Egill Hauksson, Earthquake Seismologist
For natives of Iceland, it is nearly impossible to be unaware of the dynamic processes of the Earth's interior. As the first in his family to translate that awareness into a scientific career, Egill Hauksson began his professional studies at the University of Trondheim in Norway before moving to New York to complete a PhD at the Lamont Observatory at Columbia University. With nearly five decades of research in geophysics and seismology, Hauksson has been present and involved in many of the key advances in these fields, from pursuing the question of earthquake predictability to mitigating earthquake danger.
Upon moving to the University of Southern California, Hauksson became closely involved in the conceptualization and launch of the Southern California Earthquake Center, an academic consortium dedicated to studying all aspects of earthquake science which has the ability to pool resources beyond the capacity of any single academic institution. Recognizing the enormous professional opportunity to join the Seismo Lab in 1989, Hauksson concentrated on modernizing the Seismic Network and ensuring the network's access to cutting-edge software and monitoring tools with a dual benefit to earthquake safety and fundamental research.
In the discussion below, Hauksson emphasizes the importance of studying historic seismic data. While a century is a blip in the geological timescale, a historical analysis of seismic data remains a vital resource to probe basic questions relating to cyclicity, the role of stress in the Earth's crust, and seismotectonics - the subfield that focuses on how earthquakes deform the Earth's surface. Over the course of his career, Hauksson's contributions have spanned from applications of basic science and their translation to public policy, and to gaining better understanding on the most basic questions relating to how and why earthquakes occur.
Interview Transcript
DAVID ZIERLER: This is David Zierler, Director of the Caltech Heritage Project. It is Tuesday, September 6th, 2022. I am delighted to be here with Professor Egill Hauksson. Egill, it is great to be with you. Thank you so much for joining me today.
EGILL HAUKSSON: Thank you for the invitation.
ZIERLER: To start, would you please tell me your title and affiliation here at Caltech?
HAUKSSON: My title is Research Professor of Geophysics, and I am in the GPS Division, or Geological and Planetary Science Division, at Caltech. I am also housed in the Seismological Laboratory.
ZIERLER: How long have you been at Caltech?
HAUKSSON: I have been here since December 1989, or more than 32 years.
ZIERLER: Was that as a postdoc, or were you already part of the faculty?
HAUKSSON: I was hired on as a Research Associate of Geophysics, because that was about eight years beyond my graduation from the PhD. Later, I was promoted to Senior Research Associate, and a little later after that, Caltech changed those titles, and my title became Research Professor of Geophysics.
ZIERLER: Is your primary appointment in the Seismo Lab? How does that work administratively?
HAUKSSON: My primary appointment, like everybody else's, is in the Division of Geological and Planetary Science. The Seismo Lab is a sub-entity within the Division. My supervisor is the director of the Seismo Lab, as opposed to most professorial faculty who view the division chair to be their supervisor.
ZIERLER: Just a snapshot in time; what are you currently working on?
HAUKSSON: Currently, I'm working on maintaining and improving the earthquake catalog that we have generated over the last 90 years or so. I'm focusing on the last 40 years that have the best data, refining it so we can better use the data to identify active earthquake faults and study earthquake physics using the data.
The Historical Seismic Record
ZIERLER: A catalog that goes back 90 years, do you have a sense of its history, how it got started, and who has worked on it over the decades?
HAUKSSON: I do, some, but the early years, it's somewhat vague, though it's clear that Charles Richter was quite active. Before him, Harry Wood, the director of the Seismo Lab, was the one who laid out the plans for the Network and was instrumental in having seismic stations installed. At the time of the Long Beach Earthquake back in 1933, they had eight permanent stations up and running with local recording on photographic paper; they used similar records of Morse code to time the data. At that time, they had two portable stations, which they could move around, which they had generators to operate those stations. That was quite an undertaking at that time, to operate those. Since 1933, the catalog has grown. Mostly, technology has made it grow, in that in the early years, they were able to locate a few hundred earthquakes per year, and then as technology improved and the stations improved, we have been able to locate more and more earthquakes. Nowadays, we easily locate, in a regular or slow year, 20,000 earthquakes. If it's a busy year, we may be locating 40,000 or 50,000 earthquakes.
