop chemical sensing tools with applications across both translational and fundamental research environments. A passionate mentor and educator, Imperiali conveys her love of explaining science to curious and dedicated students, and she provides generational perspective on the pressures that young academics face today, compared with her own experiences. In the last part of the discussion, Imperiali takes stock of all the discovery to which she has contributed and witnessed, and she notes a related challenge: how does one synthesize and systematize accumulated knowledge? The question is academic in the best possible sense, and the answer requires not just scientific expertise, but historical perspective.
Interview Transcript
DAVID ZIERLER: This is David Zierler, Director of the Caltech Heritage Project. It is Thursday, March 7, 2024. It is my great privilege to be here with Professor Barbara Imperiali. Barbara, it is wonderful to be with you. Thank you for joining me today.
BARBARA IMPERIALI: You're welcome. I'm glad to be here.
ZIERLER: To start, would you please tell me your titles and institutional affiliations?
IMPERIALI: My current title, and I can explain it because it sounds odd to people, I am the Class of 1922 Professor of Chemistry and Professor of Biology at MIT in Cambridge, Massachusetts. I always get the same corny joke where people say, "You don't look that old," and I say, "Well, it must be good, clean living or something." [Laugh] But anyway, it was a fund put together by the MIT Class of 1922.
ZIERLER: Are you the inaugural holder of that chair?
IMPERIALI: No, it's been around a while. The graduating class of 1922 raised the funds to endow the chair. Until a few years ago I would write brief notes to the surviving member of the Class of 1922 telling him what was going on. He was in a retirement home at the time. I actually moved to MIT from Caltech as the Ellen Swallow Richards Chair, and I'm happy to tell you about that later on.
ZIERLER: Is it a dual appointment? Are you a professor of biology and a professor of chemistry?
IMPERIALI: Yes, that's right. which is a nice thing for me. They are full appointments in both departments. When I first moved to MIT, I was Professor of Chemistry. And as my work continued to evolve, and I had more interactions with the Department of Biology than Chemistry sometimes, and biology offered me a second appointment where my appointment would be secondary in biology and primary in chemistry. And then, about a dozen years ago, I shifted to primary in biology, secondary in chemistry, so they're both real appointments, they're not adjunct.
ZIERLER: And this means that there are two department chairs to report to, two faculty meetings to go to. It's double everything for you.
IMPERIALI: Well, it felt like it was at the beginning until it seemed to make more sense to focus on the primary appointments. Right now, I report to the biology chair, Amy Keating, and I teach in Biology, and I continue to teach a joint class in upper-level class in Chemical Biology with my colleague, Ron Raines, in Chemistry, so that's good. I stopped teaching first semester organic chemistry when I moved to biology. That wouldn't have made any sense. And since my move to the biology department, I have taught general biology and principles of chemical biology. My teaching actually is actually spread between the departments, but you can only go to so many meetings. [Laugh]
A Chemist Who Does Biology
ZIERLER: In the way that you have shifted your primary appointment, I wonder how much of a stand-in that is for how your research agenda has changed. In other words, perhaps earlier in your career, you might've called yourself a chemist who did biology. Are you now a biologist who does chemistry?
IMPERIALI: You know what, I'm still a chemist who does biology [Laugh] It's funny, though, right now, because to the chemists, I seem like I'm a biologist. I'm in a different building. Wow, it's scary, it's the bio building. They must have mice in their attics and in the basements. And to the biologists, I'm the go-to chemist. Like, "I need to run this reaction. Can you help me?" I've always followed my own mission of applying as much chemistry as I can into studying biology, but I still really think like a chemist. The molecules are in my head, the interactions are in my head. But I've gained so much more being resident in biology; I have a richer feel for the sorts of problems to tackle, and a better grasp of the new technologies for the study of different biological processes. Also, I can see where new technology might be needed that could be solved by chemical biology approaches. These are what I feel I've gained from my transition to a primary appointment in the Biology Department. The way I think about the Chem/Bio interface is that - I was trained as a chemist, so I come from that background and often I tell people, "You can't go uphill. What does that mean to me? Well, one can go from quantitative and molecular to a little less quantitative and less molecular, but you can't go uphill. [Laugh] It's tough to say, 'I'll study mice and flies for now then soon, I'm going to think about doing synthesis and organic chemistry.'" So, you can roll down the hill a bit from chemistry to biology although you probably won't look very smart for a while. But that's okay, I don't really care about that.
ZIERLER: Thinking about chemistry and biology, I'd love to gain your perspective on chemical biology as a discrete field, and particularly perhaps the influence of people like Dennis Dougherty in your thinking about these things.
IMPERIALI: Oh, yes, of course. It is so strange, this field is something that just, at a certain stage, wouldn't go away. But, when I was a graduate student, you could be either an organic chemist or a biochemist, you couldn't be a chemical biologist. I chose to patch together my training in the synthetic organic lab of Satoru Masamune at MIT and then the biochemistry lab of Bob Abeles at Brandeis. I evolved across those borders to something in the middle that would've been called chemical biology now, but just didn't exist at the time. Then, when it did start to emerge, we (the community) started to call the field bio-organic chemistry. And what was that? Well, at the time it was mostly dominated by organic chemists developing simpler model systems that mimicked biology. It wasn't what it is now. It continued and continued to evolve. There was a lot of molecular recognition work and a lot of work on model system. But I think there emerged a subset of organic chemists who were pretty fearless, including Dennis, who is one of my best friends from Caltech, where colleagues just weren't afraid of trying to leap over the Chem/Bio border and bring chemical tools and thinking along to study, solve, and bring in new hypotheses into the biology realm.
Early in my career I did something, which I only learned much later on, [Laugh] that my colleagues at Caltech thought was completely crazy. Quite soon after I moved to Caltech–I had been three years at Carnegie Mellon, but for a variety of personal and professional reasons, I needed to start my independent career again (most fortunately!) at Caltech. At the time I was being invited to Gordon Conferences, and one of them was called the Natural Products Chemistry Gordon Conference, there were others all deeply entrenched in organic chemistry, with names like Heterocycles and Stereochemistry. But at every one of those meetings, the organizers would try to broaden the "feel" of the conference by bringing in a few speakers who were more biological, like me, so biological and bio-organic chemists. And at the Gordon Conference I went to, a group of us got together and said, "We're fed up of being add-ons to other people's meetings, let's change this!"
A group of us, Glenn Prestwich, Craig Townsend, and I, said, "Let us start a Gordon Conference in bio-organic chemistry"–by the way, that term still holds, but it's morphed into chemical biology–". We felt that the field should define itself and we wanted to make the case to the Gordon Research Conferences that, 'You need this meeting because the field evolves and evolves, and the people who are involved in it need to talk to each other and need to evolve together and understand the possibilities and the far horizons. We need places for young people to go to meet the people in their field, not be add-ons to a very organic-centric meeting, but rather have their own meeting.'" So, we applied. It was a lot of work, and as a second-year assistant professor at Caltech, I suddenly found myself co-chairing the meeting, [Laugh] which at that time was way beyond my pay grade. But I did it because I really believed we needed it.
We got permission to run the new GRC the meeting in 1992, and I will tell you, the thing that was so exciting to me is that I just sat down and wrote my dream list of bio-organic chemists, who we would now all call chemical biologists. I said, "I'm just going to invite all these people." And I let Craig Townsend to do fundraising because I didn't want to do that. And everyone I invited agreed to talk. These were people like Peter Dervan, Ron Breslow, Koji Nakanishi and Albert Eschenmoser just as examples - some of the greats of bio-organic chemistry. And what was even more amazing was that a huge number of the young attendees became famous chemical biologists themselves. Over the years, the "bio-organic" name morphed to chemical biology, but the "chemical biology" name was nowhere near as broad as it is now. It was much more focused on things like screening chemical libraries and diversity-oriented synthesis, such as the initiatives that Stuart Schreiber pioneered. But I think, what happened is, that term got broader and became all-encompassing. And what belongs in chemical biology is simply defined by the community.
Each year, I challenge the students in my class to give me their best definition of what chemical biology is. And they all come up with ideas, and they'll often come up with parts of the field. My best definition is that the field is all about using chemical and physical tools to study biology in as much context as we can as opposed to as much of the model systems. Chemical biology, I've taught it for years and years, and I've morphed it and morphed the content. But I think there are just some amazing paradigms that one can still teach, even if they were introduced 15-20 years ago. You can still teach them because they are exemplary paradigms. What did people have to know to be able to do this chemical biology? And what can they learn that they couldn't have learned before? I make a lot about Dennis Dougherty's work in unnatural amino acid mutagenesis and receptor function, because I just love it. Because this represent a paradigm and a paradigm shift? He came from hardcore physical organic chemistry, and all of a sudden, or so it seemed, he was doing physical organic chemistry on mega-Dalton-sized receptors, in the brain. He's a personal hero of mine, and the other thing is every time I hear him talk, he just makes me laugh so much and love his contributions all over again.
ZIERLER: There was a fearlessness in his new pivot.
IMPERIALI: Indeed. I think that was very impactful for me to see that from him, even though he was very modest about the whole thing. [Laugh]
The Role of Theory and Bioinformatics
ZIERLER: I want to move to some overall questions about your research. First, the Imperiali Lab, is it a strictly experimental operation? Are there theoretical aspects to your work?
