August 10, 12, 16, 18, 23, and 27, 2021
Dave Stevenson was present at the maturation of planetary science as a distinct field. In a career spanning five decades, Stevenson has distinguished himself as a world-class researcher on planetary magnetism and internal structure, and he has innovated the use of fundamental physics theories to enhance our knowledge of the solar system.
Born and educated in New Zealand, Stevenson arrived at Cornell to pursue a degree in physics, before his interests in cosmochemistry pulled him into the burgeoning field of planetary science. After an initial faculty appointment at UCLA, Caltech recruited him to join the faculty in 1980. Stevenson served as division chair of the Division of Geological and Planetary Sciences from 1989–1994, and he was named the Marvin L. Goldberger Professor in 2011.
Stevenson is a member of the Royal Society and the National Academy of Sciences, and his many awards include the Harold C. Urey Prize given by the American Astronomical Society. In retirement, Stevenson continues to work on improving our understanding of Jupiter, and he is pleased to see convergence in planetary science as an interdisciplinary studies field involving the expertise of astronomers and geophysicists.
Interview Transcript
DAVID ZIERLER: This is David Zierler, Director of the Caltech Heritage Project. It is August 10th, 2021. I'm so happy to be here with Professor David John Stevenson. Dave, it's good to see you. Thank you so much for joining me today.
DAVID JOHN STEVENSON: Thank you for doing this.
ZIERLER: Dave, to start, would you please tell me your title and institutional affiliation?
STEVENSON: I am the Marvin Goldberger Professor of Planetary Science, Emeritus. And I'm at Caltech, which is the name everybody uses for the California Institute of Technology.
ZIERLER: Dave, could you tell me the significance of being named in honor of Marvin Goldberger? What's the broader story there?
STEVENSON: To be honest, I don't know. It is maybe a little bit more prestigious than some of the chairs. Caltech has gone in the direction of fundraising that is tied to making full professors into chaired positions. That's now very common. At one time, it was much less common. It was certainly less common 25 or 30 years ago. Goldberger was, of course, a president of Caltech. I knew him. I even talked to him soon before he died. I talked to him as late as ten, 15 years ago. And of course, I knew him at Caltech. He was a physicist. The position was made in my honor in place of a previous chair, which was the George van Osdol Chair. But I don't know how it got funded. To be honest, I don't know much more than what I've said.
ZIERLER: Dave, what year did you go Emeritus?
STEVENSON: I became Emeritus just in the last month, so that means the middle of 2021.
ZIERLER: Oh, so that's hot off the press!
STEVENSON: Indeed. My view about going Emeritus is not universally shared by my colleagues. I believe that it is important for faculty irrespective of their scientific excellence to make way for young people, to make it possible for the Institute to hire young people. And one way to help that is to stop getting a salary, which is the most obvious consequence of going Emeritus. But in fact, it has very little consequence for day-to-day activity. The day after I became Emeritus, I was doing exactly the same stuff as the day before. I still work with students. I still work with postdocs. I expect to keep teaching. I am doing my scientific research. I am less involved in committees, which is great. I'm not a big fan of committees. But in fact, it doesn't make a lot of difference. I know there are some people who think that somehow going Emeritus is tied to retirement in the sense of going fishing [laughs] or whatever it is, or playing golf, or whatever it is that people imagine their retirement to be. I don't think of it that way. I'm just going to keep going.
ZIERLER: The indication there, of course, is that you love doing the science, and there's no reason to stop.
STEVENSON: Indeed. I feel myself very fortunate for being in a position where I do what I love. Actually, that's true of most people who are successful in science. Although it's also true that I'm a little bit unusual, because I am so focused on the science. There isn't a great deal more to my life. I mean, of course there are other things I like to do. I like to read books. I like to travel. I like to go to shows on Broadway or West End or whatever. But the truth of the matter is that science very much defines my life, very much defines what I do day by day.
ZIERLER: Dave, to the extent that going Emeritus expands your bandwidth with less administrative work, less committee work, what are the things you're excited about pursuing now that you have that leeway to follow your interest in a pure sense?
STEVENSON: I think that it gives me somewhat more opportunity to pursue things that are, shall we say, a little bit speculative in science. I have some ideas like that. They may not work out. I have more flexibility. But the truth of the matter is, I always had that flexibility. There is a textbook that I have written which is online which is incomplete. Maybe I will get around to completing that. I keep getting bugged by Cambridge University Press, year after year — "When are you going to finish this textbook?" There is no good textbook in the area of science that I represent. Maybe I will do that. But truth of the matter is, it's not going to make a huge difference.
Juno and Jupiter
ZIERLER: Just for a snapshot in time—I'm looking at the equations on the blackboard behind you—what are you working on right now? What's important to you at this very moment?
STEVENSON: At this very moment, a lot of my time is Juno, is related to Juno. Juno is a spacecraft which is in orbit around Jupiter, and I am lead of a scientific group that meets from time to time, these days virtually, and we try to figure out what's going on inside Jupiter. This is a mission that, like many NASA missions, lasts over decades. It takes time to write a proposal, to get a successful proposal, to get the money, to get the spacecraft built, to think about the science, to justify the mission. A major justification for this mission, not the only one, was to figure out what the inside of Jupiter is like, which is very closely related to what I did in my PhD thesis work, so it's coming back to my original expertise. I've done lots of other things, but it comes back to my original expertise. And it has been a fascinating experience, because it has alerted me to things that we did not understand well. And doing a mission forces you to challenge the assumptions that you made long ago, and that other people made. And that is what has happened. The mission has been successful. The mission is ongoing.
Success, by the way, I think, has to do with surprises. You go somewhere that you've not been before or do something you haven't done before, and you measure something, and it's different from what you expected, and that's a surprise. And that's great! That's how you learn. That's what science is. Science is not confirmation. Science is challenge, finding out things that you did not expect, trying to figure out how it works. And that's certainly what has happened.
The Juno mission has been extended. We went through that exercise just one year ago. We wrote an extended mission proposal that was accepted by NASA. That's not so unusual if the mission is successful. But it means more money. It means that we will keep doing science until 2024, probably beyond. The spacecraft will actually visit some of the Galilean moons. That will be interesting. It will also learn more about Jupiter. So I can see that kind of science continuing through several years more.
In addition to the things that I just daydream about, it's also true that I have become interested in exoplanets, planets around other stars. As often happens in science, I get frustrated when I look at what other people are doing. I'm talking here about theory, about models. The data are wonderful, and it's an explosion of new information and it's spectacular. And it has changed the nature of the science I do. No question that that's a great thing. I feel fortunate to live in a time when all this is happening, when we are learning about our own planets as well as planets around other stars. But I get drawn into the subject through realizing that people don't really understand what's going on around other planets. I have a student who's going to defend his thesis in a month, PhD thesis, and he's working on exactly that subject right now. I gave a seminar virtually in China last month about exoplanets. There will be some of that kind of work, as well as serendipitous things that have to do with planets in our own solar system. And the Earth. I'm interested in the Earth. I'm interested in what the inside of the Earth is. And there are still unanswered questions for that.
ZIERLER: Dave, there's a certain poetry in the sense that your current interests in Jupiter bring you all the way back to your doctoral thesis work. And so that begs the question, what is it about Jupiter that's so intriguing for you? Is it a specific curiosity unique to that planet? Or are there things about Jupiter that can be extrapolated to other planets in our solar system and even beyond?
STEVENSON: I think that Jupiter is of importance, if only because it is the most massive planet in our solar system. It's much more massive than all the other planets combined. It probably formed first. We don't know that for sure. But there's a lot of evidence about what took place in the early solar system, looking at meteorites, looking at the architecture of the planets and so forth, that is consistent with the idea that Jupiter formed first. So you do need to understand Jupiter to understand the solar system of which we are part.
When I give public talks about this, I make the point that Jupiter affects us. Jupiter affected how much water came to the Earth. So you can argue for the importance of Jupiter just from a parochial point of view. It's also true, however, that as often happens in science, I came to work on that subject by chance. And to be sure, I was aware that Jupiter was interesting already, when I was in New Zealand and before I came to the United States. But it did so happen that when I teamed up with the scientist I admired most at Cornell, the institution I went to, to get my doctorate, and that's Ed Salpeter, he just happened [laughs] to be interested in Jupiter. Ed Salpeter did all sorts of stuff, but it's serendipity that I would be there when he decided to be interested in Jupiter. And then he moved on to other things.
I think this often happens in science, by the way—that people end up doing something somewhat by chance. I think if you have the right attitude and instincts, you will succeed anyway. Maybe I would have ended up thinking about Jupiter if I would have gone somewhere else, but the circumstances, the chance, the serendipity, seems also to matter.
ZIERLER: Dave, you mentioned the recent proliferation in models and theories of relevance in planetary science. That therefore prompts a broader question, which is, in planetary science, what has been the interplay between theory and observation? And I ask that in a historical sense, for you to go all the way back to your thesis research and think about the interplay then, over the course of your career, and to where things are now. In what ways have theorists been leading observation, and in what ways have the observers been leading the theorists?
STEVENSON: Well, if you go back early enough, a lot of things were theoretical, because [laughs] there were no data. You might say it's a little bit more open. When I finished my PhD at Cornell, I happened to go to a conference on the origin of the solar system, which was in Newcastle upon Tyne in 1976. And it's fascinating to reflect on what that conference was like. It was very stimulating, but it was mostly theory. [laughs] People were saying things that might have been true, but they were based on ideas that came out of people's heads. They did not come from data. There was no data!
So planetary science has evolved along two tracks, initially. One was theoretical. The recognition that things that we understood in the laboratory on Earth or by looking through a telescope might be applicable to understanding how planets form or how they evolve. One of the key people on that was Harold Urey, who was a physicist, chemist, got a Nobel Prize for finding deuterium. He wrote a famous book in the 1950s about the nature of planets. And so that was more theoretical, even though it had a link to observational ideas in the lab. To be sure, there were experimentalists, but they were looking through telescopes. Kuiper was the famous example of that. So you had the theorist, lab person, Harold Urey, and you had the observer, the telescope, Gerard Kuiper.
But then along came something profoundly important, and that was the Space Race, and the Cold War. Because let's face it; the reason why the United States, the reason why the Soviet Union, put so much money into sending rockets into space was because of the Cold War, because of this perception, probably incorrect, of being fearful of the other country. And that led of course to the Apollo mission, but it also led to unmanned exploration, which was a small part of the total cost, but came out of the same environment. And so that revolutionized planetary science. Planetary science became something that was tied to missions.
In my own trajectory, the missions were less important, although I became a parasite. I became a parasite because I chose not to get involved in missions early on, and to benefit from the results. That's the sense in which it was behaving like a parasite. More recently, I've discovered the joy of getting involved in a mission, although it is extremely time-consuming. I would say now, looking at the field, that the missions are key, that it really is data driving theory. These are complicated situations, so pure theory is less useful. In some areas of physics, which I know a bit about, like string theory, you could imagine people doing things without worrying about observation. Let's face it; there's no connection between string theory and anything you can measure. It's an astonishing thing! But in planetary science, you're dealing with complicated things. It's a little bit more like biology, much less like basic physics. Geology is the same. You rely very heavily on observation. So I would say observation drives the field.
Theory is very important. Fewer people do it. You don't need a lot of people doing it. There are a lot of people who run models. Models are not quite the same thing, but of course rest on a framework of theory. The basic point is that the observations are key, and therefore the missions are key. Yes, you can look through a telescope, but we've learned that it helps to go there. It helps to go to Jupiter. There are all sorts of things you learn about an object by actually going there. I don't mean in person; I mean you send a spacecraft that's got instruments, measures things. That's what Juno does with Jupiter. That's what Cassini did at Saturn. That's what InSight is doing at Mars, and so on. So those things were essential.
ZIERLER: Dave, a question geared to the broader public who's interested in planetary science—what sticks out in your mind today regarding what is really known in planetary science, what is settled in the field, and on the other side, what are some of the big mysteries, things that people might be surprised science really does not quite understand, even today?
STEVENSON: I think that we've come to appreciate that planets are individuals. This is actually important. It's a different philosophy, a different viewpoint than what you see in, for example, astronomy, which is closer to physics. In astronomy, and in many areas of physics, you generalize. You look at a class of objects and make general statements about their class. The famous example in astronomy is the Hertzsprung–Russell diagram, the HR diagram, which is a very simple diagram that can be used to describe the evolution of stars. It's an astonishing thing. It's very successful. And it works. Why does it work? It works because stars are actually simple. Planets are complicated. Why are they complicated? Because they're made of materials that are not ideal. Stars, for the most part, are made of ideal materials. Even when you go to things like white dwarfs or neutron stars, they're actually close to ideal, ideal in a different sense—Fermi gas. Planets are messy. They're made of materials that are complicated. Composition is complicated. The way their composition was derived through the processes of formation is complicated. And so in that sense it's more like geology or like biology, the study of individual objects.
As to generalities, I would say that what we've learned is that in seeking to understand our own solar system, we have come to realize that other planetary systems don't do the same thing. And that's fine. [laughs] That guarantees a job. But more than that, it guarantees that there are things we don't understand. And so I can see in the future a growth in developing ideas that still have some generality. There are some general ideas, to be sure, in how planets form. But the planetary systems show diversity. The individual planets show diversity. And if there is an underlying generality, we're not there yet.
By the way, I would say the same thing about biology. I once heard a famous biologist say that biology is the science of exceptions, and it is sort of true. The biggest questions in science, I think, are actually in biology. I'm well aware that physicists have the conceit of the theory of everything. I understand what they mean by that. And that's important. Dark energy is important. Dark matter is important. But understanding the origin of life is as important. And that's not a purely biological question. The consequences of biological evolution are not that well understood. And I see a lot of things in planetary sciences having commonality with biology.
ZIERLER: I wonder, from a nomenclature perspective, if you can explain where planetary science butts up against astronomy and astrophysics, and where you see it really as its own discrete field?
STEVENSON: Astronomy is heavily committed to instruments, and to ways of measuring. And there is no doubt that astronomy has been hugely important for understanding planets around other stars, and for understanding the formation conditions. It's happening right now. For example, ALMA, A-L-M-A, which is an array of telescopes, in the dry desert of Chile, that rightly belongs in the field of astronomy. But it is the instrument that is being used to characterize the existence of disks around stars, from which we presume planets form. And now most recently, just last week, the suggestion of finding a disk around a planet, within which perhaps a moon could form. So astronomy is actually playing a leading role in the data, in the instrumentation, in the characterization, in what you might call butterfly collecting. Which sounds derisory, but it's not really intended to be that way.
I think that in the future, we will see that planetary science comes more into its own right, through the expertise that we have in understanding individual objects. It's already beginning to happen. We're already looking at atmospheres around exoplanets, trying to characterize their composition. Here at Caltech, we have strength in both. I have colleagues in the same building who do exoplanets. They don't worry about our own solar system. And they do it in a somewhat planetary way. And across the road—that's one of the nice things about Caltech; it's small—across the road, there's an Institute, a building called Cahill, which houses people who do exoplanets from an astronomical point of view, and tend to be more focused on instrumentation. On imaging planets, we have some of the best expertise in the world in that area. So there's a place for both.
ZIERLER: To stay on the nomenclature question in an area where I assume there's much more overlap, and that would be geophysics, where do you see the overlap with geophysics and planetary science?
STEVENSON: I do some geophysics, and I have worked with geophysics students. I have a student right now who came to Caltech to do seismology and geophysics and switched to planetary. People in geophysics get seduced, to some extent, by thinking about planetary science. It's understandable. I mean, if you get tired [laughs] working on the Earth, it makes sense to think about Mars or Venus. The same is true of geology, by the way, and chemistry. We have people who dabble—more than dabble—in planetary science who would call themselves geochemists or geologists, as well as the geophysicists. The overlap is there. The expertise is there. The techniques are there. If you want to understand the inside of Mars or the inside of Venus, it's very natural to use the skill that you have already developed in what is frankly in many ways a more mature field.
I have in the past made myself a little unpopular here at Caltech by suggesting that some aspects of seismology are mature. It may not be the most interesting field to be in. I've actually changed my mind on that. I can see that there are many exciting things, still, in the future, in that field. But, there's no doubt that the highly specific expertise that you develop in understanding seismic signals for Earth might help you understand Venus or might help you understand Mars. Understanding normal modes of the Earth might help you to understand the same thing in giant planets. By the way, that field is part of the future of planetary science. I believe that. I have students working on that. I think seismology is part of the future of planetary science. It has not been important in the past because it's too hard to do. It's very hard to do seismology in other planets. It's a hell of a lot easier to just send a spacecraft out there, take some pretty pictures, measure the infrared spectrum. You can even do that from the Earth, sometimes. Measure the magnetic field. [laughs] It's a lot more difficult to land or to measure normal modes or whatever it is you want to do, to do seismology.
ZIERLER: Dave, is computer simulation an important part of your work, and has that become increasingly so, as computational power has advanced over the decades?
STEVENSON: Simulation is important. I think you should always have ground truth with experiments. It's true that computational ability has enabled the calculation of material properties with greater accuracy, greater confidence. I actually know something about this field. I used to work in applications of quantum mechanics to understand materials at high pressure. I used density functional theory, which is sort of state of the art in some respects, as long ago as the 1970s, early 1970s. I think that yes, it has a role to play, but you still need the experiments. I would hate to see us go in the direction where we become so sure of ourselves in the ability to compute using quantum mechanics that we set aside the difficult experimental work.
And it is difficult at high pressure. Even something like hydrogen, which you might think is so simple—it's the most common baryonic material in the universe—we [laughs] still don't understand how hydrogen behaves. And I know people who do this by simulation, quantum Monte Carlo or whatever. But experiments are still vitally important. Yes, simulation is important, but experiments are important as well. And of course, planetary science is driven by observing what actually happens.
ZIERLER: Dave, of course you operate in a basic science environment in planetary science, just understanding how planets evolve and their internal structure. But I wonder if you've ever reflected on how some of your research may contribute to applications—in materials, in instrumentation, in things like that.
STEVENSON: I have to admit that I don't spend a lot of time being concerned about that. I do believe that what I enjoy doing is more akin to what happens when somebody writes a work of literature or makes a painting or a sculpture, or just understanding better the universe of which we are part, which is sort of a very basic ivory tower scientist point of view. Maybe [laughs] there is a practical consequence; I seriously doubt it. I know people who think that you can go and mine asteroids. I've talked to people who think that we can get helium-3 on the moon. I'm a skeptic. But for me personally, it's actually not important. I feel fortunate to be doing something that is just basic knowledge.
I do, by the way, having worked on hydrogen a lot, I have to confess also that someone who I worked with back when I was a student, Neil Ashcroft, he got money from NASA, because of the idea that hydrogen in its metallic form might be a fuel, a rocket fuel! [laughs] And going into space is good. I hope Elon Musk succeeds. I hope SpaceX succeeds. So sure, I follow that stuff. But I don't think I'm contributing, and I don't feel the need to.
ZIERLER: What about even with the internal structure of the Earth and what we might learn there?
STEVENSON: I don't see that as being of much practical interest. I suppose if you go far enough in the direction of thinking about things that are closer to everyday life, like why do earthquakes happen, can we ever predict earthquakes, why does the climate change—I mean, I take interest in those things. These are not what I work on. But my interests are sufficiently broad that I suppose there's a possibility that I could come up with something that's relevant to understanding those subjects which would influence society. Certainly, I have met faculty, colleagues, students especially, who care about the future of the Earth. And that's fine. And of course, I've met people who want to colonize Mars, which I think is silly. But again, I don't actually spend a lot of time worrying about whether anything I do is of practical use.
ZIERLER: Well, I can't help but ask now, why is the colonization of Mars a silly proposition to you?
STEVENSON: To me, it's silly because the impetus would seem to be in part the notion that it's readily achieved, and in fact it's not. It's extremely difficult. And I don't think the people who imagine doing this realize how extremely difficult it is. If you wanted to preserve life on Earth, then short of something truly catastrophic, which is very infrequent, the most sensible thing to do is to dig a hole in the ground. It's unromantic, but you can survive all sorts of horrible things just by going underground. I'm not advocating that; I'm just using that as a comparison. It is so much easier to create a living space underneath Earth's surface with the resources that you need and the energy that you need, than doing the same thing on the surface of Mars. It is a romantic notion. I'm not against romantic notions. I'm not against people going to Mars. I think colonization is a different concept. It also suggests a connection to colonization on Earth, which is enormously easier. I mean, I had ancestors who helped colonize New Zealand. They came from Scotland. That's easy! Even though they spent four months on a sailing ship! It's easy! Going to Mars is incredibly difficult. It's possible. It's technically possible. There are problems, but it's probably possible. It's also possible to build a Starbucks at the summit of Mount Everest. Would you do it?
ZIERLER: [laughs]
STEVENSON: I'm sorry, it just seems silly to me.
ZIERLER: By referencing the ease of digging a hole in the ground, it sounds like one of the things you assume motivates discussions about colonization of Mars are apocalyptic scenarios, that we need a plan B, an escape hatch if you will. Is that part of it?
STEVENSON: I do get that sense, that some people are thinking, "Well, we have to do it, because of something terrible that will happen on Earth." It's also true, of course, that there are people who have this—I call it romantic—notion that it's man's destiny to go into space or something. It's difficult for me to articulate that view, because it doesn't fit with my understanding of the nature of the universe of which we are part. It's actually very, very difficult to travel in space. It's extraordinarily difficult to go from star to star. I suspect there are people who don't understand the difference between a technological challenge and the practicality of doing something. It's more than just a technological challenge. It's actually somewhat impractical. That doesn't mean you can't do it. It just means it's immensely difficult. Of course, things may change in 100 years. There were people standing around in 1900 who said that physics was finished. [laughs] And the world has changed enormously. A hundred years—I mean, I'm talking about the next decade or so. People talk about doing something on Mars that soon. That's the part that troubles me. There are all sorts of things we can do on Earth that are easier.
ZIERLER: Dave, last question to round out our current talk, and that is one that we're all dealing with. How has your research and even your world view changed as a result of the pandemic and the mandates of social isolation and remote work?
STEVENSON: For me, the pandemic has been a fairly major effect on traveling. To be sure, teaching has changed. I've taught a couple of classes remotely using Zoom. Actually, I meet with a class every week, in person, here on campus, freshman. Previously that was done remotely. But travel is the biggest effect. In 2019, I traveled more than 100,000 miles. I went to China twice. I went to Europe several times. I went to Beirut in Lebanon. I went to Jerusalem. I went all over the place. And in 2020, nothing. [laughs] Well, actually, that's not quite true; I traveled in February of 2020 [laughs], to France. Switzerland. But so travel has been a big consequence.
Interaction with faculty has sort of continued through seminars online and so forth. But casual conversation of the kind that should happen and at Caltech usually does happen has diminished. I participate in lunchtime meetings occasionally on Zoom, and we talk about anything, including the virus [laughs], with people like David Baltimore. That part hasn't changed a lot. But travel has changed dramatically. I think travel is important. But I guess the bottom line is, I have come to appreciate, like many people, that personal interaction actually matters. Now maybe that will change. Maybe this kind of interaction which we're doing by Zoom will actually improve. People are trying to do that. But I haven't seen it yet. Interaction virtually, for example by Zoom or WebEx, is actually not as good as just sitting in a room, seeing the body language of people, being able to talk casually, being able to tell by eyesight whether they're eager to say something, or they're going asleep [laughs], or they're looking at their iPhones or whatever. So, yes, things have changed.
ZIERLER: There's a lack of spontaneity, which of course can be so important to the science.
STEVENSON: Yes, yes.
New Zealand Origins
ZIERLER: Dave, let's take it all the way back to the beginning. Let's start in New Zealand, first with your parents. Tell me about them.
STEVENSON: I was born in Wellington, New Zealand, which is the capital city. My father was a carpenter. He worked for the New Zealand railways at a time when a lot of things were made of wood. Actually, he did cabinetry, and he built things. He built an additional bedroom on our house. He helped build a couple of classrooms at the local school that I went to. New Zealand has a do-it-yourself culture so people tend to do things themselves if they can. I helped him put a new roof on the house. He left school at the age of 12 in the Depression in the 1930s in New Zealand, but he was an inquisitive person, and he took me to the local library, and so I read books and so forth.
And on that side of the family, my great grandfather with the name Stevenson came to New Zealand as a child from Scotland in a sailing ship. Background as tailors. The New Zealand I grew up in was an egalitarian society—nobody poor, nobody rich, an open society. My mother was a housekeeper much of the time. I grew up in a society where that was normal. Women did not work for the most part. They worked a little bit, and get a bit of extra money. She did seamstress work part of the time. But it was a 1950s culture in which family was centrally important. But it was a pleasant environment. New Zealand is a nice place to be a kid.
ZIERLER: How many generations back on your mom's side does the family go in New Zealand?
STEVENSON: I don't know for sure. I have less information on that. I think it is English and maybe Scottish. My mother's first name was actually Welsh, but I remember overhearing a conversation once, when somebody asked her about that, and she said that as far as she knew, there was no Welsh background. But my grandparents on that side of the family were profoundly deaf. My mother grew up in a family where neither parent could hear anything. It's an amazing thing, actually. My mother thought it was perfectly normal. My grandmother, who we lived with—we lived in the house of those parents—I never had close contact with that grandmother because I could not talk to her. And it's amazing on reflection that everything like that seemed so normal, but in fact communication was done by lip reading. They did not know sign language.
ZIERLER: From the stories you heard from your parents, in what ways were their lives affected by World War II?
STEVENSON: They were not that affected by World War II. My father was in a line of work that required people to continue doing it even though there were many people, including many of my relatives, who served in the Armed Forces. New Zealand of course did have people in the Army, and in the Navy, who served—I had an uncle who served in the Pacific. I had cousins and so forth who served in the Mediterranean theatre. They told me stories about when they fought in Italy, and at Cassino, and they had been to Trieste and places like that, at the end of the Second World War. Certainly, when my parents got married, there was no notion of a white wedding. They got married along with other people. Everything was simplified, if you will. But New Zealand was a little bit immune from many of the things that happened in the Second World War.
The First World War was a huge effect. A very large number of people of the appropriate age were killed in the First World War. A brother of one of my grandmothers was killed at Gallipoli. A brother of my father's father was killed in 1917 in the trenches in Flanders in Belgium. So the First World War had a big effect, and you can see it still in New Zealand, with monuments at the center of towns and so forth. The Second World War, less so. Of course in the Second World War, Americans came to New Zealand. It was a place for R&R. So people knew about Americans. They had met Americans during the Second World War.
ZIERLER: Dave, tell me about your neighborhood in Wellington. Was it more urban? Was it more suburban? What was it like where you grew up?
STEVENSON: In the 1950s, the area I grew up in, which was Miramar, was viewed as an outer suburb of Wellington. You could get to it by tram, by streetcar in the language that's most commonly used in the United States. But it was sort of like a village. It was not rural; it was urban. But it was somewhat open. It's true that the flat land was covered in houses, and there were shops. You only had to go a block to go to the dairy or to the green grocer or whatever. But it was relaxed and somewhat open, not like being in the center of a city.
ZIERLER: Growing up, did you have interaction or general awareness of the indigenous peoples of New Zealand?
STEVENSON: I had very little interaction with the Maori who came to New Zealand about 800 years ago. Most of the Maori were actually in rural areas at that time, or in the northern part of New Zealand. Which, to some extent, is still true. When I go back there now, I see a more diverse mix of people. I think it's a good thing. New Zealand has changed. The population has doubled since I was a kid. There are a lot more Polynesian people.
I need to explain that in New Zealand, there were the original Polynesian people, which we called the Maori, who came primarily 800 years ago and developed quite a sophisticated society, although they had no written language. But then much more recently in the last 50 years especially, there have been people coming from the Pacific Islands to the north of New Zealand, partly because those places are overpopulated, and they had free entry to New Zealand in many cases, although they are now independent countries. I'm talking about Tokelau. I'm talking about Samoa. I'm talking about Tonga. Places like that. These are Polynesian people, and they have come to New Zealand, and have families in New Zealand and so forth. So there are more Polynesian people than there used to be. There's a lot of intermarriage.
The people are highly regarded. When I grew up, as a kid, there was never the sense that the Maori were somehow in any way inferior. In fact, almost the opposite. We learned a little bit about Maori culture at school, in elementary school. Not a lot, to be sure. But there was this sense that these were dignified and highly valued people, maybe a little bit more happy go lucky than the Pakeha, which is the word we use for the white people, but never a sense of inferiority. I've come to recognize that in a less obvious way, they might have been less well treated. They certainly had land taken away from them in the 1800s. And that has been fixed to some extent. New Zealand interestingly has had some reparation. There was something called the Treaty of Waitangi, which was signed in 1840, between the Maori chiefs and basically Queen Victoria. [laughs] That's how New Zealand became English. Could have become French. And that supposedly recognized the rights of the Maori to the land. But of course, the Maori, as is often the case with indigenous people, had no sense of personal property. And so they gave up their land rather easily, and sometimes were forced to give up land, which is bad, of course, in subsequent decades. But now some of that land has been given back. And so you will find parts of New Zealand where it has been recognized that the land now belongs to a local Maori tribe. It's not that obvious, but if you poke around, you can see that that has happened. There are even some groups that are actually quite wealthy, because they have land obtained that way, and people who got money. I think New Zealand has actually done fairly well on that score.
The other thing that has happened in New Zealand is that Maori is now an official language, so if you go to any website for, say, the university where I got an undergraduate degree, you will see the name of the institution listed in Maori, in the Polynesian language, which of course is phoneticized using English characters—they never had written language—as well as the English language. So in some sense, Maori has become an official language, although in practice, it's not used a lot except to name things.
ZIERLER: Dave, were there other immigrant communities in Wellington when you were growing up? People from Africa, Asia, South Asia? Were there Jews around your neighborhood when you were growing up?
STEVENSON: New Zealand, when I was a kid, was much more homogeneous than it is now. It was much more, shall I say, English. My best friend when I was in elementary school was Dutch. He came to New Zealand knowing no English. His family came. And so there was a Dutch community. There were people from other European countries who came, especially the Netherlands, but also people from places like Hungary and so forth. But they were a small fraction. It wasn't actually like Australia. Australia was different. Australia had a much bigger influx of people from the Mediterranean countries, especially from Greece and from Italy. They had a large number of Italian immigrants and Greek immigrants. Parts of the United States had that, too. New Zealand had it less so. We were more heavily Protestant, more heavily people who had ancestry in Great Britain.
That has changed over time. I think it's good. We did have a small number of people who came from China because of the gold rush, just as in the United States. They stayed in New Zealand. I knew Chinese people when I grew up, but they were not large in number. They actually ran the green grocer stores [laughs]. They were market gardeners, having shifted to that from being in mining. There's a bigger influx now of people from Asia.
New Zealand has a policy that is different from the United States in that there's a point system for immigration which tends to emphasize people with some kind of ability that you can measure, so doctors and so forth can get in from diverse backgrounds. United States of course has a policy of immigration that is complicated, probably should be changed, but has often emphasized family connection, irrespective of whether people have some kind of specific ability that can benefit the society financially. I don't want to pass judgment on the relative merits. They're just different. Australia had a White Australia policy. New Zealand, somewhat so, I have to say, preferring white people.
The Jewish population was always small. I knew one Jewish kid at high school. It was not common. There was probably some anti-Semitism, but there weren't very many people from that background at all. Probably less so, per capita, than Australia. The person I worked with at Cornell when I was a graduate student was Jewish, Ed Salpeter. He went to Sydney. His family escaped from Vienna after the Anschluss and went to Australia. There were things like that in New Zealand, but less so. It was very homogeneous. I don't think that's a good thing, but it worked out okay.
ZIERLER: A very abstract question, but I wonder if you've ever reflected on the possible connections in being from New Zealand and your interest in planetary science. In other words, the remoteness of New Zealand, the fact that it's an arduous journey to get there essentially from anywhere else—have you ever divined any connections between where you're from and what you study?
STEVENSON: I don't see a big connection of that kind. I should first say that I think people make too much of an issue of nationalism. I am uncomfortable with nationalism. I think it has been a problem in the modern world. I cringe when people talk about or think about American exceptionalism, for example. I think that being exceptional in some sense is an individual trait. It's not necessarily tied to where you grew up. Of course, it's an advantage to have come from New Zealand than to have been a child in Bangladesh or Nigeria or Syria. Of course. I'm not sure it would have made a huge difference if I had been a child in the United States.
That said, New Zealand is an open society. It's outward-looking. When you're isolated like that, and when you're small, you look to the rest of the world, even more so than other small countries. I know people in Denmark. I have a high regard of Denmark. I think Denmark does very well. I don't want to pick on Denmark; it's just an example of a small country. But they also tend actually—and I know this, having talked to students in Denmark—they would like to stay in Denmark. In New Zealand, at least the New Zealand I grew up in, if you were good enough, you wanted to leave. New Zealand has been very good in exporting outstanding scientists. Being in an isolated place can be a good thing in changing your world view towards looking outward rather than looking inward. In the United States, I see a lot of people who look inward. Sad to say, there are a lot of people in the United States who would have trouble if they were asked where a particular European country was, and given a map, they wouldn't know [laughs] where to find the country. They don't know much about the world. I know a lot more through having come up in a society where it's natural to recognize your smallness and see the rest of the world at large. So being isolated can help in that sense. Being isolated can also be a disadvantage.
When I was a student, it was unthinkable to go back to New Zealand. Nowadays, people can do it. Travel is a lot cheaper. But in the 1970s, it was unthinkable. It was just too expensive. If you went off to be a student in England or the United States, you just expected that this would be four years. The notion of going back for Christmas or something was unthinkable.
ZIERLER: [laughs] You mentioned that your father's educational opportunities were cut short because of the Great Depression. Is your sense that he had intellectual or technical abilities that had he had different opportunities or circumstances, he would have pursued higher education and a professional career?
STEVENSON: It's possible. I simply don't know. I am unsure about the genetic connections between the skills I have and those of my parents or grandparents. I know people make an issue of this. It's an interesting question. I think the way genetics work, you just naturally get large variations. I do think that some people benefit from growing up in an environment that is better off economically. They have more options available. I actually see this and worry about it, because I see it sometimes in Caltech undergraduates who come from Asian countries or elsewhere. I've come to realize that they are actually from families that are well off financially, and so I suspect that matters. And being well off financially might mean that the parents are professional. It's noticeable, by the way. I read lots of application folders of students who want to come to Caltech, and it includes information about what the parents do. And they're professionals! [laughs] So I wonder about the role of that. And I don't have a simple answer. And okay, part of it is genetic. But then I also see people from backgrounds that are not professional, and they do just fine. Fred Hoyle did just fine, growing up in the north of England in an environment that was not fostering that kind of intellectual expertise that he had. I'm wandering a bit from your question. It's possible. I simply don't know. By the way, my mother was good at algebra, and maybe that mattered, too. I don't know.
ZIERLER: Beyond the genetic speculation, what tangibly did you learn from your parents about science, or more broadly about how the world works? What sticks out in your memory?
STEVENSON: Certainly, my father was interested in astronomy, and that mattered. He took me and my older brother to a local observatory when I was about eight years old, and we saw the moon through the telescope. And that was exciting. Although the most exciting part was going in the cable car [laughs] up to the top of the hill. And perhaps even more significantly was Sputnik. That wasn't specifically my father, but he took an interest in it. He took us out to the back yard and we looked at Sputnik as it came overhead. You could see it, especially through binoculars.
And I got interested in what was going on with the dramatic developments in going into space. I wrote a letter to the local Soviet embassy, and they sent me publications about Yuri Gagarin and the Russian space program, which of course was ahead of the U.S. at that time, around 1960, 1961, 1962 even. So yeah, those things mattered. Going to the library mattered. Although when I went to the library with my father, I just sought out what I found interesting, and I found interesting stuff almost independent of his interests. That was important. Looking back, I think it mattered. Certainly access to books mattered. I realized later in life that that was actually not so common. Because I would be surprised—I would go to other people's places and look for books, and there were no books! [laughs] And that surprised me. I thought that was normal. I thought everybody read books. But actually, it turns out it's not true.
ZIERLER: Was your family more secular or religious, would you say?
STEVENSON: More secular. Nominally Presbyterian. The Protestant background is strong in New Zealand, less so now. I had the amusing experience that because my father was the scout master for the local Boy Scout tribe which was affiliated with the church, that we were visited by the elders, who pointed out that I had never been baptized. And so I had the embarrassing experience of going with my family—the only time we went to church, as a group—at the age of seven and sitting in the front row, and having holy water sprinkled on my head. [laughs] Which was very embarrassing. [laughs] And then I went to Sunday school and bible class and so on and so forth, and then decided that this was not of interest to me, when I was a teenager.
Interestingly, when I was in elementary school, there was a Roman Catholic school across the road—Marist Brothers—and we fought with those kids. It's funny in retrospect. It's silly. But there was this natural antagonism between Protestant and Catholic. It was silly and it didn't really mean much, and I think it's diminished, but that was noticeable. And as I mentioned before, there were no Jewish people, essentially. There were no Maori. There were a lot of different Christian organizations around. They still exist. But it was mainly secular.
ZIERLER: In your primary schooling, was it always science that was most interesting to you, or were your pursuits more broadly defined?
STEVENSON: I think that what I related to most was mathematics and science. Certainly mathematics. I do think that pure mathematics is truly wonderful and extraordinary and I still don't really understand the more esoteric aspects. It's different from science, and it's a wonderful thing in its own right, and it intrigued me early on. But I also got naturally interested in science, and that became more important with time. I do think that part of what happens when you learn something like physics is tied to experience and to the elapsing time. And you can pick up math more readily. It's better defined. I found physics difficult, to begin with, and then easier as time went on. Applied mathematics was always easier for me. I've taught applied mathematics. It's easier. Why is it easier? Because it's better defined. Physics, you have to decide what's important. That's not a mathematical procedure. That's more mysterious.
ZIERLER: Were there particular teachers in high school that were influential in your intellectual development?
STEVENSON: Certainly, there was one in particular, a mathematics teacher, Dennis McHalick, who I had on two or three occasions, two or three years. He was inspiring. And he did things that may sound mundane, but were actually inspirational. And good teachers still do this. And it's funny; when I look at it now, I think well, that doesn't actually mean very much. But it's still important. An example would be series, in which you write down three numbers. What's the next number in the series? I now know of course it can be anything! [laughs] But it's an interesting intellectual exercise, because it forced you to think about structure and about organization and what's simple. You get to appreciate simple notions, simple ideas that might define a sequence, and things like that. And also geometry. Geometry of course is a profoundly important subject. I think it is underappreciated these days because people are so focused on computation. But just the business of congruent triangles and how to make different structures on a two-dimensional plane and so forth; I mean, that's amazing stuff.
ZIERLER: In the New Zealand system, when it's time to think about university, is it fairly straightforward? In other words, you go to the local school because the schools in New Zealand are essentially the same? Or is there a hierarchy there, where the best students go to a particular school? And on top of that, when you were thinking about university, were you thinking beyond New Zealand? Was that even on your radar at all to leave New Zealand as an undergraduate?
STEVENSON: It was unthinkable to think beyond New Zealand as a beginning student, as an undergraduate. And if you wanted to do something like physics or chemistry or biology or mathematics or something in the humanities, you just went to the local school. That may have changed a little bit, but not a lot. I think it's still true to a large extent—you just go to the local school. Schools were rather similar. They still are. I think that this might actually be a bad thing. When I got an honorary degree at Victoria University 20 years ago, and I had to give a speech, I actually suggested—it's not a novel suggestion—that thought should be given to making one of the universities be the best place in physics, say. That hasn't caught on. [laughs] So, there are exceptions. Engineering, you go to a particular place. If you eventually want to be a doctor, you go to a particular place. New Zealand is a small place. But it's a very local way of thinking. It's different from the United States in that respect. But it's also kind of true of Australia. Maybe less true in England, because there is this aspiration to go to Cambridge or Oxford or something like that. But it's very localized. Going outside New Zealand, it wouldn't even occur to you. When you're a graduate student, after you've got a bachelor's degree, if you're good enough, you would never stay in New Zealand. New Zealand is very good at exporting people, some of whom go back [laughs]. But in the New Zealand I grew up in, you would as a matter of course go elsewhere. That was what you had to do.