ZIERLER: To the extent that you have gone through the catalog, what are some of the things either from a technological perspective, a theoretical perspective, or an observational perspective, that would seem the same to you as it would have to Harry Wood or Charles Richter? The same kinds of questions.
HAUKSSON: There certainly are a lot of questions that are similar, and some are different. If you look at the technology, in the old days they made all their own instruments. Nowadays, we don't do that. We buy them from commercial manufacturers. You need to, at each seismic station, know exactly what the time is, because you want to know when the seismic wave arrived there, because that allows you to triangulate back to the epicenter of the earthquake. For that, they used Morse code back in the 1930s, and then various navigation systems were used later on, until GPS came around. Since then, we have used GPS for timing the data, and that's much more accurate than was possible in the early days. The other difference is having digital data. When the USGS joined Caltech in the early 1970s, they brought along a lot of stations that were deployed, and they provided what we call analog data, which was simply a tone that was transmitted back to Caltech on a phone line. Then that was recorded on microfilm, real time. It's a real mess trying to record data on microfilm, because you have to develop the film as the data come in, so it has to go through the various liquid processes. Sometimes, those tanks and that plumbing would go bad, and the fluids were all over the lab, to the aggravation of our neighbors on the first floor. We were on the second floor, the Seismo Lab.
Development and the application of theory to analyze data real-time in the early days was hampered because the data was off-scale if the earthquake was of any size. There's this tradeoff that if you want to detect numerous quakes, you can amplify all the signals that you record and really detect the smaller events, but the price you pay for that is then, when a big earthquake happens, it knocks everything off scale because the motions are so big. Caltech and USGS did that with the Network in the early days, starting in the 1970s. Prior to that, most instruments would also go off-scale for the bigger events. However, there were some instruments that they operated—just in case a big earthquake was to happen, they kept them at a very low magnification. That meant the instrument would sit there for decades and not produce any useful data.
But suddenly, when there was something like the Chilean earthquake back in 1960 of magnitude 9.5 or so, when that happened, actually Caltech collected some very important records on some of the instruments, that were on-scale and can be analyzed with modern theory. Nowadays, it's all about speed and getting data transmitted very quickly to drive earthquake early warning. That means we have to have very good data communications. We have to have very good equipment in the field that is well- calibrated and is sending data all the time, so when an earthquake hits, you don't have a bottleneck, because you happen to have more data. That's an important progress that has been possible based on the commercial technology that we all use today, like cell phones.
ZIERLER: Do you have a sense, going back 90 years, if the founders of the Seismo Lab thought that one day the technology and the cataloguing would progress to a point where something like early warning would be feasible? In other words, were they working towards some applied goal?
HAUKSSON: They were clearly working towards both understanding earthquakes better as well as earthquake faults. They talked about deploying both a regional network that would capture data from all the different faults on the West Coast, as well as deploying very sensitive instruments inside fault zones using bore holes instrumented with microphones. It was clear that they thought one day they could maybe possibly predict earthquakes if they understood how small earthquakes related to big earthquakes. I don't think they may have so much thought about early warning in those days, because those timeframes are quite a bit shorter than probably they were thinking about, but certainly they wanted to be able to understand if there was a lot of things that happened in the ground prior to a major earthquake. Unfortunately, the research that has been done since shows that the preparation zone for an earthquake can be extremely small. It's maybe eight miles down there below your feet, and there's a lot of geology between your feet and going down eight miles. Then it may be that you're looking at a zone that's maybe ten feet to 100 feet across that is moving or doing something. Detecting that at the surface is extremely challenging.