IMPERIALI: Well, we do things that are not completely experimental. We've dipped into aspects of bioinformatics and computation, and also biophysical that are more quantitative and mathematical than a lot of the other things that we do. All that was made possible by having really smart students at Caltech, and later MIT, that were able to take my ideas and convert them into reality in ways I could not have done myself. My group continues to cover a broad spectrum of methods. Over the last decade, I would say, the power of bioinformatics and the abilities to predict protein folds and decipher the functions of proteins has really been absorbed into our group. Nowadays, there's so much one can do now through that kind of comparative chemical biology across millions of sequences. It's been irresistible to incorporate that into what we do. I will say that we initially integrated a lot of the bioinformatics through my longstanding collaborations with Karen Allen, a dear friend of from our days the the Abeles group at Brandeis who's now the chairperson at Boston University. After a while, my group started applying the informatics methods at MIT – we had learned from the best. Those types of approaches are really critical now.
We have, over the years, also done integrated computational simulations. We've most recently needed this because we wanted to compute the shapes and dynamics of proteins in lipid bilayers. For this we collaborated with the Straub group and the Allen group at BU. It was a great partnership. I think when you do the computational modeling and the bioinformatics, you need to get a clear-eyed view of the questions. Because if you don't have good questions, you can end up barking up the wrong tree and think that great insight will emerge. I'm not a fan of applying methods for the sake of it - I'm a fan of going in and saying, "I have this data. Could you predict it by theory or bioinformatics? And if so, can you then help me develop a model to predict new things that might occur?" There should always be the dialog between experiment and theory.
ZIERLER: Your embrace of bioinformatics and computational biology in more recent years, has that gotten you into machine learning and analyzing the data beyond human minds?
IMPERIALI: I think once you've got a ton of data, you're going to use machine learning. And it depends on whether you're using it to figure out what laundry soap people buy or to figure out what amino acids determine specificity. Yes, you could call it machine learning because it's using mountains of data that we can't assimilate manually. So, we need to put that data into algorithms and have it assimilated for us. But once again, it's really so important to understand how you're looking at the output. And what's in your input that might vary the output? And what parameters do you need to set? It's so important, even in those big, mega projects, that you keep yourself, for me personally, grounded in reality.
Startups and Sensing Technology
ZIERLER: Of course, your lab is a fundamental research operation, but I wonder, being in Cambridge, being around all of the biotechnology, being right next to Harvard, have you gotten involved in translational biology to some degree?
IMPERIALI: Well, I have a small startup company (AssayQuant Technologies) that is located outside the greater Massachusetts Highway 495 ring. The company is founded on applying the sensing technology that started to think about at Caltech and then put into practice at MIT. Specifically, in the initial phases, AQT has been focusing on protein kinase sensing, protein phosphatase sensing. We have about 20 coworkers at AQT, we have products, we have sales, and we're now in that startup hell, where you think it looks great one day, and the next day, the market took a downturn, and it looks a little worse. [Laugh] But I think we're on track. I cofounded the company in 2015, and we've grown up to this size to reasonable revenues, and we hope to be revenue-positive soon.
I think the whole company idea, was really because of the biotech hub that I now live in. But as much as that, I think a lot of the influence on me when I started really realizing the technology that I had developed could be useful to pharma and biotech, were my interactions with colleagues in bioengineering at MIT, especially Linda Griffith and Doug Lauffenburger. Because when others seen the work in a fresh light and, "Oh, someone needs to develop that more." I gained confidence from that. Probably at Caltech, it would've been comparable, within Chemistry and Chemical Engineering because those sorts of people would've been more embedded together at Caltech. But at Caltech, I was more focused on the earlier goals of my career. I definitely reached out to colleagues in biology and in chem E, but to be honest, I think you get the benefit from doing that more when you're more established, and you're bringing some different things to the table.
ZIERLER: What are the kinds of ailments or diseases for which this research might ultimately be relevant?
IMPERIALI: The sensors that we have developed are for biotech and pharma companies, as well as academic and government labs where quantitative analysis of the functions of critical enzymes in cell regulation and signaling are needed. The big headline is always oncology because the enzymes we are able to quantify, study, and build drugs for are the ones that are the most common in cancer. So those enzymes are a major focus of inhibitor development for therapeutics. There's about 100 kinase inhibitors that are actually FDA-approved. But the truth is, there are well over 500 different human protein kinases, and for many of them, we don't have good tools to even assess them. And while they may be good targets in disease for therapeutic development, they're needing sensors as tools, and that's what we provide.
The sensors can be used in anybody's lab and can be used in high throughput by big pharma and biotech labs to do drug discovery, and indeed to do all the many steps of drug development. One of the big things we have our sights on at AssayQuant, is bringing the sensor tools all the way to where they could be applied in diagnostics or personal medicine. For example, if someone has a solid tumor, nowadays, a PDX (patient-derived xenograft) mouse would be made where you implant the tumor into the mouse and see how it responds. What you can do with sensor tools that we make is follow the progression of treatment of a tumor to see if the enzymes that were unregulated get brought back into control with an anticancer treatment. Also, to see if other enzymes emerge that compensate and cause resistance. So, what this knowledge allows is more targeted therapeutics. Now, I said the banner applications are in cancer, but there's just as much interest emerging in kinases in neurodegeneration, inflammation, and metabolic disorders. This is because kinases are the traffic lights of the cell - controlling all actions and reactions…... "Go, slow down, stop, go, etc."
Protein Structure in Historical Perspective
ZIERLER: We'll move now to more technical questions as they relate to your research. The centrality of protein structure and function. What are the big questions today? What's the frontier of knowledge in this area? And how does that compare with when you began your career?
IMPERIALI: Oh, [Laugh] that just tells you quite how old I am. Let me say that when I began my career it was tough. Every small victory purifying a single protein, starting to learn about its structure, maybe studying its function was all very precise, step-wise, labor-intensive. It was often what we called "reductionist biochemistry." So, you would purify a protein, and study it in its simplest state - all on its own. You would see how it behaved and you hoped you could solve a structure. It was very painstaking. Where we are now with protein structure is beyond belief! Using AI-driven approaches and the world of known protein structures and sequences, new structures can be predicted. Maybe they can't be predicted to perfection, but programs like AlphaFold have changed the way people think. We've got mountains of sequence data that can be deployed. Where the big questions are now, is understanding at a molecular level is how does everything interact in the presence of everything else. How do living systems work?
Because no protein or enzyme is a solo player. They're all players that form pathways, and networks, and higher-order assemblies. And that's where we are now, with the ability to do the science at a bigger scale. Really, the problems are different from the problems we started working with early on, which involved meticulous stepwise characterization of single proteins. One of the big questions that is obsessing for me currently is focused on a critical frontier which concerns the coordinated functions of pathways and networks in the membrane bilayer. Maybe 40% of all cellular proteins will spend some of their time attached to or embedded in membranes. The rest of the proteome is soluble and is in the cytosol or outside cells. What we're trying to understand now is, and this is looking at teams of enzymes that work in a precise sequence, how does the membrane facilitate them coming together on a platform and performing these functions with great efficiency and accuracy in a defined order?
Because maybe each one of them doesn't work very well in isolation, but how does the metabolon, the machine, work? And for us, the membrane is key because you've got a lot of proteins all floating around in solution in three dimensions, they've got to collide with each other to interact. Whereas in the membrane, it is like a stage when the players come and go, but at least they are all in two dimensions. Part of the computational work that we were doing with the Straub and Allen groups at BU is to understand protein dynamics in the membrane so we can start to develop questions about how the membrane alters these dynamics, what it does for the reactions, what it does for the pathway. To me, some of the biggest problems in biology involve looking at networks and pathways, and developing multidisciplinary approaches to study this. To make headway, we will bring in any tools that we can - structure, informatics, biochemistry, biophysical measurements, synthetic inhibitors etc. We won't restrict ourselves in any way because each of the single approaches together can give insight. And each of the approaches involve disciplines that I've spent parts of my career on so that I can deploy into studies of systems embedded in membranes.
ZIERLER: The term protein modification, is this to say that it is nature doing the modifying? Or are you doing bioengineering to modify these proteins?
IMPERIALI: Well, nature gets everywhere first. [Laugh] Please recall that. We like to talk about the elaboration of the proteome in the form of what are called post-translational modifications (PTMs). So, a gene, a chunk of DNA, encodes an RNA, which dictates a messenger RNA, which dictates a linear polypeptide sequence, which will fold. We sort of scratch our heads at this stage because there are only 25,000-ish protein-encoding genes. And we ask – "am I as simple as 25,000 proteins?" and the answer is "no" because of protein post-translational modifications–The synthesis of the polypeptide chain is complete, the protein is probably folded, but then it is the PTMs that are really what explode the diversity of the building blocks of the proteome (the amino acids) by 100 or 1000-fold. It means we don't just have 20 different amino acids in a sequence, we have hundreds of modifications that may be done individually on different amino acids in sequences to alter the protein structure, the protein dynamics, the protein localization.
So, for example, I can stick a greasy tail, called a prenyl group, on my favorite protein, and it cause the protein to run to the membrane and stick on it. It won't be free in solution any more. I can put a phosphate group on my favorite protein, and the protein will do some acrobatics where it'll change its shape and suddenly start interacting with another protein, and then it may go run into the nucleus. Protein modification represents a central theme in biology that takes you beyond the central dogma. But while there is a lot of valuable insight into living systems to be had, some of the modifications took a long time for people to be convinced to study them. Because they're damn hard to study, frankly. [Laugh] For example, a protein kinase puts a simple phosphate group onto some amino acids. No problem, I can picture that in my mind. That seemed a bit too simplistic for me, so I figured why don't I go for something harder – there will be less competition – and that was how I decided to study glycosylation, the addition of large sugar molecules, which results in an enormous change in a protein structure - wherein the equivalent of 20% of a protein's weight worth of sugars get appended to the protein's surface.