In my case, the big decision and the one I did in an unusual way maybe, was where to go. I was unusual in choosing to go to the United States. In those days, if you were good in physics, in math, in something like that, then of course you went to Cambridge. Unthinkable [laughs] to go anywhere else! You'd go to Cambridge. I had lecturers who had gotten their degree at Cambridge. Maybe Oxford [laughs], but it was usually Cambridge. And so, you didn't actually encounter many people who had had experience in the United States. Nowadays it's much more common.
ZIERLER: Now, in the New Zealand system, when you enter undergraduate, is it like in England, where you commit to a field of study right away, or do you have a few years of general studies, and you can choose from there?
STEVENSON: You have to commit. There was no such thing as options. There was a slight flexibility, but if you had decided, as I did, that I was going to do physics, then it was almost completely defined. You had to do the first year of chemistry. You had to do the first year of pure math. You had to do physics, of course. That was basically it. And of course, it's a year-based system. It's not semesters or quarters. So when I say physics, that means a real year, which in our case was from March through November, that was physics, including lab. So you didn't separate classroom from lab. It was all tied in together. And then in the second year, you didn't have to do chemistry anymore, which was nice. [laughs] But you had to do applied math! So maybe by—I don't think I really had any choice. It was unthinkable, for example, to grab a class in geology. It would never have occurred to me to do that. But it wasn't an option. You just couldn't do that.
Of course, when I went to Cornell as a graduate student, part of the attraction was doing classes. If you went to a place like Cambridge, you did a degree. You might sit in on some classes, but classes at the graduate level were not regarded as an important part of education. By the way, that's still true. I still think the American system is better. Of course, the American system also has the need [laughs] that comes from the fact that students are less well prepared as undergraduates. That's actually true. You just get less math and physics as an undergraduate, typically, in the United States. Not at Caltech [laughs], but at Ohio State or something, or most places, you would get less math and physics than as an undergraduate in the English system or the New Zealand system. New Zealand was maybe a little bit more like Scotland. That's understandable. There's more of the Scottish influence, Protestant influence. I think it worked okay, although it's interesting that it meant that any interest I had in things like political science or philosophy or history, anything like that, I had to pick up later. So it has advantages and disadvantages.
ZIERLER: Entering university in the late 1960s, I'm curious if any of the political tumult that had engulfed campuses in the United States and Europe, if any of that had reached New Zealand, or if you were sort of generally aware of these political revolutions more broadly?
STEVENSON: I was aware of them. I was apolitical. Maybe should not have been, but that was the way I was. If you were attending a university in New Zealand at that time, like Victoria, you would have to be aware of the fact that people were protesting the Vietnam War. And that was happening around us. In New Zealand specifically, there was another protest, which eventually affected the entire world, somewhat, because it affected the 1980 Olympics. And that was the protest against Apartheid. Because New Zealand—we talked briefly about religion earlier—the true religion of New Zealand, of course, is rugby. [laughs] And New Zealand had a very good rugby team, the All Blacks, and they played against South Africa, which was also very good. And South Africa, of course, had Apartheid. So there was this concern—why are we playing against a country that is so disrespectful of the majority of its population? It's a very serious issue. That blew up more after I left, became a very big issue in the late 1970s, but it was already an issue in the 1960s. People were complaining—"Why are we playing against South Africa?"
ZIERLER: What were some of your favorite courses in physics, and how might that have influenced your later specialty in graduate school?
STEVENSON: I think I enjoyed all of physics. I think that I was more comfortable, shall we say, with those aspects of physics that overlapped with applied mathematics. That meant, for example, things like differential equations describing physical systems. The modes of a drum head, or things like that, which involve Bessel functions. Things like that to me were so natural. I can remember when I took a high-level applied math class at Victoria University as an honors student, the instructor handing back my attempt at the homework problems, commenting that I was the best student in the class. But I wasn't even signed up to the class. [laughs] So that's an example of what you might call an option. [laughs] I was taking it not for credit, because I couldn't take it for credit! But I just enjoyed that stuff. Quantum mechanics was a natural to me because most of quantum mechanics at the level I was doing it was differential equations. And I was good at that. It sort of was in parallel to that. But I have to say that physics really only grew on me when I became a graduate student, and that was at Cornell. The teaching was good, and it was people like David Mermin, Neil Ashcroft, Hans Bethe. Hans Bethe of course in quantum mechanics. David Mermin who would have been statistical physics, where he was brilliant. So yeah, it's a bit related to what I was good at, and a bit related to how good the teachers were.
ZIERLER: Now, was the curriculum in physics broad enough at Victoria that you had some even rudimentary exposure to things like geophysics or seismology or planetary science?
STEVENSON: No exposure to planetary science. Planetary science did not exist as a subject. I didn't even know it was a well-defined subject until I came to the United States. Cornell—it's really a coincidence—Cornell happens to be strong in planetary science. At that time, there was only a very small number of places that took planetary science seriously. Cornell was one. Caltech was one. University of Arizona. A little bit at MIT. That was about it! [laughs] Not very much at all. Certainly, in the case of planetary science, it was just a matter of chance that I encountered that at Cornell, because I went there with only vague notions but thinking perhaps about condensed matter physics which was then called solid state physics, which was my main exposure, because those were the best people at Victoria University. I think that's what happens when you go to an institution that has some good people but is in an average sense not especially good. Which is actually true of Victoria University. Victoria University has a small number of outstanding people, but is in some average sense not very good.
By the way, that's part of the specialness of Caltech. At Caltech, everybody's good. [laughs] But at a lot of institutions, it's not true. It's not true at UCLA. I mean, UCLA has people who are as good as the people at Caltech, but it's only a subset of a much larger group. It's a very important distinction between institutions. And it really matters, if you're in an environment where everybody's good. If you're in an environment where there's only a small number of people who are good, and you have enough ability, then you gravitate to the people who are good. [laughs] So that's how I became solid state physics, condensed matter physics, which happens to be useful for planets as well. But other areas? Not much exposure to that, although some of the teaching at the undergraduate level was quite good. But certainly there are a lot of areas of physics that I was unaware of, before I came to the United States.
ZIERLER: Tell me about David Beaglehole. What was his research and why was it so influential for you?
STEVENSON: So, David Beaglehole is an example of the importance of having someone who's outstanding. He had a background that was actually in the United States. He had gone to the University of Maryland. He—I forget now—probably spent also some time in the U.K. But his background was in optical properties of materials, but he had an excellent grasp of solid state physics. He taught a class that I took based on Kittel. Kittel is a classic textbook—I think Ashcroft and Mermin is better, of course—but [laughs] Kittel was the textbook in solid state physics in those days. It still exists. It's a good book. But we went through systematically that entire text. And so he had a big influence, because he was a good teacher, and because he had a good understanding, and he had ideas. And I met him in later years. He stayed in New Zealand. But I think his main expertise was optical properties.
Neil Ashcroft and Metallic Hydrogen
ZIERLER: What was so important about meeting Neil Ashcroft?
STEVENSON: So, Neil was actually born in England but came to New Zealand when very young, and was basically a New Zealander. His mother lived in a suburb of Wellington. He came to Victoria University from Cornell. He died earlier this year. I visited him actually a few years ago in a retirement home. I chose not to visit him last year because by that point, he didn't really know what was going on around him. It was kind of sad. Anyway, Neil was an outstanding teacher, and funny [laughs], and—
ZIERLER: And he came to Victoria as a visiting scholar, or it was a talk?
STEVENSON: He came as a visiting professor. I don't recall exactly what his position was, but he taught a class. That was the second class I took, which probably was quantum mechanics. I don't remember exactly. It may have been some combination, but I remember taking a class from him at the same time as I was doing master's thesis. That was probably just, if you will, for entertainment. It wasn't something I had to do. But I took a class from him. He gave a lecture on Jupiter. [laughs] Which is interesting in retrospect. He was interested in Jupiter, but of course not a planetary scientist.
ZIERLER: Do you remember what he talked about? What was the topic specifically with Jupiter?
STEVENSON: He was interested in metallic hydrogen. And metallic hydrogen, at least if you think about the material, leaving aside the planets, leaving aside the stars, which are kind of boring—I mean, the sun is an ideal gas, essentially; very hot but it's an ideal gas, always—but if you think about planetary matter, which is kind of the interesting stuff, then metallic hydrogen is the most common planetary material in the universe. Surely, because it dominates the mass of Jupiter. We have no reason to think that planets like that are uncommon. Certainly the most common material in the solar system, if you look at planets, most common metal. And he was interested in the possibility that Jupiter's magnetic field might arise because metallic hydrogen, he thought, was a superconductor, at room temperature. Maybe it is! [laughs] It can also be a superfluid.
It turns out that metallic hydrogen is complicated. In the 1970s, I thought everything was simple, and we would have it all figured out in a few years. [laughs] We still haven't figured it out. There are still people who dispute whether we've made metallic hydrogen. It's probably not metastable. It's not like diamond, which is metastable. But, it's a perfectly reasonable idea. He wrote a paper, published it in Phys Rev. Letters. Abrikosov was also involved, famous Russian scientist. Hydrogen, because of the protons being of low mass compared to most nuclei, has a very high Debye temperature. The Debye temperature is characteristic of the energy of phonons, and in BCS theory of superconductivity, the natural scale for the superconducting temperature is actually the Debye temperature, which is thousands of degrees. Then you multiply by the exponential of some number which can vary a lot, and can take the temperature all the way down to millikelvin, so not a simple scale. But still, it's perfectly plausible that metallic hydrogen would be a superconductor. And of course, that's allows permanence of magnetic field, maybe. I don't think that's what Jupiter is doing. Jupiter is too hot. But that's what motivated his interest.
And for me, it was interesting—he also pointed out to me at that time that there was an idea out there about why planets had magnetic fields. The idea was due to Wilhelm Malkus, who I met later. Wilhelm Malkus was a very distinguished scientist at MIT. Malkus published a paper on precession as the cause of this magnetic field. This is no longer believed. But all of this led to the very first thing I did in planetary science, which was to write a summary of our understanding of magnetic fields of planets, a term project for a class given by Carl Sagan. And so I, having already heard these ideas about how precession might drive a magnetic field and other ideas about magnetic fields, I looked into this a bit more, did some calculations, wrote a term paper. Carl Sagan said, "That's nice. Why don't you write it up as a real scientific paper?" And so that was my first planetary science paper. Which of course was published in Icarus, because Carl was the editor of Icarus.
ZIERLER: Now, when you first met Neil, how well formulated were your graduate school interests at that point? Were you thinking about programs abroad, or did the timing work out perfectly where Cornell really came on your radar at the time that you met Neil?
STEVENSON: I don't know. I was thinking abroad. As I've mentioned already, that was what everyone did who was good enough. It was unthinkable to stay in New Zealand.
ZIERLER: But you did have the master's degree? You were pursuing that at the time?
STEVENSON: I applied to Cornell at an early stage of doing the master's degree. The master's degree was done in a hurry and was not even conferred until after I started at Cornell. But yeah, I don't remember the exact timing. Of course, in those days, the web did not exist. The internet did not exist. You had to do everything by sending letters, and letters took forever. [laughs] You sent something away; you might get a reply in a month, so you had to think well ahead. I don't remember the details of that. But it also meant—it limited how many options you might consider. But certainly, I had decided for reasons I already mentioned that I preferred the United States or Canada. I actually thought of Canada. I actually applied to Canada. I thought of those places. Canada, in case you don't know, Canada actually has a system that's not too different from the United States. So I did think about places like McMaster, which is in Toronto. But I did think about places in the U.S. I was probably not as widespread in my thinking as I could have been.
Why did I prefer Cornell? I think that in large part, it was just simply that Cornell was appealing independent of the fact that Neil Ashcroft happened to be nearby. So Neil was of some importance, but it might have happened anyway. Why did Cornell appeal? At that time, and to some extent it is still true, although less true now, Cornell was very highly regarded in physics. Especially solid state physics, but all aspects of physics, Cornell was strong.
ZIERLER: Were you aware of Ken Wilson, even before you arrived?
STEVENSON: I was not aware of Ken Wilson. It was a more general perception. But the other thing was astrophysics. I was aware of Ed Salpeter. I was aware of the—Cornell at that time ran Arecibo. It was very strong in radio astronomy. But it was strong generally in astronomy. It also had Tommy Gold, who I got to know. It had that broad strength. It was a large place. And I don't know, upstate New York was somewhat appealing. It was nicer then than it is now. It was actually my preference, so I was pleased that I got accepted. Neil may have helped. I can't ask him now. I suspect he may have helped. Because in those days, if you were applying from New Zealand, the letters must have mattered. I think Neil wrote a letter. I forget. He may have helped. But I got accepted at Cornell; I did not get accepted to MIT. And that's okay. I went to the place I wanted to go.
ZIERLER: I think that's a perfect narrative pause for our first session today.
[End of recording]
ZIERLER: This is David Zierler, Director of the Caltech Heritage Project. It is August 12th, 2021. I'm delighted to be back with Professor David J. Stevenson. Dave, it's good to see you again.
STEVENSON: Good to see you.
ZIERLER: Dave, I'd like to return to an earlier comment you made, that I think would be important to get some clarification on. And that is, prior to coming to Cornell, you really did not have any exposure or were aware of the field of planetary science. And so, did you study what you would later come to appreciate that would be called planetary science, at all, as an undergraduate? Or not just the nomenclature, but the entire field, the discipline, was new to you when you arrived in Ithaca?
STEVENSON: The entire field was new to me. I knew a little bit about astronomy. I had a little bit of curiosity about Jupiter, although that came from Neil Ashcroft when I was already doing my master's degree. But essentially, I went to Cornell unaware of planetary science as a legitimate scientific discipline.
ZIERLER: So what was the game plan? What were your intentions prior to arriving at Cornell?
STEVENSON: They were vague. I had this vague notion that it would be fun to do something that involved condensed matter physics. I had this vague notion that astrophysics was of interest to me. I knew of one person—that's Ed Salpeter—who did these kinds of things. He wasn't the reason I went to Cornell, but I was certainly aware that that was a possibility.
ZIERLER: You left off the list particle physics, which is interesting, because this was a particularly exciting time for the field.
STEVENSON: I did not know much about particle physics, either. I did not think of that as a direction to take. And I think that in part is ignorance. You know, that's not so unusual. I think it's quite common for people at the time of entering graduate school, quite common for people to have rather little understanding of the field as a whole. I even think that people sometimes make choices too early. And by the way, that's part of why I wanted to go to the United States—because it seemed as though the options were greater. If you went into the English system, which for me would have meant Cambridge, then you had to choose [laughs] right at the outset. I did not feel comfortable with that.
ZIERLER: So it was a good place in terms of going there and really exploring, following your interests, seeing what was compelling to you?
STEVENSON: Yes.
Cornell and Introduction to Planetary Science
ZIERLER: On the cultural side, just arriving in the United States, what were your initial impressions?
STEVENSON: I knew very little about the United States. I came from a country that was influenced by both the United Kingdom and the United States through, for example, comic books and so forth. I had seen Superman comic books and Batman, and so forth. My parents didn't like Batman. [laughs] And some of the popular culture through radio. No television. A little bit of television, just before I left. I had some awareness of U.S. culture but not a great deal. I do remember the what you might call culture shock, of arriving actually in Honolulu. Because in those days, of course, you could not go directly from New Zealand, nonstop from New Zealand to, for example, Los Angeles or San Francisco. That was impossible. You had to go through Honolulu. And I remember sitting at the airport, and there were people sitting in chairs watching television and so forth, for which they had to pay. [laughs] And all of that was new to me. But upstate New York was not hugely different. I mean, obviously the culture is different, but what I saw at Cornell fit in fairly comfortable with my background. It wasn't as big a shock as I think it would be for somebody coming from Asia, say.
ZIERLER: Between your wardrobe and your constitution, how well prepared were you for that first New York winter?
STEVENSON: [laughs] Well, I actually had to buy a really good winter coat. I remember that. After I arrived at Cornell. Of course, I arrived there in late summer. It was beautifully sunny. Cornell is actually quite nice in the fall. It can be miserable [laughs] most of the rest of the year. It snowed on Thanksgiving! Thanksgiving was a novelty to me. I actually went to Neil Ashcroft's house. And there was snow on the ground. So yes, I had to get used to that. And I learned to ski, in Ithaca, which was not easy, because Ithaca is not a good place for skiing. Ithaca does not have a particularly good climate. And the problem is that it oscillates in temperature, so that you get snow, the snow partially melts, it becomes ice, it gets mixed in with the mud. It's actually better further north. [laughs] But you get used to it. When I went back to Cornell to do a sabbatical years later, I realized that it actually wasn't too bad. There was quite a bit of sunshine, even in the winter. But it is true that you get the conditions I described, where it's actually not quite cold enough that you have the right kind of conditions.
Of course upstate New York, especially when you get up to Syracuse and to Buffalo, has a very large amount of snow, and that's called the lake effect. It's because of Lake Ontario. It's not quite so evident in Ithaca. Ithaca, by the way, is still a nice place, but it was even nicer back then. It's a pleasant environment. The lake. The gorges. The area around Cornell. It's really different from Harvard or MIT or even Princeton. It's not rural; it's actually a pocket of progressive points of view, whereas a lot of upstate New York in the political language would be regarded as Republican. It is a nice place.
ZIERLER: What about politically, coming in as the Vietnam War was grinding on, and all of the political tumult from the Nixon administration? How did you deal with those issues on campus?
STEVENSON: Well, the environment at Cornell is certainly to the left of the general environment in the United States. I think that is still true. They had problems, many of which predated the time I was there. There was a famous incident that happened before I arrived, which showed up on the front page of The New York Times, where there were African American students with guns in front of the main student building called Willard Straight, a building I got to know well. But I never saw that aspect. But of course I did see demonstrations. The demonstrations were supported by some of the faculty. I was apolitical, but I was certainly aware of those events, and aware of the generally progressive view, which was against the Vietnam War and the things that followed on from that. I even had to leave the room in the dormitory where I lived because of tear gas or pepper gas that was released in the streets immediately below, at the time of the protests to the Cambodian bombing. I also remember the generally positive attitude—this is years later—but the generally positive attitude when Nixon resigned. [laughs]
ZIERLER: When you got to the department and you were open minded, what was your sense of the things that were most exciting? What was going on with the faculty and other graduate students that might have provided some direction for you in those early days?
STEVENSON: Well, I certainly was attracted to the kind of science that Ed Salpeter did, which was the application of many aspects of physics, but in particular condensed matter physics, to bodies. He had already worked on white dwarfs. And so, I was attracted to condensed matter physics, which was then called solid state physics. But I was also attracted to astrophysics. Those were the things that I noticed most. I was aware that Cornell had a synchrotron. I was aware that there were people starting on the work that eventually led to a Nobel Prize having to do with superfluid helium-3. David Mermin was involved. Doug Osheroff, who was a student slightly older than me, was involved. David Lee was one of the professors associated with that, also Bob Richardson. So I was aware that there was a wide variety of things.
But even though I was a little bit vague about what interested me most, and I wanted to pick that up in classes, I must admit that it was the attraction of condensed matter physics, which Cornell was very strong in, and had people doing lab experiments but also people doing theory. And that was one of the strengths, that it had that balance. It had a balance between experimental and theoretical work. Ed Salpeter, of course, was in the building that was nominally nuclear physics, just along the corridor from Hans Bethe. And so you had the condensed matter physics in one building, and then another building next door that was doing nuclear physics which was merging with elementary particle physics. Then you had a separate building again which was also strong, which was the place where they ran Arecibo, and one of the centers—I wasn't aware, as I said—of planetary science at that time.
When I chased down Ed Salpeter after arriving at Cornell, he correctly told me to do an elementary class rather than one of the ones he was teaching. And so I took a class from Martin Harwitt, which was basically astronomy. And that was fine, since I had never taken anything like that before. But I was at that point mixing things that would have been labeled astronomy with things that were basic physics. The Cornell curriculum, by the way, unlike some schools, is quite flexible, so you have a lot of flexibility in choosing what you want to do. And that worked for me.
ZIERLER: Hierarchically, was Hans Bethe really at the top of the pyramid in the Department, at that point?
STEVENSON: I didn't see it that way. Actually, this was even before he got the Nobel Prize, although the prize was awarded for things that he had done earlier, of course, which is common. It did not seem as though there was really that much of a hierarchy, and I think that's actually good. There were young faculty. There were older faculty. I did take a class from Hans Bethe. I was his teaching assistant for a short while. He was on my exam committee, the committee that decides whether you go on to do thesis work. He asked me a question in that exam, which I answered fairly well, which I think was actually important. Ed Salpeter was there. This was even before I was really working with Ed. Hans certainly had an influence. Hans was highly regarded, and a lot of people wanted to do well in his class. It's the only class I took where you had the sense that there was competitiveness, because you had the sense that people really wanted to do well in that class, because Hans Bethe was teaching it. It was what was called intermediate quantum mechanics, and it was well taught. It was in a large room. There were a lot of students. There were dogs wandering in and out. It's one of the Cornell traditions that there are dogs wandering around [laughs]. I remember a couple of famous occasions where Hans is lecturing, and a dog comes in, and sits on the floor, and listens to Hans! [laughs] So certainly people were aware of his presence and his importance to the physics program, but it wasn't really a hierarchy.
ZIERLER: Dave, of course you wouldn't have anything to compare it to in real time, but in retrospect, the intellectual atmosphere where you have people with interests that brush up against astronomy and solid state physics, does this strike you as a uniquely interdisciplinary or multidisciplinary approach to the field?
STEVENSON: Certainly in what Ed Salpeter was doing. And yes, it's true that the disciplinary boundaries were not strong, and I think that's one of the good things about Cornell. I simply don't know whether that would have been true elsewhere. I doubt that it would have been true at MIT, which might have been another possible place to go. I don't know enough about how things were done at Harvard to make a comparison. Ed Salpeter had an office in the nuclear physics building, but he had people all over the place who worked with him. He was regarded as a good advisor.
And famously, I never saw his other students except at parties. And by that, I mean the students never saw each other. Ed would make a phone call to one of us and say, "Why don't you come over and talk?" And I would go and talk in his office. Which was an interesting experience. But you never saw the other students, except at a party. [laughs] But they were there, and some of them were very distinguished. For example, Bruce Draine, who worked on interstellar matter, became very distinguished, became a professor at Princeton. I only met him by chance after we had both been working with Ed for a couple of years. [laughs] And that was not unusual.
ZIERLER: What was Ed like as a person? Was he approachable? Could you have regular conversations with him?
STEVENSON: Ed was approachable, and you had conversations at a rate that he pretty much determined. It was a very stimulating experience, because you would go into his office, which was not particularly big, and he would be standing, you would be standing, and he would say something that was startling, and that you did not immediately understand. There might be something on the blackboard that was argued over. But it was the kind of situation where you might have to go away afterwards and think about it for a while, to really understand what happened.
Ed was not a good lecturer. It was stream of consciousness. He would arrive at a lecture seemingly a little bit flustered, moving fast, maybe with one piece of paper that only he understood. And things would show up on the blackboard in an order that he might change in real time. Almost no equal signs; everything was approximations. Which is good, but made it harder [laughs] to figure out what was actually going on. He had the reputation of not being a particularly good lecturer, but an outstanding mentor. Because the conversations you had with him were so stimulating. He was certainly friendly, and as I mentioned, he ran parties. He would set up a party at his own home, which was within walking distance, and those were lively occasions.
ZIERLER: Dave, do you have a specific memory of coming to the realization that planetary science was a discipline, and that this is where you'd focus your efforts?
STEVENSON: That never really happened. I was always in the Physics Department. I always had an office in Clark Hall, which is the place where the condensed matter physics is done. That's still true. And Ed was in nuclear physics. And Ed Salpeter actually had a second office in what was called the Space Physics Building, but he never used it. [laughs] And I never went there, except to collect my paycheck or to go to seminars. He went there to go to seminars. But that was the way things worked.
ZIERLER: Was there an official planetary science program at Cornell?
STEVENSON: Cornell has a structure that is different from many places, and it's still there, and it sort of works. And the structure is one in which planetary science is within astronomy. This is not true at Caltech. It's not true at MIT. It's not true at Harvard. It's an interesting and different structure, which leads to a different kind of interaction. And it seems to work at Cornell. I think that what we have here at Caltech and what is very often found elsewhere also works. I'm not sure which is best. What we have, of course, is planetary science together with the people who do earth science. Cornell, at the time I went there, was not strong in earth science. It has become stronger. It has a separate building, which is way across campus, called Snee Hall, which is where the geophysicists and so forth reside. It's an unusual history, because Cornell is famous for doing everything. That's one of the selling points of Cornell, that they do everything. Even figure out how to grow apples.
ZIERLER: [laughs]
STEVENSON: Not far away from the astronomers, because they have a state university aspect as well as being a private college. But they were not that strong in geology. They have become stronger.
ZIERLER: What was the process intellectually, even administratively, in developing your thesis research?
STEVENSON: It's hard to reconstruct exactly how it came about, except that I liked what Ed was doing, and I gravitated towards him. But it took time. The qualifying exam which I mentioned was an important part of alerting him to the fact that I could do that kind of science. I don't think he was convinced earlier. It's perfectly understandable, if you're someone who is viewed as an attractive advisor, that you have to fend people off. And I think he may have done that, to some extent, at an earlier stage. So to begin with, since I was in the Physics Department—he was too, but he did other things—it was more likely for me to be doing something that was conventional condensed matter physics. And I did in fact do something with Neil Ashcroft before I worked with Ed Salpeter.
ZIERLER: What was your project with Neil?
STEVENSON: The project with Neil was to understand the electrical conductivity of liquid metals that arise through complete ionization. And the most obvious case is metallic hydrogen. We actually constructed a theory which was very much consistent with the work that Neil had done previously, based on work originally done by Ziemann in Great Britain, where you'd treat the liquid structure as similar to that of an ensemble of biapoles [?], hard spheres. And that gives you a particular structure factor, as it's called, which is just a description in Fourier space of the distribution of the protons, in the case of metallic hydrogen. And then you have a theory for the interaction between the electrons and the protons, and that can lead through [?] a theory originally developed by Ziemann for the electrical conductivity. We came up with a fairly high value. It's not clear whether that's right. We also came up, or I came up, with ideas for the pressure above which hydrogen becomes a quantum fluid, meaning that it melts at absolute zero because of zero-point motion. This work was motivated, I suppose you could say, a little bit by Jupiter, but I wasn't really thinking about Jupiter that much at the time.
ZIERLER: When Ken Wilson was developing his ideas on renormalization, was there a general sense in real time just how revolutionary this thinking was? Or did that process happen more gradually?
STEVENSON: I'm not sure how we realized it, but it's clear that we knew something revolutionary was happening. It might have come from somebody like Michael Fisher, who was in the chemistry department, another example of the strength of Cornell that you had people like Fisher, who was basically operating like a physicist but in the Chemistry Department. That's still true, with people like Roald Hoffmann. It's something special and very good about Cornell. Somehow, we as students, I can remember this even in the first year, we became aware that something special was happening. And I can even remember Ken Wilson was also in the nuclear physics building, where Ed Salpeter was, and Hans Bethe was. I can even remember going over to that building with another student, perhaps at the end of the first year, to get a copy of stuff that had not been published yet, that Ken Wilson had written. I don't think we talked to Ken directly. We must have talked to a secretary or someone. But anyway, we got a hold of the notes, because we knew that something special was happening.
The story is that Ken Wilson, who had worked with Murray Gell-Mann, wanted first to understand elementary particle physics, but then realized there was an easier problem [laughs], which is the Kondo effect, which is in condensed matter physics. And so, the renormalization group ideas moved over to an application to critical point phenomena, where Michael Fisher was an expert. So it was very much a Cornell story. And I can remember well when the seminar course was set up, and you went into the lecture hall where Ken Wilson was to introduce the subject, and sitting directly behind me was Neil Ashcroft and John Wilkins and so forth. You knew something special was happening because it wasn't normal for other professors [laughs] to show up at a class.
ZIERLER: What was Ed's style as a mentor? In other words, for your thesis research, did he give you a problem to work on that was related to his research agenda? Or he let you go off on your own and present him with possible ideas?
STEVENSON: He certainly gave me the idea of looking at the question of how hydrogen and helium mix. But he didn't really tell me how to do it. I had to figure that out for myself. Ed had worked on the possibility that helium settling in the giant planets was an important energy source, and he wrote a paper. I can remember at that time I was not working with Ed, but I can remember seeing him complete that paper. In those days, you had to physically go to the library to check on references, because of course there was no computer source of information of that kind. And I can remember seeing him in the library. And he was actually responding to a very famous scientist who wrote on that subject even earlier, by the name of Ro Smoluchowski, who I met later. He was a professor at Princeton, but of course also came from Europe and was Jewish. So Ed was responding to Smoluchowski, and wrote a paper in Astrophysical Journal. And he knew that a key question was unanswered, and that is whether helium would be insoluble in hydrogen when hydrogen was a metallic liquid. And so that was essentially what he was suggesting to me.
He did suggest an interesting piece of information that he knew about, which turned out to be less important, and that was that Freeman Dyson, who of course he knew, at the Institute for Advanced Studies, another Cornell connection actually, Freeman Dyson had worked on the lattice energy of a set of protons and alpha particles, the fully ionized state. And Freeman had shown that you actually had a lower energy state if you made an alloy. That turns out not to be relevant, because if you fix the pressure as you must to minimize the Gibbs free energy, the electron density changes as you change composition, and that's key to understanding how the total energy changes. This was subtle.
But that was my PhD thesis work. And it was a lot of work. And quite a bit of it, I had to figure out for myself. Although I know that some of these ideas about how to construct phase diagrams were things that Ed knew; it's not as if it was something completely new. Because I can remember him once giving a seminar about metallic hydrogen and the possibility of it being metastable. And he talked about Gibbs energy, and I had not heard about it previously. In physics, you don't for the most part learn about what Gibbs did. Gibbs, in my opinion, was the greatest American-born scientist of all time. There's no close competitor.
ZIERLER: Wow. Can you tell me why, Dave? That's high praise. What makes him the number one?
STEVENSON: Gibbs set down the framework for everything that we do in chemistry to decide on phases and composition. So when you decide where elements go in some mixture, it's Gibbs energy minimization. If you want to know what the Earth's core is made of, it's partitioning between liquid iron and the silicate that makes up the rocks which we call the mantle. That's Gibbs energy. That's all about Gibbs energy. People don't on all occasions mention Gibbs, but he did it, at Yale, around about the time of the American Civil War. Of course he has a postage stamp [laughs] but a lot of people in the general public have never heard of Gibbs. And of course he did some mathematical things as well—the Gibbs phenomenon and so forth. But the truly extraordinary thing, which he wrote out in an obscure way in the Transactions of the Connecticut Academy, of all places—I have a copy of it somewhere. [laughs]—it talks about how you decide about chemical potential. The idea of a chemical potential comes from Gibbs.
ZIERLER: Dave, when did you first interact with Carl Sagan? And was he already famous at this point?
STEVENSON: At that point, he had not yet appeared on the Johnny Carson show, which was the very well-known late evening TV program, The Tonight Show. And so Carl was known in a particular scientific community. He had been denied tenure at Harvard and came to Cornell. And he was known to be interested in the question of life in the universe, moderately well-regarded as a scientist. But he became famous in the general public through Johnny Carson's enthusiasm for astronomy. And so when he first showed up on the Johnny Carson show, I saw it—I had to wait until 1:00 a.m. in the morning [laughs] because he was the last person on the show, and there wasn't much time left. In those days, The Tonight Show went until 1:00 a.m. Eastern Time. And finally, Carl was there. And he has a very good presence, which works well with the general public. And Johnny loved him and brought him back. And he could subsequently talk about the Viking mission, which he was involved in, which went to Mars. And he had a colorful way of talking about the nature of the universe and life in space, and so forth. And many years later, of course, there was the Cosmos TV show, which was actually done in Los Angeles. So he became much better known, still.
But I did have a lot of interaction with him in later years, as well as at Cornell. But at Cornell, it was just taking a class from him. And he was responsible for the first thing I did, which led to a completed piece of work in planetary science, because in his class, he set a term project, and I wrote up a discussion of planetary magnetic fields which included some new stuff. And he liked it, and he said, "Well, you should publish it." And so I wrote it up for the journal for which he was editor, which is Icarus. So I already got to know him as a student.
ZIERLER: I'm looking for that dramatic narrative turn where you commit intellectually, academically, to planetary science. Is it Carl Sagan's influence? Is that part of the story?
STEVENSON: It's part of the story, but I think it's mainly Ed Salpeter and the fact that he was temporarily interested in giant planets.
ZIERLER: Who else was on your thesis committee?
STEVENSON: I don't remember all of the membership. And the committee changed. There was a committee that decided whether I should continue to do a thesis, and that's a qualifying exam. And at that point, you don't have a thesis. And that certainly had Hans Bethe and Ed Salpeter and Neil Ashcroft. And there must have been one other person whose name I don't remember. In the final exam, it would have been Ed Salpeter, Neil Ashcroft, probably Bob Silsbee or somebody like that, in—I think somebody who did infrared work. It would have been a solid state physicist. But no, I don't remember.
ZIERLER: How did you get involved in the complex work that was run by the National Research Council?
STEVENSON: Jerry Wasserburg, who was of course a professor at Caltech and very well known in geochemistry, was chair of that committee. And he was looking for expertise in the area of giant planets, and he asked Ed Salpeter. And Ed said, "No, I don't want to do it," which is very typical of Ed, although he did work on JASON. [laughs] That's a rather special committee. Ed said, "No, I don't want to do it. Why don't you try my student?" So [laughs] something very unusual happened. Because normally that committee would be people who already have established their careers or are well into their careers. This is the early days of planetary science. This is perhaps 1974 or thereabouts. And so I'm sitting in my office at Cornell, in Clark Hall, and I get a call from this guy I know nothing about, poor Jerry Wasserburg [laughs], and he persuades me to be on the committee. It wasn't that hard for him to persuade me. It sounded very intriguing.
And it was a kind of important committee. It was an interesting and eye-opening experience that required traveling to Washington D.C. and to Seattle, maybe elsewhere. Eventually I had to quit the committee because I left the United States. But I did get involved in discussions that concerned the future of planetary exploration in a fairly major way. At that point, there was a controversy or a conflict between the Jet Propulsion Lab and NASA Ames, which had to do with the kind of spacecraft that you might send on a future mission to Jupiter, the mission that eventually became Galileo. These things happen over a long period of time, so discussions about these things were already taking place in the mid 1970s. And the difference was that JPL was putting forward missions that were three-axis stabilized spacecraft that were good for imaging, not so good for magnetic fields. They already had expertise in that area. Of course, some company was building the spacecraft, but it was a question of instrumentation and so forth. NASA Ames was more in the Pioneer mode, which are spinning spacecraft. And there was advocacy for what you would call a magnetospheric mission.
On that committee that Jerry Wasserburg chaired was Jim Van Allen, who I got to know. In fact, we had a meeting right here at Caltech, in this building even, that I'm sitting in right now, in the mid 1970s, when I was still a student. And Jim Van Allen wanted a mission that went way out from Jupiter, measured the magnetic field. I mean, magnetospheres have all sorts of wonderful phenomena, and so you can imagine people advocating a mission of that kind. And this was in the context of a period where for many people the Galilean satellites were not interesting. [laughs] Nowadays that sounds ridiculous, because they're planets in their own right. They have all sorts of fascinating stuff. Io has volcanism. Ganymede has a crazy surface. Europa has an ocean underneath the ice. Ann so on and so forth. And Callisto is the ugly stepsister who does its own thing. But back there, they were just fuzzy balls. And so it was not so obvious that you should devise a mission, as we eventually did, that got really good images of the satellites and stayed close in to Jupiter. That was an important decision. We were also involved in discussions, as always happens with NASA, about the funding of a mission to Venus, called Pioneer Venus, and whether or not that would get cancelled and so on and so forth. You can debate whether COMPLEX, which now has a different name—I'm still on it [laughs], which is ridiculous [laughs]—not all that time, but I'm on it for the third time. You can debate whether those committees have a lot of effect. There are some people who say with cause that it's a waste of time being on committees like this, because what happens is that you make reports, and the reports get filed away, and NASA can say to Congress that we asked the opinion of scientists and so on and so forth. But there are occasions where it can be important, and I think that was actually an important time.
ZIERLER: Who was driving the most important research mission that NASA wanted to do? What was your sense in the mid 1970s of those big decisions with regard to planetary science and research?
STEVENSON: I'm not sure that there was a single person. Certainly there was interest in Mars, and Carl Sagan was involved in Viking. He was a prominent figure by that point. I don't know that you can—I mean, it could just be that I was too young to really know who were the power players. I'm not sure that there's anyone in particular.
ZIERLER: After you defended, what opportunities were available to you? And given your status, what were you trying to accomplish?
STEVENSON: The world has changed enormously. Back then, the number of positions that could be called planetary science could be counted on a single hand. Very small in number. The field was young. There might have been a number of people who were hired in the late 1960s, including at places like Caltech, but the number of departments that did anything that could be called planetary science was small, so the opportunities were small. I did not find that depressing, because somehow at that point, I just wasn't too worried. I enjoyed doing science. I wasn't focused that much on concerns about career path.
But I had come to the United States with support from the Fulbright program, which is an international program that supports people within the U.S. to go elsewhere, and importantly, supports people outside the U.S. to come to the United States. One aspect of that program is travel support. I came to the United States with money provided by Fulbright—travel support, not a stipend, which was what Cornell gave me, to be a teaching assistant additionally, and subsequently research. I knew although I did not really understand the legal aspects, which were murky, I knew that there was this expectation that I'd go back. But what did "go back" mean? If you're in the United States, and looking at a map, and you don't know much about geography anyway, Australia and New Zealand look more or less the same thing. [laughs] So when a position was advertised in an area that did not look much like what I had done, geophysical fluid dynamics at Australian National University in Canberra, Australia, I applied. It's the only application I made. And I got it. I didn't think about it that much. I wasn't trying that hard to find a planetary science position in the United States at that particular point, because of this notion that you should go back. And so that's what I did. Although of course within one year of going to Australia, I was coming back to the United States, traveling around, trying to get people interested in me, and finding a position, which I did at UCLA.
ZIERLER: I'll ask the same question that I did at the outset of this conversation, when you got to the United States. When you got to Australia, culturally what were some of your experiences? And what might have been even disorienting, given that New Zealand is close and yet still far?
STEVENSON: Well, certainly Australia was much more like New Zealand, but again, somewhat different. In those days, more so than now, more cosmopolitan, more like the United States. I think the transition of living in Australia was actually in many ways easier, because it was intermediate between what you see in the United States and what you see in New Zealand. So it was not really that difficult to figure out how to live in Australia. Of course the physical environment is different. Australia—Canberra, that is—is very hot in the summer. It's actually quite cold in the winter. And there are interesting native species, so I had interesting close encounters with snakes [laughs] which was novel, and got to know the natural environment. Once saw a koala in the fork of a tree, just driving down a road, and pulled over, and there was the koala, happily munching away in the tree, totally unconcerned about its environment. Things like that.
ZIERLER: What was your home department at ANU?
STEVENSON: Ah! That was the Research School of Earth Sciences. I think it was a matter of luck, serendipity, that I applied there because they had just hired a very distinguished geophysical fluid dynamicist, Stewart Turner, who had worked at Cambridge, in England. Had worked with G.I. Taylor, a lead person in the world in a particular kind of fluid dynamics, which has some application to planets. So that was the connection.
ZIERLER: Did you see this as an opportunity to expand on your thesis research, or to take on new research areas?