ZIERLER: The idea of earthquake prediction in this early era of the Seismo Lab—as you mentioned, they were looking at the relationship of small earthquakes to large earthquakes. What was so fundamental in terms of understanding that relationship?
HAUKSSON: What was fundamental is that I think they already understood at that time that small earthquakes are a lot more frequent. The size distribution of earthquakes follows a power law, which means that if you have one magnitude 6, you get ten magnitude 5's, then a hundred 4's, then a thousand 3's, then ten thousand 2's. They were wondering if that had some pattern that would change, prior to big earthquakes, as an example.
ZIERLER: For you, having access to the catalog, does it improve your research? Are you able to do things simply as a result of having this catalog and looking at it?
HAUKSSON: It does, yes, because it allows us to recognize patterns—where are earthquakes occurring, and where are they not occurring, and allows us to understand some of the fault properties, because some faults will accommodate small earthquakes, and some will not. When a magnitude, say, 7.1, like the Ridgecrest earthquake back in 2019 occurred, we were able to go back to the 1930s and say, "This is unique for this region. It hasn't happened before during our catalog." That's pretty important to understand how these earthquake faults behave, being able to look in detail at what has been going on over the last 90 years.
ZIERLER: I wonder if you can talk a little bit about your sense of the field. You're a research professor of geophysics, but you're primarily interested in seismology. Do you do anything that is outside of the bounds of seismology but within geophysics?
HAUKSSON: I have not done that here at Caltech, but I did so for my PhD thesis. I did study radon, which is a gas, dissolved mostly in water or geothermal water, to see if there was an anomalous radon quantity being emitted prior to earthquakes. I also did some studying of rifting episodes in North Iceland at a volcano called Krafla, which was very active starting in 1974.
ZIERLER: I want to ask some nomenclature questions, just what the definition is, and what you do in that field. Let's start with seismotectonics. What does that term mean?
HAUKSSON: In Greek, tectonics means "to build," so seismotectonics in my mind is a way of describing how earthquakes are deforming the surface of the Earth. What we get out of our earthquake catalog is not only the location of the earthquakes; we get the depth of the earthquakes, which gives us sort of a 3D view, which is different from a geologist looking at the surface. We can also determine the geometrical configuration of faults, and we can infer from that the forces that are at work and driving that particular earthquake sequence. In some cases, it's sideways motion, and sometimes it's vertical motion. In the case of vertical motion like the Northridge earthquake back in 1994, we can see uplift or changes in the landscape that are important to understand.
ZIERLER: Is the idea of crustal stress that it's a dynamic process, that it's always changing? Do you look at it in a historical perspective?
HAUKSSON: We do look at crustal stress over time and region. Now, we can get a fairly good idea, over the last 40 years, using the earthquake catalog that we have collected here at Caltech. There are other people who do modeling of what is called geodynamics, which is looking at things on a much larger scale than just Southern California, understanding how the whole Pacific Plate, for instance, is moving past the North American Plate. The plate tectonics process is extremely steady, and you can measure that with GPS, for instance, and determine what the speed of the Pacific Place versus the North American Plate is. But you don't see steady movement on, say, the San Andreas Fault, or on a detailed plate boundary. What we do see is suddenly, when there's s a magnitude 8, the plates jerk past each other maybe 20 feet or 30 feet, and then they settle down, and nothing is happening for the next 150, 300 years, and then suddenly there's another earthquake and it's a big step. So, understanding what is actually happening between those major earthquakes, which is the time period that we happen to be in now, since the last one was back in 1857—on the San Andreas Fault, the last big earthquake in Southern California—that ruptured the fault from Parkfield all the way south to Cajon Pass, or San Bernardino. We analyze data from both small and large earthquakes to understand, how are they changed the state of stress, and what is exactly the state of stress. Because if we don't have any earthquakes, we don't have measurements; if we have the earthquakes, we have measurements. That's also important. Using seismicity data from across southern California we can also map out where we expect sideways motion and where we expect vertical motion in big earthquakes.
