May 6, 2022
Carl Tape knows first hand that Alaska is one of the most interesting places in the world to conduct seismological research. A pioneer in the development and application of techniques to create images that tell us more about the internal structure of Earth, Tape's focus has been at the cutting edge of both computational and observational advances to establish more accurate representations of earthquakes, in Alaska and around the world.
Tape provides a recent perspective of the Seismology Laboratory; he completed his graduate work in 2009 working under Jeroen Tromp on building computer code to enhance seismic imaging. Tape recalls that he had a well-developed sense of the rich history of the Seismo Lab and now past advances informed his research. After a postdoctoral fellowship at Harvard, Tape was happy to return to his native Alaska, where he joined the University of Alaska Fairbanks faculty in 2010. His honors include the T. K. Moore Prize from the Geophysical Institute.
Interview Transcript
DAVID ZIERLER: This is David Zierler, Director of the Caltech Heritage Project. It's Friday, May 6th, 2022. I'm very happy to be here with Professor Carl Tape. Carl, it's great to be with you. Thank you for joining me today.
CARL TAPE: Happy to be here.
ZIERLER: Carl, to start, would you please tell me your title and institutional affiliation?
TAPE: I'm a [pause] Professor of Seismology at University of Alaska Fairbanks. I paused there because I'm still confused whether I can add anything beyond professor. But I'm a Professor at the University here. [laugh]
ZIERLER: [laugh] What might be some other titles or affiliations that gave you pause?
TAPE: I think you have a choice of what you want to be. We don't get told what it is we're professor of here. [laugh]
ZIERLER: [laugh]
TAPE: I'm just Professor, but I think seismology best reflects what I do. I'm in the Geophysical Institute but I'm pretty devoted to the discipline of seismology.
ZIERLER: Carl, tell me about the Geophysical Institute. How big is it, and how far back does it go?
TAPE: Great question. Historically, it was founded by an Act of Congress in 1946 here, kind of separate from the university but under a recognition of the strategic importance of the Arctic for the US. Alaska wasn't even a state at that time. It's an interesting history, kind of centered on space physics. Space weather is a big deal, and seismology intersects in strange ways. The first seismic station in Alaska was at a magnetic observatory that had been run by Russians in Sitka, and so you see where seismology, over the long-term, has come in and followed some of these fields that, at that time, were considered more important: space weather, geomagnetic storms. But seismology has come into its own with the nuclear era, basically, in the '40s. I'm already on a tangent. But that story starts with the Trinity Test, and Beno Gutenberg ["Interpretation of records obtained from the New Mexico atomic bomb test, July 16, 1945," Bulletin of the Seismological Society of America, 1946] telling them [those who executed the tests] that they're [seismologists are] able to—he got the origin time of the Trinity Test to within 2 seconds, and they [the government scientists] themselves didn't even know, to within 15 seconds when they detonated that bomb.
Seismology in Alaska
ZIERLER: Carl, being in Alaska, has that changed your research agenda, just by virtue of your location now?
TAPE: Big time. For starters, I grew up in Fairbanks, so I'm one of the only people at this institute who's from here. I'm sort of this anomaly. It's not a big town, so all the expertise is generally coming from outside the state. It's not a populous state either. But that was one of the things that drew me back here was the opportunities. It's sort of a frontier for undiscovered things, and it attracts a different kind of people to come and work here. As 84% of all earthquakes in the US occur in Alaska, they don't have the same significance, of course, due to the population. But there are lots of things to study that don't get a lot of attention. The main reason I came up to take this job was actually due to high-performance computing resources, which is odd because my family [dad and brother] was here but I tell them, "Sorry, but it was actually the resources that were most appealing"—
ZIERLER: [laugh]
TAPE: —"and the data opportunities."
ZIERLER: Why are the computational resources so significant in Alaska?
TAPE: That tracks back to a couple of things that are significant to me because coming to Caltech out of a master's [from Oxford in 2003], I was drawn into these studies going on at Caltech in 2000 and 2001 [specifically: Komatitsch and Tromp, 1999; Komatitsch and Tromp, 2002ab]. That [computational seismology] founded my PhD studies, and the resources, the laboratory, the tools I need to do science. At the time, back in the '90s, Alaska had been given some sort of appropriation under probably Ted Stevens—Department of Defense-related work where some fraction of the high-performance computing resource could be used for academic purposes. When I was in the job market in 2010, and as it is today, the question of what kinds of resources are available to faculty, for me, and many of us in seismology … computation resources are part of our laboratory. That was factor for me to be able to come to a place, and not have to wonder, "How am I going to do my work or am I going to have to shift my studies?" I did this partly when I came to Alaska. I started doing major field experiments, because I had to diversify as a faculty member, putting out seismometers [in parts of Alaska]. But my comfort zone is really: "Let's model the data that are already there." There's just tons of data out there that haven't been explored, and [we can] discover what kind of secrets they're holding. For that, the computational modeling helps us get at the understanding of these large data sets.
ZIERLER: Carl, you mentioned modeling, you mentioned field work, so that begs the larger question. Where in your research agenda is it about the theory, and where is it about observation and experimentation?
TAPE: In some ways, [considering] the complexity of some of the wave propagation solvers at Caltech when I was a student, the question is: "What questions about the Earth warrant the sophisticated resources?" Some parts of the Earth are very simple, and they don't need complicated resources. But the parts I'm interested in, for example, wave propagation and amplification of sedimentary basins like Los Angeles or the Seattle Basin, pretty much along the West Coast, the Great Valley of California, Bay Area—these places are geologically very complicated [which have] created these really complex and interesting structures from the mountains to valleys. There's bowls of sediment kilometers deep, miles deep. When the earthquakes happen, they [basins] trap these waves, and they make very strong societally relevant effects. It's those problems that warrant the resources that we need to study, so I would call that on the sort of theoretical side that we're trying to accurately represent how waves propagate in really complicated parts of the Earth.
When I came to Alaska, there are certain parts of Alaska that had never seen seismometers. This EarthScope Array had just come to Alaska. [The EarthScope Array was deployed starting in 2014 and completed in 2017. Most of these stations were still active as of 2022.] It sort of rolled across the United States, and then went up to Alaska. But the two main projects I did, I put seismometers out in places that many people would say that's really bad data. It's a bad place to put a seismometer. What they mean is that, for a lot of scientific problems, you wouldn't want to put a station there. For a lot of simple problems, you wouldn't want stations in the Los Angeles Basin. There's all kinds of people and cultural noise, and there's this deep pile of sediments that really makes it complicated. But that's exactly what we're interested in. In the Cook Inlet Basin in South Central Alaska in the Anchorage region or in Central Alaska, we went to some really hard-to-reach places, and I mean helicopter, plane, boats, by ocean, by river. We went to places to get these seismometers out to give us data set to help us later on down the line to understand whether we're getting it [our modeling of the ground motion] right. I can make up something [such as a model for a sedimentary basin], and try to understand wave propagation in a complex part of Alaska, but I really need data. I need seismometers to tell us whether that's correct. That's how I went into the field part [of my career]. Then the other big change for my career since my PhD was really toward the nuclear monitoring side of trying to characterize sources for these nuclear explosions. That coincided with, for better or for worse, I guess, North Korea actually detonating quite a few nuclear tests, culminating in 2017 in September with something on the order of a 5.5 earthquake size but as a nuclear test. That avenue has been interesting for me because it also helps us understand a lot of exotic other events that are in this non-earthquake category, like landslides, mine collapses, glacier events, processes at volcanic settings. All these things warrant a little bit different view, and it's in this sort of exotic category that nuclear events fall into.
Climate Change Impacts
ZIERLER: In the exotic events category, climate change is a big deal, obviously, in Alaska. Are you seeing things with glacier melts for which a seismological observational component might be important?