STEVENSON: I did take on new things. I got involved in thinking about how the Earth works, how the Earth cooled off over time, a subject that is still unsolved. Somewhat controversial, at the time. Worked on aspects of plate tectonics. The Research School of Earth Sciences at that time had a tradition that is in many ways an English tradition, of tea, morning tea and afternoon tea. And at that time, that meant meeting people in the morning, and out of that came some work that was collaborative about how big G—Newton's gravitational constant—might have varied across geological time. And I was able to provide some theoretical constraints for that on top of the paleomagnetic constraints at that time. The Research School was a world leader in understanding paleomagnetic data, so there was a good combination. And I got to know a very distinguished and very distinctive scientist, Ted Ringwood, one of the world leaders in geochemistry. Got interested in the origin of the Moon, because he was interested in that. So it was a stimulating time, and it was an opportunity to do things that were completely unrelated from my thesis.
ZIERLER: And another nomenclature question at ANU—geophysics, earth science, planetary science—how are these terms used there?
STEVENSON: Well, certainly there wasn't planetary science as we know it now. There were people who were interested in the Moon, because they had been involved in the Apollo project. The Apollo program, which was mostly in the United States, actually involved scientists in Australia and to some extent in Europe, through the need to look in detail at the rocks. The Apollo program brought back a ton of rocks, a lot of which are still sitting there in Houston. You could analyze them. The Research School of Earth Sciences had the ability to do sophisticated analysis. They still do. And so people were interested in the Moon. They were a little bit interested in other planets. Ted Ringwood had some interest in Venus, for example. But mainly it was geophysics and earth science in particular. And so I got to learn about that subject.
ZIERLER: What was Stu Turner working on at the time you connected with him?
STEVENSON: He had started up some laboratory work at Australian National University. There was a student there, Ross Griffiths, who I got to know. The work was in many cases what we called double-diffusive convection, which is a bit of a mouthful. But the essence of it, the common feature of all that work, is the need to understand how fluids flow when there is something else in the liquid that changes the density. So if you have something dissolved like salt, or sugar—remarkably, I had even done a bit of work like that as an undergraduate in New Zealand, back in 1970. I did not get involved much in that, except of course that it has relevance to understanding planets. And astonishingly, has become perhaps even more important in understanding how Jupiter works, how exoplanets work. There is a common feature there. The common feature is how to understand fluids where the density is affected by temperature which is the obvious thing to think about, natural convection, but also how the fluid behaves if the density is affected by composition and if you have both things present. If you have, for example, salty water that is cold, and less salty water that is hot, these things have different densities. They can even have similar densities. If it's hot salty water and cold fresh water, do they mix? How do they convect? Questions like that.
ZIERLER: Did you want to return to the United States because you wanted to pursue more work in planetary science? Was that simply not available in Australia?
STEVENSON: Yes. The United States was the natural place to be, if you wanted to do planetary science. I think in retrospect, you could have imagined doing some of the same things, staying in Australia, although you would have had to do it as an isolated scientist. There are people who do that successfully, especially in New Zealand, but also in Australia. Maybe in France. France has quite an active community in people doing planetary science, in those days, linked somewhat to the Soviet Union, which still existed, which still had a substantial program. Going to Russia was not an option. The United States had a strong program because of NASA, and that was attractive. I have to admit that for me, my interests were somewhat ivory tower, somewhat abstract. I didn't actually feel as if I had to be associated with missions. I've come to appreciate the importance of missions, and the attractiveness of being involved in any doing measurements. But certainly being in the United States, at one of the top institutions in the United States was attractive. That was the place to be.
ZIERLER: Did you recognize at some point that your academic interests means that you would make a life for yourself in the United States? That you would not be going home to New Zealand?
STEVENSON: I think that I realized that, although I didn't give it much thought. To me, science is so important to what I find interesting in life in general that that's what drove my decisions. And so I didn't feel a great deal of tension, even though New Zealand is an attractive place to live. It's a nice place to live. And of course in later years, I got offers. I was offered the opportunity to be a professor of geophysics back at the school where I got my undergraduate degree, in Wellington. I was offered a similar kind of position, informally, in Auckland, which is even bigger. But they're not attractive to me, because I'm in the country where the most interesting science for me is being done.
Advice from Freeman Dyson
ZIERLER: Dave, tell me about your interactions with Freeman Dyson at the Institute.
STEVENSON: Freeman Dyson is—was; he's no longer alive—a fascinating character. I knew of him because Ed knew him, and because he had spent time at Cornell, although when I was at Cornell, of course, he was at the Institute for Advanced Studies. Freeman knew about me through Ed, and maybe through my work on Jupiter, which had some relationship to things he had done, and when I visited the United States from Australia in 1977, I had lunch with him. It was a strange experience. I was at Princeton, and I gave a seminar. That's also where I met Ro Smoluchowski and his family. He had a house within walking distance. Princeton is nice that way. And so I was staying overnight at Princeton, and had agreed to meet up with Freeman at the Institute for Advanced Studies, which I thought of as being just down the road. [laughs] And so I set out to walk to the Institute of Advanced Studies, which of course is not difficult to do, if you know the right direction. [laughs] But I actually got lost. It was—amusing. And eventually I found the Institute. I was somewhat flustered at that point. Freeman was pacing up and down in front. And so we met, and we went inside, and we had lunch, and Freeman said to me, "Why don't you think about objects that are like Jupiter but more massive?" And of course nowadays that would sound completely natural, but at that point, this was a novel idea. There was one other person who had worked on such objects and had even modeled them, and I met her later. That was Jill Tarter, and she became director of the SETI Institute in the Bay Area.
ZIERLER: Dave, what advances in observation would have made Freeman's advice feasible at all?
STEVENSON: So that's the amusing part about it. At that point, there were no observations! [laughs] The observational story blew up a decade later, and became brown dwarfs. In the early days of brown dwarfs, we would all joke, because what would happen is that somebody would make a huge splash, and they would publish a paper saying, "I found a brown dwarf!" and then a year later, they would say, "Oops! [laughs] It wasn't a brown dwarf after all." But then finally, they actually survived tests. We still talk about the brown dwarf desert.
There's a very interesting phenomena that I still think is not fully understood, which is that when you go to really low-mass objects and try to make them the way we make stars, that there aren't a lot of them. I'm talking about objects that lie in the mass range between the bottom of the main sequence where the number is already dropping off, and things that might be called planets, which would be ten times the mass of Jupiter. Just to set the scale, if you had ten times the mass of Jupiter, then this is of significance because that's the onset of deuterium burning> It's much easier to have thermonuclear emission with deuterium than with ordinary hydrogen. Then you have to go up to 70 Jupiter masses, and that's the bottom of what we call the main sequence, to find objects that burn light hydrogen, as does the sun. And then of course you just go up to much more massive objects. The sun, by the way, people think the sun is an ordinary star. Most stars are less massive than the sun. The sun is a thousand times the mass of Jupiter. Anyway, brown dwarfs are bodies that are like Jupiter because they don't have much thermonuclear reaction, and so they cool to become degenerate bodies. Degenerate is a condensed matter physics word. It means that the support against gravity is provided by the Fermi pressure of the electrons.
ZIERLER: As a gauge in the maturity of the field in planetary science, as you were going on the job market, were there positions available? Were there distinct planetary science departments that were hiring at this point?
STEVENSON: I did not try very hard, so I'm not really sure. But I certainly had the impression that there were very few positions. And I think that was the nature of the times. The NASA funding had declined. The number of people going into the field was fairly small. If you went to a conference on planetary science, you could put everybody in one room. So it was very much a different time, and the science was different. It's funny to reflect on that. It's very much the case that even though the jobs were few in number, the opportunities in some sense were substantial, because there weren't many people doing things. There wasn't a lot of competition, either.
To compare it with what happens now, what I see now, is a very large number of people wanting to do it. The number of positions has grown dramatically, but the number wanting to do it has grown even more. [laughs] So you talk to a young person now; they feel under considerable stress, and they're in postdoc positions. And there are a lot of postdoc programs. There's a lot of funding now. It's really quite remarkable. Before I was talking to you, I was on a Zoom conference with people involved in what is called 51 Peg, which is a program for people who do exoplanets. There's lots of people, and they get full funding for several years. There are other programs. There's a Carl Sagan program. There are various other fellowships. There are opportunities for people in excess of the positions available at universities, or the positions available at NASA centers. So it's much more competitive now. It's a more difficult field in some ways, more mature. Although there are abundant opportunities in observation, because an explosion of information about exoplanets has revolutionized the subject. Now, lots of people are looking at spectra, looking at various aspects of planets around other stars, all sorts of things that you couldn't even think of doing, back in the early days of planetary science.
ZIERLER: And what was available to you? Where did you end up going?
STEVENSON: What was available to me was at that time from an observational point, a little bit of data from the Pioneer missions, which had gone past Jupiter and Saturn. And there was the beginning of what was really quite a major development, which did not happen—well, was starting to happen in the mid 1970s, but didn't really fully blossom until the 1980s—and that was Voyager. Voyager was arguably the most successful planetary science exploration of all time, until now, perhaps ever. Actually run by Ed Stone, who I knew, and who was—is—a professor here at Caltech. And two spacecraft that visited Jupiter and Saturn. One of them visited also Uranus and Neptune. That was an explosion of information about the planets in the outer part of our solar system.
There is an interesting story that I have to relate, related to the Voyager mission. The concept of the Voyager mission was originally something called the Grand Tour, and it was conceived by a Caltech student working at JPL in the 1960s, somebody I met only a year or so ago. His name was Gary Flandro. He figured out that the gravitational slingshot effect, which was already known—it was known by the people who worked with the Nazi program, with Wernher von Braun—they published on it. So that idea of going to the outer part of our solar system was already there as a theory in the 1960s. And Gary realized that the configuration of the planets could enable you to go Jupiter, Saturn, Uranus, Neptune, and even Pluto. They didn't do Pluto. [laughs] And the whole thing got delayed a bit. It was never really a grand tour. But the Voyager program grew out of that idea, so it was very much linked to Caltech. Of course I didn't know these things until much more recently.
ZIERLER: Tell me about the opportunity to join the faculty at UCLA.
STEVENSON: UCLA was advertising a position in honor of Bill Libby, who had worked in the Manhattan Project. That position was not in Planetary Science specifically; it was more broadly advertised, and it was not a tenured position. It was a fixed-term position, but otherwise had all the attributes of being an assistant professor. I applied to that, because at least, as far as I could tell, it was the only thing at that time that was attractive. This would have been 1978, perhaps. And I got it. I learned later from Leon Knopoff about some of the competition for that, which included people who were doing earth science. So I ended up at UCLA in 1978 after spending two years at Research School of Earth Sciences in Canberra.
ZIERLER: What department did you join at UCLA?
STEVENSON: That was the Department of Earth and Space Science. I had someone who took an interest in me. He had met me already at a famous Jupiter conference back in 1974 or 1975. His name was Bill Kaula. He was a famous geodesist, no longer alive, and he was in what we would now call planetary science, although he also did things that could be labeled geophysics. Certainly he was one of the pioneers in geodesy, which is a central technique for understanding satellite orbits and understanding the gravity of planets.
ZIERLER: Did you enjoy Southern California?
STEVENSON: Yeah, Southern California is different from upstate New York. I got used to Southern California. The climate is nice. [laughs] Rode my bike back and forth from an apartment I had on Bundy Drive, the same drive that O.J. Simpson made famous years later. A different part of Bundy Drive. Riding my bike through Westwood, through what was advertised in those days—it might still be true—as the most congested part of the United States. Traffic was enormous. Along Veteran Boulevard, and along Westwood, it's just an enormous number of vehicles, six-lane roads, and so forth, so somewhat of a challenge to ride your bike. But UCLA was nice, and it was an interesting experience. I even taught a basic class in Earth science, even though I had never taken a basic class in Earth science. So I stayed ahead of the students by reading an appropriate textbook by Press and Siever and of course started on some important scientific projects.
ZIERLER: Dave, now that you're in your first faculty position, I wonder in what ways you thought your own educational trajectory, which was in the Physics Department at Cornell, in what way did that background serve you well in these new fields of research? And in what ways were you perhaps playing catch-up in ways that you might not otherwise would have, had this been something that you pursued more centrally in graduate school?
STEVENSON: Well, I didn't feel uncomfortable. It's interesting—my background is indeed physics, but I had some inclination towards the kind of science that is central to understanding how the Earth works. I had been interested in that already. It just seemed like a natural fix. It's true that you have to learn, and I did learn, some basic geology, the nature of various rocks and so forth. But it was a comfortable enough transition and I think it worked out fine.
ZIERLER: A rather fun question—I'm not sure how concrete an answer you can provide, but when you have on your research agenda Saturn, Jupiter, Earth, Mars, in what ways do you treat Earth as just another planet in the solar system? And in what ways is it most obviously not that, because it's our home, and it's close to us?
STEVENSON: When I work on the Earth, I treat it as just another planet, and I think of it that way. The difference comes when you compare with data, and you can take advantage of the fact that there are aspects of the Earth that we know much better. The most obvious difference is that we have seismology. Of course, we are starting to get seismology in other planets. But in the case of the Earth, we had a great deal of information about the density distribution, about the existence of a rather sharp interface between the core and the mantle, about the behavior of water and so forth. Things that you simply did not have for other planets.
But a lot of the basic questions, at least at that time, perhaps still, were not answered, are not answered. Like exactly what goes on with Earth's magnetic field? How has it behaved through time? How does plate tectonics work through time? How does the heat flow change with time? These are sort of the kinds of questions that can be asked for the Earth and for other planets, and you tend to approach them with a common point of view.
The Uniqueness of Earth Below the Surface
ZIERLER: Beyond obviously the biosphere, beyond the obvious fact that Earth supports life, below that, below the surface, what do we know about how unique or not the Earth is, relative to other planets?
STEVENSON: Ah, it's an interesting question. I don't think we know the answer for certain. The suspicion would be that the existence of life on Earth does not have a profound effect on the nature of the Earth at depth, but the truth is we don't really know. When I say at depth, I mean of course much deeper than ten kilometers. We have reasons to think that there are organisms that live beneath Earth's surface, but conditions become pretty difficult for life once you get deeper than about ten kilometers. There may be some consequences for the recycling of material, for the isotopic makeup of the Earth. Certainly people who are interested in the history of life on Earth look at the isotopic makeup of material on Earth, and some of that gets recycled. So there may be consequences for the relative abundance of carbon isotopes, for example, which are affected. Certainly there's no doubt that the biosphere, that the existence of life on Earth has had a profound consequence for the nature of Earth's atmosphere, and for the nature of the ocean, although somewhat less so, and for the nature of the sediments that we have. There are a lot of sediments on Earth that have been affected by biological activity. In that sense, the surficial Earth is profoundly affected by biology. In fact, you could argue that in a certain sense, the existence of life on Earth is relatively easy to find. It's not clear that that's true for Mars or any other planet.
ZIERLER: Is it then, in your view, too great a leap of logic to deduce that if the Earth is unique in its ability to support life, by definition wouldn't that mean that its deep interior must be different than other planets? Or are those perhaps unconnected?
STEVENSON: I think it's unconnected. I don't see a major link. Why? Because as I see it, life as we know it, which exists at fairly low pressure and makes use of water in its liquid phase, is a property of the surface environment, and that environment is not much affected by what goes on inside the planet. It is somewhat affected. Volcanism matters. Plate tectonics matters. But those are still surficial aspects of how the planet behaves. And they may even have some commonality.
You're asking a very interesting and perhaps profound question. And I have thought about this. But I'm hesitating with my answer because I don't think there is one, at least not one that I'm comfortable with. It has been suggested—I've even suggested it myself—that plate tectonics is an important part of life. Plate tectonics produces variation. You might argue that life, or at least the particular way that it developed on Earth, is affected by the presence of change. If you had a planet that was boring, meaning that it had a very constant yet suitable environment for life, then maybe life would behave differently. I mean, if everywhere was like Southern California, that might not be good. I don't know. That's just speculation, because we don't know.
I do, by the way, have rather strong opinions about notions of habitability. I think that people make too much fuss about this. I think that the notion that the Earth is somehow particularly well suited is an extremely anthropocentric point of view. I don't see why we necessarily should exclude life in the clouds of Jupiter. By the way, there's a book written about this, and there was a paper that Carl Sagan asked me to review when I was a student at Cornell, on exactly that subject. There's a paper written by Carl Sagan and Ed Salpeter. It's a very scholarly paper about life in the atmosphere of Jupiter. A lot of people don't know about this. I don't see anything wrong with that. There's plenty of water in the atmosphere in Jupiter. It's pleasant if you choose the right level. Of course you might get carried by convection to nasty places, up or down, so it's not obvious that this would work. But I think there's a problem if you're really hot.
It's not clear to me that there's a problem on Pluto, because inside Pluto, you have oceans, you have energy sources. Not as much as sunlight, but—the bottom line is that I don't think the Earth is that special. But of course once you get into this game of talking about life, you're talking about things that are highly speculative. We're just going to have to wait for discoveries.
ZIERLER: While we're in that neighborhood of pure speculation, what has your research suggested about the possibility that life formed on Earth from alien sources? In other words, from microbes or bacteria, from asteroid impacts and things like that?
STEVENSON: I think it's a possibility. I have a colleague, Joe Kirschvink, who thinks that we came from Mars. I argued this once with Sam Epstein, who was a very distinguished geochemist here at Caltech, did some of the pioneering work on carbon and oxygen isotopes and many other things. My argument with Sam had to do with the idea of a magic bullet. My definition of a magic bullet is something that comes from space that is the seed for life. This is a metaphor, of course. What I said to him, and what I would still say, is that when I think about the early Earth, I think about all sorts of possibilities happening. And when I think about stuff from space, I think that a lot of it gets vaporized upon arrival, and not suitable. [laughs] Obviously if I send in a meteorite, we know what happens to a meteorite. A meteorite survives through the Earth's atmosphere. Organisms can survive within meteorites. But meteorites are not typical delivery to the Earth. And why should you bother anyway? Because that's just a small fraction of the stuff delivered. So I see a richness, a diversity of environments and process in the early Earth that could lead to the things that might be necessary to make structure, and I don't see the need to invoke something from space. That of course is not an argument about exclusion. It's just an argument that perhaps life could start on Earth of its own accord.
ZIERLER: Back to the world of observation and away from speculation, what were some of the advances when you were at UCLA to study Saturn and its magnetic field?
STEVENSON: We already had some data, and I suppose it must have been Pioneer. I actually don't remember the details. It certainly wasn't radio astronomical, although Saturn's magnetic field was actually detected from Earth orbit before we went there. It was the IMP-6 spacecraft, but that would not have given the dipole tilt. Certainly one of the most important things that I did scientifically was at UCLA—began certainly at UCLA and was mostly at UCLA—and that was the thought that you could explain why Saturn had a magnetic field that was almost aligned with the spin axis, did not have a dipole tilt, and therefore was different from Jupiter, different from the Earth, certainly different from the sun. And that was because of a fundamental physical effect that you could create in the lab in which if you placed yourself in a frame of reference where you saw the field as time-varying, and you had a conductor, then through the electromagnetic skin effect, you could attenuate that field. This is a well-known consequence of Faraday's laws. And so I must have already started thinking about that at UCLA. It was completed at Caltech. I remember giving a seminar at Caltech. But that was certainly something that I did at that time that was different from worrying about whether helium could form rain.
ZIERLER: Dave, tell me about your interactions with Gerry Schubert and your work on thermal history of terrestrial planets.
STEVENSON: That was certainly something that was primarily linked to my two years at UCLA. I don't remember the specifics of how it began. I do know that it was not entirely Gerry Schubert; it was partly me. Because I was already interested in thermal history. Already when I was in Canberra in Australia, I had tried to understand how the Earth cooled through geologic time, and so I had already been thinking about how convection works in a solid. But Gerry was interested in the same kind of thing, and so the project developed in which we coupled the behavior of the core of the planet to the convection in the overlying mantle. Exactly how those ideas developed is no longer clear to me, but it certainly led to the recognition that you might be able to build a story about the different magnetic fields observed for the terrestrial planets—this was work motivated by Mercury, Venus, Earth, and Mars—that you might understand their differences and similarities through thinking about the nature of their cores and the extent to which the core could cool because of the overlying convection in the mantle.
And in developing that story, it became apparent to me that Venus could be different from the Earth, because Venus did not have an inner core. Earth does. And we know, or at least we think we know [laughs], that right now, a key aspect of understanding why the Earth continues to have a substantial magnetic field is the presence of the inner core. The inner core, as it grows and as it excludes light elements, providing a gravitational energy source, together with latent heat, the inner core as it grows provides an energy source for Earth's magnetic field. There are other ways of explaining Venus, none of which involve its rotation rate.
People always mention the fact that Venus rotates slowly. I don't think that's relevant. If anything, slow rotation is good for a dynamo. But the difference between Earth and Venus having to do with the slight difference in mass, slight difference in temperature, different convective regime which is a later idea, actually—these things were beginning to be part of my thinking about how these planets behave. Gerry Schubert was crucial. He was important in encouraging this, and he also played a central role in something that I'm bad at, which is that sometimes I do science and then can't be bothered writing it up. And so I can remember that at the time I left, or around about the time I left UCLA to come to Caltech, he was encouraging me to write it up. And basically what ended up happening is that somebody else wrote it up. [laughs] And that was Tilman Spohn.
ZIERLER: You were scooped.
STEVENSON: No. Tilman was a postdoc with Gerry Schubert, but he became an independent—he's a quite accomplished and well known scientist. For many years, he was in Berlin. He came of course from Germany. He is now, or at least recently, was the director of the Institute for Space something-or-other in Bern in Switzerland. Anyway, he's quite well known in the field.
ZIERLER: Dave, you mentioned earlier that there was some controversy surrounding research on the Earth's core. What were those disagreements, and where did you fall in those discussions?
STEVENSON: I think that it's not clear anymore what was really at the heart of the controversy. Certainly when I was in Australia, I can remember writing a paper about cooling of the Earth, and sending it to Nature and having it rejected. The first time I'd ever been rejected by Nature, and one of the only times ever. It was probably Dan McKenzie who was [laughs] responsible for the rejection. Dan McKenzie, a very famous scientist, who I met and got to know much better later on. I think part of it—but there were other people. There were people like Dick Peltier in Canada and other very distinguished scientists. Here's what I think was happening. What I think was happening is something that usually did not happen to me, which is that several people were thinking about the same problem at the same time.
And that's a recipe for controversy. [laughs] If you have several people who think that they know how something works and they have slightly different ideas, and they're working on it at the same time, and they get what are in retrospect not hugely different conclusions, they will still argue. This is a property of academia, probably of aspects of life as well. That people will squabble over what are actually minor issues, in the interest of establishing some kind of supremacy. It's like animals, right?
ZIERLER: [laughs] Henry Kissinger had a famous phrase along those lines.
STEVENSON: [laughs] Yes.
ZIERLER: Dave, you mentioned gravity as it relates to the Earth's core. And again, this is a very abstract question. But given some of the central mysteries surrounding gravity in cosmology and our inability to understand why it's so weak and how we can't connect it with the other forces, is there a planetary science perspective that enhance our broader understanding of gravity?
STEVENSON: I doubt it. Most of what we do in planetary science with respect to gravity is of course Newtonian. There are corrections that we take care of, that are general relativity. There are issues still that I think that may have to do with dark matter. There are some people who would like to have us believe that dark matter, which may be elementary particles for all we know, interact very weakly but could get captured in planets, so that you could imagine the particles, whatever they are, weakly interacting massive particles (WIMPs) getting captured within the core of the Earth, and then annihilating with each other and producing an energy source. People have written things like that. That's not really connecting with the theory of gravity. I'm aware, of course, that there are people who want to think of gravity as an emergent property, as something like entropy. But I'm actually rather pragmatic when it comes to physics.
ZIERLER: When you say emergent property, what does that mean?
STEVENSON: It means that the nature of gravity could emerge from purely geometric considerations. Of course this is a different language from just writing down a Lagrangian or something in basic physics. But I'm just saying that in passing. I think, to finish what I was about to say, that to me gravity is—[laughs] and it's not—it doesn't have to be the role of physics to explain at some more fundamental level what it is. It just is.
From UCLA to Caltech
ZIERLER: Dave, to set the stage for our next talk, which is a great place to pick up, by the time you got the offer from Caltech, were you on the tenure track at UCLA? Were you tenured at that point?
STEVENSON: Yes. They were sufficiently impressed by me that they tenure -tracked me. I'm not clear about the timing.
ZIERLER: But the position initially was not tenure track.
STEVENSON: Correct. They must have persuaded the dean or something. I don't know the details. I do know they wanted to keep me. I had a trajectory that was a little bit like Peter Goldreich, who had also been at UCLA and then was grabbed by Caltech. Some people thought that this was a similar thing a decade later. It was even suggested to me that they might give me tenure, which is different from being on a tenure track. To me, I just wasn't anxious about that. Caltech was not offering tenure. I did not ask for it. I just thought Caltech was a better place. And it's true.
ZIERLER: That's a great place to pick up for next time.
[End of recording]
ZIERLER: This is David Zierler, Director of the Caltech Heritage Project. It is August 16th, 2021. I'm back with Professor David J. Stevenson. Dave, good to see you again.
STEVENSON: Good to see you.
ZIERLER: Dave, before we head to Caltech, I'd like to return to an earlier comment you made about the relative maturity of the field of planetary science at the tail end of your time at UCLA. Just to get a sense administratively, academically, were you taking graduate students? Were there undergraduates who were specifically interested in taking classes in planetary science? What did the field look like, at least microscopically, from your vantage point at UCLA?
STEVENSON: At that point, the number of people interested in pursuing planetary science was quite small. However, I think that's really true of all of science. The scientific enterprise has grown so much, and planetary science more than most. It's certainly true that there may have been more people interested in physics, more people interested in astronomy, but planetary science grew in large part because of the missions, some of which did not come along until later—Voyager in particular, the information coming back in the late 1970s through the 1980s, and then Galileo. And then the modern era, where we have so many missions. I think that has driven the field, together of course with exoplanets. Exoplanets has completely changed the character of the field through the involvement of instrumentation associated with astronomy. And so now you see a very vibrant field, much larger than it was back in the 1970s.
ZIERLER: Was there a graduate program in planetary sciences in UCLA?
STEVENSON: Yes, UCLA was one of the very few that had such a program. The number of students was small.
ZIERLER: Now, what were you doing, and whose attention did you garner at Caltech when you got that offer?
STEVENSON: I don't actually know, and I've never tried to find out what the dynamics were at the time of that appointment. Certainly Caltech from an early time was involved in planetary science. Famously, the division that I'm part of made a decision back in the 1960s to get involved in planetary science. This was controversial at the time, because there was an alternative proposition which was to get involved in oceanography. The decision was made, guided in large part by a very famous scientist, Bob Sharp, who was chairman at the time. The decision was made because of the existence of the Jet Propulsion Lab, which was and in some senses still is, a pioneer in planetary science, in particular unmanned. I don't mean so much sending astronauts to space, although of course the two are connected. So Caltech had to make a decision. Oceanography was already in place at Scripps down near San Diego, and also at Woods Hole and other places. Caltech would have been a newcomer to a field that was already recognized as being important in Earth science. We decided instead to go into planetary science. Among other things, that meant that Frank Press left Caltech and went to MIT. Anyway, we started up Planetary science late in the 1960s. We hired people. I came along somewhat later, about a decade later, but it was already a well-developed activity at Caltech, tied, understandably, to the fact that the Jet Propulsion Lab was a world leader in sending missions to space to do planetary science.
ZIERLER: Dave, what about the dual traditions of excellence in both astronomy and physics at Caltech? To what extent did both of these endeavors drive Caltech's early interest in planetary science?
STEVENSON: As best I understand it, they did not have a big role to play in the decision. The decision was very much centered within what was originally a geological division and then became Geological and Planetary Science, which is our current title. My understanding is that the reason why astronomy and physics played rather little role is because at least in astronomy, a lot of the attention in the 1960s, for example, was outside of our galaxy. It had to do with the universe as a whole. It had to do with quasars, which Caltech led in the understanding of. These were things that were disconnected from questions that have to do with the origin of the solar system, with meteorites, with planets, and so forth, and so we were not getting a great deal of support in our drive towards doing planetary science. It's important to remember that Caltech is small, and we make choices. And I support that. I think that's a good idea. You make choices. You don't try to do everything. And so the astronomers had their own agenda of what they thought was most important, and for the most part, it did not include planetary science. I'm exaggerating slightly, because there have always been people at Caltech who have interests that overlap with planetary, and I knew them. I'm talking about people like Neugebauer, no longer alive, who was chair of physics, math, and astronomy at one point, and was a pioneer in infrared astronomy. And infrared astronomy has played an important role in understanding planets, so of course there was some overlap. But for the most part, it was as I said, an activity that began connected to geology as it is now, rather than an activity driven by astronomy.
ZIERLER: Who was it at Caltech that reached out to you?
STEVENSON: So, the person who made the offer to me was Barclay Kamb, and Barclay was a quintessential Caltech person, meaning that he had spent his entire career at Caltech. He came to Caltech as an undergraduate. He aspired to be a theoretical physicist. He was seduced over to geology, and eventually he became chair of our division after Bob Sharp ceased to be chair. Barclay was chair of our division for a long time, about 11 or so years, and he was the one who made the offer. There were other people connected to the division who were evidently enthusiastic, for example Pete Goldreich, who was also connected strongly to astronomy and physics. But it was essentially the Geological and Planetary Science Division that sought me as a faculty member.
ZIERLER: Now, were you happy at UCLA? Did you jump at this offer right away? What were your reactions to this offer?
STEVENSON: I thought UCLA was quite good. I could tell that it had some outstanding people. It still does. The average is not as high. In other words, there's a spread in abilities of the faculty. I could tell also that a lot of the students who went to UCLA had no particular interest in being outstanding in any area of science that I was interested in. When I looked at Caltech, I saw something different. I saw a more universal excellence, and I saw outstanding students, and I liked the smallness of the place. But to come back to your question, I was happy enough at UCLA. I was happy enough on the west side. I did not immediately jump at the appointment. I visited Caltech. I saw for myself how outstanding it was. It was easy to visit, of course. I just hopped in my car and drove across the valley. So I could see that Caltech was the right place for me, but I did not immediately make that decision. I took a couple of months doing so.
ZIERLER: Why ultimately did you decide to accept the offer?
STEVENSON: I decided to accept the offer because I could see that Caltech was the best place in the world to do planetary science.
ZIERLER: Did you physically move your house, or was it convenient enough for you to just stay put?
STEVENSON: I physically moved. I had an apartment on the west side, and then I moved to an apartment and eventually a condominium in Pasadena, or in fact Altadena, which is next door.
ZIERLER: Now is your current appointment, in terms of where you're housed administratively at Caltech, is it the same as when you first joined?
STEVENSON: It is indeed. I think there could have been an option to get involved in other divisions. I chose not to. Some people have felt that that was something that would work for them. I think in the end, I've done the right thing. I have had, at least in recent years, access to outstanding students. I think getting involved with both undergraduates and graduates at Caltech has been one of the pleasures of being at this institution.
ZIERLER: I've heard so much about the culture of interdisciplinary studies at Caltech. Did that strike you at all when you got here, that there would be things that you could discuss with professors who might not necessarily be as centrally focused as the things you were working on?
STEVENSON: Certainly there was that opportunity, and you did not see it so much at UCLA. UCLA is a very large place. And there is something that happens when you're at a very large place. It even happens a little bit at Cornell, although I think Cornell is structured better than UCLA in terms of people talking to each other. But when you're at a large place, there can be a sense of isolation. It's almost paradoxical that you have a big place where you feel alone, and a small place where you feel connected. I do think that for the most part people work hard doing their own thing, and don't actually spend a lot of time talking to other people. But at Caltech, it was possible. I went and still go to physics colloquia and to astronomy talks. Not all of them, but many of them. And I have chance meetings with people, and did have meetings in the past, with people that you might not have encountered if you had been at a really big place. I'm talking about Feynman, who I talked to; Ed Lewis, who was a Nobel laureate in biology, who I used to have lunch with; and so forth. Caltech benefits from being small. There is this opportunity to talk to people across a wide range of fields.
And physical smallness also matters, because it means that when you're walking across campus, you might see someone who is in a different field, but you know who that person is. And that was true with the people I just mentioned, that you could encounter them just when you walked across campus. You could also encounter people who were running the place, like the president at Caltech. The person after whom my chair is named is somebody that I can remember meeting just by chance—Goldberger—just by chance walking across campus in the 1980s, and having a pleasant conversation with him. I think that would be unlikely to happen at a big place.
ZIERLER: Did you take graduate students along with you, or you were looking to build up a group from scratch when you arrived at Caltech?
STEVENSON: I did not take graduate students along with me. I worked a little bit with graduate students at UCLA, but my style of science has never involved working with a lot of students. And frankly the students at Caltech are of higher quality anyway. So I was happy to leave UCLA and come to Caltech and become involved in working with students here.
ZIERLER: And what were your early interactions with JPL? I'm curious, given how important they were to the push in planetary science for Caltech.
STEVENSON: My style of doing science does not actually require very strong interaction with JPL. I am or was in some sense a parasite, meaning that I benefited from the data that were returned from spacecraft, but did not get involved in the acquisition of that data. I did not get involved in the people who were developing the missions, developing the instrumentation and so forth. My approach to science was more, shall we say, ivory tower. So JPL was there. I think we benefited from it in the sense that students saw the value of the place. But in a strange way, it didn't actually have a huge impact on the way I did science.
Voyager and JPL
ZIERLER: In what ways were you following the Voyager spacecraft mission? What was important for you at this point, as you arrived at Caltech in the early years?
STEVENSON: I think Voyager was very important. Certainly I can remember the experience of seeing the news conferences that were held at JPL, where the data from the mission were presented to the general public and discussed. Scientists who I knew were involved in those presentations. And this was the way you learned about the exciting new discoveries. I can remember being here at Caltech in the middle of the night, seeing for the first time images of satellites that we had never looked at before. This came somewhat later, when the Voyager mission got out to Uranus or Neptune and we saw, for the first time, for example, Miranda, which is one of the satellites of Uranus. And that was breathtaking, because you were seeing remarkable pictures of a surface that you had never seen before.
So there was indeed an impact to my science that came about through those missions. It influenced the choices that I made about the subjects that I got interested in. I was still interested in the giant planets but I got interested in Io and Europa and Ganymede and Callisto and Titan and so forth. And those interests were driven in large part by the NASA missions, especially Voyager.
ZIERLER: What was known at this point, and how did your research contribute to these findings, regarding the existence of ice, or even water, outside of Earth?
STEVENSON: That's actually a complicated story. When people first started thinking about icy satellites—large icy satellites like Ganymede, Callisto, Titan—Europa is somewhat special; it's mostly rock, but has ice on top—when people started thinking about that, there was a group that I was not part of involving people such as Stan Peale and Pat Cassen —I knew these people, of course. Also Ray Reynolds. I knew these people, but I was not directly doing research with them. They were wondering whether there was water underneath the ice. And in the 1980s, the picture moved a bit in the recognition that ice could convect, and that as a consequence, the water could go away.
ZIERLER: What does that mean, ice could convect?
STEVENSON: Ice, as a solid, can undergo convection, thermal convection, carrying the heat from inside the body to the surface, without ever melting. So you had the equivalent of glacial flow, and that in fact is what these people were saying. That unlike even earlier ideas, you might actually have solid ice. I got involved in this subject at a time when it still seemed possible. Subsequently, towards the late 1980s, it became apparent that ice might not be quite efficient enough, and so you could have water, and that is the current situation. In the case of Europa, we have a special circumstance where it seems likely, perhaps helped by tidal heating, that you have an ocean underneath the ice. And that was already the view at that time. I had a student who worked on this issue, constructing models of what the ice might be like on Europa, how thick it might be, what the temperature variation might be, and so forth.
ZIERLER: Despite those particular characteristics, just to be clear, when we're talking about ice and water on places like Europa, is this H2O? Is this something that we can cool our cocktails with, if we had the ability to do so?
STEVENSON: Yes, it is indeed H2O. When you get further out in the solar system, there are more exotic ices, if you will, other materials. I was a big fan—I still am, to some extent—of ammonia. Ammonia is hard to see. It's much less abundant. But it has an important role to play, perhaps, in ice volcanism. Because ammonia, when you mix it with water—that's basically cleaning fluid—has a lower freezing point than pure water ice, much lower. And so you can have liquid in a body at a temperature well below the freezing point of pure water. So other constituents matter. Methane matters. Methane is not as abundant as water, but more abundant than ammonia. In the outer reaches of our solar system, you also have nitrogen ice, carbon monoxide ice, perhaps other things.
ZIERLER: What was some of the initial excitement about discovering ice and water beyond Earth? Did it center around the possibility that non-Earth planets or satellites could support life, or was there something more fundamental at play?
STEVENSON: I think at that point in the 1980s or even more recently, people were not talking that much about life, or about the role of an ocean in supporting life. My sense is that that's a more recent idea. It is of course true that people knew even earlier that when you had liquid water, you had a host for a variety of chemicals that could dissolve in the water. You had an energy source. And so you might have the seeds of life, because what you need is an energy source, and a certain level of complexity, and that could indeed come with a water ocean. There were certainly ideas already in the 1980s that centered around Europa. That was an attractive possibility. But the enthusiasm about life in oceans outside of Earth seems to me to be something that happened a bit later and wasn't heavily pushed in the 1980s. I'm not sure, to be honest, that this is something that is so new. I think that this was always there. Planetary science is after all in part a political enterprise, and so you seek support from the general public, and the general public gets excited about life elsewhere, so naturally enough this becomes part of the rhetoric that people put forward in support of the science that we do.
ZIERLER: Dave, tell me about your proposal, along with others, for the existence of an ethane ocean on Titan. What were some of the advances in instrumentation that made that proposal feasible, and how well has that aged over the years, that initial idea?
STEVENSON: That was actually an interesting story in that it shows how a graduate student can play an important role in creating a bridge that might otherwise not happen between faculty. The student in question was Jonathan Lunine, who is now a professor at Cornell in the chair that Carl Sagan once occupied. Jonathan and I were working on other things. He was really the first student I had at Caltech. He commented to me one day that another professor who was literally yards away, Yuk Yung, had worked on the fact that through photochemical processes, methane, which we knew to be abundant, was converted to ethane and other things, on bodies such as Titan. I did not know much about photochemistry, but I got intrigued at that point. There are other things, we now know, that get produced as well as ethane. But ethane, which is C2H6, is a liquid under the thermodynamic conditions on Titan, and so I could see immediately that there might be a conversion from methane, which is known to be present in the atmosphere of Titan based on work by Gerard Kuiper way back, decades earlier. So we knew it was there, was being converted to ethane, and it should rain out on the surface. And so over geologic time, there should be a conversion, and the conversion should be the equivalent of a kilometer thickness of liquid. It is in the liquid state under the relevant conditions. And so then you might have the global ocean.
I can remember even going with Jonathan across campus to the Chemical Engineering Library at Caltech—in those days, there were branch libraries that were useful—and looking up the phase diagram for ethane and methane, perhaps with only limited success. But starting to get an idea of how things work. To be sure, there were people elsewhere, outside of Caltech, who had also been thinking about the photochemistry. But we did come up with this idea that there might be oceans on Titan. It's not right. Although interestingly, it still affects the way people think about the surface of Titan, because of the puzzle of where is the ethane. So the story is probably more complicated; that's often true in science. But it was an interesting illustration of how information that became available through spacecraft missions, but also through modeling, drove a development in our understanding.
I'll be honest with you; I don't actually remember anymore the extent to which there was a connection between that effort and the new data coming back from Voyager. There was certainly some information, and it was indirect, because what Voyager did for us was actually find the surface of Titan. We didn't have that before. So I think that's the answer to the question of where's the connection to the spacecraft mission. I think it is in the recognition that through radar, we actually found the surface, and so we could characterize the surface conditions. And so that was very important. But a lot of the ideas about conversion of methane and so forth had already been circulating among scientists.