Plate Tectonics and Continental Drift
ZIERLER: I wonder if you see the term "plate tectonics" as replacing continental drift, or if it is essentially the same concept as continental drift?
HAUKSSON: It's different. Continental drift was derived from looking at a map and seeing how Africa would match up with South America, and how some parts of Europe would match up with North America, including Canada. The plate tectonics is more of a process that is a more detailed look at things. You take into account what's happening along the plate boundaries. So, the Mid-Atlantic Ridge rift is spreading, where the two plates are moving apart. The North America plate is moving away from the Eurasia Plate, as an example. You can see that on land in Iceland, where the Mid-Atlantic Rift cuts across the island. There are various studies that have shown that the material is hot under the rift, and then it cools as it gets pushed away from the rift. Then there are of course all the deeper processes that other people deal with that I'm not involved in. But plate tectonics is the theory that we base all our work on, and has so far proven to be extremely useful to understand modern earthquake activity and the geology that we see at the surface.
ZIERLER: A generational question—when do you have a sense, in your career, when plate tectonics felt like a mature discipline? That the so-called plate tectonic revolution had happened, and it was the going theory for the field?
HAUKSSON: In the early 1970s, when I was working at the Science Institute in Reykjavík, Iceland, it was interesting, because there were some Russian scientists who wanted to do some field measurements in Iceland, and they seemed to be quite convinced that plate tectonics was not useful. They thought things simply moved up and down. That was their way of thinking of the world, while Icelandic scientists and others from the US doing measurements were into plate tectonics and all excited about how they could collect more data to improve the foundation behind the plate tectonic theory. That was in the early 1970s. Since then, plate tectonics certainly has grown and been accepted much more than what previously was called vertical tectonics.
ZIERLER: As a theory, it suggests that there are some parts of plate tectonics that have not been experimentally or observationally verified, that there are some things that are still up for debate, as you say. What are they?
HAUKSSON: That's a tough question here, for me. It's not so much that people disagree with plate tectonics or are debating whether it applies; it's more trying to put together all the details of how things happened in the past, and what was the chronology of the things that happened, and putting things in a holistic picture of how various processes start, like how a subduction zone initiates, how old faults are reactivated rather than breaking through solid rock and forming new faults, and things like that.
ZIERLER: I wonder if you can talk about the concept of extrapolation, in other words studying earthquakes locally but then being able to extrapolate those findings for a more global understanding of earthquakes.
HAUKSSON: If you record seismograms for an earthquake in California versus in Japan or in Europe, they more or less look the same. There may be minor regional differences. In the East Coast of the US, the geology is a lot more solid, the rocks are more solid, so the seismic waves will travel further without being attenuated. What we found is the power law distribution of earthquakes with size holds up globally, and also the decay of aftershocks with time holds up globally. So, there are various things that suggest that earthquakes are natural phenomena, more or less in my mind like topography, that you can find anywhere, almost, and it's a similar set of processes behind these natural phenomena.
ZIERLER: In looking for patterns in the earthquake catalog, going back 90 years, what does that tell us about the possibility that there is something cyclical about earthquakes?
HAUKSSON: Unfortunately, the historical record not quite long enough, because the expected average return time of major earthquakes on the southern San Andreas is 150 to 300 years. Here, we have 90 years of data, but ideally we would like to have 900 years, so we would be able to analyze numerous cycles to understand the details of the phenomena many times. Just like the meteorologist who predicts the weather every day, he gets a new chance at it and actually sees how predictions have gotten a lot better over time than what they used to be, and much more detailed and much more accurate.
Having said that, there are people who work in the field of earthquake geology who like to dig trenches across the major faults. By studying the walls of a trench, they can find cracks that come up from depth, and some of them don't go very far up, and others go all the way up to the surface. Some of those cracks they identify as being caused by an older earthquake, and that allows them to get information maybe back 2,000 or 3,000 years. They date those cracks in the trench wall by dating charcoal with carbon-14 methods, and that gives an idea of what has happened over the last 2,000 or 3,000 years. That's where the numbers 150 to 300 years comes from. But it still is not a detailed enough view to exactly say what's going to happen next.