What does glycosylation do to protein dynamics? Where and how do sugars get added? How do they change interactions with other proteins? We've made a big business of studying protein glycosylation over the years. And I've come to the conclusion that studying glycosylation is like an onion – it just has so many layers. The more you ask, the more you find out, and then the more you have to ask. The PTMs are important in many aspects of chemical biology. In fact, even the enzymes that catalyze PTMs, are sometimes hijacked by chemical biologists to attach new groups to proteins in engineering approaches. So, chemical biology approaches can be deployed to do engineering on proteins, not necessarily to change the structure, but to put a flag on the protein, like a fluorophore, or to put a localization sequence on that will send the protein somewhere in the cell or make the protein stick to a membrane. The PTMs are at the same time nature's design and us doing the best we can to co-opt nature's design.
The reason I went into this field in the first place, PTMs in general, was that I felt that I had slogged away at a bench as a synthetic organic chemist through my PhD work, and for every step in the synthesis that I did on a complex molecule, I'd have to protect every other reactive group so I could do chemistry at specifically one place. And then, I'd do that chemistry. Then, I'd carefully peel off the protecting group and do chemistry somewhere else. And that was my PhD thesis. And I said to myself, "How does nature do all this?" A protein kinase doesn't phosphorylate all over a protein, oligosaccharyl transferases don't glycosylate all over a protein. There are in fact short sequences that have an embedded code that says, "Put the phosphate here if you're this kind of kinase." I was fascinated by PTMs because I was fascinated how nature did a selective job to execute a selective outcome. And that simple curiosity took me down this sort of strange path, really.
ZIERLER: You're on record for being amazed at the complexity of glycoconjugates. I wonder if you could explain what glycoconjugates are and why their complexity is so amazing.
IMPERIALI: Glycoconjugates just mean biological molecules which include sugars–…. Let me digress a moment, you'll understand why in a minute: T. S. Elliot wrote a poem on the naming of cats where he pointed out that "Every cat must have three different names" – these are his sensible everyday name, his peculiar and dignified name, and a third name that he will never confess. Well, sugars have at least three names. They're called carbohydrates (a more chemical name) or saccharides (comes from Greek for sugar), and they're called glycans. They're all the same thing, although glycans tend to represent many carbohydrates or saccharides or sugars joined covalently in complicated structures, branching, linear. Glycoconjugates are when you take those glycan structures, and you stick them onto another biological molecule, like a protein or a lipid. Or, carbohydrate chunks may come together to form large glycopolymers. Those are all glycoconjugates. As chemical biologists, when we think of the biological complexity of biopolymers we think of numbers of building blocks.
If you think of DNA, we have four building blocks. We can do a lot with four building blocks. We have four building blocks for RNA. One of them's a little different from the DNA ones, but still only four letters in the vocabulary of polynucleotides. For proteins, you have 20 letters, but in proteins they can be varied through post-translational modification, so we're looping back to that, and the sequences can be varied. But DNA, RNA, and polypeptides are all generally linear polymer structures. Each building block is bonded to another one. They're not branched structures. The amazing glycan complexity comes about because there is not this simple, regular, linear polymer. But it's a complex polymer that may be branched, there may be all kinds of different places to form bonds to, so there's way more variety of structure. And for carbohydrates, say, in humans, there are about 15 sugar building blocks, but the glycans are much more complicated than polypeptides and oligonucleotides.
But, when it comes to bacteria, which is a focus of my current research, there are hundreds and hundreds of different building blocks. What's that about? Why did bacteria literally go crazy with sugars? It's not that every species of bacteria has hundreds and hundreds of building blocks, it's just the diversity amongst all the bacterial species. That, to me, is amazing complexity. It's crazy complexity because there's so much variation possible. Why is it? What does it do? Basically, the glycans and glycoconjugates are the language. Single-celled organisms need to talk to each other. They need to make an announcement, "I'm so-and-so, and I'm going to interact with that host cell." And all this complexity of carbohydrates is really a bar code that's describing the bacterium to other organisms. Other such as viruses, human cells, the extra-cellular matrices around human cells. Can we even make a dent in understanding this complexity? Recently, I was bold, or stupid, enough to give a talk that I titled "Bacterial Glycoconjugates: Making Sense of the Madness". Because what we need to do is to at least start being able to categorize all these different sugars, or signatures of sugars, or bacterial glycans, to try to discern their meaning. What is that glycan code? Never could there possibly be a better application for AI than that, but we need more ground rules first. That's the soup we're in right now. It's kind of fun. [Laugh]
Building Probes to Discover Cell Function
ZIERLER: You alluded to your interest in building chemical tools. More specifically, I want to ask, your work on fluorescent and luminescent probes, how do you build these probes, and what can they see?
IMPERIALI: Fluorescence and luminescence are really important tools for biology because cells and organisms don't have molecules that either fluoresce or luminesce very much. Well. apart from the green fluorescent protein and that cadre of proteins that were discovered in the last three decades. My group has focused on small fluorescent molecules or small luminescent assemblies because they can report the function of processes within cells or in complex media because the fluorescence signal is unique, and all the background that might come from all the constituents of the cell is effectively invisible. That's why you want to use fluorescence. Actually, the technology that AQT is founded on is a small fluorescent probe that allows us to spy on phosphorylation and the activity of kinases.
So, for example, if I want to study protein phosphorylation, I use an unnatural amino acid with a unique fluorophore nearby that sends out a fluorescent signal in the presence of magnesium when phosphorylation has occurred at a specific site in a protein. That signal can be observed in real time. What I like to focus on are small organic chemical fluorophores that give you a lot of bang for the buck by enabling mechanisms of fluorescent signal turn-on. We specifically try to make probes that are small, a bit of a Trojan horse. In this way you can easily incorporate them into peptides, and you can use them for assays without creating secondary interactions that will give you off-target measurements i.e. "bad data"! That's what we've done with the tools we use in the company. I actually have another shoebox of interesting fluorophores that we hope to transition to AQT one day that do what's called "environment-sensitive fluorescence", where if one of these small organic molecules is within a peptide, for example, and that peptide binds to another protein or another entity in a cell, you would see a light-up, so it's a real-time observation of interactions.
We've also worked with luminescent probes that are chelates of europium and terbium luminescent species. What's different about them to the organic fluorophores? Let me just give you a tiny bit of a primer. Organic fluorophores are small synthetic molecules that you can make in the lab. Lanthanides, such as europium and terbium, are trivalent metal ions of the lanthanide series. And they just have the craziest photophysical properties because they luminesce with really long lifetimes, and you can do a lot with that property in studying biology. Karen Allen (my collaborator at BU) and I, came up with the concept of lanthanide binding tags (LBTs – not to be confused with "BLTs"!), which are short peptides that bind selectively to lanthanides and exhibit some of the fascinating photophysical properties.
So, what you can do is express your favorite protein with a lanthanide binding tag, then when you add in the europium or terbium, your protein has the unique photophysical properties that can be used to study cellular destinations, and interactions, and dynamics in ways that you can't necessarily do with organic fluorophores. I just became fascinated in the power of these, and I think there is a lot of potential to still be exploited.
Ultimately, I think that research in the life sciences will always benefit from new tools and approaches. Biological tools such as antibodies and all the many different kinds of genetic and molecular biology tools, have been tremendously powerful for mapping out the complexity of living systems.
I believe what we need more of in the post-genomic era is tools that help us study functional proteomics. For example, colleagues at Stanford and Scripps, Ben Cravatt and Matt Bogyo, have all worked on what is called activity-based profiling. So, the sorts of probes that I and plenty of others are developing are designed with the goal of mapping out cellular function over time and how it changes. I honestly think the most important uses for the types of tools enable a researcher to say, "Okay, the community has determined that this protein has gone haywire in this disease. If I inhibit this protein and stop its function, do I have a good therapeutic?" The tools I make or have made and deployed into AssayQuant are tools to help people discover inhibitors, which could be potential lead therapeutics. Also to help determine whether the lead inhibitors hit 12 other proteins, which would cause mayhem, or are selective. By deploying the methods I developed in activity profiling, we can say "let's check on-target activity, but also let's really check off-target activity." That's really useful and it puts the researcher in an enviable position.
Every day, research in biology reveals new discoveries and new proteins that have critical functions in certain disease states, or developmental states, and so on. And we have the opportunity to deploy tools to say, "You've shown that that is a critical protein. You've shown that its function has to be tightly regulated. Can we help provide tools that will get you to an inhibitor faster?" Sometimes we don't really realize some of these facts, but when you send compounds through drug development and clinical trials, this takes years. And every step of the way, compounds fail and drop out of the pipeline. "This compound's no good. I'm going to follow this one." When compounds get into clinical trials, that's where you're first checking toxicity in humans, but then going all the way through to testing in actual patients. Only 1 to 2% make it successfully through to being an IND (Investigational New Drug). And what we believe is, if you could make sure your compounds were cleaner early on before clinical trials, the maybe that success rate wouldn't be so poor. Because all the cost of drug development is in that tail end of clinical trials, that is where the billion-dollar price tags come in. The earlier parts are expensive, but nowhere near as much. That's really where I believe that my stance on the importance of powerful chemical biology tool plays a role.
From Medicinal Chemistry to Organic Chemistry
ZIERLER: Let's go back now and establish some personal history. Let's go back to London. Your degree is in medicinal chemistry. You don't see this as an undergraduate option in the United States. Is that another way of saying you were thinking about being pre-med?
IMPERIALI: No, no, no. [Laugh] England is different anyway. At the time when I was growing up in England, you were tracked into higher education pretty quickly to see if you were good enough. When you're 11 years old, you had to really do well on a test called the 11-plus, if you failed you were toast, if you wanted an academic career. [Laugh] And then, based on that, you got to go to either a grammar school or a comprehensive school. Grammar schools would be the top tier. Straight away, you choose a specialty. I went into science, so focused on chemistry, biology, physics, math by the time I was fourteen. I had my cadre of other classes as well French, religion, history, English, even Latin and so on. By the time an English student would get to the end of high school, they would be more like a college student. I had a bit more breadth of horizons because my father was Italian, my mother was British. We grew up back and forth between Italy and England. I was already bilingual. Things like that. Oh, and by the way medical school is an undergraduate degree in England – I never would have gone in that direction.