By the way, it happens in Earth's atmosphere as well. If you read about global change on Earth right now, what you will read is that people are concerned about methane, because it is released from natural gas, so biological origin, of course, unlike Titan, probably. And then I saw it in The New York Times just last week—somebody said, correctly, "Well, methane doesn't stay around." And they're right. There's actually a residence time, because methane is continuously broken down by UV photons from the sun. Carbon dioxide, on the other hand, which is the main concern, continues to accumulate, modulated only by the ability of that gas to be ingested by the Earth's oceans. So you can see ideas that come up in the understanding of planets also affecting our thinking about the Earth.
ZIERLER: Dave, a broad question at this point about the powers of extrapolation—once you start to think about and have the ability to verify or not the existence of ice and water beyond Earth, how easy or not is that a leap to ice and water on exoplanets, or just the very idea that there might be exoplanets out there that might not be very different from the ones in our own solar system?
STEVENSON: Certainly the idea that liquid water might be common in our solar system, common meaning that we would find it beneath the very cold icy shell of bodies, that does stem from a theoretical understanding of the ability of ice to carry heat, along with the recognition that there are constituents that function as antifreeze. I've mentioned that already in connection with ammonia. And that, by the way, is why I advocated 20 years ago, maybe even more, that there should be oceans in Pluto, oceans in Triton, possibly oceans in small satellites and so forth.
When it comes to exoplanets, in some ways the issue is a little different, maybe, because so far, a lot of these bodies that we've found are actually fairly hot, and we don't know much about how much water they have. Water is certainly the most—or should be—the most abundant condensable material in the universe, simply because oxygen is the third most abundant element, and in the presence of a lot of hydrogen, you should have water. That is expressed in our solar system; it's actually not so clear for the rest of the universe. But to get to your question, from a theoretical standpoint, it would seem reasonable to suppose that water is very common, that water in its liquid form is also quite common. A lot of it may be dissolved in hot rock, and so it may not be the everyday idea of liquid water that we have on Earth, that we can drink. I am not so sure, actually, that so many of the exoplanets are like Earth. But, the number of exoplanets is so enormously large that of course only a small fraction of them need to be like Earth in order to have a lot of Earth-like planets.
ZIERLER: Dave, at this point, Caltech, mid 1980s, where is geophysics in your overall research agenda, and does it change as a result of your coming to Caltech?
STEVENSON: I've always been interested in geophysics. I've always been interested, in particular, in how Earth has a magnetic field, in how Earth gets rid of heat, which has to do with convection. So I had a natural interest in Earth's core. I was a recognized participant in discussions, papers about how Earth's core worked. So that was beginning already at UCLA; it continued at Caltech. I did see planetary science as an opportunity to skim the cream. Planetary science is—certainly was, maybe even still is—less mature as a field. And that gives you greater opportunity for the kind of science I do. It means that you can do what I call skimming of the cream, identifying things that people haven't done yet, getting in first simply because it's not as well developed as a field. My way of doing science fits less well with the very mature development of, for example, the structure of the Earth, the composition of the lower mantle, and so forth. So I find these things interesting, but I fit less readily into the community that do these kinds of things simply because they are getting into the detail, and I'm less interested in detail.
The Origins of the Moon
ZIERLER: How did you get involved in questions about the origins of the Moon, and what orthodoxies about its origins may have needed to be revisited at that point?
STEVENSON: So, I cannot claim to be a leader in working on the origin of the Moon, even though I helped run a conference and played a significant role in conferences from the beginning of discussion of this subject. By coincidence, by chance, I was present as a student at the meeting in 1974 where the currently favored idea was first discussed, as best I can tell. This was a meeting at Cornell. That's why I was there. It was a meeting on planetary satellites, which was not my interest at the time, but because it was at Cornell, I attended. And the idea was put forward by Bill Hartmann in a conceptual phenomenological way of possibly having large impacts on the Earth as it formed. It was developed much more quantitatively by Al Cameron, who was already involved in the subject and was at that meeting. Al Cameron became a professor at Harvard who I knew quite well. And also Bill Ward, who was actually a Caltech student at that point, working with Peter Goldreich. And indeed, Cameron and Ward put forward the ideas that quantified the giant impact of the Moon in the same era. That is, around about 1975, 1976. So I already knew about the subject at that point but was not working on it.
The really big thing was 1984, and it was a meeting I attended. It was in Kona, Hawaii, and it was the closest you come in science to a revolutionary meeting, because you saw a major shift in the way people were thinking about the subject. I had already been thinking about it, working with a Caltech undergraduate called Chris Thompson, who is now a professor. Chris and I had worked on ideas of the giant impact and what happened when material was put in orbit and coalesced to make the Moon. And so I turned up at this meeting in Kona, Hawaii, unaware that other people had reached a similar conclusion. And so you had this interesting phenomena that sometimes happens in science, where people come forward with a common idea that changes the perspective of the field.
It was resisted by some people. Rightly so, because there are issues that are still unresolved about the chemistry. And so it was resisted by some chemists in particular. I think they were right to resist it. I remember Ted Ringwood being concerned about it, Mike Drake being concerned about it. These people are no longer alive, but they were prominent geochemists of that era. But, Al Cameron came forward with the initial attempts to do simulation in the formation of the Moon by giant impact. I presented some work on analytical approaches. Other people were commenting on this. So out of that came an effort that changed the perspective of the field. Somebody who was too young at that point to directly contribute but later became a central figure was Robin Canup, who actually was a visiting professor at Caltech at one point, but who much later and at present is a distinguished scientist working at Southwest Research Institute in Boulder, Colorado. So Robin was very important to the story. There were other people who were important later on.
The story is not fully resolved, but the idea of a giant impact is persisting, and I think it is correct. And it is not just important for understanding the formation of Earth's Moon. It is an aspect of the way we think about the formation of planets that pervades all of our ideas of planetary formation. It is also an exasperating subject, because it becomes a sort of universal panacea, something that you invoke when you don't understand what's going on. [laughs] And so you can always say, "Well, that was a giant impact, and it did this and that." And who can tell? So it's not an entirely satisfactory theory, but it's an important idea.
ZIERLER: What are competing theories, if not the giant impact?
STEVENSON: So the competing theories largely subsided at that 1984 meeting. They were present in a prominent way at earlier times. One of the ideas was capture. Capture was never that popular actually, but it sounds like a good idea. It sounds like a sensible thing to say that the Moon formed somewhere else, and the Earth just grabbed it, and it ended up in Earth orbit. That is possible in principle, but it fails to explain what we see, in many different ways. From a purely physical point of view, capture is actually exceedingly difficult, and the reason is elementary physics. If something falls into the Earth's gravity field from great distance, then unless you impede its motion by putting some stuff in its way—friction if you will—unless you impede its motion, it will come in on a hyperbola, and it will leave on a hyperbola. You do not capture using gravity. Gravity is a conservative force. And you might say, "Well, okay, I can put stuff in orbit that will act as friction." But then you get into trouble because in most cases, if you try and do that, you get a disaster. You get too much friction, and it ends up coalescing with the Earth. So it's a neat trick to put something in orbit, and a giant impact succeeds in doing that.
The other idea that was actually fairly popular was co-accretion. Co-accretion just simply meant that as you were aggregating stuff to make the Earth, you were simultaneously aggregating stuff in Earth orbit. This turns out to be difficult to pull off, and the main reason is angular momentum. When you are adding small bits of material together to make a body, it's difficult to create the spin that you see. The spin tends to average out to zero when you have a lot of small bodies coming together, some from the left, some from the right. There's no special sense in the motion of solid bodies. This is not true, by the way, for gas. So it's not true for Jupiter, for example. But it is true for making something like the Earth. So, the advantage of the giant impact, which was really the key idea that Cameron and Ward put forward in 1975 or 1976—the advantage is that a single impact of a Mars-sized body would explain the angular momentum of the Earth-Moon system, which basically meant the initial spin of the Earth. Of course right now, most of that is expressed in the orbital motion of the Moon about the Earth. The Earth is now only rotating every 24 hours, but early on, it was rotating once every five hours. Or, some people have even suggested once every two and a half hours, which is near rotational breakup. You do that through giant impacts.
ZIERLER: Dave, I'm curious if manned missions to the Moon, collecting samples, simply being there, was helpful in a way that studying other satellites would have been, by definition, more provisional, in terms of how they were formed.
STEVENSON: I've never been a big enthusiast for manned exploration. I understand, of course, the tremendous benefit that can come from putting a geologist on a solid surface, collecting rocks, and bringing them back to Earth so that we can study them in the lab. Of course you can bring rocks back without sending a person, and we're doing that for Mars right now. Jack Schmitt, who was the only geologist to ever actually be on the Moon, is somebody that I met. He came to Caltech. He was known here. He was a student here [laughs], in geology. Although that was before my time, but I met him later. So the Apollo program certainly had some impact on the data that we have for understanding the Moon.
But interestingly, a lot of the ideas that have emerged about the way the Moon formed could have developed without having the Apollo program. You certainly needed to know about the fact that high temperatures were involved. That was evident from the anorthostic crust. You needed to know some of the other things that astronauts found. But these could have been obtained through unmanned missions. So I don't think that manned missions are particularly important. They are very expensive. It is very expensive to send people into space.
I'm expressing a view that is not special to me. It is common within the planetary science community for people to say that unmanned missions are the way to go for exploring the solar system. The idea of sending people, whether it be to the Moon or Mars or whatever, is a romantic notion. That doesn't mean it's bad, but it has to have some other justification. You are not, in my opinion, going to justify sending people to space because of the science that they can do.
ZIERLER: Dave, of course another major event in 1984 was you winning the Harold C. Urey Prize, and I believe, if my notes are correct, being the inaugural holder of that prize. What did that feel like?
STEVENSON: Well, it was nice to be recognized that way. Of course since it was a new award, it was hard to know the significance of it. It has of course become the planetary science equivalent of the junior scientist award that you see in other societies. So yes, it was a pleasure to be involved in that. Maybe I did not think of it as hugely significant at the time simply because it was completely new.
ZIERLER: Now, being that it's within the Division for Planetary Sciences of the American Astronomical Society, is the AAS your home society, so to speak? Or are there other planetary science societies out there?
STEVENSON: When I was a student at Cornell, the time came to figure out what society to belong to, and I actually applied to become a full member of the American Astronomical Society in the mid 1970s. At that point, the Division for Planetary Science was very small indeed, as indeed it still was into the 1980s. I remember getting a letter back saying, "Do you really want to be a member of the American Astronomical Society?" [laughs] Because it costs more money, and I guess they were unsure about why I was doing this. I did it, so I am in fact a full member of the American Astronomical Society, and also became an affiliate member for the DPS. In the late 1970s, I attended meetings of the Division of Planetary Science, and in the 1980s as well. In the late 1970s, everybody easily fitted into one room. So it was a small activity. [laughs] So that's the one that made sense.
But actually, I have always had a strong connection—you might even say a stronger connection—to the American Geophysical Union, which of course is much bigger. The American Geophysical Union has a section on planetary science. I was even the president of that section at one point. And planetary science has become an increasingly larger part of the American Geophysical Union. AGU, as we call it, is an enormous organization. I've lost track of how many members it has. Absolutely huge. It is very inclusive, and so it has opened itself out to all manner of related areas including things that might most naturally fit with the Geophysical Society of America, including things that are done by people in Europe and in Australia and so forth, so it has become truly international. It has been very successful. It has very well-run meetings. It runs publications and so forth. So AGU is very big, and I was involved in that from the early days as well as DPS. I have not attended the regular American Astronomical Society meetings except the very first one that I went to when I was a student at Cornell, which would have been in Rochester about 1973 or 1974.
ZIERLER: This dual membership, I wonder the extent to which it's a microcosm for something broader about planetary science, and that is, it's not a field that should have its own scientific society because it's so multidisciplinary, if you will.
STEVENSON: Well, I think historically, at least the Division of Planetary Science has, as its core, people who are interested in the parts of planetary science that fit naturally with astronomy. Of course it has spread out more in subsequent years, but it's still true that a lot of it has to do with atmospheres, and with those parts of planetary science that fit naturally with astronomy. There are other kinds of planetary science; you're right. I once had to write for an encyclopedia an explanation of what planetary science is, and I began by saying that planetary science is an in-discipline, meaning that it's not a discipline. Or an un-discipline, meaning that it's just the combination of everything that everybody does that normally gets labeled as geology or geochemistry or geophysics or geobiology or whatever, but applied to planets. And that's still true.
So yes, it's true that there are other avenues that are available to people who do planetary science. There's one other very important one that I haven't mentioned, which I was also involved in, less so now, and that's the Lunar and Planetary Science Conferences, which initially were inaugurated for the people who studied the return from Apollo. These began as meetings in Houston. When I first got involved in it, it was still held at the Johnson Space Center, south of Houston. I attended those in the late 1970s, and I attended quite a few more in the 1980s as the meeting evolved. It is still held every year in Houston, different location, but very much tied historically to this notion of understanding planets from the point of view of the materials. Because after all, that's what you do, when you look at Apollo rocks. Like everything else, it has spread out. It has become broader. It covers a wider range of things. But it's still true that the core of LPSC, as we call it, is the people who study the materials, such as meteorites.
ZIERLER: Can you talk a little bit about the early high impact flux on Earth? What is that, and what were your findings regarding the way that it may have delayed the origin of life on Earth?
STEVENSON: So, back in the 1970s and 1980s and maybe even more recently, there have been many speculations about the delivery of material to the Earth. I think it's fair to say that it is still an unresolved issue. Certainly in respect of starting life on Earth, I posed that issue as a project in the class that I taught at Caltech. I am fond of creating term projects for students, and some of these term projects end up as published papers. And so it occurred to me that impacts on Earth were known from round about 1980 to have a potentially important consequence for the development of life—I'm referring, of course, to the famous impact, implications of which I was not involved in but observed, that got rid of the dinosaurs, the demise of the dinosaurs 65 million years ago. Still somewhat controversial, but we know that there was an impact. We see the crater in Chicxulub in Mexico. So there's no question that there was an impact at around about that time and it had a major consequence for the Earth. It may have acidified the ocean. It changed the atmosphere. It caused global fires. So we knew a whole bunch of things. These were things that we learned in the early to mid 1980s.
So given that context, it was natural to wonder, well, if the development of life on Earth can be so much affected by impact, why not consider the possibility that the origin of life on Earth was delayed or greatly affected by the greater flux of impacts early in its history? And so that led to the idea that you could have had a scenario where life began on Earth, and then so to speak got hit on the head. You could then imagine life starting over several times before you finally reached a point where the flux of impacts on the Earth had diminished sufficiently to allow life to persist.
Why was this an attractive idea? Well, it was in large part the recognition that impact flux had certainly been larger at earlier epochs. How do we know this? We actually knew this from looking at the Moon. And this was the work of Gene Shoemaker. Gene Shoemaker, by the way, was division chair at one point at Caltech. He is somebody that I co-taught a class with. So he was associated with Caltech when I came to Caltech, for a while, although he was also at Flagstaff. He was the world's leader in understanding impact flux on the Earth. And he said that throughout most of Earth history, the flux has been low and rather constant. It's not clear whether that's true, actually, but that's what he was saying. And then at an earlier epoch, marked in substantial part by what is called the late heavy bombardment on the Moon, which was 3.8 billion years ago or earlier—in that earlier epoch, extending back to the formation of the Earth 4.5 billion years ago, there was a higher flux. You could naively imagine some exponential tail of formation of the Earth extending from 4.5 billion years ago to 3.8. That was the basis for the idea that the Earth was hit by things, some of which were quite big and could destroy life so that it had to start over. So that was sort of attractive.
I also knew actually from my time back at UCLA that some people thought that life was easy. What they meant by that is that the beginnings of life were accomplished on a rather short time scale, geologically speaking. We don't know this, by the way. I had a conversation with Stanley Miller, of the famous Miller-Urey experiment, at University of Chicago. He was part of a group that I got involved with at UCLA, and we met in a restaurant in Westwood, Stanley Miller and I, and some others. And I asked Stanley, "How long did it take life to begin on Earth?" And he said, "Oh, a million years." Of course, he didn't know. Nobody knows. [laughs] And so this was sort of a joke, but it wasn't entirely a joke. Because what he was saying, in effect, was that it couldn't be really long, because the evidence that existed for life on Earth already suggested that life was complicated 3.6 billion years ago. I'm talking about what we now know in the geological record to be stromatolites. These are algal mats from Western Australia, for example.
So we knew that life could be quite complicated at 3.6. And we also knew from people like Gerry Joyce, who I also know, down near San Diego, that if you tried to manufacture life in the lab, it's not so easy. You don't get things crawling out of the test tube. [laughs] So it can't be really, really short, but it can't be really, really long either, and a million years fits in between. But given numbers like that—you know, ten to the power of six years is tiny, compared to the age of the Earth. It's even tiny compared to one billion years. So why not imagine a story where there was some delay in developing life on Earth, but once it developed, things could happen quickly? I have to say, having talked to people who study the subject of the origin of life, that it's not at all clear that these stories are the right way to think about it.
I have to also say, in respect of that particular work, that by coincidence, we were thinking about this problem at the same time as people in the Bay Area. I'm talking about Norm Sleep, who was at Stanford. Kevin Zahnle. I know these people. Kevin Zahnle at NASA Ames. They actually wrote a better paper on the subject. Both of us ended up being published in Nature. Norm Sleep even suggested to me that we get together and do it jointly. At the time he made that suggestion, we were far enough along in what we were doing at Caltech that we went ahead with what we had. But frankly, they did a better job on the problem. I think it's a fascinating idea—impact frustration of the origin of life. I don't know whether it's right.
ZIERLER: Dave, what about approaching the issue from the diametrically opposed position? As we said in an earlier conversation, what about looking at these high-impact events as a source of life? Either with the delivery of microbes or bacteria, or alternatively, what about the possibility that these high-impact events changed the chemical composition of the oceans, for example, that would have made them hospitable for life in a way that they might not otherwise have been?
STEVENSON: Well, we're in the realm of speculation where you can say almost anything, and there's no obvious contradiction. Sure, it's possible. I do, however, know enough about impact physics to be skeptical about the notion that impacts are a way of starting life, at least in respect of big impacts. Because when a body comes in, as it must, at at least 11 kilometers per second, because it's falling into Earth's gravity field, it's horrendous. You get vaporization. You get high temperatures. You get a shock event that is spectacular. Who knows what the consequences are far away? Maybe you could even conjure up some story where they're good. But on the face of it, it's not a very attractive idea.
Meteorites, different story. Because meteorites slow down high up in the atmosphere. They hit the Earth's surface essentially at a velocity that is set by air drag. And so they're not moving particularly fast. They make a small hole in the ground. They're hot enough that you wouldn't want to pick them up immediately, but they cool off quickly, and the center part of a meteorite actually stays cold, even after passing through the atmosphere. So there's no doubt in principle that you could deliver stuff from elsewhere that's survivable and is suitable.
I look at the problem and I say to myself, "Well, the early Earth is complicated. There are many different environments. There's the whole periodic table to play with. Why invoke things from outside? I don't see a good argument for doing so." But of course, like everybody else, I'm ignorant.
ZIERLER: Dave, tell me about your work with Huw Davies on subduction zone volcanism. How did that get started?
STEVENSON: That work is a curiosity in the sense that it is quite well cited but is not central to my research effort. The way that began was actually with another person who was a very good student, David Scott, and it concerned melt migration. I got interested in the problem of what happens when you take a rock and start to melt it. The melt, being typically less dense—not always, but usually less dense than the rock from which it's formed—tends to migrate upwards, tends to percolate, just in the same way as water percolates through coffee grinds. And I got interested in the theory of that.
As it so happens, contemporaneously with that effort, Dan McKenzie at Cambridge in England was doing something much more complete. But we did end up doing some significant work on melt migration, as applied to mid-ocean ridges. Huw Davies was a very good student in geophysics, another one of my geophysics students. David Scott was a geophysics student. And Huw picked up on the other obvious possible application of melt migration, which is subduction zones. So that work was very much driven by him. It was his choice. It was not something that I gave to him. It was something that he realized would be a natural direction to take that kind of work. And so he developed some very nice ideas about how melt migrates above a subducting slab. This, of course, is the origin of arc volcanism, the kind of volcanism we see with the Cascades in northwestern U.S., volcanism in the Aleutian Islands, volcanism in Japan. New Zealand, for that matter. Many places. South America.
So this is a common thing associated with places where material is going back down into the Earth, material that might include some water. And so part of the story had to do with the role of water. It's an interesting story. I don't think we really understand yet fully what's going on. But it is a good example of an interdisciplinary subject where you're trying to combine geophysical ideas that come from fluid dynamics with geochemical ideas that come from looking at the composition of the rocks that make up the volcanoes in island arcs.
Feynman and the Challenger Disaster
ZIERLER: Dave, a question that's not germane to your immediate field of interest, but just by dint of what you were studying and where you were, what was the day like for you when the Challenger exploded in January 1986? Do you have clear recollection of that? And more broadly, how did Caltech and the associated research communities respond to this tragedy?
STEVENSON: I remember it well, for several reasons actually. On the night before the Challenger disaster, I had an extended conversation with Feynman about Earth's gravity field. And that's not directly connected, except of course that it was the last time that I had a discussion with Feynman, because he got involved in trying to understand what happened after the Challenger disaster. So in that sense, it had a major consequence for some of the things I was thinking about.
ZIERLER: What was the nature of the discussion with Feynman? What were you talking about?
STEVENSON: [laughs] That was an interesting story. It was the only occasion I can recall where Feynman contacted me with no forewarning. What I mean by that is that there were a small number of other occasions where it was natural to talk to Feynman, because we both got interested in something at a seminar, or I happened to see him at a freshman camp on Catalina Island or whatever. But on this occasion, I was sitting in my office, and I got a phone call, and it was from Feynman, and he wanted to know how well we understood Earth's gravity field. The reason he was asking is that he got interested in what was then called the fifth force. The fifth force was the term that was used for a modification of Newtonian gravity—this had nothing to do with general relativity; it was just a purely classical—essentially classical notion about an additional contribution to gravity that came from particles of finite mass. Whereas gravity as we understand it is mediated, we think, by things that are massless, and so the signal goes at the speed of light and so on and so forth.
Now, if you have something that's massive, then the potential becomes Yukawa-like, and so it has a finite range. And at that time, there were people advocating this based on geophysical measurements. There were people who thought they saw evidence for this, by measuring Earth's gravity field as you went up some high tower above the Earth's surface. People who thought that gravity changed when you went down in a submarine, in a way that was not explained by conventional Newtonian gravity. And so Feynman wanted to know how well we actually knew gravity. I'm not [laughs] an expert in the subject, but he just reached out to the person he knew.
And so I remember going away to the library and actually reading up on satellite measurements and telling Feynman the accuracy with which these were made. He made a suggestion to me, which was never followed up. And his suggestion to me was to use a low-flying airplane in someplace such as upstate New York [laughs]—he actually said that—where you could basically map out Earth's gravitational potential, in effect by doing a lot of measurements in a cube. A fictional cube. Not an actual cube, but just a set of points in Cartesian space. And then that way, you could test the basic ideas of Newtonian gravity.
So that was his suggestion. It's an interesting one. Nowadays, I think we could do better. I'm not sure that anyone has really followed up on this, the main reason being that the fifth force went away. People realized that the claims for the fifth force were based on things that they didn't fully understand, like the fact that salinity in the Earth's oceans actually varies, and that changes the gravitational signal that comes from water, which was previously assumed to be constant density. And so these were small effects, but understandably of interest if you thought that there was some deviation.
By the way, if gravity were more complicated than inverse square, that would be profoundly important for the way you thought about planets, the way you thought about satellite orbits and so forth. You have to remember that when people quote the mass of the Earth, or the mass of any celestial object, they're actually assuming that we know the gravitational force, and specifically that we know big G. Big G, Newton's gravitational constant, is measured in the lab. So you can measure Earth's gravity to one part in ten to the 12, but that doesn't tell you the mass of the Earth. You still have to use big G. You still have to know what the force is. So it's actually of some importance to establish whether Newtonian gravity is correct.
This idea was still around in later years, because there were people who I knew at JPL, for example, who wanted to say that spacecraft that were leaving the solar system, the Pioneers and Voyagers leaving the solar system, were exhibiting a change in their velocity, acceleration, that was inconsistent with Newtonian gravity. It turns out there are other non-gravitational effects. But that explains, perhaps, why this is an interesting question.
ZIERLER: Just to be clear, the conversation with Feynman, did it concern the Challenger itself? Was that part of the discussion?
STEVENSON: No, no. So, I'm sorry—it was a slight digression, but I only made it to explain something that happened to me, which is that I never talked to Feynman again. [laughs] But as for the Challenger disaster itself, I saw it, in real time, on television, in the building, the very place I'm sitting now, and I saw it with Jonathan Lunine, who at that point was a former student of mine who happened to be visiting because he was part of a committee that met at the board room in Millikan Library that morning. And so we were sitting there together, and watching this thing take off.
I wasn't a big fan of the shuttle. He was. And he knew that something bad had happened. I was just looking at it and not knowing what was going on, because I didn't know what to expect. But he knew immediately that something bad had happened. So that had an effect. Of course it didn't directly affect my science. Well, indirectly it did, because of course it led to a change in the timing of the Galileo mission, which had previously been scheduled for launch soon thereafter, and they had to delay that, and do it in a different way. And so we didn't get the scientific return of the Galileo mission until almost a decade later.
ZIERLER: To clarify, was the Galileo delayed because there were similar safety concerns, or just because everything was haywire at this point?
STEVENSON: It was delayed because you could no longer use the shuttle to do the launch. So the intent was to use the shuttle as a means of getting to Earth orbit, and then the Galileo spacecraft would go beyond that. They instead had to launch it from the ground and much later. And so there was a delay. Of course the shuttle eventually got back into business, but there must have been safety concerns as well. I don't remember the details anymore. It just meant that Galileo was launched much later.
ZIERLER: What was compelling to you as Galileo got off the ground? What were you interested in its findings?
STEVENSON: My approach to science at that time was not heavily tied to what we were going to learn in future missions, but it's certainly true that when the information came back from Galileo, it had a big consequence for the science I was doing. Perhaps the most important was the confirmation—we already thought we knew this, but it was confirmation that helium was depleted in the atmosphere of Jupiter. So Galileo had a probe, the probe dropped into the atmosphere. That was early in the mission. And everybody understood that it was important to measure the helium abundance to great accuracy, so it was done by two different methods. The methods agreed, and it showed that there was a 10% depletion of helium. It also measured other constituents that showed that heavy noble gases were enriched. It showed that neon, which is a light noble gas, was strongly depleted. So the behavior of helium and neon agreed with my theoretical ideas. It was confirmation of what I had been saying back in the 1970s. So that was one thing.
The other thing was a completely different story and had to do with the magnetic field measurements of the satellites. The Galileo spacecraft, after dropping off the probe into the atmosphere, wandered around the satellite system, giving us spectacular pictures of the surfaces, some of which we already knew about in less detail from the Voyager mission. But in particular, it got close enough to the satellites—the icy satellites, and to Europa—to measure magnetic field. And it found near Europa that the field was greatly changed by Europa. Europa is in the magnetosphere of Jupiter, and I set about, actually with a Physics 11 student, to try to understand those results. There were people at UCLA responsible for that part of the mission who had also worked on this, and as best I could figure it, had dropped it. They had not been able to understand the results. But I started working on this—the Physics 11 student did not actually succeed in figuring it out, but I figured it out after he finished his summer's work, and realized rather quickly that the magnetic field near Europa could be explained by electromagnetic induction, essentially by eddy currents.
Europa, like the other satellites, experiences the time-variable part of Jupiter's magnetic field arising from the dipole tilt, and that time variable part, according to Faraday, must induce an electromotive force inside any conductor. This is a well-known physics effect. It was studied way back in the late 1800s. Of course Faraday was even earlier. And so I realized that Europa was behaving essentially as a diamagnet, meaning that it excludes the field perpendicular to its surface, which is exactly what you expect from conductors. So the question is, what is the electrical conductor inside Europa? And you don't need a very good conductor, it turns out. You just need an electromagnetic skin effect, and you can do it with salty water, and salty water is the natural thing to think of. In principle, you could do it with graphite, but it doesn't make sense to have a continuous sheet of graphite. There's no chemical reason to have such a layer. So the natural conclusion is that Europa had a salty ocean. And that's what I decided.
I actually gave a seminar on this, in planetary science, at Caltech. It's funny in retrospect to think that when I did this, I never thought of this as being particularly remarkable or especially interesting or anything, because the idea of an ocean was not entirely new. But I should have realized that having an observational constraint like that was important. Anyway, I wrote it up, I sent it off to the people at UCLA, and forgot about it, until they contacted me months later and saying, "Would you like to be co-author of a paper on this?" So, ended up in Nature. So it's slightly embarrassing to think back at an example of something I did where I didn't realize how important it was. Because nowadays, of course, the idea of an ocean on Europa plays a major role in our thinking about oceans in general, and the motivation for the Europa Clipper and so on, and so forth. I still think that we have the best evidence for an ocean on Europa. People look at the surface and say, "Well, look at all that broken-up ice, chaotic terrain. Doesn't that mean you've got an ice shell overlying an ocean?" Well, it's consistent, but geology is a tricky way of deciding whether you have an ocean. Electromagnetic induction is the best way to do it.
Of course the big surprise, which I didn't realize—this is also true for Callisto—so there's a certain irony in that. Europa is special. The ice shell is thin. It's a more attractive environment for thinking about life elsewhere in the universe, perhaps. Certainly, in our solar system. I mean, Mars is the natural one to think about, but Mars is boring. It's like the Earth. Europa is different. So it's an interesting idea. But Callisto has an ocean as well. I look back at it now and I say, "Well, yes, of course." I sort of even knew that that's a possibility. But your views of science change over time, and you come to realize how things fit together in a way that you might not have realized a quarter century ago. This is 25 years ago.
ZIERLER: [laughs] Dave, an overall question bridging chronologically the 1980s to the 1990s is your sense of the budgetary environment for planetary science. On the government side, for example, did you feel the impact of Reagan's increased military spending? Did that spill over into planetary science? And consequently, at the end of the Cold War, was there a pullback in budget? On the private side, today we know of course about people like Jeff Bezos and Elon Musk and their impact on planetary science. Was there such a thing as the billionaire or hundred-millionaire private benefactor who could bestow gifts that really were capable of changing the field, in the 1980s and 1990s?
STEVENSON: Planetary science underwent major changes through those decades, because of the funding, which at early times was almost entirely the government—NASA or agencies of other countries. So the Apollo era, of course, as a political imperative, there was a huge amount of money spent. And of course, most of that went into building the spacecraft and so forth and actually getting to the Moon, but some of it also went to scientists, and so you had people studying the rocks and so forth.
Then there was a dramatic drop-off in support for that kind of science, including what we would now call physics, through the late 1970s and into the 1980s. The decline of the budget led to the stretching out of the timetable for sending missions and so forth. I think it's fair to say that in the 1980s through the 1990s, there was this general sense of diminished activity in planetary science. It showed up in the number of students interested in pursuing the field. It showed up in the pace at which new results were made available. The Voyager missions were spectacular. Galileo did well. We did Magellan to Venus, which I was not involved in. So it's not as if there was nothing, but there was less per unit time than there is now.
And there were no sugar daddies. There were no rich billionaires who had money to throw away, as we have now. Stunningly wealthy people like Bezos, or even for that matter somebody like Elon Musk—it just didn't exist. By the way, I think those people are remarkable. I think they did something special. They added value to the world economy. So I don't want to take anything away from them. But [such people] did not exist [back then].
So, what happened? Probably the most important thing that happened in the history of the subject was something that we now recognize as a mistake, or a misdirection. And that was Allan Hills 84001, which revived exploration specifically for Mars. Allan Hills 84001 was a meteorite from Mars that was picked up in Antarctica. That's why it's called Allan Hills. And some people claimed in a prominent paper that it showed evidence for life. It doesn't. Although some people may still disagree. But it certainly revitalized the science. It got people very interested in life on Mars. There were other things happening. But in general, as you went into the first decade of this century, there was an improvement in the budget, and that has more or less continued. It's not tied to whether you have Republicans or Democrats in control. I'm not even sure I'm in a position to explain exactly why this was happening, except to say that I do know from personal experience that there are some people in Congress who love the idea of life in space. So that's certainly part of it.
I was part of a group, a decade ago, that mapped out the future of planetary science. This was called a decadal survey, and it's done every decade. There's one taking place right now, which I declined to be involved in. That survey, like previous ones, was begun by the astronomers, but that survey in planetary science attempted, for the first time, a decade or so ago, to construct a budget consistent with what we thought would be the amount of money available. We had what's called a budget wedge, and we had to try and fit missions into it, and it led to some important decisions like downgrading the mission to Europa so that it would cost less, downgrading sample return from Mars so that it would cost less. These things are now becoming more expensive.
But other things are happening, and there are cheaper missions happening, and the budget has grown. As best I can figure it, the growth, which is real dollars—it wasn't a lot of inflation in the last decade; maybe there will be now. But in the last decade, inflation was modest. The budget in real dollars has grown. Science has become more expensive, but we're doing a whole bunch of things. There's a vibrant community. And I'm not sure I'm a big fan of why it is happening, but as best I can figure it, it is this enthusiasm for life.
ZIERLER: Dave, the last subject I'd like to touch on for today, and this is to foreshadow to your tenure as division chair, is if you can explain administratively the overall structure at Caltech and some of the idiosyncrasies at the Institute. So for example, what is the difference between a department chair and a division chair, and what might we call deans, even though we don't use that word in Caltech, in understanding relatively how much power is contained in these administrative responsibilities?
STEVENSON: Caltech is small. It does not try to do everything. It has things that we call divisions. At other institutions, you might call them departments. But perhaps an important distinction to make is that what we call a division is a combination in many instances of things that elsewhere would be separate departments. The best example of that would be Physics, Mathematics, and Astronomy, which we call PMA. At most universities that aspire to excellence in those fields, those would be separate departments. Completely separate, subject to some dean. At Caltech, we have maintained a leaner structure. We have six divisions. We have no desire for more than that. I happen to be in a division—Geological and Planetary Science—that is not that different, actually, from a comparison department at MIT, which is called Earth, Atmosphere, and Planetary Science, EAPS. I like to call it the Department of Everything.
ZIERLER: [laughs]
STEVENSON: And so the structure of the part I'm in is not hugely different from other places. Although, to be sure, there are places that have a different structure, and Cornell is one of them, because Cornell has put planetary science in with astronomy. We have the astronomers separated out into a different division. They happen to be across the road. And of course there's a lot of interaction, and that's one of the good things about Caltech.
Main point about Caltech—the administration is lean. There are division chairs with substantial budgets, and a great deal of power that comes with those budgets. There are no academic deans at all. This makes us different from every other place I know about. I think this is a strength. It means that at Caltech, the decisions about who to hire and the most important decisions about the academics, which have to do with promotion to tenure and so forth, that those are done by a small group of people. And the group I'm referring to is called the Academic Council, the Institute Academic Council, and it consists of the six division chairs, the provost, and the president. In that particular group, the provost is very important. The provost is responsible for the academic part of the Institute. I think we benefit from that structure. I know from looking at what happens at places like MIT or at Harvard that one of the benefits is proximity, the sense of having a connection with the important decisions, whereas elsewhere you get the feeling that professors are not so closely connected. They're not as aware of what is going on. I'm exaggerating a little bit. I'm not sure to what extent this is universally true. But it's certainly the sense I get, having spent some time at Cornell, where I'm a professor at large, and having been on a visiting committee at MIT, where I see the interaction between the deans and other people high up in the administration who are viewed as remote by the professors. At Caltech, you actually see these people who are at the top.
ZIERLER: And so as full professor from 1984, it would probably be fair to say that in the intervening five years, you were already in a position of seniority where you saw how these things worked at the Institute.
STEVENSON: Yes, I could see how the Institute functioned. And I thought it was accessible. That's a benefit.
ZIERLER: Scientifically and administratively, you're saying.
STEVENSON: It's a benefit scientific and administratively, but I'll say it now, although we may get into this later, that I actually think that a desirable thing certainly at Caltech is to have an administration that doesn't do much, that stays out of the way. Because after all, the essence of a place like Caltech certainly is the excellence of individual scientists. And that's what you want. You want to be in an environment where the existence of the administration is not pervasive. It's actually better that it be, so to speak, invisible. That it's better that you just do your own thing and do it well. I have to say that I'm not so sure that this is true anymore, but it's certainly the way Caltech used to function.
ZIERLER: Dave, that's a perfect place to pick up for next time when you decide to become division chair. So we'll cut it there.
[End of recording]
ZIERLER: This is David Zierler, Director of the Caltech Heritage Project. It is August 18th, 2021. I am delighted to be back with Professor David J. Stevenson. Dave, once again, it's good to see you.
STEVENSON: Good to see you.
ZIERLER: Dave, today, what we're going to do is get into your administrative responsibilities at Caltech. As prelude to your own tenure as division chair, first tell me a little bit about who your predecessor was, in that role, and what were some of the major issues that the division was facing at that time.
STEVENSON: We went through a little bit of turbulence. We had, for a very long period of time, including my arrival at Caltech, somebody called Barclay Kamb, who had been chair, in the end, for 11 years, I believe. We struggled to identify somebody to take his place. In the Caltech tradition, it is standard to look for somebody internal. And I think that makes sense, because Caltech is kind of special, and you need to have somebody who understands how the place works, and that's usually not true for somebody from outside. But to make a long story short, after some meetings, quite lively meetings, we were unable to identify somebody to take Barclay's place.
I should explain, perhaps, that we are democratic, and we do involve junior faculty. I suppose by the time of those decisions, I was no longer junior. But the point is that everybody in the division who was a professor, including assistant professors, were involved in the discussion. This was not a decision made by somebody high up. It was an attempt to reach a consensus view of everyone, and we did not succeed.
We then moved into a phase which was unusual for us, in which we looked at outside candidates. We eventually settled on Peter Wyllie, who came to us from the University of Chicago. He was the one who became division chair in 1984 or 1985; I don't remember exactly. He was not hugely successful. It's not clear to me in retrospect that you can read a lot into that. Some people were unhappy. I was not one of them. For whatever reason, it became apparent after two years or so that we should look anew, look for somebody new. And so we did, at that point, perhaps with a little bit of pressure from the provost in that particular case—the provost was Robbie Vogt—we did end up choosing Jerry Wasserburg, who of course was a prominent faculty member, very prominent scientist, geochemist, who had a large lab at Caltech at that time. And so he became division chair I believe in late 1986.
And, things changed. It's clear in retrospect that he was not popular as division chair. And it was an interesting story, because he had some fairly strongly held views about the direction of the division. I knew about these. I didn't necessarily disagree with them all that much, but some people certainly did. And he certainly operated more like a king, operated more like a forceful leader, and this did not go down well with some people.
During that period, he pushed the idea of cosmochemistry. I even wrote a proposal for that, along with him, to the National Science Foundation. They were supporting centers at that point. This was not a regular proposal. This was a really big proposal, because the National Science Foundation was setting up new centers at that point, and so they liked this top-down approach, which I personally don't like. But anyway, that's how I knew what was going on. Jerry Wasserburg was also rather forceful to some junior faculty, in particular Joe Kirschvink. That did not go down well. So to make a long story short, there was a considerable amount of discontent going into 1989, and it became apparent that Jerry could not continue as chair of the division.
ZIERLER: Dave, I'm not familiar with the term "cosmochemistry." What is that?
STEVENSON: Cosmochemistry, as he and I interpreted it, really meant the relationship between Earth and the universe around us. So we received stuff from space that made up the Earth. We also received rocks that come from the asteroid belt. And so it's to some extent a bridge between what we call Earth science and what we call planetary science, but a bridge that is built through looking at material properties. So you think about the gaseous medium in which the planets form, you try to understand the consequences of the formation process. Cosmochemistry also has links to the interstellar medium, but the way we were talking about it, it really had to do with links to the Earth as we see it.