TAPE: Yeah, this comes in. There are a couple of examples. The sea ice extent is one that was sort of documented in Alaska. As the sea ice comes and goes, you can look at sort of just the ambient background noise of the seismic signal. The Earth is always in continuous excitation. That's one of the fundamental discoveries of the last sort of 20 years or so. That we can use that information in sort of environmental monitoring. We had, for example, one project [Kyle Smith and Carl Tape, "Seismic noise in central Alaska and influences from rivers, wind, and sedimentary basins," Journal of Geophysical Research: Solid Earth, 2019] where we could monitor the break-up of river ice, the wind, the changes in wind, [and the river conditions]. But in terms of glacier processes, yeah, you see glaciers. There's tons of activity: glaciers calving, surges of glaciers. But I will say, to be fair, the question is: "What's the best instrument for that information?" Seismometers are already out there, so it's great if we can get a little more information, and we do tend to detect some really big landslides that have happened that went undetected until maybe a pilot looks over and notices this huge landslide, and someone goes back and sees there's a seismic signal from that. But I think the opportunity with global seismology is just that it's always recording, and so there's always opportunities for environmental monitoring at basically no extra cost. Across Alaska right now, the stations have meteorological packages attached for the weather. There's holes in the ground to monitor permafrost ground thaw [using thermistors]. Those infrasound sensors could pick up volcanic eruptions, or even the [January 15, 2022] Tonga eruption could be heard across Alaska. There's this push toward like multi-instrumentation, and climate is certainly one of the motivating factors. With the EarthScope arrival across Alaska [in 2017], we got a better weather map of Alaska by a factor of 10 probably, number of stations, just the simple wind speed and temperature, by putting power in remote parts of Alaska where there never was a power system. I see environmental monitoring as a big and growing field. I do push back just to say, "That's cool but is that the best instrument for that particular thing, or can I just get a satellite image to tell me where the sea ice is?"
ZIERLER: Carl, you mentioned North Korea. Now, that it seems that we're on the verge of a new Cold War, whatever this new tension is with Russia, do you see an increased need, even an urgent need, for a seismological approach to nuclear verification with regard to Russia?
TAPE: It seems logical, [given] that the Cold War that drove the field of seismology forward was primarily two countries, one versus the other. While a lot of the recent support in what would be called geophysical detection of nuclear proliferation—and that sort of realm of study's been driven by the sort of newer players into these fields, like thinking about Iran or India, Pakistan, North Korea—those have been the focus of a lot of studies in the past decade or so. It seems kind of obvious that we [would] want to continue our interest in documenting, for example, the historical tests that have been done in the former Soviet Union—and work does continue on that. The recordings from those legacy or old tests are still important because they provide information about how waves propagate from a specific point in the Soviet Union where there was a test, to these global [seismic] stations. As you can imagine, we don't have tons of recordings from within the Soviet Union, so we're often looking from a long way away, and we're often looking at these older tests. Just to say as an example of why this matters, at the university in this building is what's called a University Affiliated Research Center. There are about 18 of these in the US. They're sort of funded through Department of Defense. The one topic for here is called geophysical detection of nuclear proliferation, so that's also where some of my interests have been pulled a little bit toward, just on these kinds of problems based on the sort of focus of that research here.
ZIERLER: Carl, all of the industries involved with resource extraction in Alaska, are you involved in a consulting basis at all with what's going on there?
TAPE: It's an interesting question. I would say for the university as a whole here in Fairbanks, where we're the center of the University of Alaska system, we do not have a history or ongoing strength in that realm. We have geologists and we've had funding in the past related to very interesting structural geology mapping of the North Slope. I think something like 10% of US production of oil is coming from Alaska. [This number peaked at 25% in 1988 and was down to 4% in 2021.] In this realm, we [at UAF] don't have an academic connection [in seismology]. If you look at sort of Colorado School of Mines or Stanford, a long history of sort of connections with oil industry, both support and then sort of creating and training graduate students for those programs. But even within Alaska as a whole, even Anchorage, for example, there aren't the research entities. There's sort of business headquarters—BP, ConocoPhillips—but if you have an intern doing research in these realms, they might get sent to sort of Houston where the research centers for those entities are. It's not just not Fairbanks but even in Anchorage, you don't have sort of research centers. They're smaller-scale operations. We have techniques that intersect with relevance in the industry. But, currently, no, I would say, strong academic. There's no consortium or anything. Similarly, by comparison, Caltech has received funding for certain things in the past but also, I wouldn't say, has that kind of connection [with the oil and gas industry], whereas School of Mines or Stanford would be more on that focus.
ZIERLER: Carl, a few nomenclature and technical questions as it relates to your research. First, how do you use seismic tomography to pursue the questions that are most important to you?
TAPE: Seismic tomography, this idea of finding something about the subsurface or the interior structure of something without directly going there. I like to think that the direct version would be probing into someone's body directly to get information, which we try to avoid. We don't want to go into someone's body. Similarly, going into the Earth at the shallow level is drilling a well. Drill directly down at sort of $20 million apiece. Just go make some holes and see what's down there. That's expensive, and so you also want to avoid that. Both of these things motivate trying to get information indirectly by sending waves through and measuring them as they come out. That's what tomography is. We've got sources, which are usually earthquakes, and then we've got stations or receivers that record these waves. For me, the big breakdown in seismology is source and structure: trying to understand the structure of the Earth, and also trying to understand these earthquakes, and both of these are very interesting to me. With tomography coming out of my research at Caltech, and where we're headed now [in my group], we're interested in understanding the structure, meaning, the variations and variability beneath our feet. My own interests are in the crust, the upper 30 kilometers of Earth where a lot of interesting things occur in terms of, yes, that's where oil and gas is, and these sedimentary basins. It preserves the tectonic history of how the Earth came to what it was. This, you could argue, is just a curiosity-driven question. But if you look at the motivation for the EarthScope Array, this biggest-ever rollout of stations across the Lower 48 and then to Alaska, the motivation was [to understand] the evolution of our continent. Like, how did we [the North American continent] come to where we are? When people start to probe "why do we care," it gets to the level of, well, think about space exploration. We're not really looking to colonize other planets and extract minerals from other planets. It's just curiosity-driven and human nature. Why are we here? How long have we been here? How did this place get to where it was? For me, those are the driving questions. I'm not primarily driven by societally relevant questions. I like to pursue them when they're right in front of you, and we steer our priorities based on the needs of funding, and the training that's offered out there. But ultimately, you have your own curiosity, and you don't really change that. You can go one way or the other, but that's why we're in academia, to have the freedom to pursue the things that we think are interesting or things that we think we can contribute toward.
ZIERLER: Carl, you mentioned earlier seismic wave propagation. When is that technique or interest complementary to tomography, and when is it separate? When is it its own field of work?
TAPE: I think immediately to Caltech science. Wave propagation is what we would call forward modeling. It means I tell you what the Earth and the earthquake looks like, and you propagate the waves through that with an earthquake and through this structure, and that's going forward. That's telling you, "Here's a prediction of the wave field." That tool, when it gets buried inside of some other what we would call the inverse model, where you're going from information that's recorded, and you want to improve the state of the structure of the Earth and the earthquake that you think it is, you tell it, "I think the Earth looks like this," but you're going to use data to actually improve that description. Tomography—the way we do it and the way it was trained at Caltech—was that it uses as a workhorse seismic wave propagation on high-performance computing, meaning, the most accurate numerical methods that we have for propagating waves through a sort of computer-described version of the Earth. That is a tool. It's the workhorse for the way that we do seismic imaging and the kind of techniques that were pioneered at Caltech.
ZIERLER: Carl, because you do both 2D and 3D modeling codes, why are both advantageous? In other words, why is it simply not 3D is better than 2D?
TAPE: Having become a professor, and even looking back on being a student, what really matters is that certain concepts can be illustrated in two dimensions. Everything [in 2D] is way simpler, and so when I want to train a student, we're preparing for workshops with the codes that we use, we stick with two-dimensional because you only want to use these sophisticated tools [like 3D] when the problem warrants it. When you get to a real application in a part of the Earth that is inherently three-dimensional, meaning, there are parts of Central Canada where you might even say Central North America, it's mostly a one-dimensional Earth, meaning, the variability, it's just layers of model. One-dimensional would mean that the variability is only in one dimension: up or down. We can do a lot of understanding in amazing things with using one-dimensional wave propagation solvers. Then you might get to a place—like, here we are in a subduction zone in Alaska—and in certain parts, one plate's going down but, laterally, it's very similar. I could sort of model things as just this slab going down because, in and out of this picture, things are pretty similar. But when you get to South Central Alaska, Southern California, there is no 2D. Every direction, it's a train wreck. We joke that it's called a terrane wreck—
ZIERLER: [laugh]
TAPE: —because there's lots of terranes in Alaska that are like crashing into each other to form this tectonic picture. There's actually a Ray Troll painting of this that's really great. When we have 3D, when you have real data 3D, that's when you need the 3D techniques. You have to be cautious because the concepts—you don't want to learn a lot of these things in 3D because it could be 10 times slower, and you can't even visualize things well. Like with all problems, you sort of step your way into it. But I think you want to be aware that there is this high-end tool. For the real answers, and sort of what we would say quantifying the uncertainties of what you're trying to say, you want to be using the most sophisticated tools, and the largest and best data sets possible for those problems.