I already had plenty to wrap around the science interest. I was always interested, even as a kid with my microscope and chemistry kit. But I didn't like parts of chemistry that I felt were too dry. I didn't like inorganic chemistry, so I didn't do it, and I didn't really like a lot of physical chemistry, except I need to like it now for biophysics. And there was this amazing opportunity that I lucked into in the UK in those years, which would've been the mid-70s, where there were three or four programs, one was at University College London, and others were sprinkled around the country, where you could actually take a degree in medicinal chemistry, not straight chemistry. If you went chemistry, you had to do organic, inorganic, chemical. You were really tracked on only chemistry.
By choosing medicinal chemistry, I kept my horizons open, and I got to do a lot more organic chemistry, but bundled with biochemistry, pharmacology, membrane chemistry, immunology, and just enough physical chemistry that I wouldn't make a fool of myself with kinetics and stuff like that. I purposely chose that track because it represented my ideal, what I really wanted to do. Honestly, I always wanted to do exactly what I'm doing now…Use chemistry to study biology. I'm pretty lucky, right? If I'd gone into a biology track, it would've been completely away from the molecular sciences in those days. Then, going from the UK to MIT as a graduate student, I made a decision that what I really needed to be able to do to keep on following my dream, which was to study biochemistry, biology, and so on, was know how to make molecules. My PhD was in synthetic organic. It was pretty focused. But it let me emerge from my PhD with really strong organic chemistry credentials, so I could then move forward in biochemistry or what became chemical biology.
ZIERLER: As a student at University College London, this is a generational question and a cultural question, how many women were there studying things like biochemistry? Did you ever feel like you were disadvantaged as a woman in terms of what you were capable of doing or where you could end up?
IMPERIALI: To be honest, before I left England, I didn't even think about it. I was completely surrounded by women. My mother and my older sister were really strong characters. I went to a Catholic girls' school, so my high school was all girls. Also, strangely, the med chem option at UCL actually had more women than men. This was very unusual because the average chemistry program had way less women. But that wasn't anything that really struck me at the time. When I came to the US, to do my PhD at MIT that was when I went, "Whoa, this is pretty different." In my incoming graduate class in chemistry, we numbered 60 total, but only six women, and by the end of the year three dropped out. Those were the numbers. And I naively hadn't paid a lot of attention to it until then. I hadn't really thought that much about women being disadvantaged. I didn't feel boundaries, I didn't feel limits. I started to feel a bit odd when I came to the US, thinking, "Well, this does seem a little peculiar. We are so few. Why are all the men so outspoken?" But I attributed a lot of it to the fact that when you grow up in England and do your undergrad studies there, you don't speak up in class. You don't say a word.
And suddenly at MIT I was faced with classes of students in my first year in chemistry, where all these people would fling their hands up, ask questions, answer questions. I was like, "Oh, no, I'm never going to make it here," not because I was female, but because my culture and upbringing were so different [Laugh]. Also I happened to be want to study in the field of organic chemistry, where women were really, really, really in the minority. What felt like happened over the years is that there were great women in organic chemistry, but they all morphed over to chemical biology – perhaps we were just better at multi-tasking [Laugh].
ZIERLER: When you were thinking about the graduate programs, were you specifically motivated to come to the United States?
IMPERIALI: Yeah, I'd always grown up moving around between Italy and England. I think everyone else in my high school class probably still lives within two miles of where they grew up. I didn't feel any need to stay near home because the concept of home had been rather fluid. And I decided that I would have a lot more opportunities if I came to the US. I felt that the UK academic system was very hierarchical, and you had to be about 70 before you could be a full professor, and you had to be about 60 before you could decide on your own research. I knew that wasn't going to work for me. And I envied the US system. It was much harder, but you could get out there, get an independent career in academia, and decide what you wanted to do. That was why I made that transition. I came to MIT, I worked for one of the tough professors because I didn't want anyone to tell me I was taking short cuts, and then I went on to do a postdoc with someone who was pretty tough as well. [Laugh] Because I wanted to be sure that I had the credentials for the job that I really wanted, which was to be an independent researcher doing what I wanted to do, not waiting in line. That was why I did the US migration.
ZIERLER: Why MIT? Where else did you look?
IMPERIALI: Nowhere. [Laugh]
ZIERLER: Is that because of MIT's reputation in organic chemistry?
IMPERIALI: No, there was one of the lecturers at UCL who said, "MIT's pretty good for organic chemistry." And I was doing very well in organic chemistry in undergrad. He said, "You should think about going there because there are good opportunities." There was one professor who I actually (!) dared to ask about it, Franz Sondheimer, who was at University College, he was a real senior statesman, and he said, "Oh, yes, go to MIT. But don't work for George Büchi, he's a mean man. Work for my friend, Sat Masamune he's a nice man." [Laugh] To this day I think "wow," George Büchi must have been really tough - if Sat was a "nice man". Because honestly Sat Masamune was an absolute samurai.
Aside from choosing who to work for, my roommate at University College was on a year abroad from Mount Holyoke, and she convinced me that Massachusetts was the place to be, so I focused in on Massachusetts. At the time I thought that Harvard seemed a bit too much like Oxford and Cambridge – strictly for the upper classes. [Laugh] And, I'd made an executive decision not to go to either of those early on. I wanted to go to the university established by Jeremy Bentham a proponent of modern utilitarianism. So, in a similar vein I thought MIT was more up my alley, and that was my choice. And then, I was really nervous that I wouldn't get in because at the time, I think they didn't take in very many foreign students. And then I was filling in the forms thinking, "I'm putting all my chips on this one, and I'm not going to get in." [Laugh] I carry on filling in the form, and it asks, "What fellowships have you applied for?" I go, "What fellowships have I applied for? I didn't even know I should apply for fellowships." So, I went around the notice boards at UCL – this is 100% the truth – and I saw a sign for the Kennedy Memorial Trust Fellowship, which was a fellowship set up after Kennedy's assassination to bring 10 scholars a year to MIT and Harvard to start their graduate work.
It was only a one- or two-year scholarship, but I thought, "I've got to put something on this form. Let me apply for that so I can at least put it on the form." I sent in the form. Most of the people at UCL didn't really think I was serious about going to MIT. In fact, I had one senior professor come to me in a class he was teaching, because I'd asked him for a letter of recommendation, and he said out loud to the whole class, "I believe you wanted this recommendation for the MI5." [Laugh] He was joking, of course. And I'm like, "Ugh, okay." Then, someone else wrote me a letter. Then, the third person didn't bother to write a letter. But, to my amazement, I was actually invited to interview for the Kennedy Fellowship, and then I got the Kennedy Fellowship. However, MIT said they couldn't admit me because there were only two recommendation letters on record. I literally walked into the head of department's office, and you just don't do that in England, and said, "I've got to have that letter. Because I have a fellowship now, and I'm going to MIT." [Laugh] And the rest was sort of history. But it also got me into Sat Masamune's group because it looked good. [Laugh]
Sat Masamune and Synthesizing Molecules
ZIERLER: Tell me about Masamune. What was it like working with Masamune? What was the lab focused on at that point?
IMPERIALI: We were exclusively a synthesis lab. We worked on big molecular targets, and there was also a part of the lab that did more inorganic chemistry. Satoru Masamune was really tough. He'd moved from Alberta. The lab was about a dozen people when I joined. And during the time I was there, it grew to over 30 people. It was a huge lab, and we were in subdivisions. I actually quite quickly got to be sort of one of the leaders in my subdivision, which was a great privilege. But earlier on, I think he was very circumspect about having a woman graduate student from England. He was very nervous talking to me and actually didn't really want to talk to me. He would talk to the postdoc standing next to me and tell him to tell me to do something. That kind of thing. I was getting pretty annoyed at the time. I wanted to say, "I didn't come here for this." Then, there was the famous day–and I've told a lot of colleagues this, and I don't think anyone would fault me for telling this story. It was about 1981 where ‘the man' as we nicknamed him actually came up to talk to me in the lab, and he said, "By the way, do you know how to type?" And I'm like, "What?" Those were the days you had to type everything on an IBM Selectric Typewriter. And he desperately had this manuscript that needed retyping. I just looked at him and said, "Sort of, but I didn't come here to do that." He never asked me again. Masamune was an amazing chemist and he was incredibly rigorous. I learned so much from him, but some of it was sort of wild west in terms of relationships. The group, of course, was also predominantly male and sometimes, almost predominantly Japanese.
ZIERLER: Tell me about developing your thesis.
IMPERIALI: Organic chemistry, especially syntheses, are not very exciting. [Laugh] They're sort of dry as a bone. You do an introduction saying how great it would be to make a compound, then you have three chapters where you're describing the logic and the methods that you developed to make it. They're not huge, hypothesis-driven, inspirational works. They are quite pro forma, if you will. He gave me two weeks to write it. [Laugh] I rented a Selectric typewriter, went into my tiny apartment in Central Square, typed out the draft, read it, and typed out the revision. I have it on my shelf. All the drawings were done with ink and a stencil, not with a ChemDraw program or anything – it didn't exist then. Honestly, it's only cool to me.
ZIERLER: Looking back, how biological was this research? Is this the origins of you starting to think about combining chemistry and biology?