ZIERLER: Now, what specifically was controversial about the push during Wasserburg's tenure for this new field?
STEVENSON: I don't think it was so much the push. It was just that Jerry had strong opinions about directions we should take, and he was interpreting his role as chair more like a king. Like a dictator rather than one of the mob.
ZIERLER: And so what were the circumstances of you being asked to succeed him?
STEVENSON: It all happened rather quickly. It was decided without my involvement—which would be normal, to exclude the person who is going to be asked to do it—it was decided that there would be a temporary change of chairmanship, and Peter Goldreich became chair for a limited period of time. He was one of those who was taking considerable interest, appropriate interest, in the difficulties that Jerry was encountering. He basically said to me [laughs] that he was going to be chair for a few months, the summer of 1989, and then I would take over, at what you might call the normal time. Because the academic year, of course, begins in October, and so it's not the same as the calendar year. That's a detail, actually. But in any event, he was actually acting chair of the division during the summer of 1989, and then I took over the role of chair in September or October of 1989.
ZIERLER: That's notable, because I always assumed Goldreich was in PMA, but I guess not.
STEVENSON: He's in both. He was hired in our division. We actually went over that, although we didn't explicitly talk about how the appointment was finally made. But indeed, his background would have made him suitable for PMA, and they eventually embraced him. But at the time he was hired, it did not fit quite so well with their view in astronomy at least of what was of greatest interest, which was outside the galaxy. Peter did things, still does things, inside the galaxy.
ZIERLER: [laughs] Now, having only been named full professor five years earlier, was your being asked to be division chair—obviously, you're a full professor. You're a senior scholar. But just chronologically, were you relatively young for that position at that time?
STEVENSON: Yes, but not that anomalous, because Barclay Kamb had also been fairly young when he became chair. And Bob Sharp, well before him, actually, had become chair when he was fairly young. So okay, it was young when you think about the history of the entire Institute and all its divisions, but not hugely so. It really came down to a question of whether there was anybody else who was suitable. And it seems rather pointless in retrospect, but when you're confronted with a situation like that, you have the conceit of saying that, "Well, this is not really something I want to do," but then you look around—"Is there someone better?" [laughs] And you don't see that person. That doesn't mean they didn't exist. It's just the point of view that you have at the time.
ZIERLER: To probe that a bit more in terms of your motivations in saying yes, there's a spectrum, of course, when you're asked to do something like this. On one end, it can be something where you're strictly motivated by a sense of service, that this is something that you have benefited from, and it's something that you can give back to the Institute. And on the other side of that is a more activist approach, where you recognize the power invested in this position and you see specific areas where you'd like to affect that positive change. Overall, where would you have put yourself on that spectrum when you accepted this?
STEVENSON: Much closer to the first, the sense of service. But also the sense that we had gone through a turbulent period, and we needed some calm, and I thought I could provide that. But I did not have an agenda. It was not as if I had a very specific notion about what should be accomplished. I still think that way. Obviously I have opinions about the relative merits of fields, but for the most part, and I've said this before, I think of administration as being done best when you get out of the way, done best when you let people do what they can do as individual scientists, to their best capability. That's what matters most. Obviously there's more to it than that.
The other aspect that I haven't mentioned is that when you are division chair at Caltech—and this is special to us, I think—you get involved in every tenure and appointment position for the entire Institute. So there's an aspect to the position that transcends being a leader among colleagues within your own field. There's much more to it that comes from this institutional aspect. And that is educational. I don't claim to be especially good at it, but I felt as though I had enough understanding of different areas of science that I could at least do it.
ZIERLER: Dave, what is that forum where division chairs are involved in Institute-wide decisions?
STEVENSON: It's called the Institute Academic Council, and it's the six division chairs and the provost and the president. It's very powerful. It meets once a month. It operates on the basis of information provided in advance in the form of rather lengthy documents that are submitted by individual division chairs. Occasionally other pieces of information, usually put together in some rather thick folder [laughs]. You then just work it through. It also involves deciding on the salary of everyone. It involves at least discussion about key issues that are made at the highest level, which really means provost and president. Of course the Board of Trustees are important, and at the very highest level, they occasionally make decisions of great importance, but more commonly, they act as a rubber stamp. The provost on the academic side is extremely important, and is actually running that Academic Council that I'm referring to. But it leads to votes on individual cases, votes on the basis of presentation from the relevant division, votes about whether that division should be permitted to hire someone as an assistant professor, or permitted to advocate that that person go forward for tenure.
ZIERLER: What are the expectations when you are contributing to a decision of tenure in a field outside of your expertise? Does your vote weigh less in those matters, or are you expected to get smart about the topic at hand so that you can equally assess one's contributions to the field?
STEVENSON: Well, the reality is that you rely very heavily on the relevant division chair to provide the information that you need to assess the merit of the appointment, and to some extent it depends on trust. But of course, part of the process is to come up with probing questions about whether this person is really as good as some people are claiming, how well that person will fit with what is going on at Caltech, and so forth. And it varies a lot. If it is someone in physics or chemistry, even sometimes in biology, I felt as if I could at least understand what that person was doing and why it might be important, and appreciate the merit of the letters that had been written. Because of course in these processes, you get letters from outside scholars telling you about the merits of a person. On occasion, if it's in humanities or if it's in pure mathematics, it can become very difficult.
ZIERLER: What did you learn in this unique vantage point about the Institute's overall culture of promoting?
STEVENSON: My reaction to what I saw was almost entirely positive. I saw some differences between the divisions. There are different cultures, and you respect that. I think GPS, the division of which I'm part, has a very healthy culture that nurtures young scientists and takes the initial appointment very seriously and then supports the scientist so that they're likely in fact to get tenure because you made a good choice in the beginning. There are some differences among the divisions, but for the most part, I saw a very positive and healthy climate in which excellence was valued, and people were looking to the substantive evidence of that excellence.
Let me be clear about that. I believe that some institutions do not do it as well as we do. There are even institutions that look at, for example, how many papers somebody has published, which I think is not relevant. Or whether they published in particular journals, which I think is not relevant. What's relevant is the quality of the science. And so of course you have to have a set of colleagues, a set up that identifies that excellence. And that means that you have to develop some understanding of science beyond just the trivial level of looking at the number of papers or citations or glowing comments made by faculty elsewhere.
ZIERLER: Dave, if I may draw an even starker divide, at places like Harvard or Stanford, the common reaction is that junior faculty, assistant professors, do not get hired with the expectation that they will be promoted. That they are essentially glorified postdocs. It sounds like what you're saying at Caltech is that that initial hire at the assistant level comes with the hope or even expectation that Caltech will provide the resources, that faculty will shine, and that tenure is what everybody hopes for. That's the process by which things move forward.
STEVENSON: That's correct. What you said about Harvard used to be true. It's not quite so true now. Harvard brings in outside people in the tenure process, and I was even involved in that. Harvard has a very stressful system, because they bring in an external committee, and we meet with the president, and tough questions are asked, and then in the absence of the candidate, specific people from that department are brought in to make comments, sometimes critical ones. You could see that this was very tough for the president, especially. I'm actually talking about the School of Arts and Sciences, which is nearest to us. I don't know about the business school and so on. Harvard is a huge place. But you're right; Harvard used to hire people at the assistant professor level with the expectation that in most cases they would not become full professors, and then they would hire full professors in from outside. I think that's a bad system. To a small extent, Harvard still does it. I'm not so sure about Stanford. Stanford has a big school that is in some sense comparable to what we do in Earth Science. It's not clear to me that they're hugely different. I don't think their quality is as high. But I don't have personal experience about how they operate. I do have experience about MIT. I think we do better than MIT. I was on their visiting committee for more than a decade.
ZIERLER: When you accepted this position, what expectations did you have for yourself, and what expectations did the provost have, in terms of continuing your own research? And how did that work out? How naïve or realistic were you, in the end?
STEVENSON: I need to explain that the division I'm part of is relatively small. It's now bigger, but at that time, it was 30 professors. By contrast, the PMA division, which has an extraordinary mix of people—PMA is Physics, Math, and Astronomy—the astronomers actually think about science different from the physicists, and the mathematicians are in a whole different world. So the division chair that I knew at that time, Gerry Neugebauer, had a tough job on his hands. He was also trying to run Palomar.
So I'm wandering away a little bit from the original question, but the point of my comments is that being chair of PMA is a very difficult job. Being chair of GPS is less difficult. It's more like half time. You could continue your research, and I did. And it wasn't really a problem. It is a fact that during that time as division chair, I chose, because of what we had done in geophysics and geodynamics, to move a little bit more into geodynamics. It is a period where I got involved in something that I had a previous interest in, which is mantle convection, the way that the Earth gets rid of heat, the relationship between the internal motions of the Earth and plate tectonics, things like that. I still have an interest in that, but that period as division chair just happened to coincide with a period where I got quite heavily involved in that kind of geophysics.
It also happened to be a time when Caltech had the fastest supercomputer in the world, for 18 months. [laughs] So that was a temporary thing. There was a very good student, who's now a professor, Paul Tackley, who actually did a PhD thesis under my guidance, on that kind of work. But bottom line, I was able in large part to just continue doing research. And as I've already said, my attitude towards administration is minimalist, so I wasn't an activist. I wasn't spending a lot of time understanding the budget, which might have been a mistake. [laughs] But if you've got a bunch of good people, then things will keep rolling along without the need for leadership.
ZIERLER: As you say, you did not come in with an agenda, but of course the issues will find you in this role. What did you have to deal with, for good and bad, during your tenure as division chair?
STEVENSON: For the most part, not a lot. There was one issue that some people took very seriously, which had to do with analytical facilities. It was a complicated issue, but part of it had to do with the fact that there was an instrument that was funded by NASA that was not available to the faculty as a whole and was run by Jerry Wasserburg and his lab, which is very large. There were people who felt that it should be more open. I did get involved in that. That was a stressful situation in which in the end I basically required Jerry Wasserburg to make the instrument available to other people. At a cost. [laughs] There were some other issues that arose that are relatively minor, but for the most part, things just happily went along, people doing their science.
ZIERLER: Did you have opportunity to interact with trustees, as division chair?
STEVENSON: I did not interact much with trustees as division chair. There is an annual meeting, which at that time was held at Palm Springs, has been held in other places, Ojai and so forth. And at those meetings, you had over a period of a couple days quite a bit of interaction with trustees who would go to that meeting. Not all trustees go to every meeting. But the job has changed. At that time, there wasn't a push—there wasn't much push—to raise money. I got into that just a little bit, because people said, as they still do, that the Seismo Lab, which is so well known at Caltech because we have earthquakes, should be endowed. You know, "Somebody should dump a whole lot of money on us to run the Seismo Lab." After all, we are, along with the U.S. Geological Survey, which has a facility just across the road from us, we are the people who in some sense take care of what is called the Southern California Array. Or at least we did at that time. Now, it's much more heavily associated or substantially associated with the University of Southern California, but we still have a big role to play. This is still the hub of activity. If there's a big earthquake in Southern California, you have the media come to a room. I actually helped set up a media room. That's one of the things I did as division chair.
There are other things, but I just don't remember them. But the money saving aspect inevitably involves the trustees, because some of the trustees are wealthy people. Nowadays, I think it's fair to say that the division chair has a bigger job than when I did it. Because there's the expectation nowadays that a substantial amount of money come in through ways other than the federal government. The main source of money to do science here, as at places like UCLA—even though it's a state school, it's mostly federal money. Federal money is what keeps the wheels of science going, because that's where you go to, to get money to do research—through the National Science Foundation, or through NASA, or through the National Institute of Health, or DARPA, or whatever. Those organizations are federal organizations. They have the big bucks. That's where you get your support as an individual investigator. But nowadays, there is this desire to also get rich people to give you money. Back in those days, there were fewer rich people. Really rich. I mean, billions. [laughs]
ZIERLER: Was that personally important to you, to hire new faculty where you could? To make sure that the division would grow to some degree?
STEVENSON: Yeah. So this was a transitional period. What I mean by that is that the division grew back in the 1960s—of course it existed much earlier, but some of the growth, some of the hiring, predated my arrival. And some of these people were approaching the time of retirement. And so you could see that at some point, there would be the need to hire new young people. I became division chair a little bit before that time. Most of that happened in the 1990s and through the subsequent period, a decade later. So we hired a lot of young people in the 1990s and a decade later, less so when I was division chair. In the five years I was division chair, we hired four people. Actually, that's more than the previous five years. But the rate at which you hire is really dictated by the rate at which people, in those days at least, were reaching about age 70. There was a time when people had to retire. [laughs] Then the federal law changed, so people got upset about that. But some people benefited, because they were a little bit younger, and they did not have to retire.
Anyway, you're right that that's an important part of being division chair. But keep in mind that if you do it right, it really comes down to a democratic process where you have a committee that's set up that you're not part of, that brings forward recommendations. They get voted on by the full faculty. Then of course you as division chair have the job of taking it forward to the Institute to be considered by the Institute Academic Council. And of course, you have to do a good job at that. And to be sure, you provide some guidance. You try to think through what kind of planning is appropriate for future appointments.
The planning process, I forget the details of what happened before I was division chair, but actually I, towards the end of my tenure, asked a faculty member to actually do that, and he did it. The person I asked was Peter Goldreich. There is a thing called the Goldreich report, which is quite influential. There must have been something earlier; I don't remember. During the time I was division chair, there was discussion about getting more into geobiology. That didn't really come to fruition until after I was division chair, but the beginnings of it was when I was division chair, bringing people to Caltech who were more biological than we were, and learning through seminars the connection between geological considerations and biological considerations. This was an important direction for us to take. We did in fact do some of the pioneering effort in that field. Other institutions are doing it now, but we were one of the first to seriously recognize the importance of the connection between biology and geology.
ZIERLER: Dave, on the other side, chronologically, of the hiring decision, would it fall to the division chair? Was there ever any need to have that difficult discussion with more senior faculty about them thinking about retiring at some point?
STEVENSON: Not really. I don't remember any instance where I had to exert pressure. I think at the beginning and maybe even towards the end of my tenure as division chair, people were required to retire at a particular age. It was probably 70. I don't remember the details. In any event, I was not placed in the difficult situation of trying to persuade somebody who had been around too long.
ZIERLER: When you decided to step down, was the five-year term relatively short, or that's about average for a division chair's service?
STEVENSON: It's relatively short. The tradition at Caltech, common practice at Caltech, is for somebody to do it for ten years. However, five years is the term. When you agree to do the job, that is effectively the period that you agree upon. Now obviously, some people don't even make five years, But that is the notion when you agree to do the job, that you will do it for five years. That's a very common practice. Very frequently, people who are doing okay will approach the end of the first five years with the possibility that they will continue for another five years. That then becomes a discussion with the provost, and often there is a committee set up. I can remember participating in some very small group that would go around checking with faculty about whether they were comfortable with so-and-so continuing to be chair of the division. This was after I had been division chair many years later.
I did something different. I preemptively told people, after four years, that I was quitting after five. So I gave them plenty of warning. [laughs] Because I didn't particularly enjoy it. Let's face it; when you're the division chair, there's some interesting stuff, getting involved in hiring new faculty, getting involved in how the Institute as a whole runs, but there's also a lot of other stuff.
ZIERLER: That pulls you away from science, is what you're saying.
STEVENSON: That takes you away from science and is not particularly interesting. The fact that it took time away was not foremost in my mind. It was just simply that I did not find it particularly interesting. I did not find it particularly interesting to be confronted with the questions of how we should spend money and things like that. [laughs] I'm not saying it's unimportant; of course, it's important. I just didn't find it interesting.
ZIERLER: Now in terms of the reporting structure and the personalities involved, what are the kinds of issues that you would go to the provost with, and what are the kinds of issues you'd go to the president with?
STEVENSON: It would be very rare to go to the president, because the president at Caltech may be expected, and is in fact, paying attention to what's happening on the academic side, but most of his or her job really has to do with the non-academic, that is non-faculty, issues, a major part of which has to do with raising money, which of course involves the trustees. And I suppose in fact it also involves interaction with the students, which you could call academic.
And I know this may be confusing to an outsider, but when I use the word "academic" I'm actually talking about the professorial [laughs] faculty. The students obviously are part of the academic realm, but the operation of the student aspect—the classes, the teaching, and so forth, which I think is very important—there, we actually do have a dean, and we have a vice president. So there is a wall, if you will, although it's porous, between the way you think about the decision-making for hiring faculty, and what happens with teaching. Some people may find that offensive, but it's actually true. Nowadays we pay actually more attention to teaching than when I first arrived at Caltech. I think teaching is very important. We have enough faculty members that even though only half of them are good at teaching, that's good enough. [laughs]
ZIERLER: [laughs]
STEVENSON: But, let's be honest about it—the way an institution like Caltech excels is through hiring people who are good at doing research. And you sure hope that they can be good at teaching as well, but that's not the primary characteristic you're setting out to find.
ZIERLER: Dave, of course this is a long time ago, but in this role, do you have any recollection of anybody in these meetings, these executive-level meetings, talking about the importance of making Caltech more diverse? Was that on anybody's radar back then?
STEVENSON: Yes, it was, but not prominent. You know, there's a curious thing—maybe it's not a good thing—about these issues. That when you have a group that is small enough that it hasn't reached a critical mass—in this case, it would be women, even more so African Americans—it becomes less likely that you hear that voice. I think our division has actually done fairly well. Jerry Wasserburg was proud of the fact that he hired a woman on the faculty. And I also hired a woman. And we have hired many more. We have in recent times, for example, in planetary science, hired more women than men.
When it comes to African Americans or other issues of diversity, which for Caltech also means the Latino population, it's a significant issue, it's difficult, at least in the case of African Americans, for the simple reason that the number of people that are even in contention is so low that striving for diversity on that count is very hard to do. But it's not as if people were unaware of the issue. I don't recall the exact timing of some of the committee activities I was involved in, but there's no question that in that period, maybe in the 1990s more so than the 1980s, there were committees I was involved in where the issue that we now call diversity came up. And it would be partly the issue of women, and separately the issue of Latinos. The issue of Blacks did not come up so much, even though it's obviously important, just simply because the numbers are so small.
But the Latino population of course in California is high, and it was growing, and it was growing rapidly. Some of the students in that area were concerned about the lack of mentorship at Caltech. It's an understandable thing for them to have been concerned about it. I think we do a lot better now, but some of these issues are difficult to address at our level. I actually think a lot of these issues are societal issues that are of importance in families and in the kindergarten level and at elementary school and at middle school. More important than the things that people talk about for high schools and colleges, just simply because that's when the stage is set.
Caltech and the Presidential Search
ZIERLER: How did you come to serve on committees that were searching for presidents at Caltech?
STEVENSON: Well, I don't actually know how I was chosen, but the normal practice would simply be to look around and see somebody who had some awareness of how the Institute works. And so in the mid 1990s when I served on a committee chaired by Kip Thorne, a committee that eventually led to the choice of David Baltimore—when that happened, it seemed like a natural enough choice because I had the background.
ZIERLER: What did you learn about Caltech, very broadly, in the kinds of things that the Institute across the board was looking for in leadership?
STEVENSON: So what happened with the presidential search, and some would say it has continued on this pattern, is that people would often say—faculty would often say— "We need an outstanding scientist. That's the nature of the place. That's how you get respect." It's a question of respect. It's not necessarily a question of knowing what individual scientists are doing.
ZIERLER: Respect—you mean internally, or externally, or both?
STEVENSON: Internally, primarily, although external would matter as well. I will get to that, actually. But there was this feeling among some people that it would be nice to have somebody who understood science. And so, when I came to Caltech, that was Murph Goldberger, who was a physicist, a well-regarded quite distinguished physicist, elementary particle physics. And it was felt by many people, rightly or wrongly, that he was not doing a wonderful job on the administrative aspects. When it came time to look for a new president—this of course I'm talking now about the mid 1980s—people said, "Well, let's find an administrator." [laughs] And so they chose Tom Everhart, who I know well. He's still alive. I see him on occasion. And so Tom Everhart became president.
And then people were unhappy in the opposite direction [laughs], of saying, "Well, this person doesn't understand Caltech as well as he should, and perhaps that's because he's not so much eminent as a scientist" and so forth. And Tom Everhart is actually quite accomplished, although more in what for Caltech we would call engineering. What we call engineering at Caltech, other people would call applied physics [laughs], so you have to be careful about the word usage. Anyway, so when I became involved, there was a strong feeling, which came actually from Kip Thorne but was not just him—it was clear that there were other people who felt the same way—that since we had not been particularly successful with our administrator, let's find [laughs] a scientist. You can see where this is going.
ZIERLER: [laughs]
STEVENSON: This is a pendulum, swinging back and forth. And so there was great enthusiasm in seeking a very distinguished scientist, and that of course is what led up to the choice of David Baltimore. Of course, the pendulum swung again, although I would argue it was partly by chance, not by design, with the following president, which was chosen by a committee that I chaired. That was Jean-Lou Chameau. And maybe some people would say it swung again with. Tom Rosenbaum, the current president. I'm not sure that this notion of the pendulum is actually a correct description of how it happened, but it is a correct description of the data.
ZIERLER: How shiny an object was David Baltimore's Nobel Prize in the consideration leading to him becoming president?
STEVENSON: I think that at that level, and this includes many faculty members at Caltech, there is enough understanding of the Nobel Prize that people are not swayed that heavily by it. What I mean by that is that people understand at that level that there is sometimes an element of chance—being in the right place at the right time, being part of the right group of people—in how Nobel Prizes get awarded, and that there can be people who are equally capable, equally distinguished. So it was not necessary that somebody have a Nobel Prize in order that they be respected as an outstanding scientist. But it is probably true that at the level of trustees and people outside Caltech, this would be regarded as a positive thing. It looks good in articles in The New York Times, in the L.A. Times. And some people think that's important.
ZIERLER: What role did the trustees have to play in the selection of the president?
STEVENSON: The wonderful tradition at Caltech, which as far as I know has persisted up to the present—the trustees play a remarkably small role in the choice of president. The way the presidential search works is that we set up two supposedly parallel committees, one consisting of professors, the other consisting of trustees. The committee that is made up of professors does a hell of a lot of work. They talk to all the constituencies. I should make it clear that when you choose a president—perhaps it's obvious—you're not just trying to satisfy your colleagues. You want to be sure that you're choosing somebody that is suitable for other members of the community—the students, the staff, and so forth. JPL, even. JPL is important. In both searches, I was involved in meetings at the Jet Propulsion Lab. Because Caltech runs JPL. JPL is bigger than the Caltech campus, but we run it. It's a big institution, a lot of federal money.
So you have this committee of professors, and they do a lot of work. And for the chair, which was a position I took in the search around 2006, it's a full-time job for approximately six months. You don't do anything else. Because there's a huge amount of effort put into compiling a list of candidates that might be in the order of 200. That happened in both the searches I was involved in. There's an enormous amount of effort put into whittling that down to a manageable list that might only be ten or something like that. And you work away. You meet every week. You have a standalone computer that is not connected to the internet. Secrecy is very important. It's all this business involved in making sure that you do things without attracting attention, even though you may be traveling around.
So you travel around the country. You visit. You collect information by visiting professors and presidents at other institutions. Collecting all sorts of information. So there's a huge amount of work that goes into this. And then at the very final stage, essentially, although of course [laughs]—you tell the trustees what you're doing, but at the very final stage, you then compile a report which is suitably short—you know, an executive summary, a couple of pages—and you go to the trustees, and you say, "This is what we think." [laughs] And the trustees are good. Don't get me wrong. I'm very impressed by Caltech trustees. They're knowledgeable. They ask good questions. But they basically accept what we do. And that's what happened in the cases I was involved in. They accept what we do.
The final stage, the very final stage of actually deciding who we're chasing after is actually done by the trustee committee, but very heavily guided by the faculty. I'm not sure about the other searches, but in the case where I was chair, I was actually in the trustees committee meeting, which was presented to me as a little bit unusual but I don't actually know how unusual it was. Anyway, it was illuminating. It was interesting. There were some trustees who take this very seriously indeed. It's only a subset. So it may only be half a dozen people, but these are people who know a lot about the real world, which is not always true of professors.
So I think we do a good job. We do of course on some occasions seek through a professional company information of the kind that could be of concern. Like maybe this person is an alcoholic, or maybe this person has done something bad in their past. These issues do arise. So there are things like that as well. But mostly we do the work.
ZIERLER: Dave, to get back to the science, in 1993, when you were thinking about stepping down, what was your research at that point? What were you excited to be able to get back to full time?
STEVENSON: I didn't view being division chair as changing my focus that much. It is true I was doing more geophysical stuff, meaning the Earth, but I'm not at all sure that that was so much tied to being chair as it was to the state at that point of the planetary program. The planetary program, as I've mentioned already, had gone through a period of maybe not decline but certainly a steady state of rather low level of activity, at least for the things I was interested in. And it picked up a decade later, I suppose. I was interested in the Earth, but I don't think being division chair was actually making a huge difference in what I was most interested in.
ZIERLER: What were some of the issues with regard to teaching and improving the student experience in the mid 1990s?
STEVENSON: I was involved in one other big issue, in a fairly major way, and this is after I was division chair. I became chair of—in fact the inaugural chair—of something that I'm not even sure I can tell you the exact name of [laughs] anymore. Core Curriculum Steering Committee, something like that. To provide background, Caltech decided—and this is where the trustees were involved actually—way back in the 1920s, that all Caltech undergraduates should take a certain set of classes. And this evolved a bit over time, but Caltech is actually a fairly conservative place. Doesn't change things much. Certainly, in the time between my arrival in 1980 and the mid 1990s, something like 15 years, there was no perceptible change in the way physics was being taught, or chemistry, or mathematics. The feeling was that it was time for a change. There were other committees before the one I was involved in who were studying the issue. But then there had to be a committee that actually did something, [laughs] meaning push through a decision, take it to the faculty board, get it voted on, get it to actually change so that the catalog, which is the statement of what classes an undergraduate has to take to graduate, there had to be some process to actually implement change. To get that to change. And I was chair of the committee that did that.
This was a contentious process. The biggest issue was the reduction in the amount of physics, chemistry, and mathematics, and the introduction of several new things, but primarily and especially the introduction of a biology class. Prior to that time, Caltech undergraduates were not required to take any biology. The feeling was that it was timely to get students involved in thinking about biology. By the way, this was contemporaneous with being on the committee to choose David Baltimore as president, but not intimately linked. We told him about it, but it's not as if he was pushing this or anything. This was done of our own accord. And it was done in recognition of the importance of biology as part of modern science.
The reduction in physics and chemistry sounds dramatic, but in truth, it wasn't as dramatic as it would seem, because the process that we used introduced a little bit more flexibility into the curriculum. I'm actually mixing what I did with what happened a decade later, when physics was reduced to one year and chemistry was reduced to one year. But we were already cutting physics back from six units to five, and so forth. But a lot of the changes that were enforced could best be summarized by saying, "Let's have some biology. Let's have the opportunity for students to learn about other areas of science." That's called the menu class, one form of which was a basic geology class, which I taught. [laughs] I wasn't manipulating for that to happen, but that was one of the things that happened, in competition with astronomy in some sense. I think it has been successful. I certainly feel that learning about biology is an important part of being a well-educated modern scientist. Biology is a hugely important part of science.
ZIERLER: What was your role in the competition hurdles that the freshmen would go through?
STEVENSON: Are you asking about a class, Physics 11?
ZIERLER: Yes, that's right.
STEVENSON: That class was begun by Tom Tombrello, a physics professor who later became chair of the division of PMA. I got involved in the class—it's called Physics 11—about five years after it began. I believe it began around about 1990. I got involved about 1995. I'm still involved, so I've been involved for more than a quarter century. This is unique to Caltech. I think other places may have copied it a little bit, but for the most part it's unique to Caltech, and it works for Caltech because we have some particularly outstanding freshmen.
What we do is provide by competition the opportunity for students to get involved in the class. Incoming freshmen are given what Tombrello called hurdles. They're basically questions. They're unorthodox questions, meaning that they're not the kind where nowadays you would go on the web and find the answer. They're not like that. It's not even necessarily a physics question, although it may have aspects that you could call physics. The students would then submit their attempts at these questions. This is not a class; this is in addition to all their work that they're doing in the first quarter at Caltech. We would then grade their responses.
When I say "we," the way it worked once it got started is that the grading was done by the existing students. So the structure of the course, which is structured essentially around a calendar year, not academic year, is that in the first quarter, which is the winter quarter, the students would be introduced to each other, would talk about what they did in the hurdles in the previous quarter, and would start to get involved in some kind of research, which would continue for the next quarter. The classes were in front of a blackboard, and were primarily run by the students but guided by the faculty, which at that point would have been Tom and me. So the student would go up to the board, attempt to answer some question or explain what they're doing. They would then have a summer project, which in some cases might be outside of Caltech. And in the final quarter of the calendar year, which is the fall quarter, they would then be grading the input from the new freshmen. At that point, they would be sophomores. Students are in the class for a calendar year that consists of three regular quarters plus a summer.
It works because the very best physics-oriented students at Caltech are exceptionally good and can get involved in research effectively and have interesting discussions about all manner of topics. It has been a stimulating experience, coming into contact with very good students. It's labeled as a physics class, but what happens at Caltech is that people who might start off saying they're interested in physics or even students that are interested in something else get into this class and then go off in all sorts of other directions. Former students might end up on Wall Street, or I can think of cases where one of them is a professor at MIT. Another one is a professor at Rice. In that case, more biological or even medical science. It has a very diverse and interesting group of people. On occasion, we have brought them back as a reunion. So it's something very special about Caltech.
Enhancing the Caltech Curriculum
ZIERLER: Now the development of the new Caltech core curriculum, did this involve a different approach at all to the humanities and social sciences?
STEVENSON: We wanted to keep humanities and social sciences as an essential part of what Caltech undergraduates do, and we have. I'm not able to remember the details of what we did, but most of what we did in changing the core curriculum had to do with the science, not so much with the humanities and social science. We recognized the importance of that. We did not diminish that. It is of course a struggle, but it's an important thing. People here in humanities and social science—and it really is a statement more about humanities, because often social science means economics which means mathematics [laughs]—in humanities, the faculty in that area say that Caltech undergraduates are a very interesting population to get involved in, because they have no aspiration to become experts in Shakespeare or whatever.
ZIERLER: Dave, a very broad question—historically, given the fact that you served on the undergraduate admissions committee at different times in Caltech's history in your tenure, in the 1980s and then more recently, is your sense that the winning applicant from both eras was more or less the same? Or had Caltech evolved in the kinds of things it was looking for in an undergraduate?
STEVENSON: It's a fascinating question. I wish I knew the answer. I can tell you this—that when I first got involved in undergraduate admissions, the percentage of women students in the student body was very small. Maybe it was 15%. Anyway, it was very small. The files that I saw were heavily weighted towards male applicants. I think it's true, but somebody else would have to check the statistics, that this reflected the nature of who was applying at Caltech. I'm not trying to defend it; I'm just explaining what happened. It's my impression that it was simply not viewed as a place where women would apply. And maybe we should have done more even then.
I was even on the admissions committee when we made the first change, and it was a major change, and it was an interesting one. It was done by a new director of undergraduate admissions by the name of Langdale, who came to us I think from MIT. It was in the 1980s—it may have been 1987 or thereabouts—and he introduced something which I'm sure has changed since, I know has changed since. He introduced what he called a matrix system, which placed less emphasis on quantitative measures. Quantitative measures include SAT, maybe also grades, although students applying to Caltech tend to have saturated grades, tend to be all As. It's not very helpful. But still, you can read what they've done and get some understanding of their merit, and not just look at the grades. So he certainly took what I think was the correct point of view, which was that you have to look at the student as a whole, and not just look at quantitative measures.
I mentioned SATs, but I don't think, as far as I can recall, I don't recall that that was ever really a dominant factor. Again, it was because it was saturated. I mean, almost everybody who applies to Caltech has good SAT scores. The only value of the SAT score is to throw out an admittedly substantial number of people, but it's at a low level; it's not used to distinguish. It still isn't used to distinguish between qualified people. So on a scoring system where the number can be as high as 1600, we don't think of 1560 as being better than 1450, but we do think 1450 is a hell of a lot better than 1250. So in that crude sense, maybe it still matters.
To come back to your question, there weren't a lot of women scientists. At the undergraduate level, because I was not an undergraduate in the United States and don't know a great deal about high schools, I can't really judge what happened. But I can tell you this piece of factual information—when I was a graduate student at Cornell in physics, the Physics Department had 150 graduate students, and one was a woman. I actually met her and talked to her. She eventually dropped out. So [laughs] things have changed dramatically and for the better. At least in physics and in other areas related to that, there was and maybe still is to some extent a problem. It's not so evident in things that are more biological or geological, where we're much closer to 50/50.
Of course, we now have—and I know this, because I've been involved in admissions in recent years—we now have a pool where at the first stage, which is the people who are probably the best and are applying specifically to Caltech for the equivalent of advanced placement—they're seeking out the school they would most like to go to, in order to get acceptance early. I'm sorry; I'm blanking on the name of this category. But anyway, in that category, we are actually admitting more women than men. By a little bit. In other words, it might be 52/48 or something like that. That's not reflected in the final numbers. [laughs] Because women turn us down. Some of the women turn us down. But I do know from personal experience that this was not an easy place to be as a woman student in the 1980s. I saw that. I saw that, in the houses, and in the general environment. And that's unfortunate, but that's the way the world was.
ZIERLER: Dave, if we can establish a platonic ideal of a successful applicant to Caltech that merges both your experiences serving on the undergraduate admissions committees in the 1980s and more recently in the 21st century, given the extraordinarily low acceptance rate and the assumption that most of the people who apply to Caltech are extremely strong academically, they're extremely strong technically, what are the things beyond that, the something special that the committee is looking for, in terms of how well rounded they are, in terms of their interest and intellectual curiosity, perhaps even a sense of what kind of a future leader they will be after Caltech? What is the thing that the committee has prized, in your experience?
STEVENSON: Well, you're right that we do get a lot of people that look on the face of it to be very equally accomplished. Very capable in classes, able to handle the work. I have learned over the years that it's important to identify people who are able to do a lot. [laughs] Sometimes there are people who get As in classes but when you dig into it, you realize they worked really hard. So sometimes it's good to identify people who are able to handle the workload with ease. And this is important because a place like Caltech can be like a boot camp. It is like a boot camp. There's a lot of work thrown at students, and some students struggle, because they never learned to handle that much work. So being able to do a lot is important.
How do you see that? You see that through what else they do. You see people who do a whole bunch of classes, and they do them well, but they're also doing all this other stuff. They're playing the violin or something, or they're doing a sport. Interesting anecdote—I've noticed that people who do sport to the level of competitive sport actually do rather well academically at Caltech. Now of course that's a complicated filter, but it really seems to be true. Caltech, by the way, has more involvement of its students in sport than a place like UCLA. It's easier to get into a sport team at Caltech [laughs] because it's smaller. So that's a good thing.
We do look for quirky students. We look for something that's distinctive, something that's different. Having said all that, I have to add something that has worried me quite a bit and still worries me, although I think the people involved in admissions understand this and have made some effort to address it. And the thing I'm worried about is this—money. It does seem to me, still, that students who are coming from financially well-off families have an advantage. And I'm not talking necessarily about the obvious thing, which is paying for tutors, although of course they may do that as well. These students are good enough that they may not need that. But this business of being able to play the piano, or do some sport, which is actually expensive—some of them are expensive—and be successful at it, this is correlated with the economic standing of the family they're in. And so I look out for something that unfortunately you don't see too often, but helps make you feel confident that this is a good person to get—I look out for the person who happens to say in their essay that they have to get on a bus and go 90 minutes each way to school, things like that. Unfortunately, that's not common [laughs]. Because a lot of these kids get driven to school! And some of our best students are foreign students, and they're very good, often from Asia, but they come from economically advantaged situations.
So I cannot help but feel that economics is still a major factor. And to me, this is correlated somewhat with race, but actually separate from race, because obviously you can have people from Appalachia or whatever who are white, who also have this disadvantage. But sadly this economic issue, for reasons that have received a lot of discussion in recent time, those issues are often also related to, for example, whether they are African American.
ZIERLER: To return to geophysics, you referenced him briefly before, but I wonder if you can go into a bit more detail about your work with the student Paul Tackley.
STEVENSON: Paul Tackley was an interesting case. By coincidence, I met him on the very day I agreed to become division chair. That would have been 1989, he wandered into my office sort of contemporaneously with people who were passing by to check on what I had decided to do. He came to me without at that point intending to do a thesis with me. Most people at that time who were admitted to the geophysics option were coming here because of seismology. Why not? We're very good at seismology. Happens to be not the thing that I'm most interested in [laughs]. But he came from Cambridge, in England. For whatever reason, he was seeking other possible choices in addition to doing seismology. And he did do seismology. I remember a year later, when the decision came for him as to which direction to take in his thesis work, that it was not entirely straightforward. It was still a tossup at that point about doing something that was related to mantle convection, or something that related to seismology. The selling point around about that time was the fact that Caltech had acquired from Intel a supercomputer, on campus, which was temporarily the fastest supercomputer in the world.
ZIERLER: I assume this was a Gordon Moore connection.
STEVENSON: Correct. I knew Gordon Moore. Caltech had this connection with Intel through Gordon Moore, who gave Caltech a lot of money. At one point, he was even the richest person in the world. But nowadays—it was only a few billion dollars. Nothing! [laughs] So the Intel Touchstone Delta became available at low or in some cases zero cost, as a possible computational tool. It was a parallel computer. I don't want to get into the technical details, but it meant that it was not a computer where you could just go with your pre-existing program and say, "Here, run this."
Paul Tackley met with me and with Gary Glatzmaier at a restaurant in San Francisco at the end of that year to discuss how to take Gary's code, which had developed over a period of a decade, which had many applications, not just to the Earth mantle but to the core of the Earth, to the Sun, and so forth. So this was a multifaceted convection code, very complicated, hundreds of thousands of lines, probably Fortran. But it had to be parallelized. In other words, it had to be modified to run on our sort of a computer. And [laughs] so I said to Paul, "Do that." [laughs] And I can remember sometime later, probably a year later, a person who I won't name coming to me from the Seismo Lab and basically suggesting to me that I was doing something really bad [laughs] by asking this student to do such a terrible thing.
But Paul was very special, and he did it very quickly. Nobody else could have done it so efficiently. And he got it to run. He was the first person to figure out a particularly effective way of showing the results. This is non-trivial. It's one thing to get a computer to run and produce huge amounts of results. You also have to figure out how to visualize the results in terms of constant seismic velocity surfaces or density surfaces or whatever, so that you could actually see the character of the flow and so forth. So anyway, we ended up on the front page of Nature. It was an interesting experience. It also, in the end, involved Gerald Schubert, who of course I knew at UCLA, although again, it was Paul Tackley who actually took the initiative to involve Gerry. That was appropriate because of Gerry's experience with mantle thermodynamics. Of course, Gary Glatzmaier was involved. Gary, of course, independently of all this, became famous in the same decade because of a collaboration with Paul Roberts, who is now at UCLA, because they were the first people to run a somewhat realistic model of generation of Earth's magnetic field, using a related code.
ZIERLER: For a clearer window into the science of mantle convention and talking about these calculations, what are the calculations based on? What is the experimental or observational data that you're going on that would require a supercomputer?
STEVENSON: The funny thing about that whole effort is that in a sense, it's not right. By the way, that's often true with science—you do something that's influential, something that's important in our thinking about how things work, but when you get into the specifics, you realize that you were making assumptions that were not correct. So actually, the motivation for our work in large part had to do with the fact that the Earth has a phase transition. We now realize it's more complicated. But there is a phase transition at the place about 650 kilometers below the Earth's surface where you transition from the upper mantle to the lower mantle, and this phase transition has consequences for the way the material in the mantle flows, possibly limiting convection. And so we got into an idea of avalanches, which is probably not correct. By the way, Paul also agrees with this. I met up with Paul again just two years ago, and he agreed without prompting that this part of the story is probably not correct. But anyway, that's what we were doing.