ZIERLER: To square the circle, the idea that earthquakes are fundamentally unpredictable because they're chaotic, how might that work with a broader effort to determine cyclicity within a 1,000-year timescale? In other words, how can earthquakes potentially be cyclic to some degree but also are unknown how they start because of their chaotic nature?
HAUKSSON: It's not only unknown how they start. I can tell you that there's probably going to be 50 magnitude 1 or greater earthquakes in Southern California today, so we know 50 of them will start. Then, the question is, on any given day, which one of those 50 is going to become a big earthquake. That means it has to start near a big fault that accommodates the rupture. Then we have to figure out, where is this thing going to stop? That determines the length of the fault, the rupture, and the magnitude of the earthquake. So, there are all these aspects. If it starts in the south, say, down by Palm Springs, and ruptures north to Gorman versus rupturing the other way, it may pick different strands of the same fault, and that may result in different behavior. The US attempts at predicting earthquakes failed in the early 1980s, and since then the focus of the community has been more to accept the fact that we are going to have earthquakes, and rather predict the potentially damaging effects of earthquakes.
A lot of the data that we collect are measurements of ground-shaking. How hard does the ground shake in different types of earthquakes? That makes it possible to make maps to say, "Every 30 years or so, you will have these type of ground motions happening." That allows an engineer to design a building or a structure to withstand those ground motions. Also, we know which faults are likely to have earthquakes that may rupture the surface, so we tell people, "Don't build a building across this fault, because it's going to be two buildings at some point in the future." Those in my mind are very important things. Similarly, understanding liquefaction, where the ground may shake so hard that it becomes flowing mud, so any building built on that will topple over,. We have some good ideas where that might happen.
ZIERLER: Your work on what triggers an earthquake—what do we know about the different categories of triggers? Obviously, it's stress, but are there any other categories that we can consider a trigger that starts an earthquake?
HAUKSSON: The most obvious trigger is one earthquake triggering another. The most obvious example of that is aftershocks. A major earthquake like Ridgecrest, magnitude 7.1, will trigger thousands of aftershocks, and some of the bigger aftershocks will trigger their own aftershocks. Usually this happens nearby. Another thing that facilitates earthquakes happening, we think, is fluid, primarily water, being present in the rocks. If there is enough fluid in there, and an earthquake happens or earthquake waves go by, they may trigger other earthquakes. Now, sometimes humans do pump things into the ground, like fluids, and that can result in earthquakes being triggered, because you change what we call the pore pressure in the rocks, which may facilitate a fault to move. Before you pumped in the fluid, the fault was closed or clamped, but when you put in the fluids, you prop it open slightly, and that may be enough to allow an earthquake to happen.
ZIERLER: Your focus on stress triggering—what does that field look like? What are the kinds of things you're looking at?
HAUKSSON: For one thing, we're looking at how did the ground move in the main shock, for instance, that changes the stress around the earthquake fault that accommodated the main shock, which may be—like in the case of the Landers earthquake, was like 60 miles long. There, we saw at the north end, from the configuration of the smaller earthquakes and their faults, that the crustal stress seemed to have been depleted by the main shock, or the main shock stopped because there was no stress left in the ground to drive the rupture further north. Some of the data from small aftershocks in the region allows us to make the statement that, yes, the ground was almost moving backward after the main shock, so that indicates that there was no driving stress left in the ground there.
ZIERLER: Another technical question—I've heard of tomography. What is crustal tomography?