IMPERIALI: No, to be honest, it was a means to an end. To go forward, and write papers, and get grants, when you say you're going to integrate organic chemistry into a program, you've got to have the credentials. My stint in the Masamune lab was to get those credentials, to learn how to do all manner of synthesis, which I did. I finished my thesis work in three years, but he had no intention of letting me leave. [Laugh] So I spent another two years, one as a PhD student and one as a continuing postdoc at MIT, before my real postdoc. In my bonus year at MIT, I helped to start a project between the Masamune group and three other groups, the Walsh group, the Petsko group, and the Sinskey group, who were all much more biological. Petsko was a structural biologist, Walsh was a biochemist, and Sinskey worked on metabolic engineering. At the time Masamune had some dreams that he was going to solve certain problems in biochemistry that the biochemists couldn't figure out. [Laugh] So he had me kick off a mini subgroup in that area in Masamune lab. I basically had four supervisors. [Laugh] Walsh, Petsko, Sinskey, and Masamune. I got a lot more training there in that two-year interim. Then I went on to do a real postdoc with Bob Abeles in hardcore biochemistry.
ZIERLER: Masamune's insistence on hanging onto you is really the most important sign of respect beyond the difficulties interpersonally.
IMPERIALI: Yeah, I think so.
ZIERLER: What was the value in staying on for a bit with him?
IMPERIALI: The value was that it was all new. Everything I was doing was brand new to me. Loads of methods that I'd never done before like making and purifying proteins, and running gels and assays. The value to me was the new training and getting to interact with different groups at MIT, so by the end of it I was more than ready to go on to a postdoc. The irony of my postdoc at Brandeis was, that the project that Professor Abeles really wanted me to do involved exclusively synthetic chemistry. So, I was flopped back onto the synthetic path trying to make certain types of compounds that Abeles insisted were going to be terrific, but no one seemed to be able to make them, even the drug companies with big chemistry groups. That was my challenge, but I made it work by trying dozens of approaches and I think I really gained his respect.
Bob Abeles at Brandeis
ZIERLER: What was the initial connection that led you to Brandeis?
IMPERIALI: A number of very eminent biochemists worked at Brandeis, either for Bob Abeles or Bill Jencks. JoAnne Stubbe worked for Abeles, Chris Walsh worked for Abeles. It was really the happening place for mechanistic biochemistry, which I felt was my next important step in sort of moving towards my independent career.
ZIERLER: Tell me about Abeles. What was he like? What was he known for?
IMPERIALI: Working for Abeles was amazing – one the leaders of mechanistic biochemistry. But he was also a heck of a tough guy. I went for an interview with him at Brandeis, which is an interesting campus perched on the top of a hill. And I think that part of the interview was could you get up all the way up the hill without being out of breath and straight away meet Professor Abeles. Then amazing thing about Abeles was that he was 100% into the science, he didn't have a fancy office or spiffy labs. His little office actually opening right into the labs. And when I arrive he had this massive poster on his office door that he said his kids had given him. It went along the lines of, "Yea, though I walk through the valley of the shadow of death, I shall fear no evil because I'm the meanest son of a bitch in the valley." I saw that on the door, and I said to myself, "Okay – now what have I gotten myself into?" [Laugh] The first thing he said was, "I don't like people who don't work hard." So, I said, "I don't think you need to worry. I worked for Masamune for this many years and he completely shares your views." I think he was just sort of vetting my personality. He already knew my accomplishments from my record. I was there for two years. And once again, amazing mentor, tough mentor. People all thought that I was crazy working for Masamune and then Abeles. Yes, they were very both demanding, but if you worked hard, you had their respect. And that was what you needed to do.
ZIERLER: This cemented your identity as a biochemist, your appointment at Brandeis?
IMPERIALI: I think so. But when I applied for jobs, I still went for organic and biochemistry positions with the knowledge that I was going to be doing work at the bio interface.
ZIERLER: Tell me about entering the job market. What kinds of jobs were available? Where were you considering?
IMPERIALI: I basically just wanted a good academic job where I could follow my dreams of doing independent research and running a group, but my personal life was a bit complicated. So, although I got a couple of really good opportunities back in 1986, I made rather a wild card decision to go to Carnegie Mellon. At the time they were building up the chemistry department and had a lot of funds to invest in new people. In the end, even though I got my first NIH grant and even a first paper, things at Carnegie Mellon went wildly off the tracks with major problems in the Chemistry Department and so, in 1989 I basically hit the "delete" button and started again. I literally applied everywhere and ended up getting just one job – and as they say – the rest is history.
ZIERLER: Caltech to the rescue in 1989!
IMPERIALI: Indeed. [Laugh]
The Chemistry Dream Team at Caltech
ZIERLER: Were you following, for example, what the Dervan lab was doing in those years?
IMPERIALI: I was hired into organic chemistry at Caltech. I was one of the team that included Bob Grubbs, Peter Dervan, Dennis Dougherty, Andy Myers, and Jack Roberts. I was there with that group. WOW! proud wouldn't even begin to describe it. It was amazing. And Peter Dervan was so gracious. "Oh, we're so pleased you're joining." They were giving me these accolades that I just couldn't even believe were happening to me after I'd been through some serious stormy seas. Bio-organic chemistry was beginning to happen there. In fact I taught bio-organic there to the upper-level undergrads and first-year grad from the beginning. I also taught undergraduate organic chemistry as I had done in my time at Carnegie Mellon. In the end I taught organic chemistry for almost 25 years. It's a wonderful course to teach – the students show up absolutely dreading it and you get to win them over. [Laugh]
I had to finally give up teaching OChem I moved departments at MIT, but I feel I did a great service to teaching organic. Some years at Caltech, Grubbs would teach the first quarter, then it was me for the second, and then Dervan for the third quarter. The organic group would go to lunch together almost every day. It was sort of magical. We'd go to the Athenaeum, then we'd go back, or sometimes we'd split off and some of us would go for coffee at Starbucks. That was before Erick Carreira joined the department, after that there were six in the organic subgroup, plus Jack Roberts, the statesman. And Jack was just a remarkable person.
That reminds me - I want to share with you a photograph taken around 1990 or 1991 that has been in my office since my Caltech days. It shows Jack Roberts in the second row, but front and center anyway. This was how the department looked when I was pretty new there. In the front row, you can Norman Davidson, Ahmed Zewail, and Linus Pauling. Pauling was just back at Caltech for a visit and he even gave a lecture. To the left you can see the young Andy Myers and Sunney Chan. And then, others we have lost over the years like Jay Bailey and Bob Grubbs. Harry Gray is there too and he was just a great supporter to me. And - he would always make me laugh.
ZIERLER: Did the tenure clock reset at Caltech, or was Carnegie Mellon counted?
IMPERIALI: No, they said, "Look, we're going to reset the clock. You may not need it, but it'll take the stress off." So, I am one of few people who can say they did their first three years of tenure track twice, I loved it so much the first time. [Laugh] But, frankly I needed the time. Even to this day maturing a chemical biology program just takes more time. If a young professor starts a tenure track that's the classical tenure chemistry track, and they're program is pure organic synthesis, they can get what they need pretty quickly. If a young person starts a chem bio program, there's a tangible lag because you've got to get systems working and going. These are often complicated systems involving cells and life. In biology, young people often bring their projects with them. In chemical biology, young people were quite handicapped because they couldn't bring their projects with them because chemistry departments didn't agree with that, but their projects were much more complex to set up. It was a good job I took the extra bit of time. My personal life was in chaos anyways, so I didn't mind having a little extra time.
ZIERLER: Tell me about building up your lab. What was most important to you?
IMPERIALI: I think I always wanted to have people who I felt were fearless and were in it for the research and the knowledge, not for the next paper. I tried to recruit broadly and I always tried to bring people in with different expertise, especially when it came to postdocs so that the team as a whole would grow in expertise. There was always a core of material that we all knew, but people would come in with different abilities and capabilities, so I was able to morph the activities in my group through that. To be honest though, both at Caltech and now at MIT, these are places where you can very easily have a postdoc or a student go to someone else's lab and learn how to do something new. I've never felt that "I just can't do X, and I can't go ahead." I've always felt that "I'm just going to park someone over in another PI's lab, and they're going to learn how to do it." And my group has always reciprocated when it came to people learning from my team. I've always hoped to pick and recruit the type of coworkers who had that. "I just want to learn what I need." I recruited by problem, not by what they were going to do, but rather, "This is the problem."
Building a Lab Around Glycosylation
ZIERLER: By seeing, for example, what was happening in the Dervan lab and the Dougherty lab, how did you cement your own reputation in chemical biology? How did that begin for you?
IMPERIALI: Really, we of started with our flagship work in the glycosylation area, so it was always quite distinct from other activities in the division. It was more biological research in terms of some of the things that you needed to be able to do to make progress in those areas. So, I felt that we were very distinct amongst the organic group. There wasn't any actual overlap because Dervan's work was all in the nucleic acid field and then Dougherty was in what one would call physical chemical biology, so the neurobiology work, and before that, he'd been more in the area of molecular recognition and model systems to develop a physical understanding of the interactions and forces in order to understand the counterparts in biology. My work was in de novo protein design and glycobiology so for a small subgroup we synergized really, really well. And then, having really strong synthetic chemists like Andy Myers and Erick Carreira, as my sort of siblings, basically, gave me the people to ask for help if we were having trouble with synthesis and mechanisms. I think there was never a feeling that we were overlapping and someone was doing something that I was doing. I think we were very distinct – and super-happy about that.
ZIERLER: As a woman faculty member at Caltech, did you experience any difficulties? Was life well in Pasadena in that regard?
IMPERIALI: I never felt that there was an issue in the division. We were few for sure in the early ‘90s and I was the only woman in organic at the time. But my colleagues were so collegial, and helpful, and there was no hint of discord amongst us due to gender imbalance.
ZIERLER: That's wonderful to hear.