And it has consequences for the scale of convection. So when you look at the Earth at the surface, you see a particular scale. Scale means how far do you have to go to get to the next plate. Do you just go 500 kilometers, or 1,000, or 5,000? And it tends to be somewhat larger. The Pacific plate is huge; it goes all the way from California over to Japan. It's a huge plate. The others are smaller. But that scale suggested something about the character of convection beneath. We were trying to get some insight into that, but we were also trying to get some insight into time variability, because it seemed that the Earth was not convecting steadily, but in an intermittent way.
It's still true, by the way, that if you look at what seismologists say about the inside of the Earth, that it looks as though there are some places where subducting material does not go directly into the lower mantle, that it's held up at 650. This was an attribute somewhat like what we found. The reason is probably not the reason we proposed. But anyway, that gives you some background into what we were trying to understand. The key thing I should have said is that it was a three-dimensional calculation, and a lot of the previous work of comparable resolution was Cartesian, convection in a box. But the Earth is not a box; the Earth is a sphere. And so you needed to do spherical convection, and that's not easy! And certainly not easy at high resolution. Having a supercomputer helps a lot.
ZIERLER: And just, again, to probe a little deeper here, pun intended, at 650, 660 kilometers down, how do you know at all what's going on?
STEVENSON: Well, you don't, really. But of course, you do have some idea from what seismologists tell us. That's the science of tomography. There was some involvement in that area at Caltech, but actually the most important developments were done at Harvard by Adam Dziewonski, who is no longer alive. But already people were looking at the lateral variation of material properties in the Earth expressed through variations in travel time for seismic signals produced by earthquakes. They were making what are called tomographic maps. It's actually a different technique than tomography—the tomography that was invented by medical science, which is based on absorption, and which people do when they have an MRI scan or something like that. But tomography gives you some idea of the distribution of materials of different density and different seismic properties as a function of latitude and longitude. And so we were guided to some extent by that kind of information.
ZIERLER: Dave, what about vulcanology? Is that a relevant field when you're talking about the Earth's mantle at that depth?
STEVENSON: Less so. In respect of melt migration, it's important. The process whereby melt escapes as solid rises initially purely solid and then becomes partly liquid, and the liquid percolates through the solid and accumulates and erupts at the surface. So that's of interest in mid-ocean ridges. I did that kind of stuff with David Scott. Huw Davies did it with subduction zones. But that's a separate kind of geophysics for the most part. Obviously, you are guided again by seismology, but it's not for the most part thought of as a key aspect of mantle convection. I have to say, though, that in the subsequent quarter century, people have got more into modeling convection including melting. You can question how well they do that, but people try and put everything in these days.
ZIERLER: We'll talk in subsequent discussions about some more recent ideas you've had about searching and understanding the Earth's interior, but for today, I think the last topic I'd like to discuss is your collaboration with Slava Solomatov on Earth's magna oceans. Tell me about meeting Slava and the work that you did.
STEVENSON: In 1989, actually around the time—no, it must be 1990—soon after I became division chair, I was asked to be part of a small group of scientists from the United States to travel to an international conference in Moscow. That was the first and only time I have been to what was then the Soviet Union, now Russia, and that was a very interesting meeting. At that meeting, I met someone who had been a student of a scientist I knew. The scientist's name was Zharkov, who I met on a few occasions, although he didn't travel a lot outside the Soviet Union. He was Jewish. They restricted travel to some extent.
Anyway, in Moscow, I met the student [Slava Solomatov] during this meeting and we got to talking, which was not easy. His English was not so good. But I suggested an issue with how to understand the evolution of Earth's inner core, and thought nothing more about it, until he caught up with me a few hours later and presented the answer. I was impressed. Upon coming back to Caltech, the timing may be more complicated than I'm describing, but certainly I got money from the National Science Foundation to study magna oceans, and it occurs to me that actually the impetus to make that proposal was Jerry Wasserburg, so it must have been earlier. In any event, I had money from NSF, the first time I had tried seriously to get money from the National Science Foundation. Most of my support has been NASA.
I needed to find a postdoc, so I thought, "Well, let's try Slava Solomatov." I suggested to him that he come to Caltech. That was a non-trivial thing to do. Because in order to accomplish that, I had to pay round trip airfares for him, his wife, and his young daughter. [laughs] Well, the daughter may not have cost much, actually; she was young enough. Anyway, it was a serious chunk of money, a significant bit of which was lost subsequently because it was on Pan Am and Pan Am went bust. Anyway, I got him to Caltech, and he was successfully involved in the project that I originally suggested to him, but as a good scientist, he took it off in directions that were different from what I suggested. That eventually led to three papers about magma oceans, which I think are an important piece of our understanding of how magma oceans work. In particular, the idea that they partially freeze, but that you might maintain equilibrium between the solid particles of the liquid if it's convecting vigorously enough. A lot of the pioneering work on how magma oceans work was done by Slava. I then subsequently persuaded him to leave Caltech. He wasn't happy about that, but it worked out fine, and he ended up at New Mexico State, and then subsequently Wash U in Saint Louis.
ZIERLER: The term "magma ocean," what is it meant to convey? What do we mean by using the word "ocean" in this context?
STEVENSON: We mean simply that it is liquid rock. This is terminology. If you want to just talk about a liquid layer, then you could say that Earth's core is a magna ocean. People don't call the Earth's outer core a magma ocean, because it's iron, but it is a liquid, and it is a layer. You could call the ocean underneath the ice shell of Europa or Callisto or Ganymede "magma" in quotation marks. But again, people reserve the word to mean liquid silicate. So it's the kind of stuff that the outer shell of the Earth is made of, but it's so hot, actually it has to be 1800 degrees Kelvin, so hot that it's entirely liquid. It may of course start to freeze at the top and freeze at the bottom, but there's an intervening layer. The idea was invented, I think, or at least popularized in the scientific thinking, by John Wood in connection with the Moon, and that's where the evidence was strongest, perhaps still is. Because on the basis of looking at the Apollo rocks, the samples that came back with the Apollo astronauts, it was deduced that the Moon was covered in a shell of a particular mineral called anorthosite primarily, whose existence could be explained as flotation from a magma ocean.
These were particles whose composition was such that they could float to the top and aggregate and therefore be, if you will, a little bit like an ice shell. Because ice—water ice floats on water, just as anorthosite floats on a magma ocean of silicates. It was popularized for the Moon. It was then picked up as an idea for the Earth. I have to say that I was initially skeptical, because it seemed to me that the Earth could cool efficiently by convection and avoid a magma ocean. I changed my mind subsequently, in part because the Earth clearly suffered giant impacts, which deliver a lot of heat all at once. And that's a good way to melt something.
ZIERLER: When you refer to giant impacts, looking at magma oceans following a giant impact, what makes the cut? How big of an impact are you looking at in this regard?
STEVENSON: When we use the term "giant impact" for the Earth, we really mean something that has global consequences, so that you can't just think of it as a local event. As a practical matter, we're thinking about a particular story for how terrestrial planets form in which you tend to form embryos that are of order of the mass of the moon or even Mars, and they then crash into each other. Those are the bodies we have in mind. The terminology has come to be stretched a little so that people also talk about giant impacts when they talk about the difference between the northern and southern hemispheres of Mars. To me, that's a much more modest impact, if it was an impact, which we don't know. But in the context of the Earth, people only talk about giant impacts when they're talking about something that is of order of the size of Mars hitting the Earth, so it's a hell of a big impact.
ZIERLER: When was the last one? Was the event 65 million years ago, would that count?
STEVENSON: Oh, no, no. That was not a giant impact. That was quite small. And [laughs]—
ZIERLER: Just to get a scale of what you're talking about, if that was quite small [laughs]—
STEVENSON: Ten kilometers! Tiny! So Mars is 3,300 or so kilometers in radius. The bodies that hit the Earth throughout most of Earth history were considerably smaller, although there are people who have talked about later impacts that might be as much as 100 kilometers. But those are really small. So the giant impact I'm talking about is the event that arguably defined zero time for the evolution of the Earth, meaning that there were several—probably—very big impacts, objects the size of the Moon or Mars, aggregating. And then after that event, which might have happened 50 million years after the formation of the solar system, everything was small [laughs]. But small doesn't mean no global effect. I have to admit, when I said global in consequence before, I was really thinking of it in the geologic sense, of melt all over the place and so forth. The reality is that even the ten-kilometer impact affected all of Earth's atmosphere and all of Earth's ocean. But geologically speaking, in the sense of a crater, a hole in the ground, and the immediate proximal consequences, it was kind of local. It was the Yucatan Peninsula.
ZIERLER: Dave, between the administrative side and the science, this has been a decidedly Earth-based conversation. So for next time, we can pick up on the Galileo mission and your work on Europa.
[End of recording]
ZIERLER: This is David Zierler, Director of the Caltech Heritage Project. It is August 23rd, 2021. I'm delighted to be back with Professor David J. Stevenson. Dave, once again, thank you so much for joining me.
STEVENSON: Thank you.
ZIERLER: Today I'd like to focus at the outset on some scientific research you did in the mid/late 1990s, but as a bookend to that, just out of curiosity, your election to the Royal Society was in 1993. What was your status? Was that an external member, or how does that work?
STEVENSON: I am a citizen of New Zealand, and New Zealand is part of the group of countries for which you have eligibility to be in the Royal Society. That applies to essentially what used to be the British empire. It includes, for example, people from India, even though India at one point was a republic. I don't remember exactly how they're treated, but in any event, they're eligible. People who are U.S. citizens by and large are not eligible, although of course there are some, including colleagues I know, who are citizens of both. In other words, they're both U.S. citizens and—for example, Shri Kulkarni is an Indian citizen, and therefore eligible.
ZIERLER: And your election to the National Academy of Sciences here in the U.S., this membership type, if I understand correctly, is as an international member?
STEVENSON: Ah, so that is different, indeed, because I chose never to become a U.S. citizen. I am told, although this is anecdotal, that if I had decided to become a U.S. citizen at an earlier point, then there are people who would have urged that I become a member of the National Academy. But because I never became a U.S. citizen, it was delayed through the fact that membership as a foreign member or whatever you want to call it is harder if you reside in the United States. So actually the path I took to end up in the National Academy was a more difficult one.
ZIERLER: Now nomenclature aside, as a native New Zealander but as somebody who has made a career and a life for yourself in the United States, how would you compare and contrast the honor of being elected both to the National Academy and the Royal Society, in terms of your identity?
STEVENSON: Well, I don't actually spend much time thinking about that. I've been involved in both, in the sense of making recommendations about people who should become members, especially for the Royal Society, which has involved trips to London where I have served on a committee. My impression is that getting into the Royal Society is actually easier than getting into the National Academy, simply because the scientific community in the United States is so big, and at least in areas similar to what I do, substantially bigger than the pool of people that are eligible for the Royal Society. It's also true, and I have been told this by other Fellows, that the Royal Society has a peculiar aspect, which is that it tends to be heavily focused on the U.K., and especially on Cambridge and Oxford. So it still has somewhat of a reputation of being non-representative. And as a consequence, if you're like me, from a faraway country—New Zealand is about as far away as you can get, from London; it's the antipode, essentially—if you're from a faraway country, you're less likely to be admitted. So in that sense, it was certainly an honor.
ZIERLER: Now back to the science, I'm curious as the Galileo mission was being developed, were you involved at all? Were you aware of what was going on at JPL at the early days?
STEVENSON: Not much. My science in those days was very much divorced from missions. I paid attention to what people were doing, but I was not involved in the decisions that were made about what would be measured and where the spacecraft would go. As I've mentioned previously, in a strange way, I was involved way back in the 1970s, in decisions or opinions that affected the nature of the mission, but only in the highest-level sense of deciding between a mission like Galileo and a completely different kind of mission, which would have focused on magnetospheres. But once Galileo got developed, it was of course delayed, and the specifics of the instruments and so forth, which was actually done in the 1980s, those things happened without any participation from me.
Galileo and Magnetic Fields
ZIERLER: If you can explain the science a little bit, what was it about the Galileo spacecraft that allowed you to think about induced magnetic fields regarding the possibility of there being subsurface oceans in Europa and Callisto?
STEVENSON: It's difficult to reconstruct now how I came to that issue. Certainly I was aware of work in the 1970s where people had done something similar for Earth's Moon. And a lot of people don't know this, but the main argument still for the deep Moon being hot, in those days, certainly, was the induction. Earth's Moon experiences a time-variable magnetic field because of the presence of the Sun and the magnetotail of the Earth. The Moon in orbit around the Earth feels the change in field as it comes into the shadow of the Earth—that is, in the same line as Earth, Moon, and Sun, but the Earth between the Moon and the Sun. Doesn't have to happen exactly, because the tail is quite broad. Still, that means that the field varies at the surface of the Moon, and this induces eddy currents inside the Moon. And those currents could only exist, it turns out, because of the high temperatures inside.
There are other arguments, and I'm aware of those as well, for why the center of the Moon is thought to be hot, and those arguments have to do with the presence of a liquid core. That's a completely separate argument. It's a geodetic argument. It's not an electromagnetic argument. Anyway, this background of knowing the Earth-Moon system led naturally to thinking about the possibility of electromagnetic induction in the satellites. But I can't recall now how I came to pay attention to the data from the Galileo mission, except to be aware that it was published and not explained before I got involved in trying to explain it.
ZIERLER: Now some 25 years later, what do we know about the existence of an ocean on Europa?
STEVENSON: We don't have more data. I think the argument is as strong or as weak, depending on your point of view, as it was back then. When I refer to weakness, I'm getting at the difficulty of characterizing the space near the satellite, because of course it's not a vacuum. There is plasma, and so there can be currents externally. You do have to worry about the fact that there is a complicated electrical conductivity of the surrounding plasma which affects the response of the body to the field. Still, it is true that in a quite remarkable way, evident just with pencil and paper, not through some complicated obscure analysis, that you can find a diamagnetic response for Europa, which indicates an internal conductor. Then comes the question of what that conductor should be. And the only realistic choice is a salty ocean. It can't be an iron core, because that's too far down, and the response would be too weak. In principle, it could be a sheet of mildly conducting material that is continuous around the entire body, for example graphite, which is not a very good conductor, but good enough. But that doesn't make geochemical, cosmochemical sense. So a salty ocean was really the only thing remaining, and something like Earth's ocean was plausible. It doesn't have to be sodium chloride dissolved in the water. Anything that produces ions. It does have to be a liquid. You can't do it just with frozen salty water, so it had to be an ocean. And that was the argument.
At the time I looked at these data, I didn't even think about Callisto. I was mildly surprised when my colleagues at UCLA told me that they saw the same effect at Callisto. In fact, the effect at Callisto is easier to see in the data, simply because Callisto is further out, and the plasma environment is less complicated. Callisto is still within the magnetosphere of Jupiter. That's important. The magnetosphere of Jupiter is very, very large, and it embraces all of the Galilean satellites. At that point, there was no argument being put forward for Io. The data were too complicated. And Ganymede was a different story. Because in the case of Ganymede, one saw evidence for a dynamo, for an Earthlike field coming from the core of Ganymede, despite its small core. Indeed, Ganymede probably has an ocean, as does Callisto, but it was harder to see that in the data. Again, that was something that people at UCLA worked on. I did not pay attention to it. I only looked at the question of Europa.
ZIERLER: It's notable that there really isn't any more data from the mid to late 1990s, as you mentioned. What projects, observationally, telescopically, that are being planned now, or people are talking about, that might yield some more solid evidence in this regard?
STEVENSON: The important thing is the Europa Clipper. This is a mission that will be launched in 2024, with the help of SpaceX. That's a recent decision. That mission is actually devoted in large part to measurements that help people understand whether Europa can support life, whether it is habitable. Habitability is the catchphrase these days. But the mission does include a magnetometer. Remarkably, the person in charge of that instrument now is Margie Kivelson, who is 90 years old, and she is at UCLA, and she is the person who led the Galileo group. So somewhat amazingly, after more than a quarter century, we have essentially the same people doing a better measurement. Even though it's not in orbit, it will be a better measurement than what we previously obtained. In fact, it will be so good that they may even be able to tell us something about the thickness of the ice and about the thickness of the ocean.
The advance over the Galileo data is in part the fact that they will collect enough data through multiple passes that they can separate the part of the signal that has to do with the spin period of Jupiter, which changes the field as Jupiter spins out the surface of the top, so to speak. The axis of the magnetic field is aligned to the rotation axis, so as Jupiter spins, that magnetic pole creates a surface that is like the surface of a cone. In addition to that, they will get the effect arising from the eccentricity of the orbit of Europa. Even if Jupiter's field were not tilted, there would be a change in magnetic field at the orbit of Europa simply because the distance between Europa and Jupiter changes with time. That's a much smaller effect, a few percent effect, but they will see that. So in a few years from now, we will have better data.
ZIERLER: Dave, one discussion, one article I'd like to discuss in some detail, is in 1999, you published in Nature "Life Sustaining Planets in Interstellar Space." The first thing obviously that's notable there would be your postulation that planets exist in interstellar space. Now, would that be an unorthodox view, if you'll permit me some very amateur logic here, that planets need stars, and by definition interstellar space lacks stars? So would that be the first interesting thing here, the fact that there could be planets in large areas of space that lack stars?
STEVENSON: That part is actually not new, though it's certainly true that it had received rather little attention. I do have what many people would consider a somewhat unorthodox view about the definition of planets. In fact, I get a little bit annoyed when people make such an issue, such a song and dance, about our solar system having eight planets, for example. To me, a planetary body is an object of sufficient mass so that its inside is different from the outside, maybe even of sufficient mass to have an atmosphere and so forth. And those attributes have nothing whatsoever to do with the question of whether the body is in orbit around a star.
The amusing thing about this whole business is that the word "planet" comes from the Greek for wanderer. For the ancients on Earth, it was natural to think of planets in some sense as wanderers, because they looked up into the sky, and they saw what appeared to be the fixed stars in the sky. Of course, we now know the Earth is rotating, and so the variation through the night, which they might have had a different notion about, is now thought of as just being the rotation of the stellar sphere, if you will, due to Earth rotation. But they saw the stars as fixed to each other. The planets were not. The planets moved across the sky with changing positions relative to distant stars. So that's why they were called planets, because they were wanderers.
This is ironic, because of course we now know it's the stars that are the wanderers, and the eight planets that people use for the word planet are in fact in lock-step rotational motion around the sun, and they're forced to follow paths that are very, very precisely defined. They change very little. In fact that's exactly why Kepler was able to figure out Kepler's laws, because the planets don't change much in their orbits. The orbits don't change much around the sun.
To get to the key issue here, the existence of bodies of substantial mass in interstellar space is readily explained once you realize that bodies like that might form in a disk around a newly forming star and then get ejected by passing by a planet that was sufficiently massive. And as I said, this is not a new idea, because we have spacecraft leaving the solar system right now. The Pioneers and the Voyagers are leaving the solar system. Why? Because of Jupiter. Jupiter is not the only planet that can do this. By the way, the Earth cannot do it. The Earth is too close to the sun. And the escape velocity from the Earth, which is a measure of how much gravitational scattering it can do, is smaller than the orbital velocity of Earth. For Jupiter, the inequality is in the opposite sense, meaning the escape velocity from Jupiter is larger than its orbital velocity, so Jupiter can eject objects from the solar system. During the formation of the planets, you could have had something that got close to Jupiter and got ejected. So that's the basis of the argument for interstellar planets.
I have to say that at that time, we didn't know about other planetary systems, and so you looked to the solar system as a guide, and that may not be correct. If you look at the planetary systems that we know about now, of which there are many, it's actually not clear how good they are at doing the same thing. But it is clear that in the formation of planets, as we currently understand it, you can get situations where ejection takes place. It's just gravitational scattering, what you would call the gravitational slingshot effect. It's the same process that is used to get spacecraft to distant locations. We still use it. And the effect can be large enough to eject something.
ZIERLER: Dave, if we can pull a little further on this idea that it's actually the stars that are wanderers and not the planets that orbit them, are there any fixed points or reference points in space, in the universe, that make stars not actual true wanderers?
STEVENSON: Well, [laughs] I was using the term "wanderer" in a slightly loose sense, because of course our Sun is in orbit around the center of the galaxy, and the center of the galaxy we think is quite massive. The orbital period is 200 million years. The sun is also interacting with nearby stars, and so it has a somewhat variable orbit. That is, there is a random component of motion superimposed on the orbital motion.
So the universe operates at several different scales depending on what you're talking about. If you want to talk about the motion of stars in the Milky Way, then the natural frame of reference is to think about the center of the galaxy, and to talk about the motion of the stars relative to that. The sense in which you have wandering is this: that if you could imagine looking at nearby stars a few hundred million years ago, even recent relative to the age of the solar system, the sky would have looked different for the nearby stars. They would have been in different positions. Because they do in fact differentially move with respect to each other. Of course part of that could be just the orbital motion. But in fact, nearby stars do wander relative to each other. So in that sense, they are wanderers.
As for the galaxy as a whole, there is of course a frame of reference that you might talk about that is sort of tied to the local environment. If you go to sufficiently large length scales, then of course you can talk about expansion of the universe, as cosmologists do. But there isn't really any fixed point.
ZIERLER: Did the recent discovery that there is a black hole at the center of our galaxy—to what extent did that advance our understanding of how stars move?
STEVENSON: I don't think that changed things very much. This is a little bit outside my area, so I don't actually know the history of the subject. [laughs] Although I know the person who is often associated with some of the ideas for the center of the galaxy, and that's Andrea Ghez. I think that we knew quite a long time ago about the existence of a central concentration of mass. We also knew, actually, that our galaxy, the Milky Way, extends spatially well beyond the region that we can define just by looking at visible stars in the sky. This is called the missing mass problem, and it shows up in the rotation curve. The person responsible for that, by the way, is no longer alive, a very famous scientist. That's Vera Rubin, who for many years was at the Department of Terrestrial Magnetism in Washington DC.
Life Sustaining Planets Beyond Earth
ZIERLER: Now the second part of the Nature paper, which is almost as intriguing, if not more, than the first—your focus on the possibility that these planets in interstellar space could be life-sustaining. What was the basis for that proposition?
STEVENSON: So, as often happens in things that I do in science, it's difficult to reconstruct exactly how I came to thinking about this. But I was certainly aware that a sufficiently massive body made of rock or ice would attract gas, gravitationally, from the nebula in which it formed. That kind of work had been done already back in the 1970s and 1980s. I wrote about it in the early 1980s in connection with ideas about critical masses for making a planet such as Jupiter. So I knew already that if you by any means made a rocky body of order on Earth mass, in the presence of hydrogen, that you would attract to that body a significant mass of hydrogen, maybe 1%. Even less than 1% is interesting. But to give a number, 1%. And one percent of the mass of a body like Jupiter is actually a lot of hydrogen, from the point of view of the ability of that gas to allow heat to escape. And so even 0.1% but certainly 1% of hydrogen around an Earth-like body would create a blanket which in some respects operates like a greenhouse. To be clear, what I mean by that is that if there is heat coming from below, it has difficulty in escaping, and as a consequence, the atmosphere approaches what we call an adiabatic state, which is the state that you have to reach in order to have convection. And that means the temperature increasing with depth to a substantial degree, so that if the planet is radiating to space at a low temperature, like say 30 or 40 Kelvin, which is a perfectly reasonable number just based on radioactivity alone, an unavoidable heat source, that even at that temperature, as you went down into the atmosphere, the temperature would eventually rise, and so you could reach a surface temperature that could easily be 300 Kelvin.
300 Kelvin is the temperature we live at. It's a temperature at which you can have liquid water, and therefore presumably life, since there are energy sources, albeit not the ones that we rely on here on Earth. We rely on sunlight. These interstellar planets would rely on a much less effective energy source, but one that we believe still works, namely radioactivity.
ZIERLER: Dave, the term "life sustaining" – when does it mean investigating the possibility of whether life exists in a place like Mars or Galileo or interstellar planets, and when does it mean investigating whether we can colonize beyond Earth?
STEVENSON: Well, I wasn't attempting to address that nuance.
ZIERLER: I am specifically thinking of the fact that SpaceX is getting involved with the moons of Jupiter. I can't help but think that that must be part of the interest, in terms of discovering whether these bodies are life-sustaining or not.
STEVENSON: So, in our field, some of us at least try to make a distinction between situations where life could have begun, and situations where life, once placed there, could continue, using the local energy sources and so forth. But as I said, I wasn't attempting to answer that question directly in respect to interstellar planets. I still think actually that these are places where if life is easy enough, you could imagine life developing. To me, these are separate questions from the idea that you might colonize planets.
When I look at current technical capability, which is really what we're talking about, not the ability to have life continue once you place it there using natural ingredients, but when we talk about the technological challenge, which is really what's involved when you send a person to another planet, what I think is a true statement is that it's very difficult to do that. Possible, but very difficult. And I don't quite see why you would want to do it unless it's just a romantic thing. Obviously in 100 years, 200 years, who knows what's going to happen? I think we've talked about this before. I think that colonization of Mars right now does not make sense. And to me, it's a completely separate issue from whether there is life on Mars right now, or whether there was life in the past, or whether appropriate microbes sent to Mars could be self-sustaining. I don't know the answer to that.
ZIERLER: To get back to the issue that was your focus, is it possible that planets in interstellar space can sustain life, if we could just let our imaginations take over? In the absence of a sun around which these planets would orbit, what kind of life could exist there?
STEVENSON: I wish I knew. I'm adopting the simplistic view, perhaps naïve view, that it might be the case that all that is needed for life is an energy source, an appropriate host—liquid water, as an example—and perhaps you can under those circumstances have replication. You can have the development of complexity. I don't know of any fundamental reason why that is not possible. It is of course true that were you in such an environment, the energy budget would be substantially less, but I don't think we know what energy budget is required.
On Earth, we have this biosphere which relies in large part on photosynthesis, this amazing thing that plants do. And of course, human beings and animals exist in coexistence with the plants. We're parasitic on the plants. Once you are in an environment inside a planet, or an environment where there is no sunlight, you rely on energy sources that are surely smaller. The amount of sunlight that comes to the Earth is 5,000 times greater than the energy from inside the Earth. So that's a factor in favor of using sunlight, if you can. But that doesn't mean that life has to have sunlight. It is of course popular to suppose that that's attractive, and maybe that is so. We simply don't know.
ZIERLER: Would the life definitely have to be, or not necessarily, carbon-based, on a planet that lacks sunlight?
STEVENSON: I'm assuming that the most attractive choice is carbon, but I have an open mind about this. Maybe there are other ways of getting complexity developed, other ways of having replication, the things that we call life. But let's face it; [laughs] it may turn out to be something that we only choose to call life once we've seen it and understood it. And we are in the awkward position right now of having one and only one example of life around us, and basing our perceptions of life on that one example. And that might be a mistake.
There are of course many arguments that have been presented by others that favor carbon. It would seem to be an atom that can combine with other atoms in nature, other common atoms—hydrogen, nitrogen, phosphorus, whatever—to form complexes that are very suitable for sustaining complexity and self-replication and so forth. So maybe carbon is best. But we really don't know.
I think you're poking at something that I don't really claim to be an expert in, but I will say this: I am troubled that there are a lot of people who work in this area who think based on understanding life on Earth that they know what to look for in space. I think that's a mistake. I think that there's a very good chance that when we discover life elsewhere, we will be surprised. It will be different from what we thought we were looking for, just as was the case with exoplanets. When exoplanets were discovered elsewhere, they were not what we were looking for. They were different from our preconceptions. And indeed, our preconceptions were based on our solar system. We now suspect that our solar system is not so typical.
The history of science is like this. It's also a reason why one should avoid thinking of the scientific enterprise as top down. It should be bottom up. It should be individual excellence, people doing oddball things. People being individualistic. People looking for unexpected connections between things that were previously thought different. I'm a big fan of individual scientists doing their innovative and imaginative work, and I'm less fond of the idea of institutes and centers and organizations of various kind. I think there's a danger that science will do too much of that.
ZIERLER: If I can just editorialize, I would think that the very fact that we're not sure how to categorize viruses here on planet Earth, this would suggest to me we should be quite open minded about what life might look like beyond our planet.
STEVENSON: Yes, indeed. Of course, some people even argue about viruses. [laughs] I mean, there are people who wonder whether you should actually think of those when you think about life. But yes.
ZIERLER: [laughs] Now when you use the term "complexity," how far are you aiming? In other words, if we find microbes or single-cell bacteria or something like that on these interstellar planets, is that not necessarily as noteworthy as where you're taking your hopes and dreams? What might complexity look like? How would you define that, even getting beyond carbon or not carbon?
STEVENSON: Well, [laughs] you know it when you see it.
ZIERLER: [laughs]
STEVENSON: I simply don't know. It's not just a question of the size of the system. It has to be organized. There's another key idea, of course, when we talk about life, that I didn't mention and should have mentioned, and that's the idea of low entropy. Low entropy just simply means an organized structure. That means you have to do work to sustain it, and that means you have to have an energy source. I said that part of it. But it is tied to entropy. There are organized structures, for example the Great Red Spot. So the Great Red Spot on Jupiter is an organized structure. It's an interesting one, because we actually don't know how it formed, and we don't know why it's there, and we don't know why it persists, although of course there are lots and lots and lots of papers about it.
To come back to your question, I think the most exciting aspect of understanding the origin of life would be the identification of things that are a lot different from us. That's why I think that finding something on Mars that's similar to the Earth, exciting though that would be—and make no mistake about it, that's front page New York Times stuff—it's not as exciting as the stupendous discovery that you could imagine making on a body that is completely different from anything that we encounter on Earth, and finding a kind of life that's completely different from what we think about on Earth. Maybe that could happen at Europa. Maybe Europa is actually sort of like the Earth. You have an ocean. You may have energy sources at the bottom or something. Who knows? Or maybe you can find life in the clouds of Jupiter, or some other crazy thing. It's just a question of kicking over rocks and looking what's underneath.
ZIERLER: We'll stretch this concept as far as we possibly can. With all of the new missions that are in development right now, people are talking about looking not just for biosignatures, but for technosignatures. What might that suggest from your vantage point about what life might look like, even if we want to stretch this beyond the idea that life needs to be biological in nature as opposed to computational?
STEVENSON: Well, we should have an open mind about this. I'm in favor at some modest level with activities like SETI, which is the search for extraterrestrial intelligence. It has been around for a long time. I think having a diverse portfolio of science is a valuable thing. You don't put all your eggs in one basket. You look at a range of possibilities. So sure, I knew Frank Drake. Frank, I think, is still alive. I met him again about a decade ago. And of course, Carl Sagan was someone who pushed these ideas that one should be open to the possibility of something that has developed a lot.
I mentioned Frank Drake. Frank, of course, put forward an equation that describes the likelihood of finding an intelligent civilization. You were perhaps talking a little bit more broadly about something that might spontaneously develop based on silicon or whatever. I simply don't know. All that I would say about that is that I'm open to the possibility. As for something that is an extraterrestrial intelligence, Frank and others put forward a view that we still more or less subscribe to, although there is no consensus about what the numbers are. If you thought that a civilization like ours had a limited life span, then just because the age of the universe is so much greater, the probability of finding one nearby may be quite low. You could even argue that we're the only intelligent form of life in our galaxy. We simply don't know. That would arise, of course, if were somewhat improbable to develop intelligence, and then whatever you developed wiped itself out in a million years or whatever.
By the way, we don't have to wipe ourselves out. Despite all the fuss about being hit from things from outer space and the demise of the dinosaurs and all that, biology tells us that things go extinct all the time, and that's a perfectly normal process, and why should we think we're different? Maybe we can avoid that. But all of these things are just wild speculation. To be honest, I actually feel even a little bit uncomfortable talking about it, because I don't profess to be an expert on it. Of course, there are other people who profess to be experts who may not know anything [laughs] more.
ZIERLER: [laughs]
STEVENSON: It's a kind of mental masturbation.
ZIERLER: The larger takeaway here is that agnosticism has its place in planetary sciences.
STEVENSON: Sure.
ZIERLER: Moving to the turn of the new century, the 21st century, tell me about the broader questions that pushed you to write the proposal "A Mission to Earth's Core." What I'm really curious about is in the write-ups to this research, it uses the phrase "tongue in cheek." So let's talk first about what was the mission, and then what was tongue in cheek about it.
STEVENSON: [laughs] Well, that particular piece in Nature was triggered by a movie. The basic idea of sending a probe to Earth's core had been sitting in my mind for at least a decade prior to writing it up. But at some point, I was asked to comment on the script for a movie called The Core. There was a company in Sierra Madre, which interacts with producers in Hollywood, and they wanted me to comment on the scientific basis of the plot. This was at a point where the movie was largely complete.
I think the intent was that I would provide some quotable presumed positive [laughs] things about what was in the movie, about the plausibility of the storyline. It didn't actually work out that way, because what happened is when I got the script and read it, I realized that the science was poor, and I was disappointed in it, and somewhat annoyed with the concept. The movie did involve astronauts in a vehicle, getting down to the Earth's core to restart Earth's magnetic field, which had stopped and thereby created—problems [laughs]—at the Earth's surface. I have to confess that I've never actually seen the movie in its final form. I have difficulty watching something that is so bad.
ZIERLER: [laughs]
STEVENSON: Anyway, upon reading this script and being aware that the movie might come out within a few months—it was actually delayed somewhat—I decided, "Well, it's timely to write up my idea about how you would actually go to the core." Not with people, but with an instrument, with a set of instruments and a probe. And so on an afternoon—it only took a few hours—on December 31st of that year, I wrote it up, sent it off to Nature in time for it to arrive within that year [laughs]. Not that that matters much. It was reviewed actually by somebody I know, although I didn't discover that until later, and people liked it. It was to some extent a joke, because I felt and still feel that there is a mismatch between enthusiasm about going to space and enthusiasm about finding what's inside the Earth. And so, I made the point that there's as much interesting stuff beneath us as above us. The volumes are immensely different. But the inside of the Earth still has many mysteries.
ZIERLER: I wonder if part of that is the attraction of heaven and the resistance of going to hell.
STEVENSON: [laughs] Well! I never thought about it in those terms, which have a religious connotation. To be honest, that didn't really occur to me. I was only thinking about the scientific content of what's above us, and the scientific content of what's below us, and the value of understanding the inside of the Earth.
ZIERLER: But realistically, in terms of human travel, obviously we've demonstrated we can go into outer space. We cannot go to the center of the Earth.
STEVENSON: Correct. It's a lot harder. I made that point. It's very easy, relatively speaking—still actually hard, but relatively speaking, it's easy to go into space. Of course, once you're outside Earth's gravity field, given what we know about gravity, if you're moving fast enough—it doesn't have to be a lot faster, actually—you can even leave the solar system, or maybe you can go by Jupiter and get ejected. Why not? [laughs] Or you can use a laser to accelerate you. That idea has come up in recent years, usually though in thinking about something that of course has no humans. It's always a lot easier to do things if you're just trying to measure something using instruments, and you don't have to add all the huge cost that comes with keeping somebody alive.
Anyway, what I knew from my own experience was that the exploration of space had been driven in large part by the Cold War. I knew from my childhood experience how influential Sputnik was, how influential the fears about having something overhead which might drop a bomb on you—how those fears influenced the way people thought about nationalistic impulses. So I knew that even though we had shifted in large part to scientific exploration after the Apollo program, that a lot of the impetus had actually come from the Cold War, which was political and emotional. It didn't actually get driven by science. And so if one went back to the idea that the goal was to understand the science, then if you could go down into the Earth, that would be a valuable thing to do. It is [laughs] of course exceedingly difficult. So tongue in cheek, I fashioned a way to think about the problem that might work.
The important thing about what I was doing is that it wasn't fantasy. The elements of what I was proposing were actually close to being achievable if you put in an effort similar to the immense effort, many billions of dollars, that have gone into going to outer space. So part of the scientific background, probably the most important of all, is that a decade earlier, I had worked on the formation of Earth's core, and I knew from that work that people had studied how cracks propagate inside the Earth, and how fluid can move downward in those cracks. One of the ideas for forming the Earth's core, which I don't think is actually the most important, but nonetheless existed in the literature—one of the ideas was indeed liquid-filled cracks in which the liquid was heavier than the rock, and then you could use gravity. We had iron at our surface, which would like to be at the center of the Earth, if you want to make the gravitational energy as low as possible. And so, if you could accumulate enough of that iron, many tens of Eiffel towers worth, and put them into a crack, then that crack would self-propagate.
ZIERLER: Because of magnetism?
STEVENSON: Just because of gravity. And of course, there are all sorts of technical problems. Just like with the Manhattan Project. It's easy to talk about setting up a plutonium device, but actually there are all sorts of technical problems. The same is true with a mission to the Earth's core. I had no illusions about this. There were some people who took it seriously, some prominent scientists. I remember talking to one of them once. He was very enthused about the idea. Just to go a modest distance. Not to the core. Just go down 100 kilometers. Why not? [laughs] Maybe you would start with that. So I'm not saying it's completely ridiculous. Not at all. But I do recognize that it's somewhat extreme. And certainly, it was written in the spirit of provoking people to realize that the enthusiasm for going into space had reasons other than purely scientific ones, and if you thought science was important, you should also be thinking about going down.
The Mysteries of the Internal Structure of Earth
ZIERLER: When you talk about liquid coming through the cracks, is this to say that to the best of our knowledge, once you get beyond the Earth's crust, that an iron probe would not be impeded by solid material? That there would be essentially a viscous travel route?
STEVENSON: The fact that it's solid is not a problem. What is important is that it has finite strength. So this idea was not new, and we know it works. Although I will admit that the sense in which we know is different from the application I had in mind. So here's the sense in which we know. We know that if you place liquid water in a pool, on top of solid ice, water ice, it can spontaneously propagate downwards to the base of the ice sheet. This is observed. There are actually photographs on the web of this. This is observed in Antarctica, and it's observed in Greenland. There have been instances, because of the increase in the tendency to form liquid water at the surface of the ice sheet of Greenland, because of global warming, most noticeable in the Arctic regions, there have been instances of pools of water that have spontaneously propagated down. There have even been crazy people who went in boats on these lakes on the top of ice. They should have had more sense. I don't think anyone has been killed by this. But once it happens, it happens fast.
It is possible because of the finite fracture toughness—that's the parameter we talk about in this context—the finite fracture toughness of the rock or of the ice. Obviously with rock, the toughness, the strength of the material is higher than with ice, but they're both solids. You don't have to rely on any viscous properties. And the energy of course comes from gravity, in the case of water, because it's denser, has a higher specific gravity than ice. And in the case of rock, it's because the iron has greater density. In that case, a factor of two. So it actually works just fine.
I don't doubt that it works in principle. As I said, there's a huge difference between saying that something works in principle, and actually doing it in practice. I'm not advocating that we do it in practice. After I published this idea, I was actually invited by the royal family in United Arab Emirates to go out there. I visited Abu Dhabi. And people were joking—well, maybe they would give me billions of dollars to do this. But actually, I wasn't really that serious.
ZIERLER: Dave, tongue in cheek or not, what role did nuclear weapons play in this imaginative proposal?
STEVENSON: It played the role of a mistake, meaning that I made the mistake of advocating that we use nuclear weapons, and I should have had more sense, because that gets people annoyed. [laughs] And it's really not necessary. There is of course a history of thinking about nuclear weapons in the context of doing things that are peaceful, in quotation marks. The Russians were big into this, using nuclear weapons to make dams or whatever they wanted to do, usually, but not always, a long way away from where people lived. But in modern society, nuclear weapons of course have a connotation that gets people alarmed very quickly. I should never have done that. I should have just simply pointed out that it's desirable to make a crack rather quickly, and perhaps we could do that with conventional explosives. It is probably true.