ZIERLER: Carl, I've seen the term "tensors" used generally in math and physics. In seismology, a seismic moment tensor, what is that?
TAPE: Well, the simplest statement is it's just a 3x3 symmetric matrix. The word "tensor" is a description that's actually a physical quantity that's invariant under a different choice of the coordinate system. I like to think, like, this pen, as I point it in the air, this is a vector. It points in a certain direction. If I choose a coordinate system right here, and then write down the coordinates of this, it might be 1, 2, and 3. But if I rotate my fingers, I've got a new coordinate system, and this little pen, its coordinates with respect to this coordinate system, the numbers change. But this thing as a vector does not change. That's kind of the concept of stepping up one dimension [from a vector to] a matrix. You have a moment tensor, which is a quantity. It is invariant of these choice of coordinate system. But the way I would say it is it's a very simple representation of a seismic-like source. It could be an earthquake. It could be a nuclear explosion. All intents and purposes, it's a symmetric 3x3 matrix, which means there's just six numbers that describe it. You can break that down even further to say the magnitude is one [parameter], and then what we'd call the source type would be two other parameters. The other three [parameters] describe the orientation of this thing in space, which is three angles. You've got the orientation, and you can think of a fault in space. You need to describe the orientation. Then the source type tells you I could have a fault, and it could be opening like a dyke. It could be slipping on this plane. It could be slipping back, forth like that. Then there's a certain size of the event. These six numbers, you go from moment tensor to 3x3 matrix symmetric, to six numbers, to this notion that is more familiar, which is like this orientation, and then basically the type of event, and the size of the event.
ZIERLER: Carl, your observational work on earthquake nucleation, I wonder if you see that contributing to the societal need for earthquake early warning or even pursuing that holy grail of earthquake prediction.
TAPE: This study [2018 Nature Geoscience], there's a particular section in Central Alaska where we just saw a lot of bizarre things happening. Our great hope was that it wasn't anything special to that region, that there was some generalizing that we could find. If we looked in other places of the world, we'd find that the onset of certain events, there's this sort of like seconds-long buzzing activity that we can see on the seismometer growing before this larger event nucleates. It's extremely unusual to see this kind of precursor information. Earthquakes start with a bang, and there's no warning, not in the short-term, not within seconds. But in the last decade or so, some of these events, there have been some long-term growing precursors. But what we were looking at is just in the seconds before, seeing these sorts of insights into how a small event evolves and then slips into this much bigger one. In my own research, I haven't gone too much farther than this particular study that we published in Nature Geoscience. But I do think it's a realm where we need to keep studying. One of the challenges is we can't get instruments super close to the start of earthquakes. The sort of like insights into how on these really complicated sections down, deep down in the Earth, there are stressors, where and how these thing start. Part of our challenge is instrumentation that this SAFOD, San Andreas Fault Observatory at Depth, the whole idea there is drill a hole—millions of dollars—drill a hole down and then across through the fault, and really get right in the realm of where these earthquakes are happening. I don't think that's like the practical solution to earthquake prediction, but it would certainly help to have more observations realistically right at that depth. I think a great realm that goes alongside these observational studies is laboratory experiments. Rosakis at Caltech [has done] terrific stuff in the past, simply looking at how two little frictional contacts in the laboratory represents the Earth. That's a big step. But there's a lot to be learned about how things can start. If we can't understand how things start in the laboratory, it's going to be really hard to understand in Mother Nature how things start.
ZIERLER: The counter there, as opposed to going down and drilling and getting right to the source, is this idea—I'm sure your familiar with it—that earthquake prediction is essentially futile because the Earth itself doesn't know when an earthquake is going to happen. I wonder what your area of expertise, what your own perspective is on that.
TAPE: I should have a ready-to-go answer on earthquake prediction. I teach 100-level GEOS120 here. But, usually, I always steer the discussion toward the point that we don't understand the physics of how, with these two frictional contacts—you could be talking about it at molecular level—of two materials in contact, how you can measure those stressors, and then know when they're going to fail. It's really like a material science friction aspect. To be honest, [with] a lot of work in seismology, we're looking at the effects of what happens after the earthquake happens. We're just looking at the waves that emanate out from that. There are some seismologists who are pursuing, like: "Let's drill straight down into the fault zone after Tōhoku." Emily Brodsky, I think, another Caltech alum, led this effort in Tōhoku. Let's drill down after the magnitude 9 before this thing heals. Let's get some information about what just happened on a magnitude 9 that slipped 50 meters. Think about that. That means relative to two sides, a block of land under the ocean the size of probably like Washington or something sliding 50 meters. It's a staggering thing, and so you're going to have this sort of frictional melt of rock and things like that.
I think seismologists have occupied this wave propagation realm, and getting clues to what's happening. But when you really get down to it, and try to understand the stressors, and it's frictional—friction is a very complicated field of science that touches into outside of what I would call seismology. That's a long answer. But prediction, the sort of forecast of looking at patterns of tons of earthquakes, where they occur during aftershock, seismologists are good at that. Dots on a map evolving with time, are they related to each other? Are these aftershocks? Can we forecast where we expect there to be based on overall plate tectonic stressors in a region? But this term between forecast and prediction is a big difference. I think seismology goes to it a little bit toward forecast, saying, within this given time in this place, do I expect an earthquake? The Hayward Fault in the East Bay, San Francisco, with a long record of recurrence, that gets at the realm of very high likelihood in our lifetime: within the next 40 years or so, there's going to be a magnitude 6.5 to 7 on the Hayward Fault. That's why we put so much attention on that because that's not a guess. It's a good example of where we're sure. But on the day, what day is it going to happen? What size will it be exactly, like, how it's going to rupture, we can just sort of try to do our best to get what would be realistic scenarios. Give me 1,000 examples that are plausible on that fault. That's hard to convey to the public. Well, which one is going to happen? I don't know. Here's 1,000. That's our best guess. You can explore all the effects from those 1,000 earthquakes, and that's probably our best hope for trying to convey to the public. We have to be able to convey this notion of variability. Even with regard to when and how it's going to happen, we can get a pretty good idea on, I think, what the shaking effects would be, and that's partly driven by geological evidence in the past too.
Progress on Earthquake Prediction
ZIERLER: All of which is to say then you're not totally unconvinced that earthquake prediction is an impossible pursuit? That there might be some availability in the future to get better at earthquake prediction?
TAPE: Definitely, some progress could be made. If someone said, "[Here are] Unlimited resources, how would you attack this problem?" [I would devote] a lot on the modeling side, massive on the instrumentation side, but just get information to try to understand. Then maybe focus on—early warning is a sure thing. That's a practical [and] useful thing. But we're really getting at prediction. In the past, understanding that plate tectonics never stops. It's probably been 800 million years of plate tectonics. We're certain that there are locked faults building up stress, and that they're going to go. That's a huge success of seismology and plate tectonics to say we know for sure that the San Andreas Fault is going to rupture. That's why there's so much talk about it. We shouldn't take that for granted. There were times for the Cascadian, the Pacific Northwest, up until the '80s, people thought that was potentially just a quiet subduction zone with nothing happened. Actually, Tom Heaton [from Caltech] played a big role in that. They were trying to build a nuclear reactor right up in Olympia area [Satsop]. If you drive past this region, here's an unfinished nuclear reactor as a reminder of the value of sort of seismic studies in these areas. They've figured out there was a magnitude 9 earthquake or so in 1700, and that stopped building nuclear reactors in those areas. It's not prediction but it's an understanding that there will be a big event. Then, from there, you're trying to sharpen your focus on what you mean by to forecast—there's scenario possibilities—but then to this notion of prediction as in the next day or the next week, there's going to be something. Volcanic monitoring is the same idea. They're in the dark. Sometimes, a volcano will blow out. In other times, there'll be huge swarms of activity, and nothing. [laugh]
ZIERLER: Carl, all of your work on earthquake simulation, I wonder if you've been following all of the excitement about how quantum computation is going to revolutionize simulation. Have you thought at all about at some point in the future, what the relevance of this technology might be for earthquakes?