IMPERIALI: Where we did get clobbered was in the outside world because there started to be a certain mandate on the representation of women on committees, so we were always asked to do way more than we should have been. "Oh yeah, it's 10% of the population, but we want 40% women on this committee or that conference line up, so we're just going to ask you anyway." [Laugh]
ZIERLER: David Baltimore being named president of Caltech in the mid-1990s, the first biologist to lead Caltech, did that register with you? Did that change chemistry or biology to some degree?
IMPERIALI: I think it probably changed biology more than it changed the Chem & Chem E division at the time. I think it was a big deal to have a famous biologist come and be the president. I wouldn't say I saw a whole lot of him. But I think it was a great step for Caltech to have a president with such credentials in biology at the helm. I think that he didn't quite understand the chemists as well as he understood the biologists. [Laugh] Sometimes, there would be things that were very peculiar problems to chemistry, such as NMR instrumentation and hoods for synthesis, and I'm not so sure he had the measure of it, but it was a boon for biology, for sure. In the divisional structure at the time biology was quite separate, really. It was the Division of Chem and Chem E and the Division of Biology. I certainly had friends and colleagues in Biology, like Pamela Bjorkman and Steve Mayo. But there was a pretty big chasm between me and them at the time. Maybe it was just because it was several years later when I came to MIT, but I didn't feel the gap so much. Around 2010 I moved my labs to the biology department. Really, all the universities have changed, some have blurred boundaries a lot and some – not so much. But strong bridges between Chemistry and Biology are all to the benefit of the Life Sciences as a whole.
ZIERLER: Did the tenure process change your research at all? Did you feel less bound to do anything in particular?
IMPERIALI: No, because I was already doing what I wanted to do. I just wanted to do more of it. I don't feel it was like, "Oh, thank God. I can ditch that boring work and do something different." [Laugh]
Returning to MIT
ZIERLER: When did you start to think about the big return to MIT?
IMPERIALI: The opportunity came up in 1999. There were some changes happening at MIT – these were not related to my hire, although a lot of people decided it was. So, in the late nineties, there was an enormous investigation (or call it an exposé if you want to charge it up some more) on how women faculty were being treated in different institutions of higher learning around the country. And it was the MIT senior women who had more or less forced the administration to do a full autopsy and with all the data that they had it became clear that things were not as fair as they should've been. And a lot of other institutions were forced to self-reflect and, no big surprise, if you start peeling off the layers, you find there was inequity all over the places. It was "almost" funny, I remember traveling at the time, and someone at an institution that will remain nameless actually asked me in a way that everyone in the room could hear, "Did MIT have to hire you because of the woman thing?" [Laugh] And I said, "No, they hired me because I'm good." [Laugh] It really wasn't coupled to that. I think MIT Chemistry was excited because they wanted to bridge more chemistry, and biochemistry, and chemical biology. I was an alumna who'd done well there, so I think there was a lot of excitement about trying to get me back. And I was ready for a move.
Some of the decision rested on the opportunity and some of it was personal. I did not really have any strong ties to the LA area; my family was all in Europe - England and Italy - and LA felt like such a long way away - even in time zones. So, there was some attraction for me moving back to the East Coast in that respect. Then, the other part was just sort of sociologically, I wanted to be back in an urban environment. Pasadena's a lovely place, but it was quite suburban relative to where I felt comfortable having grown up in London. It was too far for me to move to more urban areas in the LA basin because I didn't want to have some horrendous commute.
In the end I thought a change would be good and that I could have an impact on my field by moving. The cogs fell into place. My family at the time was thrilled because I had been so far away, and, especially during tenure-track years it was very hard to see that much of them. My father would make a pilgrimage to Pasadena every other year, but it was a hell of a journey for him at his age. There were just a lot of things aligned that made it a good time for me and appropriate for MIT. But I missed the lunches at the Athenaeum, that's for sure.
ZIERLER: These are all external factors you're emphasizing, the community in Pasadena, being so far away. Just in terms of the students, the research culture, and your faculty colleagues, in that regard, you were happy.
IMPERIALI: There was no big impediment there to getting great science done at Caltech – it was an amazing place to rise through the academic ranks. In fact, it was probably easier because it was smaller and a more nurturing. [Laugh] Incidentally, the students at MIT have "IHTFP" stickers on their computers and T-shirts. You can Google that if you need to [Laugh] – a little less nurturing. I thought the moving would shake me up, shake my science up, maybe I would ditch some stuff I didn't want to do any more, and I hopefully my work would grow in some new directions. I felt 10 years was good service and I wasn't leaving too quickly. And I was rather proud to come back to MIT as a named full professor. That meant a lot to me. Although sometimes, I suppose I felt like, "Well, it's time to move along." And then ten more years later I decided to move from chemistry to biology, to shake things up a bit more as well. I think it was probably good for my science, in all steps.
ZIERLER: Moving back to MIT, the plan that this would shake up your science, how did that play out? How did your research change?
IMPERIALI: I'm not sure I can put my finger on it. I think it became bigger and bolder. I think we took on more of the biological and biochemical problems. Also, in addition to the faithful team that moved with me from Caltech I got new grad students, who were somehow different and some awesome postdocs especially when I first arrived. I felt that I was getting more postdocs from Europe than I did in Southern California. I think, that is because the Europeans favor the northeast quite a bit. And the group just grew and was very, very productive. A lot of the small molecule fluorophore work happened for the group at MIT, and I don't think it would've necessarily advanced as much when I was at Caltech. My research areas expanded. I was mostly doing the glyco work at Caltech with a some de novo protein design, but after the move we expanded the probes and chemical biology tools work in full measure. That included the sensor work, and research on photochemical caging as a method to study biological process controlled by light. What did I leave behind? I can't really remember. And whole group moved with me, which was really impressive.
Steady Path to Pursuing Biological Questions
ZIERLER: You're experiencing MIT through a very different perspective now as a member of the faculty, not as a graduate student. Still, I wonder if you can reflect on how the culture at MIT changed or did not over those years.
IMPERIALI: It just felt like a different planet, being a faculty member instead of a grad student. Although, I always joke that the main thing that changed was that I could afford to buy lunch. [Laugh] But it was a little better than that. It was really a different place. Some of the old faces were here, but it was a very different. The main chemistry building was all renovated, there was a lot less of the hardcore organic chemistry in the department and there was more biological chemistry. And then, there were opportunities to form great allegiances with the bioengineering and biology departments. Honestly, when I was a graduate student at MIT, I would've never strayed beyond my lab on the third floor of building 18. When I became a faculty member I interacted broadly and, by the way the sizes of the classes I taught exploded!
ZIERLER: The transition to becoming more biologically oriented, was your work in protein glycosylation sort of the pathway to that?
IMPERIALI: It was certainly a necessity to do more biological work there because the problems could only be addressed through more biochemical or biological approaches. Also, with respect to the biological significance of glycosylation, I will tell you, it was really dark-ages stuff when I started working on it. Some colleagues were telling me, "Oh, don't work on glycans. They're too complicated – and who cares?" But, now the significance of glycans and glycobiology in biology is clear and clearer. It's still not so well-represented in many departments because you had to be half mad to take it on. But over the time I've been in this field, it's accelerating and the importance of sugars in biology is as clear as day – but only if you want to look. [Laugh]
ZIERLER: The transition to biology as your primary appointment, was this a gradual process? Was it one particular paper?
IMPERIALI: I think what drove me to change department was a mixture of the science, a personal decision - again, and the desire for a different environment – again.
ZIERLER: What was the research? What really compelled you to sort of make that shift?
IMPERIALI: It wasn't so much the specifics of the research, it was as being more surrounded by people who were talking about different biological phenomena, or things they'd discovered, or things they were working on. To me, it was more about what does the world need from chemical biologists? It was much more about bringing attention new knowledge about a lot of the techniques that are so prominent now in biology. I didn't want to do another lab move, I was happy on the campus and loved teaching and living in Cambridge. So, I made a different transition that would change my environment in a different way. It really did happen quite quickly.
ZIERLER: When do you start thinking about DNA damage?
IMPERIALI: The work you are referring to was related to all the work we were doing with kinase assays and developing strategies for assembling caged phosphoproteins. With uncaging phosphoproteins with light energy, you that you could turn on and off a function in second and look for a cellular output. So, we did some really cool work with Mike Yaffe's group, in the Koch Institute, which is where a number of the biology faculty have their labs. So, we developed chemical biology tools to study the DNA damage response. Another important influence that I had, was the opportunity at MIT to join an initiative funded by a U01 grant. The U01 focused on cell migration. U01s are big, multi-PI grants. This one was run by Rick Horwitz at UVa and Doug Lauffenburger and Linda Griffith in MIT's bioengineering department took the lead for the MIT component. So, because there was a big interest in having a chemical biology tools person on board, they brought me into that big initiative. In this way I could do probe development, but be amongst the biologists who studied cell migration. It's still an issue with even NIH funding. The NIH favors support of hypothesis-driven work, and if you're making tools, you need to be applying them to do hypothesis-driven work. But making tools takes time in the first place, so you need funding to do that before you can do hypothesis-driven work. On the U01, I had the opportunity to develop tools and apply them into the cell migration work.
The Strangeness of Polyprenols
ZIERLER: When do polyprenols enter the picture for you?