I should say that in my opinion, the anxiety that people have about nuclear power, which is a closely related issue actually, is disproportionate to the risk. People should be very concerned about the huge number of people who are being killed in coal mines and other activities that have nothing to do with nuclear power. But the word "nuclear" just leads to an emotional response. That of course is also why the word "nuclear" is not used when people talk about MRI.
ZIERLER: Dave, a question we've talked about before, and one you've alluded to just now, is in a probe to the Earth's core, the value from simply the basic science aspect, which is the world that you inhabit primarily, what about some of the possible applications, in terms of capturing energy, in terms of extracting resources? Simply by going to the Earth's center or as close as we can get to it, what might be some of the human values we can extract from it?
STEVENSON: My guess is rather little, but I would say the same about going into space. It is of course true that if you had plenty of energy available—and the sun is good at doing that, solar energy—then extracting material near Earth's surface might be a smart move. A lot of the interest right now and things that have technological value like lithium and cobalt, some of the heavy rare Earth elements, which go into high technology devices, could possibly benefit by having energy sources in the near future that you would use to go down beneath Earth's surface.
But I don't think about going really deep like the Earth's core as having any material benefit, just as I don't think about going to near-Earth asteroids as having material benefit. Maybe the Earth asteroids have more benefit, actually, than going down deep into the Earth. I don't know. Just because it might be easier to go out there, and maybe Elon Musk will figure out how to do it. But no, it's just basic science. I don't feel the need to make an argument for practical benefits for the same reason that I don't think people should feel the need to say that the Mona Lisa by Leonardo da Vinci has to have a material benefit. It doesn't.
ZIERLER: Coming on 20 years, when you first published in Nature, what work if any has been done on possibly making this a reality?
STEVENSON: None. That doesn't bother me. I suppose it's possible that at some future date, it will get revived in some form, probably in a more practical, less ridiculous form. I simply don't know. What is known is that drilling is really hard. People devoted time to drilling. It was done in Germany and in Russia, or perhaps it was the Soviet Union. It's difficult to go down more than ten kilometers or so. There are still good scientific arguments for finding out what is beneath Earth's surface. And maybe that's how the subject will evolve, by first doing things that we already know how to do. Drilling technology, of course, is as natural thing to think about in the context of finding natural resources, more prominently finding oil and gas. What's going to happen on Earth, surely, is that even though we had this bonanza of natural gas recently, the world is going to run out of fossil feels, and we may have to dig deeper, or figure out how to use solar energy more efficiently. But, I'm not worried.
ZIERLER: On the question of being worried, is there any concern that if drilling technology does get better so that it can go significantly deeper, that this would create some unforeseen catastrophic scenario?
STEVENSON: I don't know of any. I'm not so concerned about that.
ZIERLER: Like a super volcano, or something like that.
STEVENSON: Oh. Well, no, I don't really see that as an issue. Although it's certainly true that there are a lot of geophysical phenomena that have this property that the biggest events are very infrequent. The technical word for this is one upon f-noise. And so yes, there could be volcanic eruptions even bigger than the ones that have happened during the existence of human civilization. I suppose that's possible. But I don't see that as being connected to something that we do. If it happens, it happens.
ZIERLER: Dave, in thinking about your work or your inspiration from Alessandro Morbidelli, on Earth-Moon similarities, what's the reason for the focus on oxygen?
STEVENSON: [laughs] The Moon story is a curious one. I think I mentioned previously that I had become involved in the question of the origin of the Moon in the 1970s, picked it up again in the 1980s, even somewhat prominently, although other people chose to work on it more than me, and in fact I stepped out of the limelight in thinking about the origin of the Moon. But then one day, I was sitting in my office at Caltech and Alessandro Morbidelli, who everybody calls Morby came in. He's a very interactive person. Happened to be visiting Caltech. And he asked me about why it is that the oxygen isotopic character of the Earth and the Moon are so similar.
I need to be clear at this point about the particular thing that we look at, that everybody looks at. It has to do with the fact that oxygen has three stable isotopes, mass 16, 17, and 18, and there's a particular pattern. This pattern has been known about for a long time. But it has never been possible to understand why the Earth and the Moon are so similar. They should not be, if the Moon formed elsewhere. They should not even be so similar if the Moon had formed from material that had crashed into the Earth and went into orbit around the Earth. That would not necessarily work. So the puzzle was to explain why the Earth and the Moon are so similar. I think the puzzle is still there. There is now sufficient accuracy in the data, that you can see a slight difference between the Earth and the Moon. There are other isotopic signatures that people have looked at, that might even be more impressive. The bottom line is that I was triggered by Morby to think about the possibility that there was some kind of mixing process, equilibration, so that after the giant impact, which we all accept as the way the Moon formed—
ZIERLER: The giant impact from the Earth?
STEVENSON: A giant impact, being some body, perhaps the mass of Mars, hitting the Earth, leading to material in Earth orbit, which then coalesced to form the Moon. So that's what we mean by the giant impact story. That in that event, there was enough mixing, enough equilibration of the isotopic signature, that the material that went into making the moon ended up being the same as what is in the Earth. I think that story has serious problems, but that is what brought me back into the subject working with somebody who was then a student of mine, Kaveh Pahlevan. And so we came up with that story. It is not universally accepted, nor should it be. I think it's fair to say that we still don't really know what happened in the formation of our Moon.
There's a tension here, between two kinds of science. One kind of science is where you don't seek to understand specific accidents. Think of it like an automobile accident. You're at an intersection and two cars collide with each other, and you end up with a mess. You're a forensic scientist, and you come along and you look at the mess, and you say, "Oh, this person was going 40 miles an hour. This person was going 60, and he was slightly off the center." And so on and so forth. So you have some complicated story that's of interest. But that's different from talking about the generic impact, or talking about the generic interaction of automobiles going through intersections. There's the generic science, and there's the highly specific. They both have a place.
In the case of talking about Earth's moon formation, the difficulty lies in the fact that you hope to learn about these kinds of processes, but there's always a possibility that this is an oddball event. Until we look at other systems elsewhere in the universe, we're not going to know whether the Earth-Moon system is an oddball and we were spending too much time trying to understand an oddball, or whether it's a somewhat generic event, that there are giant impacts all over the place and it's a very common process. We simply don't know.
ZIERLER: To pull on this a little further, the focus on the similarities between the Earth and the Moon—so to any lay observer, that would be a preposterous observation. They're so very different. So what exactly are you referring to, in your focus on the Earth and Moon's similarities?
STEVENSON: Well, we're focusing on the idea that they formed together. That they are part of the same process, and that this is special to the Earth-Moon system. You can quickly get into technical details, because some people would say, "Well, okay, that just simply means that the Moon didn't form somewhere else." Most people don't like the capture story anyway. It's too hard to make capture work. But it's more profound than that. Because it does turn out—this is work that goes back a long way—it does turn out that for many impacts, the material that ends up in orbit tends to come from the projectile that hit the Earth. And that's where the puzzle arose. Because then you would say that the Moon should have retained memory of the projectile that hit the Earth, and the projectile that hit the Earth should be different from Earth itself, just as Mars is different from the Earth.
I should have made it clear that this is all in the context of what we know about stuff elsewhere. We know about oxygen isotopes in the Sun. We know about oxygen isotopes in meteorites. We know about oxygen isotopes in Mars. And then we have the Earth and the Moon, essentially indistinguishable, almost indistinguishable. And that's the puzzle. By the way, the missing piece in this story—and I've made this point several times—the missing piece is Venus. We do not know Venus. If it turns out Venus is like Earth, I think that might change people's perspective, because then they would come to think that the Earth-Moon similarity is perhaps not so mysterious after all.
ZIERLER: What would it take to develop that understanding of Venus?
STEVENSON: Measure. You want to measure the oxygen isotopes on Venus. That's not so easy to do. The mass 17 isotope of oxygen is much less abundant. You need very high precision instruments. But in principle, you could do it. Maybe even in the atmosphere of Venus, which is mostly carbon dioxide. The oxygen in the carbon dioxide might already be a clue. Of course, you would like to look at the rocks as well. Venus, of course, is coming back into focus. NASA has in all likelihood future missions to Venus. I think that's wonderful. We ignored Venus for a quarter century. That's a travesty. But people get all excited about Mars and possibility of life on Mars, so they forget about the planet that most of the time is closer.
ZIERLER: I'm curious if you can explain a little more how the original ideas from Morby led to your work with Kaveh Pahlevan.
STEVENSON: Morby was seeking from me a suggestion about what could have happened in the impact event that led to the mixing. I had already worked a bit on what happens in the impact and published on it, so that's why he posed the problem to me. As sometimes happens in science, the original thinking about how you might solve the problem only took a few hours. I can remember that very week, within a few days of his visit, that I was presenting my ideas to a group of people here at the building at an informal seminar. So it was somewhat spontaneous. If you're asking me to define how one comes up with scientific ideas, I don't know how to do that. That's a creative process, and it happens spontaneously. And what can I say?
ZIERLER: One question of simple translation—I'll need one entirely for the title of your work with Konstantin Batygin, Inflating Hot Jupiters with Ohmic Dissipation. Let's start first with inflating hot Jupiters. Why the plural? Why Jupiters?
STEVENSON: Ah! Around about that time, or a few years before, people had started collecting information from exoplanetary systems about the radii of those planets. The earliest discoveries were not the most common, as we now know, but they were in fact hot Jupiters. Hot Jupiter means a planet that you think has a similar composition to Jupiter, but is sufficiently close to a central star that its atmosphere has to be 1,000 or 1,5000 or even 2,000 Kelvin. By comparison, Jupiter itself is way down at 150 Kelvin. It's much colder, because it's a long way from the sun. These are Jupiters that are close in.
That was already, to many people, somewhat of a surprise. But then came the surprise that these planets had a substantially larger radius. And why is that a surprise? It's a surprise because you should not really be guided by the temperature of the atmosphere. You should think about the interior. And in our normal way of thinking about Jupiter-like bodies, the interior is set by the ability of the planet to lose heat from the interior to the atmosphere. The fact that the atmosphere is hot doesn't prevent that from happening, it turns out. And so you expect the deep interior, in the absence of an additional energy source, to actually cool off into a degenerate state. Degenerate simply means that the pressure that comes from gravity is supported by the Fermi gas. Fermi gas is effectively a low-temperature electron gas that comes from the pressure ionization of the material, in this case hydrogen.
This harks back to my earliest work, actually, because I knew from my earliest work that Jupiter was the largest cold object in the universe, more or less. I used to say this. And so you shouldn't get something that's bigger than Jupiter, if it is a cold object. That's the catch. So it was somewhat surprising. Before Konstantin and I got involved in this, there had been work done on the thermal evolution of these kinds of planets by a variety of people, and they had failed to explain why the radius was higher, although in doing those calculations, they recognized already that if you had an additional energy source—not in the atmosphere, so it can't just be radiation from a star, but somewhat deeper; exactly how deep is not clear, but it had to be somewhat deeper so that you were in the convective region—if you had that deep energy source, then you could keep the planet hot and prevent it from cooling off, and prevent it from contracting, and then you could explain the inflation, if you will. The word "inflation" is slightly misleading because what it really means is that the planet never got to contract down to a Jupiter-like radius. So it's a failure to contract rather than some process that actually inflated it. That's the background. Maybe I've answered the first part. Did you want me to also talk about the actual idea that we came up with? That's a separate story.
ZIERLER: Yes, planetary science.
STEVENSON: At that time, actually a couple of years earlier, I had worked with a student, Junjun Liu and with Peter Goldreich on the question of whether the winds that we observe in the giant planets, for example Jupiter, interact with the magnetic field deep down. Actually, I had worked on this decades earlier, but this particular project led to the recognition that the winds had to be truncated at, we thought, about 3,000 kilometers depth in the case of Jupiter. I have to say, by the way, that Peter Goldreich was indispensable to this project. Even though the ideas were not new to me, he organized [laughs] the research of the student so that it became successful. It's a great credit to him. It's actually the only project where I had substantial sustained interaction with Peter Goldreich.
I'm bringing that up because the same idea, more or less, came into the story that was developed with Konstantin. When Konstantin arrived at Caltech as a new student, he wandered into my office seeking suggestions about a project to do. I mentioned a couple of them, one of which was this one, on ohmic dissipation, and the other was on Mercury. He sensibly chose the one about ohmic dissipation, and went away, and very effectively determined the important thing, which is that these hot Jupiters have strong winds. That was already known. But because of those strong winds, and because of the likelihood that these planets had magnetic fields, you would create electromotive forces (emf) and therefore currents deeper within the body, deeper than the part of the atmosphere that is nearly isothermal, and so that would act as an energy source.
This is actually the same idea as what went into the previous work with Jupiter itself. Because the previous work with Jupiter was based on the idea that you could never have so much Ohmic dissipation that it would be far greater than the energy coming out of the planet. So in both cases, it was really an Ohmic dissipation story. I should explain that this is a bit of jargon that is not understood by everybody. When I say Ohmic dissipation, I really just mean that in accordance with Faraday, if I have a flow, and the flow is crossing an electric field, and the material is a conductor, then you make an electromotive force (emf). Think of it as just a voltage, like a battery. And when you have a battery, and you have a continuous medium, you have an electrical current. And when you have an electrical current, you have heat being produced. Same thing happens in an electrical circuit. The same thing happens when you turn on a light bulb in your kitchen. Energy is flowing through a resister. You get what's called I squared R Ohmic heating, or call it Joule heating, same thing. And there are bounds on how much of that can be present.
In the case of what I did with Konstantin, the essential energetics was that you used the small amount of energy that came from the central star to drive the winds—that's a well-known physical effect—which in turn created the Ohmic heating, the Ohmic dissipation in the interior. So essentially it was using solar energy to heat the inside of the planet. And you might say, "Well, why not just use the sun directly?" You can't. Because the sunlight doesn't get there. So this is a way of using sunlight, star light—it's not the sun, of course—it's a way of using star light to provide an energy source to something at a level much deeper than the place where the star light impinges.
ZIERLER: I'm curious the extent to which you were following the developments of LIGO at Caltech. I know you referenced LIGO in your thought experiment about the probe to the Earth's core. Were you in regular contact with colleagues like Barry Barish and Kip Thorne? More broadly, what was it like when the Nobel was announced?
STEVENSON: I think I was a little bit remote from those things, but to answer your question, yes, I was aware of what was going on. I was aware that LIGO was a little bit controversial. When I was division chair, I became more aware of it, because there were these arguments and concerns about the person who was running the project. His name is Robbie Vogt. Of course, at that point, there was a scientist involved in the project called Ron Drever. I knew Ron, saw him on occasion. His office was actually immediately adjacent to the area that we used for Physics 11 classes. I saw him almost every week. I didn't know him that much personally, but I knew about his background in developing some of the high technology ideas. So I was aware of the conflicts. I was aware of the gossip about what was going on, and even through being division chair and involved in discussions with the provost at that time, Paul Jennings, I was aware of the conflicts and the attempts to resolve those conflicts.
I don't really want to say a lot more about that, because it's all secondhand, and so I don't know through personal experience how much of it is actually true. The only thing I can say from personal experience is that it created a lot of controversy. I did know, through efforts after I was division chair, about conflicts between Barry Barish and Robbie Vogt, because I had to go talk to both of them. I was instructed to do so by the provost at that time, Paul Jennings. So I was sort of acting as an unofficial chair of some hearing committee. I don't remember specifically what was going on.
Caltech has this system where faculty can raise concerns about things that are going on, and so the provost has to designate somebody to find out what's going on, possibly set up a committee if there's a formal protest, and so forth. So I became aware through talking to Barry Barish, who had taken over from Robbie Vogt, of the conflicts between those two. Of course I knew about the extraordinary technical challenges of LIGO, and about what was being done. But I feel sufficiently remote from it that I am uncomfortable about saying more. Because anything more that I could say tends to be of a secondhand nature, and you just don't know whether it's true or not.
The eventual success was spectacular. Incidentally, I also taught one year a major part of the class that Kip Thorne was involved in, so I also had contact with Kip Thorne. I had other contacts with Kip Thorne. Enough said. [laughs]
ZIERLER: Dave, last question for today—tell me about your collaboration with the student Joe O'Rourke.
STEVENSON: I met Joe when he was an undergraduate at Yale, encouraged him to come to Caltech as an undergraduate researcher. We did a paper on Titan. But when he came to Caltech to be a graduate student, he worked on several things, as he still does. One of those was a project that I suggested to him, that like many of my projects, had been sitting around for years. I had realized a decade earlier, roughly, that there was an aspect of Earth's core that was imperfectly understood, and it came from laboratory measurements carried out in Japan. I can't remember off the top of my head exactly whose work I'm thinking of. Later it would be Kei Hirose, who is very active now. But to begin with, it may have been somebody else.
Anyway, I knew through that work, and also through interaction with a very distinguished geochemist no longer alive called Ted Ringwood—so this goes way back to the 1970s—I knew that there was this interesting question about the partitioning of elements between those that are primarily in the mantle, and those that are in the core. When we talk about Earth's core, we're talking about something that's primarily iron, But of course, we've known for decades that there are other things in there. The focus has been on light stuff—oxygen, sulfur, silicon—Which is not a lot lighter, actually [laughs] but silicon also perhaps goes into iron especially if it's not too oxidizing an environment. I knew that there was this interesting question, and it had occurred to me that one should think about something that we still don't really understand, which is the phase diagram that describes this multi-element system where one of the coexisting components is Earth's core, which is primarily metallic iron, and the other component is the Earth's mantle, which is primarily magnesium silicate, although of course it has oxides.
And so a natural question to ask in that situation is how hot do you have to go before the sharp distinction between those phases goes away. In thermodynamics, we call that the critical point. We still don't know what it is. My guess is that it's 11,000 degrees, but of course it will be pressure-dependent. Above that temperature, everything mixes. Below that temperature, there will be some mixing. And so there should be some magnesium in Earth's core, just as we know there is some iron in the Earth's mantle. In fact, there are things on the Earth's surface that are made in large part from iron. Stainless steel is mostly iron. And that's because iron partitions partly into the mantle and not entirely into the core.
So this is a chemistry question, and I realized that if you could put a small amount of stuff that was very different in density from iron into the core, that this could be an important energy source, since we had known for decades that the growth of Earth's inner core could act as an energy source for Earth's magnetic field. This is still the widely accepted theory. But perhaps in addition, perhaps earlier in Earth's history, you had another energy source that was material coming out of the core and being added to the mantle as the Earth's core cooled. So that's what led to the idea of magnesium precipitation.
I still think that some people misunderstand the idea. I have never said that it was specifically magnesium, only that magnesium is a control of what comes out. Presumably what comes out, and this is true in my own calculations, is actually a silicate, MgSiO3, although of course I don't really know. Perhaps there's material being added to the base of the mantle as the core cools, which is of a density similar to the mantle, which means it's only about half the density of the core. This is a very efficient energy source. You only need tens of kilometers or less of material added as a layer at the base of the mantle over geologic time, and that's enough energy to run Earth's magnetic field.
Can you test this? Well, that's really hard. It's a story that has the unfortunate feature that you can say it should happen, but you can't make a convincing argument for its existence. I should have mentioned one other very important thing, which may not be true anymore, but was part of the motivation for the work, and that is that people had decided at the time I pushed this project that Earth's core should cease to convect if it was only cooling without any gravitational energy source, if you were only using thermal convection, in other words. This argument hinges on the thermal conductivity of liquid iron, which is something that we don't know very well. But some people have made the suggestion that both the electrical and thermal conductivity was actually higher than people previously thought. This is a factor of two effect. In science, the kinds of science I do, you should be cautious about anything involving factors of two. [laughs] Because you could so easily be wrong at that level.
But it's an unfortunate fact, and probably true actually, that the Earth's core is near a dividing line, and the dividing line is between having a magnetic field and not having a magnetic field. I see evidence for this when I look at Venus, because Venus does not have one. I've been arguing for years—of course I don't know whether it's really true, but I've been arguing for years that the terrestrial planets like Earth and Venus, Mars even, although Mars is much less massive, that these planets are close to the dividing line, that it was a close call as to whether they ended up with a magnetic field and whether they did not. Of course, some people want to say this is important for life. I have no idea. Well, I have ideas, but [laughs] I don't know. That's different from Jupiter, Saturn, Uranus, Neptune. Those planets, you can easily understand why they have magnetic fields. So it's ironic but true that in the case of the Earth, if somebody asked, "Why does the Earth have a magnetic field and why does the geologic record suggest that Earth has always had a magnetic field?" then the honest answer to that question is, "I don't know."
ZIERLER: More broadly, what does this research tell us about how dynamic of an environment the Earth's inner core is? In other words, how much does it push against the assumption that the Earth's core formed with the formation of the planet itself, and has been relatively in stasis for the past four and a half or five billion years?
STEVENSON: I don't think we know the answer to that. Certainly, for a long time, and maybe even now, most people think of this core as being unimportant for understanding the thermal history of the planet as a whole. There was a time when people thought that the heat coming out of this core was a small fraction of the total energy budget of the Earth. It's not quite so clear anymore, so I don't think we know the answer. The core is key to understanding the magnetic field, but what I'm getting at here is that when you talk about everything else—when you talk about plate tectonics, when you talk about volcanism, when you talk about the heat flow coming out from inside the Earth—the standard view is to think of the core as a minor player in that story. We don't actually know that. It's probably true, actually, that it's a minor player, but to give you just one key example of the possible role of the core, it might be the case that hot spots, the prime example of which is the Hawaiian Islands and Hawaii itself, in the current day, that those are a consequence of heat coming from the core. That's a fairly popular idea. We don't know whether it's true.
ZIERLER: Dave, that's a great place to end it for today.
[End of recording]
ZIERLER: This is David Zierler, Director of the Caltech Heritage Project. It is August 27th, 2021. I'm so happy to be back with Professor David J. Stevenson. Dave, once again, it's good to see you.
STEVENSON: Good to see you.
ZIERLER: Dave, today I'd like to start with your involvement in the Juno mission. As a way to contrast your earlier comments regarding your relative aloofness or even disdain for previous space missions, between the fact that Juno harkened back to your dissertation and the level and time span of your involvement, it just begs the question, what made Juno different for you?
STEVENSON: I suppose it was the involvement of understanding the inside of Jupiter as a student, and to some extent later. From the late 1970s through 20 years later, I did not give a lot of thought to the giant planets, but was drawn back into it by the possibility of a mission to Jupiter. In some sense, I was a natural, since what people wanted to do was a mission that actually looked at Jupiter as a planet. In other words, what's inside, as distinct from the satellites. The Galileo mission had spent a lot of time looking at the satellites, as had Voyager. There had been less attention paid to what you might learn if you got in really close on multiple occasions. So I was a natural. I may have had disdain, to some extent, but actually somehow or other, I had become more involved in mission considerations, even before getting involved in the Juno mission. I was involved in an earlier idea for a Jupiter mission. I was involved in a possible mission to Mercury, because Bruce Murray had encouraged me to be a leader for that. Fortunately, that did not happen [laughs]. Somebody else did a good job on Mercury. But it was a natural evolution.
ZIERLER: If we could pull a little deeper, can you explain in what ways Juno was a natural progression from Galileo, and to what extent was it a brand-new mission all on its own terms?
STEVENSON: Oh, it was a brand-new mission, although what Galileo gave us was probe information. Galileo really had two parts. There was an orbiter that spent a lot of time looking at satellites, and did not in fact get good data for Jupiter itself. But, attached to the Galileo orbiter, there was a probe. Now, the probe dropped into the atmosphere. The probe did not succeed in doing something of great importance, which was to measure water. It saw a very small amount of water in one particular location. People were eager to know more. Water is important. Some people may think that's obvious, but actually viewed cosmochemically, water is important irrespective of its role in life and on Earth. It's important because it's the most abundant condensate in the universe. Water is partly oxygen, and oxygen is the third most abundant element in the universe. Hydrogen and helium are more abundant, but they don't condense. So that's why water is in fact the most abundant condensate despite being present in actually rather small amounts on Earth, a part per thousand, or a few parts per thousand. So Galileo set the stage for understanding more about Jupiter, and did not satisfy people's interest in what Jupiter was like. So you could say that Juno had some connection to Galileo, but actually it was a necessary thing to do irrespective of Galileo.
ZIERLER: What was the significance of the fact that Juno was solar powered, in contrast to the nuclear-powered aspect of Galileo?
STEVENSON: You're asking an aspect of how spacecraft are manufactured and designed that I'm not so familiar with. But the obvious observation to make is that in those days and perhaps even now, there is a limitation on the extent to which you can rely on an isotope of plutonium for your energy source. That is what Galileo did. That is what other missions to the other solar system did. But people knew that there were limits in how much of that isotope you had, and it was desirable to plan a mission that did not depend on that.
It comes down to how much energy you need to run the instruments that are important. Part of that is just getting the data back to Earth, but if you have large enough dishes on Earth, that's not too much of a problem. So you can have a rather low-powered spacecraft, which is what Juno is, in some sense, and do the science. It's a trade-off. You think about what science is important, which in this case was gravity, magnetic field, learning something about the atmosphere, and you realized you could do this with large solar panels. The solar panels on Juno, by the way, are very large. But you can do it, and of course it's the first spacecraft ever built to rely on solar power in the outer solar system.
ZIERLER: What were the circumstances of your earliest involvement, or even knowledge that this mission was in the works?
STEVENSON: Those are a big vague. I was involved from the earliest stages in alternative missions, which did not get funded. I should explain that NASA runs several kinds of missions. It runs missions that are called flagships, that are multibillion dollar missions these days. The rovers to Mars are an example, and Galileo was an example. Those missions are decided upon with the guidance of scientists at NASA headquarters. But NASA in addition runs competitions. It runs competitions for what are billion-dollar missions, one of which was Juno.
The earliest New Frontiers mission, by the way, was the new Horizons mission, as it's called, that went out past Pluto. That was actually the same class, the first of its kind. So those are billion-dollar missions. But Juno was actually in a competition for billion-dollar missions. Prior to that, I was involved in competitions for what are called discovery class missions, which are half-billion dollars, $500 million, and obviously are less capable missions. Several were proposed for Jupiter. Juno came out of that process as something that cost twice as much but actually was much more capable scientifically.
The decisions leading to the existence of these missions were reached without my involvement. I became involved when it reached the stage of writing a proposal and figuring out how well you could do the science. That would have been in the late 1990s or round about the year 2000. I don't remember exactly. I do remember going to a meeting in the Jet Propulsion Lab, a meeting in the director's office. This was a mission that was proposed through the Jet Propulsion Lab, through JPL, although the PI is now at Southwest Research Institute in San Antonio, Texas. JPL has a process of deciding which missions to support and involving scientists in thinking about the science that can be done. I was a natural to be involved just simply because of my background that includes trying to understand what goes on deep inside Jupiter. I was involved in writing the proposal, but not heavily so. I became much more extensively involved after we were accepted and funded, and then led a group called the Interiors Working Group which met many, many times in the subsequent two decades.
ZIERLER: Dave, more broadly, I wonder if you can talk about how the proximity, administratively and physically, to JPL, can be such an advantage for Caltech professors.
STEVENSON: I'm not sure that it makes a huge difference. It's certainly true that if you are on the faculty at Caltech campus it's a relatively easy thing to go and have a meeting at JPL. But nowadays, a lot of things are done online. A lot of things are done through telecons and so forth. So, it's actually not clear to me how important that is. Certainly, the ability to meet in person can help. People have greater awareness of what somebody is doing if they are in geographic proximity. But I could imagine, in principle, becoming involved in a mission that was done, for example, through APL, which is connected to Johns Hopkins, which is of course on the East Coast.
ZIERLER: Just as a counterfactual, if you had stayed at UCLA, would it have been as easy to be as closely involved with JPL?
STEVENSON: I think the answer is yes. There are people at UCLA who are involved in missions that run through JPL. They are slightly further away; it's not much fun driving from here to UCLA, or back, except at midnight, perhaps.
ZIERLER: [laughs]
STEVENSON: But no, I'm not sure if makes that much difference. Being prominent in some sense through the science that you've done certainly matters.
ZIERLER: Chronologically, if the earliest proposals and ideas were the late 1990s, is that to say that there was already data from Galileo that was useful in crafting the proposal?
STEVENSON: Yes. The results from Galileo focused people's attention on what we did not know. One of those key things was water. But of course, we also did not know enough about the gravity field or the magnetic field to develop our ideas about what was going on down in the deep interior of Jupiter. So Galileo informed us, made us better aware of the issues that needed to be answered. To be clear, the Juno mission and the smaller missions that preceded it were driven by an idea that all of us still subscribe to, which is that understanding Jupiter, understanding the planet Jupiter, is key to understanding our solar system. Because Jupiter is the most massive planet. We suspect it's the planet that formed first. Maybe that wasn't so obvious back then. It has become more likely in light of prior information that came along later. So the feeling was that you really had to understand how a planet like Jupiter formed in order to develop your ideas of the formation of everything else, which of course includes our home, the Earth.
ZIERLER: Of course, this brings us full circle to your dissertation. As a matter of intellectual history, as you were involved in the proposals in the late 1990s, early 2000, what were some of the unanswered questions, all the way back to your graduate school days, for which Juno possibly had answers?
STEVENSON: The most obvious one was water. How much water? That's not a clear-cut question. We knew—in fact I even knew as a student—that there was enrichment in some materials in the atmosphere of Jupiter that said that the atmosphere was different from the sun. Actually, when I was a student, that was really only known for ammonia, but already suspected for other things. So water was key.
Another one was just having a better understanding of the magnetic field. Where is the field actually being generated? And that's related to the electrical conductivity of the material within Jupiter, and to some extent the dynamics. Another one is the nature of the central region. Does Jupiter have a core? In our proposal, we were emphasizing these things. How much water? Does Jupiter have a well-defined core? Because that was in doubt at that time, and affects your ideas about how Jupiter may have formed. What's the nature of the magnetic field? Actually, the way the mission transpired, we ended up knowing a lot of important stuff about the atmosphere as well. That's ongoing.
ZIERLER: Dave, if you can take us behind the scenes, how do you convey these questions about what the scientific mission of Juno should be, to the scientists and engineers responsible for building the spacecraft and developing the instrumentation to answer these questions?
STEVENSON: The way it works is that the scientists provide input in their estimates for how accurately we need to measure something. We might, for example, say, "Well, you should try to measure the gravity field at a certain harmonic degree to one part in ten million." That turns out to be a relevant number. You can debate whether that number is right, but that's actually the process. The process is for a scientist to make some estimate of how accurately you want to measure something. Same is true for water. You want to measure it to actually a fairly poor accuracy in that case, because it's even of interest to know whether it's three times solar or whatever. But those sorts of numbers come from scientists and are informed through our understanding of how planets work. The engineers then come up with instrumentation that can accomplish the task tied into the development of the spacecraft itself.
There are all sorts of other issues. I'm in great admiration of what takes place when people build spacecraft, because it's not simple. To give you just one example, the Juno spacecraft has to survive a severe radiation environment, because Jupiter's magnetic field leads to a magnetosphere in which there are high-energy particles moving along magnetic field lines, and they keep crashing into the spacecraft, and they can hurt the electronics if you don't put some of the most sensitive instruments in what we call a vault. It's a vault made of titanium. You choose a metal that's fairly low density, but dense from the point of view of obstructing the passage of high-energy particles. So there are all sorts of other issues that come in building the spacecraft. The spacecraft of course was built not at JPL. It was built by Lockheed Martin, and the people responsible for that are in an outlying suburb of Denver.
ZIERLER: Of course, it's beyond your immediate field, but I wonder if you can comment about what aspects of Juno were built with off the shelf components and what had to be built out of whole cloth, as it were.
STEVENSON: NASA is very conservative, and they like to have hardware that we know will work in space. It sounds easy to say that, but being sure that something will work is not so easy, and so you tend to be very conservative. You tend to rely on things that you know from previous experience has worked. In that sense, a lot of it is off the shelf. The ability to improve the accuracy and so forth might involve activities done here on Earth in the lab, but for the most part, the spacecraft relies on things that are off the shelf. In that sense, unmanned space exploration is somewhat different and cheaper than what people do in the military, where they're more willing to take risks, because human life is not so important.
ZIERLER: Dave, I wonder how you see Juno overall, as part of the New Frontiers program.
STEVENSON: I think that Juno has been very successful. Of course, it's tempting to think that anyone involved in the mission is going to say that it's successful—some sort of public relations effort, or whatever. But actually, it has been. The sense in which it is successful is important. It's successful because it surprised us. We learned things about Jupiter that we did not predict in advance. People who are not scientists may not immediately appreciate this, but scientists love it when they're proved wrong. In that sense, we were very happy with Juno, because it gave us a picture of how the atmosphere is behaving that is different from what we expected. It gave a magnetic field that is different from a scaled version of Earth's magnetic field. We didn't have a strong prediction in advance, but it was yet again an example of how planets surprise us by producing magnetic fields different from what we thought.
Personally, the ideas about the nature of the core were somewhat less of a surprise to me, but still very important. We're still struggling with what the gravity data are really telling us. But those three things—the nature of the atmosphere, which came from the microwave radiometer; the nature of the magnetic field that came from the magnetometer; the nature of the gravity field, which came basically from tracking—those three aspects each produced things that were different than we expected in advance. And everything worked! That's important. But the combined effect of all that is that it was a very successful mission. And of course it's ongoing. We have an extended mission. NASA had funded us to keep going for several more years.
ZIERLER: What was launch day like for you, ten years ago?
STEVENSON: Launch was interesting. It was the first and only instance of being close up to a launch at Cape Canaveral. I should say that some years earlier, essentially by chance, I happened to be at Cocoa Beach, which is admittedly a few miles further away, when the shuttle was supposed to be launched. Of course, by the time we were involved in Juno, the shuttle didn't operate anymore, for the United States. But I was there on the beach, actually with Steve Squyres, by chance. We were on a committee together, and waiting expectantly to see the shuttle be launched off in the distance. We knew that it was a spectacular show. But then they delayed the launch, and we had to leave. [laughs] So I never saw a launch before the Juno launch.
In the case of the Juno launch, we actually were permitted to come up close to the launch pad, and the spacecraft, which is of course on top of the rocket, the night before the launch. The launch happened the following morning, and we were half a mile away. And it is spectacular. Even though it's not as spectacular as a shuttle launch, but it is very spectacular, because of the immense brightness of the rocket, as the launch takes place, and the thunderous roar. Of course it's all over rather quickly, and you look and you see it going off in the distance, and you're hoping that everything works, and it did. But it's a memorable experience. And it was a hot and stormy day.
ZIERLER: Was there any drama associated with hoping that it got to where it needed to go?
STEVENSON: None that I know of. Spacecraft missions are sufficiently complicated that things can go wrong. The only thing I know about that caused any real concern is still with us, and in a sense has changed the way the mission transpired, and that is the orbital period, When we arrived at Jupiter, which was 2016—it took five years to get there; it takes a while to get to Jupiter—and by the way, you don't go directly [laughs]; you go out and come back, and you use the Earth to give you a little boost. So it actually came back and passed by the Earth and got some nice pictures of the Earth.
Anyway, it got to Jupiter in 2016, and there was a concern about sticky valves on the rocket, which led us to decide that we should not try to diminish the speed of the spacecraft so much as to bring it into the originally intended orbital period, which I think was 14 days. Instead, we let it stay in the initial orbit that was used when we first arrived, which is 53 and a half days, and that's where we have been until quite recently. It has modified very recently, because of passage of the Galilean satellites. That turned out to be a benefit, although I expect a NASA administrator might query it just slightly, because of course it makes the mission more expensive. But actually, the mission has turned out to be within cost, so NASA likes us. They sometimes have things that go over cost, although never as badly as the military who are used to doubling or tripling the original estimates.
So we stayed in this longer orbit, and you might say, "Well, isn't that bad? Because it means that you get the science so much more slowly?" But actually, there was enough interesting stuff to try and understand that there was a benefit to having the mission timeline expanded, as it was. We did the same number of orbits, but each orbit was 53 and a half days instead of 14. You can see that that meant that the mission went from 2016 through to quite recently in terms of what we call the nominal mission which is 32 or 33 orbits. So the need to think about what the data were telling us and to try and understand what was going on was sufficiently slow that it actually matched the rate at which data came back. In the end, the decision to stay in the longer period orbit was a good thing.
ZIERLER: Was Juno geared at all to yielding insight on the Great Red Spot of Jupiter?
STEVENSON: The Great Red Spot was not a major goal, but it attracts people's attention, people want to know about it, and so we did think hard about having fly-bys that were close to the Great Red Spot. And we had enough fly-bys. I say fly-by because each orbit brings you close in to the planet. That's what I'm calling a fly-by. A perijove. A perijove just means the point of closest approach to Jupiter. Each orbit corresponds to a perijove, and you can choose the longitude of the perijove to some extent as part of mission planning to guarantee that you get some data for the Great Red Spot. So we did get some data for the Great Red Spot. We got marginal evidence of a small gravity signal associated with the Great Red Spot, which tells us something about the depth of the feature. The depth is not so great, actually. It doesn't extend to a huge distance below the surface. It's of interest to fluid dynamicists, but not one of the main goals of the mission.
ZIERLER: Can you describe a little bit the mechanics of how Juno sends data back to Earth? And then for individual scientists such as yourself, how do you log in to see that data?
STEVENSON: The spacecraft has a microwave link back to Earth, and this is in fact how you keep track of the speed of the spacecraft and in effect where the spacecraft is. As you can imagine, navigating is of great importance. You need to know where you are. And of course, your trajectory is guided by the gravity field that you feel, so you have this continuous signal. You can also send back data at a limited rate. That data comes back to Earth and is collected in dishes on the surface of the Earth. NASA has what they call DSN, Deep Space Network, and these are three dishes, one of which is out in the Mojave Desert. That one can also transmit. I've been there. It's a fascinating place. It's on Army land, by the way, so it's not open to the public. There's another one that I've been to, because I used to live there. It's Tidbinbilla, which is near Canberra, in Australia. And the third one, which I've not been to, is near Madrid, in Spain. Of course, they are spaced in longitude on the Earth's surface so that as the Earth rotates, there's always a station in view for a spacecraft out somewhere in the space—out far away from the Earth.
So you collect those data. They are then taken care of not by me [laughs] but by people who know how to extract the information from the data stream. I never see the raw data. I could, I suppose, if I really wanted to see it, and I would not know what to do with it. [laughs] The sense in which I see the results is highly refined. It might be, for example, since I'm interested in gravity, a table of numbers that are coefficients for a particular mathematical expression called spherical harmonics that describe the gravity field as you would see it in space. Likewise, with the magnetic field. In the case of the microwave radiometer, I would see antenna temperatures, which are telling you something that is actually rather complicated to interpret, but in part it's telling you the behavior of temperature with depth in the atmosphere, and also the distribution of ammonia and perhaps water.
ZIERLER: When you say that the data is so highly refined by the time it gets to you, what does that mean? Is it prose? Is it spreadsheets? What does it look like?
STEVENSON: Tables. Numbers. Graphs. For the science I do, it's sort of at the high level, where I'm not quite so concerned with the intervening details. On occasion, you have to talk to the people who collected the information to understand what assumptions they might have made. And that does turn out to be somewhat important. So in the last 20 years, I've become a little bit more knowledgeable than I once was about the intricacies of understanding data. But I'm fortunate enough to work at a level where most of the time, I don't have to concern myself with what's inside the black box.
ZIERLER: As you made the important point that scientists actually enjoy being proven wrong, in light of that, in reviewing this data and working over it with your colleagues, going all the way back to your graduate school days, what has been most fun in being proved wrong? What has been disappointing in being proved right?
STEVENSON: [laughs] Well, it's not exactly what I did as a student or even later, but I have to say that by far the most surprising aspect of the results is the nature of the atmosphere at a level where we could not previously make measurements. We are using an instrument called the microwave radiometer, MWR, that receives passive radiation from the planet. Passive means you've got some gas deep down in the planet, way below the cloud deck, and it has a certain temperature and it has a certain composition. And like anything, it will radiate at different wavelengths including microwave. By the way, that's true of the human body, so I'm radiating all the time. Objects around me in the room are radiating all the time. That radiation comes back to the instrument as the spacecraft goes by, and is telling you something about the intervening gas.