HAUKSSON: Crustal tomography is a method that uses data from recorded seismic waves to image variations in the three-dimensional speed of seismic waves in the crust. In the simplest global tomographic models, the Earth is thought of as being made of layers. The outermost skin of the Earth is referred to as crust. Below that is the mantle. Then below the mantle is the liquid outer core, and then the solid inner core. Using crustal tomography and other imaging methods, we are able to show that crust in Southern California ranges in thickness from about 22 kilometers up to about 46 kilometers. The variations in surface topography that we see with our eyes is about—Mount Whitney is—14,505 feet, so that would be about five kilometers. As opposed to the bottom of the crust has elevation variations of 24 kilometers. So, that topography is way more significant than the surface topography that we see. We use tomography to image where the crust is thin, often the mantle below is hot, and it's coming up towards the surface, like in the Salton Sea area. In other places where it's cold, like under the Sierra Nevada, the crust is thicker but some of it is in a state of gravitational collapse. The understanding of these processes from tomography is important, because for instance, in the Salton Sea area, the crust is actually spreading or in other words the crust is being stretched and thinned.
ZIERLER: Which means what? What does that look like, a collapse?
HAUKSSON: A gravitational collapse as we see in the high Sierra Nevada means it's spreading out, so the topography is going down, because its high elevation cannot be sustained—because the mantle below it is hot enough to keep pushing it up, but the crust is no longer in equilibrium with gravity being that high, so it spreads out north-south, east-west.
ZIERLER: A general question that relates to all of your research—what areas fall more on the theoretical side, and where are you more an observationalist?
HAUKSSON: I'm mostly an observationalist. I don't do a lot of theory. I apply theory that other people have come up with, but my focus is trying to understand datasets and ask the questions, "What story does this particular dataset tell?" Like when we had the Northridge earthquake, trying to understand what fault was involved; because there was no fault mapped at the surface, trying to understand the processes that generated the aftershocks and where did the aftershocks migrate to, and what was its relationship to the previous 1971 San Fernando earthquake.
ZIERLER: In applying those theories, what stands out in your memory? What are the most foundational theories that you've used?
HAUKSSON: I think the most foundational theory actually goes way back to a Japanese seismologist named Omori. He figured out the decay or how aftershocks became fewer with time. As a result, an earthquake sequence, he could say it was over in decades, or something like that, depending on where you are at. That's pretty foundational. Another one is creating the magnitude scale, which Charles Richter did here at Caltech. That was one of the many magnitude scales that were developed over time. Another theory that I've applied is the tomography, on how to map spatial variations in seismic wave speed within the Earth's crust, which is the outermost layer of the Earth. This method is very similar to what doctors use to map our brain, when they use the various types of scans.
ZIERLER: The value of the Richter scale today—when we hear on the news 7.1 or 8.2, are those numbers roughly in accordance with the scale as Richter developed it?
HAUKSSON: That's complicated. Richter was mostly worried about local earthquakes in Southern California, and he wanted to describe them accurately and compare them correctly. His scale looked at what we call high frequencies emitted by those earthquakes, which are the frequencies that we feel. But since then, various magnitude scales have been developed, including what is called moment magnitude, which Hiroo Kanamori, who is a professor here at the Seismo Lab, has been instrumental in developing. Those focus more on what we call long-period waves that we don't feel as much but nonetheless carry a lot of energy away from the earthquake source. Those tend to provide a much better estimate for the bigger events, so the moment magnitude is the one that we use for these bigger earthquakes. It was, at the time, calibrated towards the local magnitude scales developed by Charles Richter, but now we don't talk so much about the Richter scale; we just say the magnitude of the earthquake was 5.5, and that's simply our preferred magnitude, and we leave the technical details for us to worry about and don't want to burden the public with that.
ZIERLER: Because people know the name Richter, would it be more accurate if the news said it was a 5.5 Kanamori scale?
HAUKSSON: I don't think so. There have been several other people involved. I think we want to just leave it that the magnitude was 5.5, and that's it. There needn't be a name with it. I think that's true for a lot of other scales used in the sciences, that there's not necessarily a name that goes with it.
ZIERLER: Is there global agreement? Is a 9.2 here the same as it is in Iceland, the same as it is in Japan?