IMPERIALI: [Laugh] Polyprenols are strange molecules. They're long, big, greasy molecules. You know about lipid bilayers. Long alkyl chains, that come face-to-face to get away from water. Lipid bilayers form the boundaries defining all cells. OK so polyprenols are much larger than the tails on the lipid bilayers, and in biology, the really long linear polyprenols are used exclusively in glycan assembly. The long greasy tail parks in the membrane, and all the enzymes that sequentially act to build up a glycan do it on a polyprenol phosphate. Why is that? Very early in my career, where I started studying glycosylation, I thought, "First of all, I'm going to figure out why nature uses these polyprenols." I'm still here doing that. [Laugh]
What we believe, and I think that will be a nice capstone as I move towards the later years in my career, is we're getting hypotheses that are now testable about the roles of the polyprenol in the multistep pathways that occur at membranes. We believe that the polyprenols may help create a microenvironment where enzymes that all belong to a pathway congregate in order to function sequentially to deliver a glycan product. And the polyprenol is there really not as a simple membrane anchor, but as an entity that impacts the structure of the membrane in ways that may promote diffusion and interactions of enzymes involved.
The polyprenols featured early on in my career. I needed polyprenols, but they were devilishly expensive and the commercial material was junk. Then, I discovered from papers that there was a high concentration of polyprenols in ginkgo leaves. So, I went out with my first graduate student roaming the streets of Pittsburgh to collect ginkgo leaves to extract polyprenols. There needed to be a chemical reaction to convert it to the dolichols, which are the ones that are used in mammalian biology. We developed some chemistry for that. And then, we moved further on. Much later on we studied bacterial glycosylation and needed different polyprenols. It's a funny story, so you'll get the punchline.
The type of polyprenols didn't occur ginkgo leaves, instead they were abundant in the dried red leaves of staghorn sumac, so I returned to my roots (ha ha), and went collecting leaves again, and extracted those linear polyprenols to do my research. I don't believe anything should get in the way to do science and in this case – dried leaves solved a problem because the polyprenol extracts were pivotal for the biology and biochemistry. So, coming back to what the polyprenols in glycosylation are for, "Gosh, I can't believe we still haven't solved this problem. I can't believe we don't understand. Nature doesn't do anything without a darn good reason." And I think the reason is very much wrapped up in what polyprenols do to membranes, even though they look like just another chemical structure that's in a membrane, I think the purpose is functional because of the impact that it has on the biophysical properties on membranes to promote reactions. It's not a bystander, it's not just a membrane anchor, it's functional.
ZIERLER: Arriving in Cambridge in 1999, it's 25 years later. When does biotech transfer from being just a few buildings here and there to this enormous endeavor, making Cambridge a hub? And did that affect your research at all?
IMPERIALI: It all happened while I was gone and Cambridge was not the same place when I got back. It had just grown and grown, and was still growing. I think the thing about being in such a hub is that there's just a lot more activity, there are lots of people coming and going, your students can find good jobs in the area, there's a lot of interest, your counterparts in local companies would even come to seminars. There's just a general heightened interest in the biohub. It's a little bit over the river, too, with the Longwood campus, but there's so much in Cambridge. I can look out the window and see different companies, Novartis, Biogen, and so on. I think it's great for our area. It does make parking a bit difficult, but I'm okay with that. [Laugh]
ZIERLER: We'll move our conversation closer to the present. When COVID hit, did you see an opportunity to become involved in coronavirus research?
IMPERIALI: Actually, not for my own group but I followed all the literature and the events like a hawk. Didn't we all. I did get rather angry about some of the archived reports. You see, things were moving so fast there was not time to publish papers with peer review so the was a lot of material in archived formats without peer review – but with the opportunity for public comments. But what I did try to do a little bit was to call attention to viral protein glycosylation especially the famous spike protein. I was thinking - "Guys, we've got to look at the sugars because the spike protein is covered in sugars." There were people making statements about mutations in the virus, in particular the spike protein and how each new variant that came along had this mutation and that mutation, but I felt the connection to glycosylation was not always made. You see, once you make mutations in a surface protein like the spike protein, the sugars jump around and the virus changes how infective it is and the anti-viral vaccine efficacy might change. And I actually tried to call certain people who were putting articles on bioRxiv and said, "Don't you think you should be talking more about the glycosylation of the spike protein?" But some researchers were uninterested and I think others knew a lot less about the glycosylation pathways. I would say despite the fact that glycans are so important in virology, they kind of get short shrift because viruses use our machinery to make the glycans, so we think that the viral glycans are just the same as our glycans, but there are actually subtle differences. Finally, I think interest in the sugars grew as researchers were becoming desperate to characterize the spike protein, which was the basis for the mRNA to make the vaccines from. Bottom line you really should need to have a grip on the glycosylation patterns, because they will influence the immunogenicity, which will influence the vaccine response.
ZIERLER: During the mandates to do remote work, had your lab become sufficiently involved in computation and bioinformatics where it was a relatively easy transition to be away from the lab?
IMPERIALI: It was fine for some people who had datasets and were structural biologists. It was tough for others. So, we as a group, went on a mission to basically do another round of learning, so everyone was presenting different topics that they had to prepare and present in ZOOM meetings. We also did do quite a lot of writing in that time and waited for restrictions to ease up. It was really hard. We finally got some access by June, but it was still very limited and the could only be one person per lab areas – so all the fun of being in a lab and the collegiality disappeared. Also, our biggest job was not just worrying about science but about keeping our people safe and sane. Creating enough opportunities for interacting with lab mates, even if it was on Zoom. It was the biggest challenge of my career.
Predicting the Glycome
ZIERLER: We'll bring the story right up to today. What is the Imperiali lab currently focused on? What's most interesting to you in the field?
IMPERIALI: We are focusing on the aspects of the bacterial glycan pathways. As I said, the making sense of the madness, trying to establish some paradigms and some sensible ways to predict the structures and functions. Wouldn't it be great if you predict the glycome from the genome and the proteome? There's a long way to go but we do see some glimmers of light at the end of this particular tunnel. The other thing that we're very excited about is, we've had some developments lately in making glycan-binding proteins. If you want to study a protein in a cell or in an animal, you just make an antibody to it. The antibody can be used as a reagent in all kinds of applications to probe the protein and location in or on cells. There are also therapeutic antibodies. Also, if you want to study DNA and RNA, you capitalize on duplex formation and being able to sequence and synthesize. If you want to recognize glycans, [Laugh] you're clean out of luck because the major reagents that can be used to recognize glycans are proteins isolated from plants, fungi, and some bacteria that are called lectins.
Lectins are very weak binders and they're not very specific. They won't make any sense of the madness. We also don't have near enough lectins to deal with the diversity of glycans. You can also go to huge lengths and make antibodies to sugars, but sugars are not very antigenic, and, it's expensive, and worst of all you have to use an animal host to generate them. Recently, we've been developing yeast surface-directed evolution of small scaffold proteins and demonstrating that we can make selective glycan-binding proteins out of those that can ultimately, we hope, be integrated into what we call glycan readers. If you have a small glycan-binding protein that recognizes for example two specific sugars linked in a particular way and then another one that recognizes a couple other sugars, can we ultimately make readers to read a bigger chunk? That is something I really want to push hard enough to get to commercial viability because it's so badly needed.
ZIERLER: This is really a connecting point between the lab and the startup.
IMPERIALI: Yeah, it is.
ZIERLER: For the last part of our talk, now that we've worked up to the present, I want to ask a few retrospective questions about your career, and then we can end looking to the future. First, if you'll indulge me, just one question on some of the most significant awards that you've been recognized for. Both because of where you come from and where you are now, I wonder if you can compare the honor of being a Fellow in the Royal Society of Chemistry and being a member of the National Academy of Sciences.
IMPERIALI: The NAS is such a big deal for me. By the way, in the interests of full disclosure I'm only a Fellow in the chemistry part of the Royal Academy, not a "real" Fellow of the Royal Academy. I was extremely proud to be elected to the NAS. I think even my relatives were impressed – it is rare that our accomplishments rise above our own rather cloistered community. [Laugh] But, the NAS was significant to neighbors, family and friends who were not scientists. To be quite candid, to get awards, you have to be fortunate enough to have people who want to be your advocates and get you in. I genuinely believe that, even though I was at MIT, I know that Harry Gray was really my advocate in the NAS nomination. I suspect it because he was just so happy about it all. [Laugh]
ZIERLER: Other awards honor your commitment, your love of teaching undergraduates. This is not an automatic for professors at research-intensive universities. Why is teaching so important for you? Why do you get such energy from it?
IMPERIALI: I just love explaining stuff. I love using quirky ways to explain things to get concepts into the students' heads, I love getting on the level of the student and finding out what interests them and pivoting my teaching to reflect that. Nothing gives me more satisfaction than when I've taught a good class. I really enjoy getting them engaged with the material that I'm excited and for example giving them my insight in chemical biology. Even when I was teaching general biology or organic chemistry – there are always cool stories and anecdotes to tell. I tend to overdo it though, [Laugh] and I spend infinite amounts of time running down rabbit holes to find the perfect picture or the perfect description. It is just something I get a lot of joy out of, and I think it makes a difference, especially in some courses, like sophormore organic chemistry – it's the pariah. Nobody wants to take OChem. But I taught it at Caltech and MIT, and I think I had a lot of students who loved the course, once I got them over the fear of it. My proudest achievement is being chosen for the Feynman Award at Caltech. Forget the other awards, forget the NAS that award was the most meaningful of all.
ZIERLER: That's the one. [Laugh]
IMPERIALI: That's the one, yeah.
Generational Pressures and Possibilities
ZIERLER: All the graduate students you've had, all the postdocs that you've worked with, what has changed and what has remained constant in terms of their interests, their motivations, what they see as their opportunities in their careers?