The big surprise was this: everybody assumed prior to this mission that as you went below the cloud deck, the material that makes up the cloud, which for the observable part of Jupiter is actually mostly ammonia—ammonia cirrus cloud—so when you look at Jupiter through a telescope, that's actually what you're looking at, sunlight scattering off tiny crystals of ammonia. But as you go below the cloud, the mixing ratio of ammonia was assumed to be constant. And as you went deeper still, you would reach a water cloud deck. And then below the water cloud deck, the mixing ratio, the amount of water relative to hydrogen—that's what I mean by mixing radio—the mixing ratio of water would be constant. So you would assume uniformity of ammonia or uniformity of water.
The data from the mission told us it's simply not true, that somehow the ammonia that you expect to find has been hidden, and we don't know where it is. It's got to be there, because it's there high up. And this has made it more difficult to understand the circulation in the atmosphere, but at present, we can't even be sure that we have reached a level, much deeper still, where everything is uniformly mixed. So this perhaps naïve idea that everything was nice and uniform turns out not to be true. It's also true, although perhaps a little less surprising, that the magnetic field had anomalies that were a lot different from the field that we knew before we got there, and they were showing up at a higher harmonic.
Jupiter has something that some of us call a Great Blue Spot. Color doesn't matter. Great Red Spot actually is red; Great Blue Spot for the magnetic field is just a statement about the direction of the field lines. But anyway, there's a place where the field is very strong, and heading down into the planet, near the equator. This kind of local feature in the magnetic field was also not expected. So those are the kinds of things that surprised me. Was there something that I was happy about? I was happy about the fact that the spacecraft worked. But the surprises are the important thing. There weren't really instances that I can recall where we found exactly what we expected to find. That's good.
ZIERLER: Dave, I'm curious if your earlier work, and those of your colleagues, on Jupiter's satellites, like Europa, were valuable for the Juno mission?
STEVENSON: Not yet. The spacecraft will go to Europa, but that's in the future. The spacecraft was designed to learn about Jupiter, not designed to learn about the satellites. I have to admit that at the time the mission was configured, I did not even know that that was an option. This came up somewhat recently, but perhaps it was always an option and nobody told me. In any event, we are going to be able to do science at Europa. We've already done science at Ganymede. Those results are not published yet. They're interesting. We will even do some science at Io at the end of mission, and then crash into Jupiter. A pre-ordained crash, of course.
ZIERLER: What hopes and dreams might you have from an astrobiological perspective, with Juno's mission to Europa and other satellites?
STEVENSON: I don't expect the Juno mission to tell us much about the habitability issue. The Europa Clipper will do that somewhat later. The Europa Clipper will actually be launched at a similar time, but of course it takes a while to get out there, so we don't get data from that until, I don't know, 2030 or whatever. Maybe a bit sooner. I forget the details. Because SpaceX is launching it, and I don't know what the cruise time is. In any event, no, I don't really have habitability expectations. It's not closely related to my science, anyway. I've probably said this already, but when it comes to questions like life and habitability, I think that we are going to be surprised again. That we are going to be looking and looking and looking, and we're going to discover that the place where we're looking is the wrong place.
The classic example, and I did not invent it, is the joke that people sometimes make, when they say, "I found this guy on the street under a lamp post where the light was shining on the ground, and he was looking for his car keys. And it turned out that the reason he was looking in that region was because he could see in that region, not because he thought the car keys were in that region." So [laughs] finding life is going to be a bit like that, I think. And when I try to persuade people of my point of view, I make the point that this happened with exoplanets. People had all sorts of notions about planets around other stars in the 1970s and the 1980s and 1990s. And when we finally found something, it was not what we expected. That's good.
The Extrapolative Importance of Jupiter
ZIERLER: Dave, a point you made earlier, which bears some further development—how can you extrapolate learning more about Jupiter to our solar system writ large? In other words, existential questions about how planets formed, the age of the solar system—why is Jupiter the most sensible starting point for those broader questions?
STEVENSON: The main reason is that Jupiter is so massive, and flings its weight around, and affects the gravity that was important for the accumulation of material nearby, but even to some extent further away. For example, some of the material that arrives at Earth right now from space arrives here as a consequence of what Jupiter does. Jupiter carves out regions of the asteroid belt from which small rocks are delivered, meteorites are delivered to the Earth. But Jupiter, through its migration, may also have affected the places where planets form. So you want to understand Jupiter to understand the architecture of the solar system, but also just because it's the most massive, it might be a guide to the material that was used to make planets. I think in addition, that science relies on many sources of information. You don't want to build stories on just a single body or a single piece of information. You don't want to rely too heavily on one mission or one set of measurements.
What I've seen in my lifetime in science is the necessity of synthesis, the necessity to bring together information that comes from many directions, as part of building the story. It's like putting together a jigsaw puzzle, and Jupiter provides some pieces, but all sorts of other pieces come from elsewhere. So I would not wish to argue that Jupiter is going to answer that question; that's not how science works. Jupiter is going to pose the question. Jupiter provides constraints on answers to the question. That's how science progresses.
ZIERLER: Where are we now with Juno, just as a snapshot in time?
STEVENSON: Recently we went by Ganymede. No, I can't tell you exactly [laughs] where Juno is right now. I don't keep track. But it is in an orbit that is changing, or has changed, to a smaller orbital period, and so we're going to do a lot more orbits. We're going to collect the same kind of data, but of course we also benefited from data collected at Ganymede. So the goal at this point is to better characterize the magnetic field in the region where we think we might see variation of the field. The field is not static. It changes.
By the way, that's true for Earth's magnetic field. That has been known for hundreds of years. So it tells you something about dynamics inside the planet. It tells you something about the place where the field is being generated. So we hope to do that. We hope to collect more data about the atmosphere. We are going further north. Celestial mechanics dictates that as the mission progresses, the perijove, the point where we come closest to Jupiter, moves to a slightly more northern latitude each orbit. And so we're collecting data about the new region. Those things will happen in the coming years.
ZIERLER: Just as Juno was being conceptualized while Galileo was still active, are there current projects entrained that will be the next generation after Juno?
STEVENSON: Yes, however we are realists, and the fact of the matter is that these things cost a lot of money, and they take a lot of time to prepare for. You want to do them right. NASA is very good, by the way, at having things work. There was a problem two decades or so back where they were not doing quite so well because they were trying to do things too quickly. But for the most part, missions these days are doing what they're supposed to do. Let's face it; that's remarkable, because it's so easy to have something go wrong. SpaceX has discovered that.
In the case of Jupiter, we may have to wait a while for Jupiter itself. For the satellites, we're going to get more, because the Europeans have a mission called JUICE, and that will deliver data a little bit more than a decade from now. That spacecraft will go into orbit around satellites. It will learn about Ganymede, especially, to exquisite accuracy. So JUICE will happen, so we will learn more about satellites, which are planets in their own right.
But when it comes to the big guys, I think the thrust of the future is more likely to be the others. There's a desire to go to Saturn and do what we did with Galileo, but at Saturn. That means dropping a probe into the atmosphere, perhaps doing something like Juno at Saturn at some point. That's being talked about. And of course, Uranus and Neptune. I think that in the current decadal survey which is underway right now, of course there will be—there is—a lot of enthusiasm for Venus. But Uranus and Neptune are natural choices for future missions, because we really haven't been there. Yes, it's true—the Voyager spacecraft zipped on by, a fleeting encounter because it was moving very fast at that point. But we know very little about the specifics of the gravity field and magnetic field. People argue about Uranus versus Neptune. I think they're both very interesting. That's going to happen.
There is also a technology development that I am enthusiastic about, and will, I expect, show up in future missions. The technology development is seismology. It's becoming increasingly apparent, mainly actually through Cassini observations, but also maybe even Juno—it's becoming increasingly apparent that these planets are like bowls of jelly. They wobble. That is, they have fluctuations, vibrations. We call them normal modes. That's the technical terms for them. The Earth does this as well. The Sun does this. You can measure those normal modes. Those normal modes are a guide to the internal structure in a way that you can never get with gravity. This has been known for a very long time, that if you can do an exquisitely accurate determination of the frequencies of normal modes, then you're learning about internal structure.
By the way, this is how we figured out that the Earth has an inner core, and many other things about the Earth. The excitation on the Earth may be different. It may be mostly earthquakes. On the giant planets, it's not clear exactly how they are excited. But it's becoming increasingly likely that they are somehow excited. It's very much the same thing as what a physicist does when they interrogate a complicated molecule and get it vibrating—if you look at the spectrum, which is the peaks as a function of frequency, and the response of this complicated oscillator. Just think of it as a body that has normal modes like oscillations of a simple harmonic oscillator. You're learning something about the intrinsic properties of the body, and that can be done for Jupiter, Saturn, Uranus, and Neptune.
That's exciting, and I think it will happen, and it will perhaps happen through Doppler imaging, which means that you look at the surface of the body, much as we've done actually for the sun, only it's a very different kind of body, and you see a coherent motion up and down of fluid parcels in the atmosphere, which you can pick up and Fourier analyze and get specific frequencies for. So that's something in the future. We haven't done it yet because it's very hard to do, and because people had doubt about whether it could be done. But my prediction is that that will be a major area of science in the future for people interested in what goes on inside these planets.
ZIERLER: As you've written recently, it's really spectacularly impressive that Juno can tell us anything about the interior of Jupiter. But at the same time, I wonder what limitations you see in Jupiter's capacity to see this, and what future missions might do to improve, so that we can learn even more about the interior of planets.
STEVENSON: This is an issue of remote sensing, where there are non-uniqueness problems. I'll give you the example of gravity, because this is the one that we're up against some of the time, but it's to some extent true for magnetic fields as well. When you measure the gravity of a planet, even to exquisite accuracy, and we are doing it, to a part in a hundred million sometimes—something like that; it's really spectacularly accurate—but it was known a long time ago that measuring the gravity field outside of a body doesn't tell you exactly how things are distributed inside. There's a non-uniqueness problem, meaning that there are multiple choices that you can make for how material is distributed inside that will lead to the same result outside. It's basically the difference between having a three-dimensional map and having a two-dimensional map. That's why I mentioned seismology as something for the future. That's why I think that just getting more accurate gravity is not enough. Although [laughs] it's also true that gravity might actually sometimes detect these normal modes. So the challenge really is to figure out how to do measurements outside that tell you what's inside.
By the way, the same is true for the Earth, and that issue is still with us for the Earth. Obviously, we do a lot better on the Earth, because we can put seismometers all over the place. We can even put them on the ocean floor, so we can measure signals coming from multiple directions, and basically develop what people call a tomogram. The word comes from the medical jargon, just meaning three-dimensional structure from two-dimensional measurements. But it's hard to do. That's the challenge for the future. That's the limitation. I'm guessing, without knowing for sure, just based on the past history of science, that decades from now, people will figure out completely different ways of doing things that we haven't thought of right now. Let's hope so.
ZIERLER: We've already prognosticated on future missions beyond Juno, and we've already discussed your current work way back in our very first discussion. For the last part of this multipart interview, I'd like to ask you some broadly conceived overarching questions that will have both retrospective and forward-looking aspects to them. The first I'd like to ask you is the state of education and pedagogy in the field of planetary science. Now looking at your own trajectory, where you did not have a traditional undergraduate education in planetary science, you did not even know that this was a field, and in many ways your own life trajectory, and the field of planetary science in many ways came of maturity in tandem. For students today, undergraduates today, what is the course of study that you think is most efficacious if, ultimately, they want to pursue an academic career in planetary science?
STEVENSON: I would say that the most useful thing to do is a broad education. I do have a conceit that physics is important. I don't think that planetary science classes as an undergraduate are particularly important. These are not just words. This has been debated here at Caltech. I have colleagues who think similarly to me that it's not appropriate to encourage students at the undergraduate level to get too heavily involved in planetary science. Of greatest importance to those students is a good basic science education. I say physics, but really it's chemistry, as well as physics, and even in some cases biology. And of course, the tools that you use in those sciences, which come from mathematics. Mathematics is always at the background, things like Fourier analysis and knowing how to solve differential equations and so forth, ultimately those tools came from an understanding of basic mathematics, which is first expressed in an abstract way. Planetary science, by its very nature, grabs hold of everything from every field. That's why breadth is important.
The tricky part is in the enthusiasm that young people have for getting involved. People do get involved as undergraduates. I've involved undergraduates in planetary science. But I feel comfortable doing so because at the same time, they're getting that basic science background. I'm actually less sure about other institutions. To be clear, what I'm saying is that an undergraduate at Caltech gets what they need, which is the basic science. It's boot camp for basic science; that's what Caltech is. And if they have enough enthusiasm and time to do planetary science as well, then that's fine. But I don't think of it as essential. You could go on to graduate school without it, and some people have. Other institutions, which are less strong in teaching basic science, might in some cases not be doing a good thing for their students, because they're allowing them to get involved in something that they don't fully understand. In that case, I would say they need the basic science.
By the way, this is not just words. When we look at people applying to Caltech, one of the things I look for, and my colleagues look for, is strength in basic science. We've got to see it there. Those are the things. You want to see people who have done a couple years of physics, and get good grades in physics classes, understand continuum mechanics, understand electricity and magnetism. Even though it may not be directly relevant, the tools you learn there are helpful in a wide range of things. So the basic science is key.
ZIERLER: Dave, even more broadly, I wonder if you can comment on the role of convergence in planetary science with other scientific disciplines. One of the great themes in modern science is convergence, where scientists from seemingly disparate disciplines have interests that go beyond academic departments, from neuroscience to chemistry to robotics, to you name it. What are some of the ripe areas of convergence in planetary science where you or students can be working with colleagues who seemingly are coming from a very different world?
STEVENSON: Well, planetary science is the convergence. That's what I've seen. I've seen people who start out in other areas, for example in Earth science, and they get seduced into thinking about planets. You're right; the nature of science has changed, and people are more willing and more able to make the connections. I think that's good. I wonder sometimes whether it is done at the expense of understanding the basic science. I have to come back to that, because to me that's still key. I say that because I see people sometimes who get enthused about connecting various disparate things that were previously unconnected, but they don't actually understand the basic science well enough to do it right. I guess what I'm saying is convergence is good, but basic science is key.
ZIERLER: I wonder if you can comment broadly on the state of play in the Division of Geological and Planetary Sciences, given your broad historical background? Where are we today at GPS?
STEVENSON: GPS is in good shape. We have hired young people. We have hired well. We have good demographics. We're not too heavily invested in running large institutes or centers or this or that, or instruments. I think that's good. I think people who do good individual science is key. We do that. It's a friendly environment. The students say that. People are busy, so they might not talk to each other as much as perhaps they could. I'm guilty of that, too. But we're actually in pretty good shape.
We have broadened, and that has led to tensions. Let me be clear what I mean by that. The GPS division that I knew when I came to Caltech, that I came to know when I came to Caltech, was more heavily oriented towards the traditional idea of solid body science, which includes geology. It includes people who look at faults and rocks and so forth, but also includes people who think about the mantle and the core and so forth, and to some extent, other planets. It had less involvement in the surficial science that is now more evident. Surficial means the atmosphere, the ocean, the chemistry that dictates those, things having to do with climate, global warming, pollution and so forth. Those things have become a bigger piece of the total.
But the other big thing, which is a different scientific universe in a way, is the biological aspect. Because we live on a planet where our environment has been greatly modified by life. We need to understand that. We need to know how it evolved. It plays a key role in understanding oxygen in the Earth's atmosphere and so forth. The consequence of this is that we now have people doing disparate things, and it's harder to have a common ground, so that when you have a seminar, for example, that is supposed to be suitable for everybody in the Division, it can be more difficult if somebody is up there talking about something biological, which is out of the realm of things that you might have learned at an earlier stage in your career. So it's a challenge. I'm not complaining; I'm just observing what has happened.
We are more coherent. Geological and Planetary Science is more coherent than, for example, Physics, Math, and Astronomy. Inevitably so, because PMA has these disparate pieces where people think rather differently about what they're trying to do. So we still have some common ground, but it has become a strain when you become a little bit larger and a bit more diverse. You were talking about convergence, but convergence also involves diversity. It involves bringing together diverse elements. And that can be hard to do.
ZIERLER: Your previous answer alluded to this, but it bears further development. As I've learned, an unofficial motto at Caltech is that we don't strive to do everything, but what we do choose to do, we strive to do as well as we can, or even at the best, at the highest level. In your long career at Caltech, within GPS but really across the Institute, what have you found to be the most productive ways that Caltech institutionally makes those decisions about when to emphasize depth in a particular area, and when, as you suggested with GPS, the mode is to go more toward breadth?
STEVENSON: Well, that's a difficult question. I think that the main thing still is individual excellence. What this implies is that when you're hiring someone who does something different from what you're doing now, you have to hope that that person is so excellent at what they do that they can make connections, and that they can function independently.
Frankly, that's not always true at other institutions. At other institutions, the attitude often is to say, "Oh, we already have three people doing X. Let's get even better at doing X by adding another person who does X, and then we will have four." That kind of thinking. I'm exaggerating slightly. We don't do that at Caltech. So I think that the key idea still, at least in my mind—I'm not sure everyone agrees on this, but in my mind at least—the key idea is individual excellence, and choosing people who can make those connections even when the subject is becoming more diverse.
You are right that Caltech drops areas on occasion, and I would say that in striving to maintain approximately the same size, which is what we have done, we are adhering to that notion that you're looking for excellent people, without regard to the specifics of what they do. That is important. The area I know a bit about that we dropped, one of many, is solar physics. We actually dropped that. That was an interesting one, because we had excellence in studying the sun. We were even pioneers. But we reached a point where it was not clear that we were pioneering anymore, and the people involved were in some instances approaching the end of their career, and so we decided to drop it. We even sold a telescope up at Big Bear. So a telescope that used to look at the sun up near Big Bear Lake is no longer owned by Caltech. That doesn't mean that it's not doing good science; it is doing good science. But we have to make choices.
We have avoided the notion that because you are in a particular field you have to keep that field going. When we have discussions about the future of the science we're doing, as we do on occasion, we don't start with the assumption that because we're strong in X, we're going to keep doing X. I think that's good.
ZIERLER: Dave, your emphasis on the importance of excellence at the individual level inevitably raises questions about recruitment—recruiting the best scientists and retaining them. What have you found to be Caltech's strongest selling points for a scientist who might say, "Well, a much bigger place like an MIT or a Berkeley, where there are simply more people doing the kinds of things I'm doing"—what has been Caltech's most effective counter to that, given the fact that its small size is inescapable?
STEVENSON: Well, it's not a single counter, but one of the counters is just simply choosing people who can see the value of being at a place where everybody is excellent, and who have enough self-confidence in their excellence that they can do the science they need to do without having other people do the same thing. I'm not saying that there are no complaints about this. There are people who sometimes are unsure about being at Caltech because they would like to have other people like themselves. But I think I've said it correctly, that if you go out of your way to choose people who can be independently excellent, then Caltech becomes attractive.
It's also true that the smallness of Caltech expressed through the number of students is an attraction. For people doing research, which after all is the central notion of Caltech—even though we pay attention to education, it's still research that's most important—people also look to the excellence of the graduate students. We get the best graduate students. It's also true that, for the most part, Southern California is a nice place to live.
ZIERLER: [laughs]
STEVENSON: Better than MIT!
ZIERLER: That's for sure. [laughs] You mentioned diversity in planetary science, and I'd like to ask about that word in a much different context—your long tenure in planetary sciences as a community, and the sociology of planetary sciences. In the broader emphasis to make STEM more diverse and inclusive, have you seen the community of planetary sciences more as a leader or as a follower in making the field more diverse and inclusive?
STEVENSON: Well, it's a collective enterprise. The division of which I am part has several options. Planetary science happens to be one of those options. Each option has its own culture, and they differ a little from going to, say, geophysics or geochemistry versus being in planetary science. But anyway, in planetary science, we've never adopted the notion that there is some idea of leadership, some particular person who is defining the direction for everybody else. We just do our own good stuff. In fact, planetary science at Caltech epitomizes what I said about individual excellence, because we actually have very little overlap with each other in terms of the specifics of what we do. I need to be careful what I say there, because if you're excellent at something, part of that excellence is awareness of what people are doing in other areas. I've noticed, looking around the scientific community and at other places in the country, that this is not universal. So my notion of excellence includes the notion of breadth, not just being a Delta function.
But the fact remains that we don't aspire to a particular idea of leadership, particular direction. Of course, we debate directions. Of course, we debate how important it is to do exoplanets versus something else. The desirability of having somebody who does atmospheres. These debates take place on a fairly frequent basis, but in the context of collective decision-making and no specific leadership.
ZIERLER: I'd like to ask a few questions about where you see some ancillary contributions in your own career and in planetary science writ large to other disciplines. The first I'd like to ask about is gravity. Now, as you well know, gravity is one of the major problems in physics. Physicists are still trying to figure out how to unite gravity with the other forces. And gravity can mean something very different to a cosmologist as to a string theorist. Where do you see your work in gravity fitting in with those overall questions in physics?
STEVENSON: Only at the periphery. Only at issues that some people would consider to be minor, although it depends on your point of view. Certainly, in the anecdote I gave you about Feynman some time back, it became evident that he looked upon me as somebody who might provide guidance on the possible existence of a fifth force, something that is not one upon r squared. I'm talking, of course, about classical ideas of gravity and ideas of simple equations for the force law, which is Newtonian. So it arises there, and it arises a little bit when a colleague at JPL suggests that there's something funny happening to the acceleration of the spacecraft which doesn't seem to fit with Newtonian gravity, so perhaps we'd have to change the laws of gravity. I confess to being rather conservative about such matters. I require very good data to take [laughs] things like that into account. But as I said, the way I think about gravity is very practical and for the most part doesn't draw me into those interesting questions of the nature of the gravitational force.
ZIERLER: I'm curious if not in your specific research but in the field of planetary sciences in general, you see a role in the urgent question of understanding climate change. In other words, climate change involves scientists of almost every imaginable stripe. Is a planetary scientist who looks quite literally at the whole picture, do they have a valuable role to play in climate change research?
STEVENSON: I think we have a role, but perhaps not central. The role lies in the ability of a planetary scientist to step back from the details and look at the broader questions. It has arisen, for example, in thinking about the idea that Earth's atmosphere might evolve into something nasty like Venus. And that kind of speculation involves knowing about the atmosphere of Venus, knowing about how it might have evolved, knowing about how the luminosity of the sun might have changed. These are big questions that might not occur to somebody who's looking very narrowly at a particular set of issues having to do with climate change.
It's also true that there are people in planetary science who care about pieces of the problem that might shed a light on how to model Earth's atmosphere, for example clouds. Clouds are actually a common feature in the universe. We see them all over the place. Lots of planets have clouds. Exoplanets that we've found, a lot of them have clouds. And so if you understand cloud physics a little better, and some of that understanding might come from planetary science, then that might feed into the highly specific, highly specialized work that is done in climate change.
But I need to be clear about something rather important: when you talk to people in Earth science, in geophysics or in atmospheric physics or whatever, they are working at a much more detailed level on their problem than the normal skimming of the cream that happens in planetary science. And so it sometimes happens that those people will look across at planetary science and be dismissive of what we're doing, because they see what we're doing as rather superficial. In a sense, they're right, but as I've tried to make clear, balance is important. You need the people who do the highly specific things, which are actually very complicated in the case of climate change. And you need the people who also have the ability to appreciate the problem, far removed, as can happen with planetary science.
I should say that in my career, perhaps just by chance, I've actually encountered several prominent scientists who are still alive, who question the anthropogenic aspect of climate change. And these are not people [laughs] who do the highly specific stuff in climate change. And so that's a perspective. I actually don't agree with them, but that's an interesting perspective on the problem that must make it hard for the general public to understand how these disagreements arise. Because you can have fairly knowledgeable scientists, but scientists in a different field, wondering about how accurate the climate change analysis really is.
ZIERLER: Of course, prior to the technological advancements that allowed planetary science to become its own discipline, the field of chemistry was, by definition, terrestrial only. In what ways has our understanding of chemistry changed in our ability to analyze chemicals beyond planet Earth?
STEVENSON: I think that for the most part, the chemists are ahead. What I mean by that is that the same periodic table applies everywhere. The same basic principles of quantum mechanics apply everywhere. Gibbsian thermodynamics applies everywhere. And so for the most part, you don't encounter things on other planets that are, how shall I say, alien, to a terrestrial chemist. For sure you encounter things that are less common. [laughs] Most obvious of all, of course, is hydrogen. It's funny to hear people talk about hydrogen on the Earth, because of course free hydrogen is almost completely unavailable, whereas elsewhere in the universe, it's overwhelmingly [laughs] hydrogen.
So of course, there are differences in abundances, and differences in the molecules that arise. But in the recent discussion, for example, of the atmosphere of Venus, where people were wondering whether they even saw evidence for something that required a biological process, they weren't really talking about things that would be regarded as outside of the normal photochemistry that a terrestrial scientist would think about. The only difference, of course, lies in the difference of pH values and how much hydrogen is present, and so on and so forth. Different conditions, different outcomes, but the same basic principles. Chemistry of course like physics has informed us in how we should think about planets. But it's not as if the information flow is very much in the opposite direction. I could be wrong about that. There are people—I happen to have a background in high-pressure physics—there are people who want to talk about materials in bodies like Uranus and Neptune that are very different from what you would think about on Earth. But it still involves in the same quantum mechanics, the same ways of thinking about chemistry, so I don't see it as completely different.
The Triumph of the Periodic Table
ZIERLER: Is this to say that so far as we know, the periodic table is truly universal?
STEVENSON: Yeah. In fact, I would say that's one of the triumphs of science. I would point to 1957 and something done at Caltech in 1957 or thereabouts, and in the years immediately preceding, that went into that recognition. Because it was in 1957 that a very, very famous paper, a very highly cited paper, that involved Caltech to some extent, was put together. The paper I'm referring to was by the two Burbidges who were married to each other, and Fowler, who got the Nobel Prize at Caltech, and Hoyle who I met later in his career. Hoyle was a famous astrophysicist and spent time at Caltech.
They were the ones who worked out, not perfectly, but in essential detail, the distribution of atoms, the different nuclei, in the universe, and showed that you could make sense of the relative abundance of the various elements—silicon, magnesium, iron, oxygen, carbon, and so forth—you could make sense of that through an understanding of the physics of what takes place in massive stars. And I'm going through that story, which doesn't sound like planets at all, because of course the stuff that makes up planets comes from the stuff that was previously in stars. We think that the early universe did not have heavy elements, but we also think that heavy elements are key to understanding the existence of planets. Even planets that are mostly sunlike, such as Jupiter, we think have substantial amounts of heavy elements, and the current view is that those heavy elements were essential for their formation.
So yes, the periodic table is universal. The physical laws, the chemistry, these things are tested. I'm not just making assertions. You can test these things, because you can look through a telescope, you can look at distant parts of the universe, you can see the same spectrum, albeit red-shifted, and so you can build a story, a comprehensive story, about the evolution of the universe leading to the formation of planets. The details when it comes to planets are quite complicated. They're imperfectly known. But the universality that goes into that understanding is rather well established.
ZIERLER: To complete our grand tour of the sciences and to return to something we talked about earlier but I'd like to bring up again here is a comment you made about, "We will likely be surprised about how we find life elsewhere." And to that point, what might planetary sciences tell us about biology at the most fundamental level, how life begins, and how we might come to define life that exists outside of our planet?
STEVENSON: Well, any answer to that is necessarily speculative. When I say that we're going to be surprised, the implication is that I don't know what it's going to look like. I do think, and I have said this in an article in Physics Today, that we should approach this problem on a broad basis, meaning that we should be willing to look at everything. Now, a person who funds scientists will get irritated by statements like this, understandably, because they like the notion that you can organize from the top down. I don't think that's sensible. But it does lead to a challenge, and the challenge is this: when you do science, you're always limited by the resources available, which often means money but might also mean telescope time or whatever. So you can't do everything, and you do have to make choices. But I guess what I'm saying is it's not clear to me that deciding in advance the best choices is that much better than a lottery system, or a random number generator. Obviously, you want to choose excellence, and so you need to make sure that the things you're choosing are well thought out and makes sense and so forth. But you don't want to, for example, as I worry about, have a situation where JWST, which is the overpriced, late, next telescope in space, you don't want to have that instrument focused on a particular subset of issues having to do with planets. That could happen, because there are committees that decide who gets to use that telescope. You want to have an approach to observation that just looks at everything.
By the way, astronomy fits my notion in the sense that every time we look in a new direction or a new part of the electromagnetic spectrum, we find something. So being open-minded about what to look for will be an advantage. And maybe that will come through looking at planets. Maybe not. Who knows? Planets are probably a good place to look for life. [laughs] There are people who devote their careers to what are called biosignatures. I'm mildly skeptical about their ability to really do this, because I'm not sure that they know what they're looking for. This is not a criticism of their scientific ability; it's just a recognition of our collective ignorance on the subject. But you would hope that we can just look at a lot of different things and maybe someday we'll kick over a stone and see a beetle.
ZIERLER: If I have the chronology right, next year would mark 50 years from your arrival in Ithaca to begin your career in planetary science.
STEVENSON: Actually, I hadn't thought about that, but I arrived in Ithaca in [laughs] late August, 1971. So that's 50 years, yes.
ZIERLER: All right, even better, so we're in the 50 years—
STEVENSON: Until you brought it up, I hadn't thought of it.
ZIERLER: We're in the 50 years now.
STEVENSON: Yes.
ZIERLER: With that broad historical perspective, what are some of the questions in the field of planetary science that in the intervening 50 years have been answered decisively? And what are some of the questions that remain as open as when you were first exposed to them 50 years ago?
STEVENSON: You're asking a reasonable question, but I have to say that it's not really the way I think about science. The way I think about science is that it doesn't answer questions; it just makes the questions more sharply defined. And I think we are now in a position to more precisely ask what may have taken place when planets formed. I mentioned once before that I attended a conference on the origin of the solar system in 1976. The conference was memorable for the lack of data and people speculating wildly. Nowadays, if you had a similar kind of meeting, you would still have people speculating wildly, but there would actually be a hell of a lot of data that could be talked about. I think that's immensely valuable. So I think we have a much better understanding of the kind of issue that needs to be resolved, and it has to do with what some people would consider details but are really central to understanding the process, like the ratio of particular elements, temperature structure, and so forth. I think we have a better understanding of how magnetic fields are generated and under what circumstances they should happen. I say that [laughs] because it's closer to what I've done myself.
But I have to say that when it comes to understanding the origin of planets, sort of the big question, that the sense in which we've made progress is more by a better definition of the question, a better understanding of the conditions, and most importantly, the immense amount of data. When I was a student, you could not even be confident that other planetary systems existed. Now you can say, "They're all over the place." Basically, to a first approximation, every star has it. Don't know that for sure, but the data would suggest that that's the case. That's progress! And that means that you can better define the question by saying, "Well, this means that the process of making planets is universal. It's not some special event."
You could still, half a century ago, have said, without fear of contradiction, that the formation of our solar system was some special event, and all the other stars don't have it. So in that sense, we're making progress, but it's progress driven by data more than being driven by theoretical ideas.
ZIERLER: Is that confirmation of an obvious inference? In other words, any high school science student will tell you that our sun is not a particularly special star, and therefore, just by simple logic, if it's not a special star, why should the planets that revolve around it be special either.
STEVENSON: Well, I would not accept that argument 50 years ago, nor do I accept it now, because the formation of a star doesn't tell you about whether or not planets form about it. After all, the planets are a tiny mass. The mass of Jupiter is 1/1000th the mass of the sun. So to me, that's not a logical progression. You can't go from being aware of the existence of the sun and other stars that we can see through telescopes being somewhat similar, to the statement that our solar system is a common feature. It's just not possible to do that, nor is it possible to say that because we have life, life is everywhere. Although it may be true, of course [laughs], that life is everywhere.
By the way, this issue of a logical progression of ideas also applies when you think about moons. Every few months, there's a story in The New York Times, rather too many of them actually, where people say, "Oh, they found an Earthlike planet." Often, it's not true. But anyway, that's what they say. And then the question arises, well, okay, we've got lots of so-called Earthlike planets. Don't we have lots of moons? Meaning an object that is in orbit around those bodies? Well, actually we don't know, and I would say at this point, that we don't know whether the formation of a moon, in the circumstances that we see it, is actually a common process. I suspect it is, but truth is, we don't know. Bottom line: data matters, when we're talking about something complicated. It's more complicated than what happens when you collide two protons together. Understanding planets is a complicated business. It's more complicated than understanding stars.
ZIERLER: Dave, what has planetary sciences taught us about the overall size, the vastness of the universe?
STEVENSON: I would say nothing. [laughs] We've known for a long time that space is big, and that the distance between stars is huge, and maybe that's why we don't get visited. But I don't think planetary science is helping in developing that story. Obviously, if we reach the point where the science has evolved so that you can learn more about life elsewhere, then connections perhaps could be made. But at the moment, I would say planetary science has very little connection to cosmology.
ZIERLER: Dave, a perfectly fun and speculative question, because at this point I think we've earned it—it's entirely outside your field of expertise, but given your approach to data and the way you talk about answering scientific questions, do you have any particular feelings about the existence of a multiverse?
STEVENSON: I do have an opinion about this, but it's a more general statement than the specific issue of the multiverse. And the statement is this: I am very uneasy whenever people talk about something that is not tied to observation. So when people bring up an issue like the multiverse, if they are talking about it in the context of some grand scheme that they have in mind that avoids having an origin in time or whatever their motivation is, I say to myself, "Well, you're free to speculate, but I think of science as being intimately connected with data." So I dislike it when people talk about things that are not tied to an actual observation. I would say the same thing about some of the mysteries of quantum mechanics or any other area of science that the key issue always is measurement. By the way, it's ironic, isn't it, that people sometimes think of Einstein and his ideas as being abstract? But in fact, if you look at what he actually wrote, it's always tied to observation.
ZIERLER: Isn't that one of the great lessons of LIGO, then?
STEVENSON: [laughs] Yes.
ZIERLER: Dave, two final questions looking purely to the future. I'm not sure if it's your style as a mentor to graduate students to be particularly direct in your advice on the things that they should focus on. But if you were pressed, with an excellent graduate student who was talented but not particularly focused, and your interests were in helping this student achieve an excellent career in the most fruitful areas of research in planetary science over the next 10, 20, 30 years, what would you tell him or her to focus on?
STEVENSON: Well, I don't. [laughs] If I've learned anything from being involved in mentoring students, it is that they are individuals, and they're different from each other, and some common advice usually does not make sense. Simply it's important to be curious. It's important to be adaptable. Something that I do worry about is when people become focused on a particular thing and think that that's their thing for life. Sadly, there are actually a lot of people in the scientific community who more or less do function that way. They do something, some particular thing early on, and stay with it. Of course, if you look superficially at what I've done, I've stayed with Jupiter. But anyway. [laughs] I'm trying to do other things. So I think being adaptable. I think being open to new directions, I think that's an important part of doing science.
When it comes to what the important questions in science are, of course the answer might be different to a theoretical physicist than to a planetary scientist or to somebody in some other area. But the one general answer that I would go with is not very useful, but it expresses what I think is the most pressing scientific question of our time. That question would be, what's the origin of life?
ZIERLER: Finally, Dave, you embody the notion that emeritus does not mean retired. For however long you want to remain active, what's most important to you? What do you want to accomplish that you have not yet?
STEVENSON: I enjoy doing science, and I enjoy doing it in an unstructured way. Unstructured means that what I'm doing one year from now may be unrelated to what I'm doing right now. I like it that way. So I'm always uneasy when I see people who have mapped out what they think they're going to be doing for years. Of course, I realize that when you're running a lab, sometimes you have to think about science that way. But I have the luxury of being able to think about science the way you would think about it if you were sitting on a desert island. Access to the internet is useful, but other than that, pencil and paper will do fine. Maybe it's also useful to have a program like Mathematica. Anyway, I do want to just continue doing science, being enthused about issues that arise. Sometimes frustrated by what I think are inadequate efforts by people in some other area like exoplanets. Maybe I will finish my textbook. Ha! [laughs] Who knows. But being unstructured is what suits me, and I'm happy to do it that way.
ZIERLER: What you're saying, of course, is that you've never had a grand plan, and you'll continue not having one.
STEVENSON: Correct.
ZIERLER: Dave, this has been an epic journey from New Zealand to California, from Jupiter to the exoplanets. You have provided a treasure trove of historical insight and perspective that yields just wonderful information institutionally for Caltech, for the field of planetary science, and just overall for your approach to science in general. I deeply want to thank you for spending all this time with me, and it has been a great honor to do this.
STEVENSON: Well, thank you. It has been interesting. Longer than I thought, but fun.
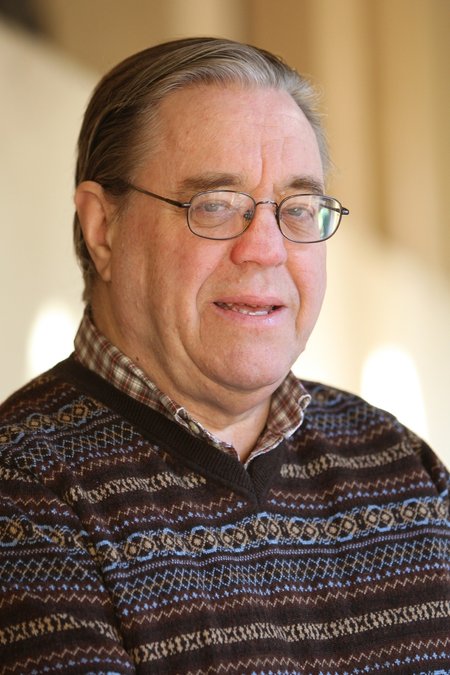
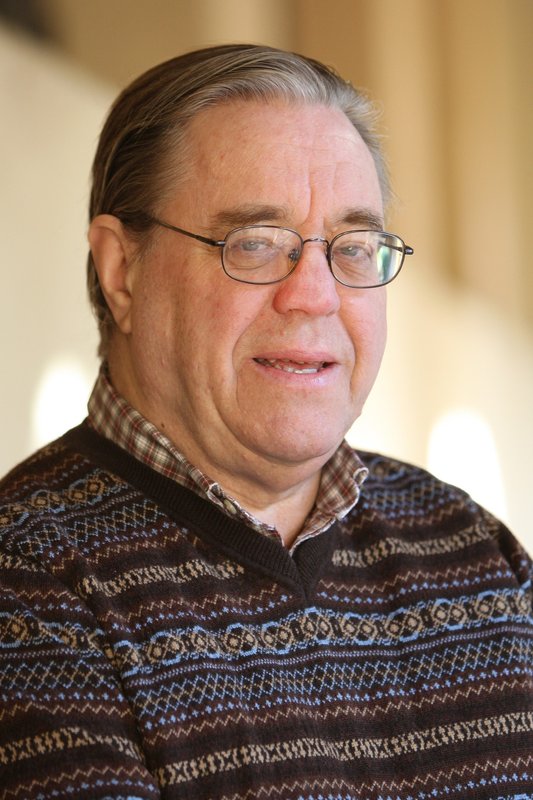
Interview Highlights:
- Juno and Jupiter
- New Zealand Origins
- Neil Ashcroft and Metallic Hydrogen
- Cornell and Introduction to Planetary Science
- Advice from Freeman Dyson
- The Uniqueness of Earth Below the Surface
- From UCLA to Caltech
- Voyager and JPL
- The Origins of the Moon
- Feynman and the Challenger Disaster
- Caltech and the Presidential Search
- Enhancing the Caltech Curriculum
- Galileo and Magnetic Fields
- Life Sustaining Planets Beyond Earth
- The Mysteries of the Internal Structure of Earth
- The Extrapolative Importance of Jupiter
- The Triumph of the Periodic Table