TAPE: I have not followed this well because my feeling is that the tools that we are trying to deliver to the field of seismology, and the graduate students today, we still haven't delivered them in a way that makes it easy to do science. We have an NSF Cyberinfrastructure project aimed at making a platform where users can come in and just use the tools that are high-end sort of in our field: 3D seismic wave propagation; the seismic moment tensor estimation. I'm coming from a little more conservative approach to these very new things [like quantum computing] because, frankly speaking, I have a certain skill set and want to have the biggest impact. Going into a brand new realm is probably not the best use of my time or skills. Another example would be DAS, distributed acoustic sensing, where Zhongwen Zhan [at Caltech] is playing a leading role in that field. That's a new and very different thing to seismology that some people would say is going to revolutionize seismology. Zhongwen gave a talk in Seattle just a couple weeks ago on all the environmental aspects that we can help inform with this kind of technology. There's a lot of capabilities that are not going to become obsolete, in my view. GPU computing would be an example where graphic processors, thanks to all these video gamers probably [laugh]—
ZIERLER: [laugh]
TAPE: —had the technology that we could exploit for our own code. This is also coming out of the code. The SPECFEM code out of Caltech, it was one of the earlier codes written to harness the GPUs, and you could argue, yeah, that speeds things up immensely. But when you really talk about like the bottleneck toward training someone to do science, it's not always about how fast the computer is. It may be even whether you can get support on that system, or whether you can train to learn the concepts fast enough. There are these moments where there are big changes to fields that happen, and I think with regard to like these wave propagation solvers, they haven't reached everyone. They haven't seen their potential, basically, where you want everyone to be able to use them without spending two years of a graduate program.
ZIERLER: Carl, just as a snapshot in time, what are you currently working on?
TAPE: Right now, in a nutshell, I am returning to the work for my PhD in Caltech: I sort of stepped away or broadened my interests starting in 2010. Now, we're coming back, stronger, to my origin work, which focuses on seismic imaging. It's been a while. I have four postdocs and four students, and we're focusing around problems related to seismic imaging. We can go from nuclear monitoring realms of seismic imaging to Alaska as a major focus for all of us, understanding both source processes in Alaska—there are faults that are active that haven't been characterized—and understanding the structure of Alaska. Most of our efforts are going that way. But there are other questions on the theory side, like, understanding descriptions of the Earth's structure, what we would call elasticity or anisotropy. But there's a lot of unknown things. We have a simplified view of the Earth, and we'll explain how waves travel through them. But there are more complicated versions. If you pick up a rock, and measure it in the lab, like a piece of shale or a piece of sort of upper-mantle rock, like peridotite or something, if you measure that in the lab, it has a lot more complexity than we would characterize with the Earth. Generally speaking, going back into seismic imaging with a major focus on Alaska, but also really interested in source characterization for earthquakes, nuclear events, also using seismo-acoustics. When something explodes in the atmosphere, earthquake on the ground, there's what's called coupling, so waves going within the atmosphere and back down to the ground. A seismometer is picking up signals from the air, and you can see with regard to like volcanic eruptions.
The Tonga eruption was a really exciting [case study]. I have a postdoc with a paper looking at the seismic characterization of this spectacular eruption that occurred in Tonga, and was heard in Alaska [laugh] audibly. It's the farthest distance anything had ever been heard on the planet. There's no record of anything like this before. What's really exciting is that in the observational realm, stuff's always happening that's never happened before. When I try to motivate students about why we study seismology, there are sure societally relevant things: insurance rates; oil and gas; nuclear monitoring. These things are relevant. The Earth is always providing surprises, whether it's this volcanic eruption. It's like each of these earthquakes has a story to it, and you try to find a little bit about what's different. This nucleation story we had, it's just amazing what's there. It's not like earthquakes just happen and we're telling the same story over and over. Some of that is needed for the record. But there are just so many oddities that the Earth produces. You think about the stuff of like underwater species that we see in the Earth. You go to a deep-sea trench, and there's these bizarre things. That's what I think about with earthquakes too. I don't think the public sees it that way.
ZIERLER: [laugh]
TAPE: But there's a lot of diversity of activity that we can pursue.
ZIERLER: Well, Carl, let's now go back to pursue your educational interests before you got to Caltech. When you were at Carlton, were you interested in geology, seismology, and geophysics even that early?
TAPE: I would say yes. Growing up Fairbanks, the biggest earthquakes to date that I ever directly felt—and I mean the biggest shaking (I've felt larger earthquakes from farther away)—was a 1995 magnitude 6, and I remember that caught my interest. I went into a liberal arts college knowing about earthquakes and some applications of physics to the Earth's data. I had a physics and geology pursuit. Physics was more natural to me. Geology was just an opportunity. I thought, well, the difference of actually getting a degree was doing an in-depth project, which I did on tidal-deposition of Cambrian—like, 500-million-year-old—sediments in Minnesota. That's just a cool problem. The idea that Minnesota preserves a record of the daily tides in 500-million-year-old sandstone, if you're not interested at all in that, we're going to have a hard time conversing—
ZIERLER: [laugh]
TAPE: —because it's curiosity. Right in front of you, here's an outcrop. You can measure it. Thinking about that relevance of geology to tides, which is physics-driven understanding. I had no real exposure to seismology [at Carleton]. But I was very fortunate to get a Rhodes Scholarship, which is to a university. Within that application, I had said, "Well, seismology would be a really natural place for me to go." I had done a research experience in undergraduate in atmospheric monitoring, which involved me like cleaning fiber optics and polishing them all summer long. I thought, well, that may have not been a fair entry point into that field. But I ruled that [atmospheric monitoring] out, and I thought, I'm going to go into the solid Earth. Oxford had a great program in seismology. Not a lot of earthquakes over there but, theoretically, they had great folks there, and that's how I went into seismology. I didn't have proper computer programming training. This is in 2001. But it was a great two-year introduction. During that two-year period, I learned seismology enough to where I saw these papers come out of Caltech. It was that moment where I saw these papers, and I just thought, "Holy crap, I can't believe what's going on. I had no idea this was possible." These are papers by Jeroen Tromp and Dimitri Komatitsch—that sort of marked a pivotal era, I would say, in Caltech. I applied to Scripps and Berkeley and Harvard and Caltech. In the end, it was Caltech and the singular academic focus [of computational seismology] that was going on there that drew me there as a PhD student, which I started there in 2003.
ZIERLER: What was going on at Oxford when you were there in seismology and geophysics? What were the big issues?
TAPE: Well, the folks there, the main people, Shamita Das on the earthquake physics side, and John Woodhouse, who had basically established seismic tomography—a seminal work in seismic tomography came in the '80s with John Woodhouse and Adam Dziewonski, [both] at Harvard. I didn't entirely know that, except when I applied, I started needing to learn about this, and started to understand the origins [of Woodhouse's career]: super strong theoretical background, understanding the normal modes. When the Earth has an earthquake, there are oscillations of the Earth, all kinds of interesting oscillations. That came out of the late '70s and was used in the '80s.
[Applying to Oxford in 2001,] It wasn't like when I went to grad school [in 2003 at Caltech], I said that's something I'd like to work on. But I didn't have the [seismological] training [in 2001]. As you can imagine, two years in a master's program, you get a better idea of the field and what is of interest to you, and what's kind an interesting new direction. As an undergrad, I had no idea. I just looked at the background. The theory sounded very interesting. We didn't have a spelled-out project or need. But we looked at wave propagation on a spherical membrane. Going back to this idea of 2D versus realistic, it would be called a toy problem, even though it didn't feel like a toy problem. It felt pretty challenging. It was sort of understanding how, instead of ray tracing on the sphere to represent surface wave propagation, we had wave propagation on a membrane, which is still an approximation for waves. This is why when I saw the work being done at that time on Caltech, I thought, "Wow, this just jumps light-years ahead in terms of this sophistication of the technique, and it'd be really cool to be part of that." John Woodhouse is a legend in seismology, so that didn't hurt. I'm sure it helped me to get into grad school. But it also helps to be able to articulate why you want to go work with someone rather than just saying, "I want to do seismology for grad school." No, I want to learn what this spectral element solver is all about.
Appreciating Seismo Lab History
ZIERLER: Carl, your appreciation for what was happening at the Seismo Lab was very contemporary, of course. More broadly, did you have an appreciation for the Seismo Lab's history, that there was this culture of innovation going back decades?