IMPERIALI: I believe that there was more optimism earlier on - the sky was the limit and there were a lot more opportunities. I think in this last decade, it's felt like the students and postdocs were worried all the time. They were worried that their PIs were struggling to get grants, or they were worried about getting jobs, or they were worried about getting to the end of a paper or chapter. They're a much more nervous crowd right now. And it's worrisome for our community. I just feel there are so many pressures and stresses on students, are we scaring them off? What is it? I don't know how there'll be a rebound. The amazing thing about science at the university level is, you often don't know what you're going to find. And it's discovery at its core and you're also counting on a dose of serendipity. If you're a student or postdoc and you're worried about, "Who's going to cover my health plan? and can I pay my rent?" it's a huge restriction on creativity. Don't get me wrong, there are still tons and tons of good students, but as a whole, there's more nervousness and worry. Wars, climate, and everything. There's a lot more on their plates. How could they not have changed?
ZIERLER: As you interact with your colleagues who are junior faculty members, what are the pressures facing an assistant professor today that might be different than what you faced?
IMPERIALI: I think when I was an assistant professor, there were so many things we didn't worry about. Now, the students are seeking to unionize and that is changing the landscape for our junior people. When I was a student, nobody asked me to keep track of tasks or count vacation days. We didn't do any of that. Maybe I had a health plan, probably, but I felt I was just lucky to be getting a PhD – I was thrilled to be a graduate student, I was getting a living wage, I was excited about science. Now, our junior people have a lot of things they have to worry about. There seems to be much less unfettered love of science. If they're the students are strong enough, they're going to do fine. I just feel, and it's tough to say, and maybe there's a better way to say it, but it seems a lot less joyful right now. I don't want to end on a down note here, but the times have changed.
Seeing the Life in Proteins
ZIERLER: Your work has been lauded in a very interesting way for your capacity to look at proteins in their living context. What does that mean to you? What does it mean to see a protein in its living context, what are the tools to do that, and what can you see that is not possible by looking at a protein in its dead context, as it were?
IMPERIALI: I think all biological systems are all very complex communities of macromolecules interacting. The most important thing to do is to try to look at them in context of what else is around when this protein functions. Because in almost no cases are macromolecules – proteins, sugars, nucleic acids singular. We try to develop tools where you can look at functions more in a community context. You can look at a test tube of clean protein. Nothing else but buffer is there. Then, you can start adding to the mix. But then, you can take a big step closer to a cell by using the lysate of a cell or the lysate of a tissue. Then, you can look in live cells, where metabolism and all celluar functions are still happening. You can even look at live multicellular organisms. I think what's important, what we all recognize, is that you can't do reductionist biochemistry and learn anything about living systems anymore. You have to throw in the complexity, and the complexity makes everything more challenging. And the complexity with looking at systems that work at a membrane is a whole new level – you can't solubilize your protein out of a membrane and expect to see anything that behaves like the real thing. Having to pay as much attention as much as possible to the native environment of proteins, and reactions, and interactions is the thing that I think is most important.
ZIERLER: I'll use a title that you came up with for an article as a way to capture what your research represents. "Interrogating biology with a chemical lexicon." I wonder if you were getting at something deeper than just using chemistry words to understand biological problems. I wonder if you can reflect on that.
IMPERIALI: What I always try to do is say, the biology field is very fundamentally important to life, right? But I think what I wanted to bring forward was that chemistry has a real seat at the table to do that – chemistry is just as fundamentally important to life – it is life. Also, there are things that we can interrogate with chemistry that we might not be able to do with genetics, or molecular biology, or immunology, or other classical methods. More and more biologists are realizing that. They're using some of these tools like BONCAT (Bioorthogonal Non-Canonical Amino Acid Tagging) that Dave Tirrell introduced. Many biologists are using it, so we need to educate them enough about the chemistry and to realize that all these tools and reagents, if they don't work the first time, you've got to talk to your chemical biology friends and think them through so they works the second time.
There is a disconnect, where people will buy a reagent from a company, and it doesn't quite work, and they say, "Oh, this chemistry approach no good" You've really got to form partnerships because coming up with the chemical lexicon to interrogate biology is an engineering process where you go back and refine, and go back and refine until it's good enough to be in anybody's hands to ask a particular question. I think what I was really trying to speak to there was the need for chemistry in the life sciences. And sometimes, I think the chemists are going to get thrown off the bus here, and it's really important they stay on it. You need chemistry to understand life.
The Quest to Integrate Scientific Knowledge
ZIERLER: Finally, looking to the future, if we can zoom out, in the grand sweep of history, if you're really part of the founding generation that has interrogated biology using a chemical lexicon, where does the field go from here? What's the frontier as you look at undergraduates who are captivated by this, graduate students and postdocs who want to make their own contributions? Beyond the timescale of your own career, what are the big problems that they're going to need to look at?
IMPERIALI: I think the sky's the limit, but the biggest challenge is integrating everything that's available to them and maintaining enough of a level of sophistication to know what's coming out that's going to make a difference to what you're studying. We are racing try to keep up as the feel is moving so rapidly. So many challenges can be tackled now that you couldn't have dreamed of 10, 20 years ago, it's a tsunami of choices. Also, I believe the biggest challenge is finding those incisive problems to study that you can say, "If I knew this, then I could do that." Finding those, and then being prepared to deploy any method you can to get to the bottom of it. Is it chemistry, is it physics, is it mathematics, is it biology? Think of the brain, how are we going to figure that out? [Laugh] Me brushing my teeth in the morning, how many processes are going on in my brain? Those are gigantic problems. And you've got to unravel it all to understand them and to enable the recognition of molecular approaches to disease or ways to prevent disease. The technologies that are available represent an embarrassment of riches, and people will just have to be prepared to keep on being aware of the opportunities. And at the same time, they're going to have to be careful because I think even in my day, you really had to be careful that people don't call you a jack of all trades and a master of none. If you're one day doing this method, one day doing the other, you've got to prove to them, "No, I've got my credentials. I'm doing this, I know what I'm doing!"
ZIERLER: Just as an addendum to that, as you survey your own career, your own accomplishments, you've articulated that it's a problem, an intellectual, organizational problem to look at all of these things. How do you view your work as a bridge to these future discoveries?
IMPERIALI: I'm not sure, but I think it's just showing what can be done with a complex problem where you start, and you really don't know where you're going, but you're prepared to work your way through it. The bridge is really if I were to write an autobiography, would I be able to demonstrate that everything was leading somewhere all along that I had in my mind? Possibly. But in some cases, it's just lucky. [Laugh]
ZIERLER: And there's some grit in there, too.
IMPERIALI: [Laugh] Far too much grit.
ZIERLER: Barbara, this has been a wonderful, wide-ranging, so interesting conversation. I want to thank you so much for spending the time.
IMPERIALI: Yes, well, thank you for your patience and interest.
[END]
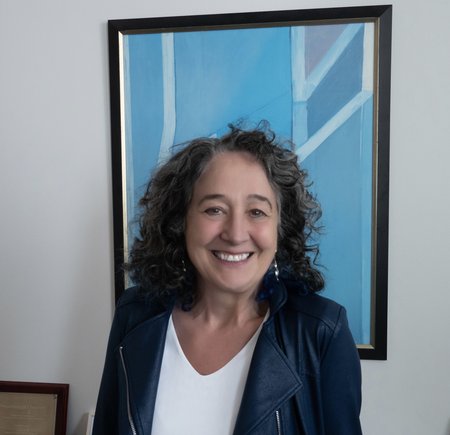
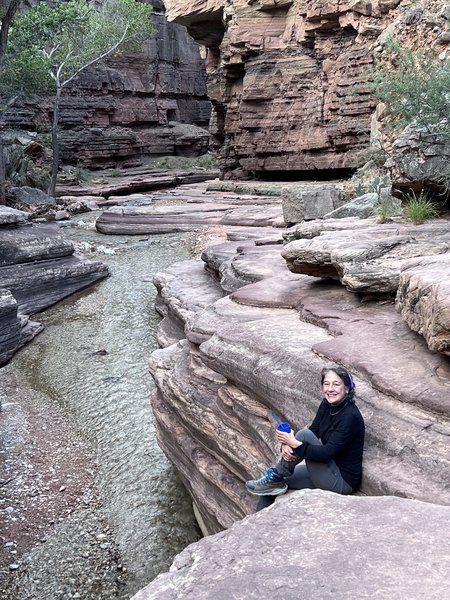
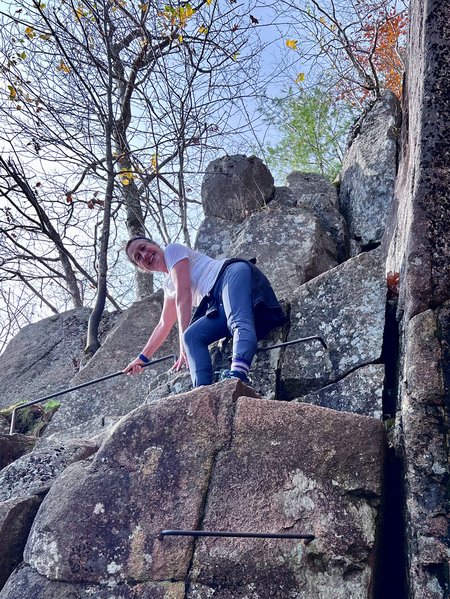
Interview Highlights
- A Chemist Who Does Biology
- The Role of Theory and Bioinformatics
- Startups and Sensing Technology
- Protein Structure in Historical Perspective
- Building Probes to Discover Cell Function
- From Medicinal Chemistry to Organic Chemistry
- Sat Masamune and Synthesizing Molecules
- Bob Abeles at Brandeis
- The Chemistry Dream Team at Caltech
- Building a Lab Around Glycosylation
- Returning to MIT
- Steady Path to Pursuing Biological Questions
- The Strangeness of Polyprenols
- Predicting the Glycome
- Generational Pressures and Possibilities
- Seeing the Life in Proteins
- The Quest to Integrate Scientific Knowledge