TAPE: Yeah. As I've left Caltech and really—I have more than a hobby interest in history. It's also interesting to look back at the time when I arrived of where that fits in. But, just to say, the moment when the computational side, when Jeroen Tromp and Dimitri Komatitsch teach you as a postdoc actually rigged together and linked these computers in what is basically an air-conditioned room [laugh], probably had no business putting that in there—
ZIERLER: [laugh]
TAPE: —I know physically where that space is in the Seismo Lab. That was the kind of leap that you're talking about. It's someone taking an ambitious step forward. When I got there, I had this opportunity to see that old history. I took a class in that fall, 2003, with Tom Heaton on engineering seismology. He said, "Hey, does anyone want to go see the old Seismo Lab? They're going to sell it. They can't afford to hold onto it. Real estate is too high." We walked over toward the mountains where the PAS station was. You could see paper records, photographic records, old instruments. Gutenberg and Richter's offices were labeled. It was this moment, the tail end of seeing what that past physical space was. If you're a seismologist, this was a shame [to lose]. If there's ever going to be a seismology history museum, it would be that place with names like Gutenberg. When I looked on my shelf, this is Earthquakes in Kern County. This is 1952. If you thumb through this book, it's got Benioff, Gutenberg, Richter. It's basically the history of the Seismo Lab tackling this 1952 magnitude 7 earthquake on the White Wolf Fault. That was a gamechanger earthquake for seismology. There's stuff in here that's fundamental to the field of seismology about understanding, for example, aftershocks, the idea that aftershocks give you an idea of where the main earthquake broke. That kind of stuff just we take for granted now. The other one was Seismicity of the Earth. This is like the Gutenberg-Richter global seismology, 1950. It came out in 1949, and then was republished in 1954. I think Gutenberg died in 1960. I took Hiroo Kanamori's last class on physics of the earthquakes. He probably taught it for so long. I feel like I was at the tail end of some era. Jeroen Tromp's class on long-period seismology was two of us: Qinya Liu, who's a professor at Toronto now. The TA was Victor Tsai. He was an undergrad at Caltech, so it was me and one other student with my advisor, that's it. Victor became actually a Caltech professor. He's at Brown now. Then reading Richter's Scale, Sue Hough's book—it provides a nice context, just because some of these folks spent so long at Seismo Lab, and they're overlapping; that Richter made it to the '80s. But you never feel like you're part of history. I feel like I missed the history. But it's probably from your standpoint—
ZIERLER: [laugh]
TAPE: —you're thinking, "No, actually, things go on."
ZIERLER: Yeah, definitely.
TAPE: Something actually important happened while you were there. One thing I'll say is that, thinking back to the nuclear side, I heard Terry Wallace—who left Caltech, became high up at Los Alamos National Lab [he was its director in 2018]—I remember he came and gave a talk here [at UAF], and said that between 1950s and 1983 or so that 85% of seismic funding in the US, even though the National Science Foundation, was under nuclear-driven research, so that even if you were doing a project that you thought had no connection to nuclear Department of Defense interests, the funding was coming from there. Caltech, if you look, lots of funding has come through that avenue, especially into the '80s. I think what has been amazing is to meet those needs but then do these incredibly creative and divergent undertakings. That it's sort of like, yeah, we're supposed to do this but, for the greater good, we just need to train seismologists. We need more people in this field if this is relevant to nuclear monitoring. They don't have to directly look at a North Korea explosion, but they need to be trained in these capabilities. That's been an interesting sort of perspective change—not change. But just looking back at what actually got done through all these decades at Caltech, I think you have the people and their interests, but then you also have the intersection with some outside forces. I think that the nuclear monitoring is one that you will see more than just a theme if you look closely.
ZIERLER: Carl, given your interest in the historical context, once you got the lay of the land at the Seismo Lab, what aspects of seismology seem to you settled science that were no longer debated, and what was still really hotly debated in the field at that point?
TAPE: Well, for sure, the techniques of my thesis project were considered a frontier. On paper, it seemed like things should work, like seismic imaging with three-dimensional wave propagation. But even on my own committee, there was some resistance to it. "Is this the right use of time, or is this going to work?" That was exciting. When I talk to students or people say, "This may not work," I often say, "What better time to take a risk than as a grad student?" Now, if you asked the grad student, they're saying, "No, no, no risk. I just want three papers and get my PhD." But from the advisor, you got this line between if we knew the answer to this problem, it would be boring. That's true for a proposal, and some proposals, that's fine. It's a contract. I'm telling you what I'm doing. It's like, "Well, if I knew exactly that this would work, I wouldn't even want to be doing it." It's just we want to be challenged at some level. I see that some of the science is incremental and necessary. Caltech, I think, has the opportunity and innovativeness to always pursue as well as incremental developments but sort of big changes in the field. You can objectively take like Science and Nature papers that have come out of the labs over the last decades. But just looking at general fields, Rob Clayton actually in the tomography realm was a pioneer in seismic tomography in the '80s. When I was there [2003–2009], I would say computational seismology was one of those pioneering things. That's where I slotted in. But, at the same time, you have Don Helmberger, who's probably produced more seismologists into academia than any single person. Just on the forward modeling, but also look carefully at all the data. There's always something to be told. Don was all over the place with that general strategy. Then you have Hiroo Kanamori in my time; Pablo Ampuero came; Rob Clayton. I can sort of go down and think through all of these. But, of course, my experience is totally biased by my [laugh] obsession—
ZIERLER: [laugh]
TAPE: —of my PhD work, and being there. Really, two huge influences on me were people who came on sabbatical while I was there, which was Albert Tarantola, I believe, in 2005 or '06, a legendary figure in seismology. He has a book called Inverse Problems and Parameter Estimation. He came on sabbatical, and just such a passionate guy. You can see from his website. He passed away in 2010, I think. Caltech brings in these kinds of people, and that's a part of the history that's not as obvious, so sort of connectivity outside of Caltech. In the following year, Malcolm Sambridge came. For me, this sort of opportunity to connect with these people was really influential.
ZIERLER: Carl, given that you had some pretty well-developed appreciation for what was going on at the Seismo Lab, even before you got there, how did that affect the topics that you wanted to focus on and even who your thesis advisor would be?
TAPE: In my case, I was pretty singularly focused. I was aware that everyone's kind of a legend there. You get an idea of where they're coming from and where they are in their stage of their career. But I had come to work with Jeroen Tromp. I was impressed by these huge high-performance computing wave propagation codes. Truth be told, I don't think I even ran one of those codes until year four, four years in. That sort of shows you the scale. We're trying a new technique. Everything was on 2D wave-propagation codes, so my own trajectory was a high-risk, high-reward undertaking. I'm grateful that I had the opportunity to go at that pace. It wasn't like, "Hey, get a publication in your first year," and you're second year is, like, "No, we're going big here, and this may or may not work." I believed. You have to have some faith and trust both ways. The advisor's got—this person probably seems capable of trying this. I've sort of diverted from—what was the question? [laugh]
ZIERLER: The question was just your focus, how you decided what topics and what your advisor would be.
TAPE: I read papers that had been published, and I'd seen a couple talks, and I was sold on that direction. But, at the same time, I had expertise around me that if I could spend a 20-year PhD, I would've—
ZIERLER: [laugh]
TAPE: —come out an even stronger person for interacting with like Kanamori and Rob Clayton, Joann Stock. Mark Simons as well was one of my projects. [As first-year students,] we were forced to do two different projects with two different people, so you get exposed to something else as well. But, after that, it got pretty focused on just this singular goal of basically seismic imaging with these sophisticated tools.
ZIERLER: What was Jeroen specifically working on when you connected?
TAPE: Basically, this code SPECFEM was unveiled around 2000/1999. It means it's a code that is openly available, and has always been openly available, if it runs on high-performance computers, which allows you to take a very detailed, humongous problem like Earth's structure in great detail, and parcel out each piece onto a different computer. It's been run on I think like a million different computers, a million of them connected. Each one of them solves a mini problem, at each new time step shares some information, and advances this huge problem for it. It enables a different scale of problem. That's what Dimitri Komatitsch and Jeroen Tromp had physically built, connected these computers together, the code had been written, and so it was this tool that could do things that no one had the capability of before. That itself actually came out of Dimitri's thesis work in France. But Jeroen and Dimitri connected and took it to the next level of both the application and dissemination. Then the maintenance of the past 22 years now—that's still the global standard code for seismology, and it's a lifelong commitment that I think Jeroen had no idea [laugh] he was getting into. I think he said, "We'll do this, we'll seal it off, and send it off." But, you see, hardware's always changing. You have to adapt to hardware to have this tool functioning efficiently in the same way. Coming to Caltech was more of a straight line, but that's partly because I had this master's degree with someone—John Woodhouse—who has a like a Venn diagram of skills to someone like Jeroen Tromp.
ZIERLER: Carl, as you say, as you took this project on, it was high-risk, high-reward. What exactly was the risk? What were the prospects at the time? If it worked, what did you see as the reward scientifically?
TAPE: Well, the risk was just that it had not been done before, and it's sort of a risk in that there's so many other lower-risk, interesting scientific problems out there. Relative to other PhD thesis topics, and the skills that I had, I guess, there were other opportunities. But this one was, at that moment, there was a theoretical sense that this, on paper, should work. But when you get to real applications, there's messy things. Data are corrupted. Your problem is never set up in the way that you really want it to be. There's things you hadn't thought about … applications. It took us a long time that even when we demonstrated things could work in 2D, in principle, this should work, we demonstrated it on a paper, but then the question is, will this really work with a real problem? It works on paper. It works with a simple, made-up problem. That's where the risk—it felt less risky by the time we demonstrated it. But, in the end, we got somewhat lucky and published the paper in Science, so that was a great thing for my career, just from the standpoint of getting a wide audience there. But, really, it was just being able to demonstrate unequivocally that it works.
That's the beauty of the problem, is that this [new] model of the Earth is much, much better than this [previous] one. We can say that through all kinds of different ways. That's a much easier way to convince someone of the merit of the approach. I've always felt like there was going to be—that's what the high risk is. It's going to be out there. It either didn't work or it did work, roughly. If it didn't work, that would be very clear too. [laugh] There's all kinds of things that could be improved and done better. If I look back at my last years as a grad student, it was insane. On our [computing] cluster, 15% of the jobs would fail, the hardware wasn't functioning, there's hundreds of thousands of seismograms to look at. I was like living in the Seismo Lab. I was stereotypical—just so obsessed with that stage in my life. That was probably a good thing, and something I'll never revisit. But it was driven by, "Like, I think this problem's going to work." That sort of built upon itself where I wanted to be there. I didn't want to do anything else but that problem.
ZIERLER: To go back to this idea of curiosity-driven research, what were the big science objectives? What was the fundamental curiosity that drove this near obsession for you?
TAPE: It's fascinating because, in a proposal, you have to identify a part of the Earth that has an unanswered question. That was not the curiosity. Our curiosity was: "Can this method really work?"
ZIERLER: It's really proof of concept?
TAPE: It is. The Science paper is about Southern California, but the title is Adjoint Tomography of Southern California. It doesn't say, "We just learned the tectonic history based on a better image of the subsurface of California." Yes, we can say something about better imaging contrasts across faults. But the power of that discovery was methodological. For me, that just depends on who you are. Some people truly are driven on what's the history of the Midcontinent Rift in the Midwest. There's basalt everywhere. The continent pulled apart. What's that story all about? But, for me, it always gets toward a lot of speculation when you look at geological history and reconstructions. I want to say, "What's the snapshot? What is the actual structure of the subsurface? What are those uncertainties?" Someone else can take that [information] and try to interpret the history. But we all have different skills and interests, and I'm on the conservative side of making statements. I'd rather say for sure, "This is what we see." We can document this. But this is what makes the field fun. You've got a lot of differing ideas and approaches to understanding the Earth.
ZIERLER: Carl, given that the methodology was the central focus, and you needed a particular place on Earth to do the actual study, would the thesis have been different if you picked a different part of the world to focus on?
TAPE: Yeah. Southern California was critical in this regard. We needed both a good idea of what the best guess of what the 3D structure was. But, more than that, we needed really good data. We needed dense, reliable, well-studied data. I say "well-studied" because just because you have stations on the planet, if no one's looking at them, there may be some issue. You want stuff that lots of scientists are staring at day in, day out, understanding the nuances of a particular station, and Southern California was a great place for that. Just the number of earthquakes available—we need earthquakes to do this kind of imaging that are local. It's all local. We're not looking at things from far away, and so Southern California was a perfectly place for that—and remains a really good place for demonstrating this. Both societally and in terms of the complexity of the landscape and this geology, it warrants these techniques more than almost any other place.
ZIERLER: What was Jeroen's style as a graduate advisor? Was he hands-on? Did you work closely with him? Would you check in as you needed?
TAPE: I'm sure I saw Jeroen every week or so. He wasn't going to sit over my shoulder, and do any kind of coding. To be honest, the perfect in-between was Qinya Liu. She was in the group, and she's now in the physics department at Toronto. Without Qinya, I would've had [laugh] a tough grad school run. She was so great as a mentor. I don't know whether all students have that experience at Caltech. But you need this hierarchy within a group. You need someone to pass on the training because Jeroen would be the first to admit he has some idea but, in terms of implementation, he's operating at a managerial scale, such as, "Let's secure a [computing] cluster." No grad student's going to get the cluster. That's the laboratory. He's sort of, "Here's the big picture of how this ought to work; this thing should work." But then in the implementation of FORTRAN coding, visualization, all these things, that's at the grad student level. There's room within there to explore when it comes to like imaging Southern California and trying to figure out what we're seeing. That's all within my core. Jeroen's not going to be obsessing over the details in the tomographic image. Collectively, we could pull things together, but it definitely was more than just a student and an advisor.
ZIERLER: Carl, looking back even over the past 10, 15 years with computation advances, were computers at a place when you were a graduate student where they did everything you needed them to do, or were there obvious holes in the research just based on where the technical capabilities were at that point?
TAPE: With the resources in terms of desktop computer and then the cluster, those were not time-limiting factors. I think the science, like, the understanding, visualizing all the results was always limiting, and remains a challenge. Like, how do you look at 3D models? How do you look at tons of seismograms efficiently, processing data? One sort of major change since that time is something called ObsPy, for example, a Python-based infrastructure for handling seismic data. These are things that a new grad student has now. If you say, "What do you have that I didn't have?" Everything Python, open-source, and sort of a platform for data access, trivial access from data centers are now in seismology. You're starting off at a much stronger place. In terms of HPC, it's still not as easy as it should be. Nobody has automatic access to HPC resources where codes are ready to go. That's where this cyber-infrastructure project we have—it just got funded last year. We're still trying to bring certain things to the community. But as a grad student, you don't necessarily ask the questions like, "Where are things headed?" You're just like, "What's the problem at hand?"
ZIERLER: [laugh]
TAPE: "I'll make the most of whatever I have." Caltech had a lot to offer across the board in resources, expertise, data center, so it was an exciting place to be.
Risk and Reward in Thesis Research
ZIERLER: Carl, to go back to this continuum of risk and reward, at what point in your thesis experience did you feel more on the reward side, and less on the, "Oh, my goodness, this was a big risk, and it was a mistake"—it was not that? When did that happen for you?
TAPE: I had a couple rough thesis committee meetings, probably year 3 or 4. I started in 2003. I made a contribution to a paper. The paper Jeroen Tromp wrote ["Seismic tomography, adjoint methods, time reversal and banana-doughnut kernels," Geophysical Journal International, 2005], pulling things together, it was Jeroen, me, and Qinya, and I think there's 1,000 citations to that paper. It's the highest-cited paper probably of mine, for sure—maybe even his. I made a contribution at that level. I did all the 2D examples. It was terrific for me; still sort of like proof of concept. That was two years into my thesis. Then, another two years later—so, 2007 was my first first-author paper. We're talking about [being] pretty long into your thesis to be getting that first paper. At that point, like any grad student, you're thinking, "I'm over this big hurdle. I have one paper." I felt the confidence to at least just keep pushing away at this one problem. But my thesis was a straight arrow. [laugh] There was one side project that I published at the end with geodetic stuff, but [my thesis] was not a sort of a wander, Brownian motion [laugh], around to find a way. I think after we got over the major technical hurdles, and started just getting some reinforcement—like, on paper, this ought to work, and we're seeing things that ought to work—but I just went pretty cautiously because people hadn't done this problem at this scale before, and so I felt the worst thing I could do is try to rush it and get a bad result. I felt responsibility that this needs to work because if it doesn't work sufficiently this time around, no one else is going to try it. I may have brutal [habits], like scripting codes that you'd never want to follow directly behind, but in terms of demonstrating that it worked, it was important to me that it worked well. Not just it was working but it worked well to the point to say, "Yeah, this did tell us something new about the Earth."
ZIERLER: Carl, as you mentioned, as a graduate student, you're hyper-focused in the here and now. But, looking back, what were some of the ways that your thesis research was responsive to some of the broader questions in the field at that point?
TAPE: The seismic imaging, I feel like I was in an unusual situation. There were a couple of other groups. There's one at USC and one at Munich pursuing similar approaches, different-scale problems. Now, as a professor, [if] a crazy earthquake happens in Alaska, I'm much more opportunistic. It wasn't there [for me] at Caltech. You look at my publications. An earthquake happens and Seismo Lab just completely shifted: the Sumatra earthquake's a great example. That should be in the historical record, because the whole Seismo Lab just shifted to this magnitude 9 earthquake. Papers were going everywhere. Meanwhile, I'm just straight on, not distracted. [laugh] I missed those opportunities. It could've been a different story with a different project. But I have no regrets. I didn't have as many papers maybe, but in terms of like trying to push this part of seismology forward, we did a good job on that.
ZIERLER: What were your prospects after you defended? What was available? What was the job market like?
TAPE: To give the full summary, there was some sort of prestigious Lawrence Livermore postdoc across the whole lab I applied for. Did not get. But then I got an NSF postdoc to go to Harvard to work with John Shaw, who's a structural geologist with close connections to the Southern California velocity models and how they're constructed from oil industry data. You don't have to all do seismic tomography. You can take someone else's image, like direct borehole measurements, talk about drilling holes in the ground, direct measurements of the P-wave velocity of the density of the shear wave velocity. I was drawn into looking in that. The proposal was on looking at the Great Valley.
But life intervened. I met my wife, who was a Caltech grad student. We actually did a Joann Stock research cruise between McMurdo and Christchurch. Even though she was in the same department, I [had] never formally met her. [laugh] But we met on that geophysical research cruise. She got a PhD in geology, ended up doing a postdoc at Brown, which explains why I was so obsessed trying to finish my thesis work in my last year because Jeroen had left. He went to Princeton. She left [laugh], doing a postdoc, so I'm by myself just like trying to crank this thing out. The postdoc to Harvard made sense. We got married in 2007, and went to start a postdoc at Harvard while she was at Brown. She ended up teaching at Connecticut. All these sort of two-body problems that you can't tell when you read someone's CV. You're like, "Well, why'd you go there? What? Oh, you had a …?" Yeah, I had a kid in 2010. Ended up taking jobs at the university. Things went fast in the one year and a half years after leaving Caltech. I defended on April 1st, 2009, started a postdoc, we had our first kid the year later, and then the dual job opportunities came up at University of Alaska Fairbanks, where I never sort of imagined going back there because I figured it'd be too hostile to expect a spouse to [laugh] go there.
ZIERLER: [laugh]
TAPE: But, anyway, it is a nice place for kids. It's harder as an adult to get adjusted, I think, to this place.
ZIERLER: Carl, to go back to an earlier question about the way that being in Alaska has affected your research agenda, was that apparent to you immediately? Did you see an obvious connection of applying what you had focused on in graduate school and for your postdoc in the Alaska environment?
TAPE: Yeah. My first proposal I wrote was quite I guess you'd call it high-risk. I'm not sure about the high reward. But I said, "Let's look at glacial calving, events happening on the edge of glaciers, where you have topography. You have ice. You have rock. You have water. You have events that are ice falling into the water, exotic processes." I wrote a proposal [laugh] to NSF thinking, "This has just so many cool things going on." Never got funded. [laugh] It was then I started to realize, well, high risk is not desirable from an NSF standpoint.
ZIERLER: Right. [laugh]
TAPE: The next proposal I wrote was basically my thesis proposal, replacing the word "Southern California" with "Alaska." Sure, I could make that sound interesting, and motivated by different problems in Alaska. But if you looked at that, you'd say, "Well, this person clearly knows how to do this problem. They just did it for their PhD. Here's a frontier. Here's some data." That was the sort of starting point into larger projects. Then it [my career] went this field route of: "Let's go get data in these places where we don't have Los Angeles Basin-level instrumentation." Going to Alaska was sort of a natural extension. But that's the good thing about seismology. You can take your skills and, regionally, get drawn in wherever you are, whether it's the East Coast. But I'm totally biased that Alaska definitely has a lot of opportunities.
ZIERLER: Carl, I'm curious if you see your hire more as an ongoing building effort at Alaska, or were you brought in because there was a blind spot in what the faculty was doing at that point?
TAPE: I see that I am in Geophysical Institute. We started talking about 1947-48. We are here primarily for excellence in data collection. We have an InSAR satellite acquisition. There's satellites in the woods collecting data. There's field experiments all over Alaska, permafrost, oceanography, space weather, aurora studies. I think, historically, we're strategic in data acquisition. I came in with computational seismology, quite divergent from that, saying, "All this data's out here." That includes the Alaska Earthquake Center: 55,000 earthquakes a year with hundreds of stations, millions of waveforms being analyzed. It's bringing in someone to say, "Let's model the data. Let's take the data, and do something with it." I think that was probably a strong point of my arrival here, I guess, and not to mention just, "Hey, let's use the computation resource." No one was really using that, even throughout Geophysical Institute, I think. There may have been some monitoring wildfires [laugh] and smoke propagation. These are the kind of things that happen up here.
ZIERLER: Who are the kinds of graduate students that would be attracted to come to Alaska and work in your group or in the institute generally?
TAPE: I always encourage students to try to find at least two faculty [members] at a place that you could imagine working with. All our funding is—like Caltech would be—research RA funding where you have money for a project, maybe a few projects, and you're trying to match that with the interests of the students. I have really a lot of international students, similar to Caltech. I've had multiple students from India, Bolivia, Indonesia. I've had a Navajo student from northwestern New Mexico. I think it's student that are highly trained. The fact is, highly trained, technically trained students for things like computational seismology—just a math and physics background—occur outside of the US in large numbers. We have them also within the US, but [outside the US] there's certainly a predominance of very, very highly trained people, hungry for education at places within the US. That's been certainly characteristic of some of the students in my group. But I often say to people just like, "I'm from Fairbanks, and I went to Los Angeles for grad school." I always use that story to say, "This is a temporary situation. Yeah, it may be five years, but it's temporary, and you can survive." If you don't have a good academic connection, that's going to be a long five years." If things don't work out at work, I don't care whether you're downhill skiing, going in the mountains in the San Gabriels, you need that academic experience to be successful.
That's why I say to find two people. Make sure that project looks good. I'm telling you, I've got people from Bolivia and India. It's 40 below here. This is the coldest, darkest place you can imagine. It's not easy but I always will say, like, let's get the academic strong. You want to show up to work and want to show up to work. I loved LA, but it felt like, wow, I could never [live there permanently myself]. Just flying into LA, where I'm from, my heart's racing. But then what you realize is you walk three miles or you go up to the end of Lake Boulevard, and you go hiking up there in the San Gabes. There's no one there. You're away from everything in one hour. You feel like you're in the scruffy. There's going to be a bobcat or a cougar attack you up there. That's only the news. [laugh] Anyway, that's a long aside.
ZIERLER: Carl, between your graduate students' technical capabilities and just their long-term interests, what insight does that give you into where the field is headed?
TAPE: I'm pursuing things that sort of make the agenda within our group, and so I show that, and people can come in. But my big picture is just trying to expose people to the tools that will generalize: 3D seismic wave propagation, source estimation, looking at data, these things. You could go into different careers, non-academic. You could go into National Labs, USGS. You could go into academia. But trying to get skills that push the field forward. We've settled on a couple of these, being the seismic imaging, seismic wave propagation, and source studies, and trying to train people to be curious and look at when earthquakes happen, what is the story to tell there? But I don't have a long record. I've had probably a few—I don't know; I should know this number—maybe three PhD students and three master's students. It takes a while to build up and get something to be said about where people are headed with their careers. But, more than anything, especially for a grad student, for the pay you're getting, you want it to be fun. That's what I'd like to deliver. I'd like them to keep these skills and keep them in the field, but I want this time to be fun and challenging. You want people to want to show up at work each day. That's sort of the big picture for I'm sure a lot of managers. You want them to be there.
ZIERLER: Carl, for the last part of our talk, I'd like to ask a few retrospective questions about your time at Caltech and what it meant, and then we'll then look into the future. First, looking back, what did you learn at the Seismo Lab in terms of the approach to science, building collaborations, analyzing the data, that will stay with you for as long as you are active?
TAPE: For me, it was, for lack of a better word, like the energy and the environment, the intensity—it's seen as a negative word, and I see it entirely positive—the intensity of sort of science going on within the lab, but also the openness. Everyone will talk about the Caltech coffee hour. I was not a religious member. Partly, I just don't drink coffee, but even coming from England for two years, sipping a hot orange drink to socialize. I didn't integrate in the way that many people will say. The coffee hour encapsulates this openness and curiosity. It happens every day. People come together. Big ideas are formed. That openness is emblematic of what the lab was, which is the faculty are not competing against each other. There's truly a terrific camaraderie that I hope prevails. But at least at that time, you got the feeling like this had been around for a while.
Clarence Allen was in his 70s or 80s—well, I don't know. He passed away and, turns out, donates his fortune to Caltech and Reed College and Seismological Society and others. People were really passionate, and you got that feeling. That drives me into academia, I think, seeing that. Looking at all the PhD students in my year, the five students, I think they're all in academia. That starts with that big picture. In terms of skill set, having been within a group, we had our own group, and that was a really great example for me to see how science could function beyond. It was this group with a few key people. Then in terms of skills, I didn't pick up how to become an observational seismologist. I didn't. I could have. [laugh] There were people all around me doing that, and the opportunity was there. But, really, I was exposed to those discussions when they happened. But those were things that, as I left Caltech with a fairly narrow scope of expertise, maybe enough to get a job, but not enough to form a career. That was cool too though because I had this field exploration observational seismology. It left me realizing this is what's going on at Caltech. You need some pieces of that to diversify and be able to have these conversations, be able to like train students effectively. If I had trained a student to be just what I had done, this would not be a good route.
ZIERLER: [laugh]
TAPE: It is not necessarily a route you want to reproduce.
ZIERLER: Carl, in the way that you had that wonderful historical appreciation for the Seismo Lab, going all the way back to its roots, at this point, circa 2022, is there enough historical remove for you were you can extend that level of appreciation to when you were at the Seismo Lab?
TAPE: Yeah, I've thought a little bit about that with the 100th anniversary of the Seismo Lab, and trying to see how do you partition? It's easy to partition, like: this was Hiroo Kanamori. This was Charles Richter, Beno Gutenberg, Benioff. But then, as it gets more recent, there have become many more seismologists. The field has changed in just the sheer number. But when I look back, for me, again, I'm biased by that era, but the field of computational seismology, Jeroen Tromp and Dimitri Komatitsch played a major role in that part of seismology. To connect these things, if you look, nuclear testing stopped in the '90s. At that moment, everything pivoted to high-performance computing. When the National Labs realized we can't actually detonate bombs, we have to do everything we can on computers, why do you think we have the largest computers in the world at the National Labs? It's not to do seismology. But while that changed, here we are from the early '90s, fast-forward a few years [to 1998], and this cluster computing, high-performance computing came into seismology. That era is not a coincidence. Again, it's not disconnected from the nuclear era. I see computational seismology as having a major origin with that, Jeroen Tromp's era at Caltech, and stepping into this application toward real seismic imaging; not just toward a computer problem but taking that, linking it with these spectacular data sets, and using those to say something about the Earth. That is a major step between the computational side and, well, seismic imaging—a big step in seismic imaging. That's totally my bias. If I look back, each of those people—Kanamori, Don Anderson—they had some moment in their career that was probably equally transformative. But I think that the one I stepped into, one of those was certainly Jeroen Tromp at that point in his career, and it was great to be part of that.
ZIERLER: Finally, Carl, looking to the future, in the way that you can chart what your research agenda is going to be based on what you're doing now, beyond that, what are some of the frontiers in the field that you haven't touched on yet or only tangentially that 5, 10, 15, 20 years into the future, that's where you want to see your focused efforts going into?
TAPE: Thinking to the actual topic on frontiers in seismology two weeks ago in Seattle, one was distributed acoustic sensing, and how it intersects with so many fields outside of seismology. I was convinced that this will be interesting to see seismology helping in ways, monitoring the ocean temperature at 0.1–degree-level changes—that was a big paper out of Zhongwen Zhan's group. Alice Gabriel's talk there was all right up my wheelhouse on high-performance computing with sort of joint tsunami models, frictional contacts. You can take the seismic imaging, but then when you look at the earthquake rupture, that is a frontier and will remain a frontier. How exactly do big earthquakes evolve on this frictional contact? Not just when do they happen but, like, how do they happen? That is going to be a frontier for decades. There was planetary seismology, whether it's Venus or Mars, but it's just so expensive and so limited data that I'm not personally gravitating that way—even though Caltech's Division of Geological and Planetary Science, it's sitting right there with Seismo Lab. But Seismo Lab occupied a nebulous connection within the division. It was its own thing before it became subsumed. Looking forward, the work I have, I'm ambitious but I don't have something that I'm willing to step without caution—I remember a commencement at Caltech, it may have been Jared Diamond. But I remember one of the pieces of advice was like, "People," he said, "look, you guys are all pretty high achieving. You're ambitious, and you're drawn to pursue problems that you're challenged by, and you can't necessarily solve." He's like, "Just keep in mind that you also should be aware of where your skill sets are." It's almost like saying it's separated. You pursue a problem where you may not have the skill set. For me, that was really interesting advice because saying, "Where is your skills?"—it could be managerial, it could be looking at waveforms—but, to get the broader impact, you need to be aware of those skills. Sure, when you step into a challenge, it can't be devoid from those skills. When I look at this question, I feel a little bit of that, saying, "Where's the challenge and opportunity? But am I the right person, and how would I contribute to that?" Because maybe my role is going to be to try to make this tool needed for that large-scale next frontier. I'm going to make it easy for people to get trained in that realm, so when we get funded for a cyber-infrastructure project, that's playing a different role. But we got to find where that role is. It may not be the lead author on the science paper but it's somewhere in the picture.
ZIERLER: Well, Carl, on that note, this has been a terrifically fun and wide-ranging conversation. I'm so glad we were able to do this. Thank you so much.
[END]
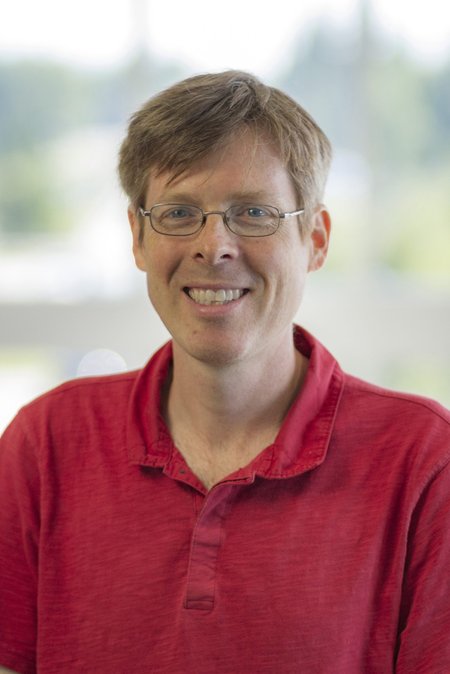
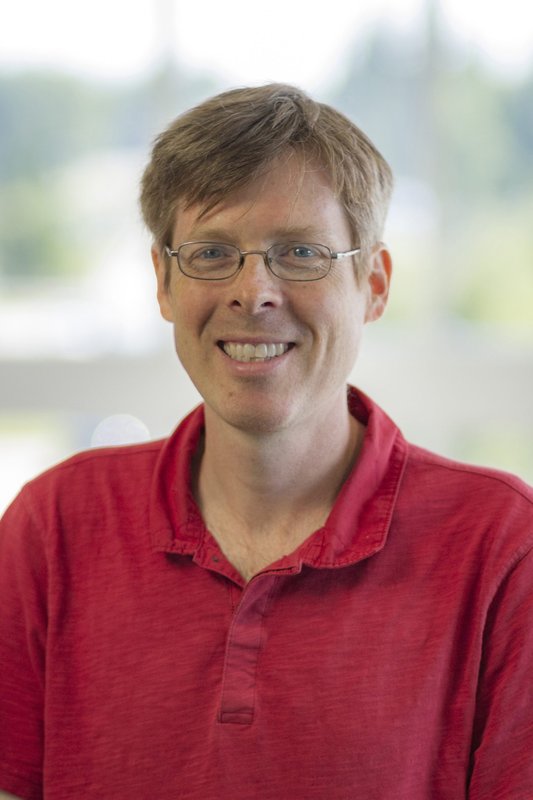