HAUKSSON: It is, yes. That's in part driven by the USGS, that operates the National Earthquake Information Center in Golden, Colorado. They collect data for all earthquakes around the globe, magnitude larger than 4 or 5, and they do systematic magnitudes, and their magnitudes are viewed as being authoritative. Having said that, there are similar centers in Japan and Europe that do the same thing, but they all apply similar methods, and their magnitudes are very similar. The funny thing about Charles Richter's local magnitude is that it's very hard to calculate or determine, even though it's a simple formula. However, the moment magnitude, no matter how many different people or whoever else you ask to calculate that, they all come out with more or less the same number. I have no idea why, but that's how it is.
ZIERLER: One of my favorite questions, because of the range of answers I've gotten so far, and it's as much a philosophical question as a scientific question—today if the orthodox view is that earthquakes are not predictable, that still leaves us with two choices. Either earthquakes are not predictable with the instruments, the theory, the technology that we currently have, or they're unpredictable because they're fundamentally unpredictable; the Earth itself doesn't know when an earthquake is going to occur, and so no advance in theory, instrumentation, or technology will get us there. What is your sense of what the correct answer is?
HAUKSSON: My sense is that the earthquake itself, when it starts, it has no idea how big it's going to be. It starts as a very small earthquake, and it just happens, in extremely rare cases, that the earthquake grows into a big earthquake. That may be almost impossible to predict—the size of the earthquake—with any accuracy. The other issue—where is it going to happen?—I think that's also pretty random. It just has to be the right earthquake at the right time, in the right place, and then it can grow. If we wanted to have technology that allows us to predict these things, I think that would be extremely expensive, and might require, say, a huge amount of monitoring that probably would never be cost-effective. We would have to get down there to eight miles where these things are starting, and really monitoring exactly what's happening down there.
ZIERLER: Given all of your interest in developing instrumentation and software, where ultimately do you hope it's heading, if you're pessimistic that it can neither tell us when an earthquake starts, where it starts, or how big the earthquake is going to be? Best-case scenario, what ultimately can technology do for us that it can't do now?
HAUKSSON: Technology can do earthquake early warning. Once we have acquired about four seconds of data since the quake began, we can tell you approximately how big it is going to be. We're not going to be able to tell you that it's much bigger than, say, 6.5 or 7, because it takes time for a major earthquake to develop. It takes a couple of minutes for a magnitude 8 to develop, and you don't know the final magnitude until that process has completed. So, I see it more that earthquake early warning will be the mainstay of providing extremely rapid information and allow people to protect in place and enable people to safeguard various things. It can be automated, and various automatic processes would be triggered to protect against ground-shaking.
The other aspect of it is basic research. It is incumbent on us, the current generation, to collect the best possible data for the earthquakes that we are having, and then in the future hopefully somebody comes along and is able, maybe in a few rare cases, to say, "Yeah, there is a pattern here that might apply." The fundamental issue here with our datasets, like with many other datasets, is that we can look backwards and say with a certain degree of accuracy, "This is exactly what happened there." Being able to look forward is a much more challenging problem. Maybe somebody will develop that as part of a different field, like computer science, and will allow us to make more progress on this particular problem.
ZIERLER: What are the most significant instruments that you've either innovated or refined, that get us closer to earthquake early warning?
HAUKSSON: I have not per se innovated instruments. I have been involved in deploying instruments that are available. I think it was a good thing that Caltech started out building all the instruments. Benioff in particular was the professor who headed up building new seismometers, and he was also a tinkerer, so he was always changing the response and the gain of the instruments, which made it very hard for scientists to interpret what they were looking at, because he didn't always tell them exactly what he did the day before the earthquake. Now, we have more standardized commercial-grade instruments, and those have allowed more studies of bigger earthquakes around the globe, and understanding the wave speeds not only in the crust but in the upper mantle beneath Southern California. Those instruments are important in that they capture a much broader range of frequencies than we had available to us before. That gives a more detailed picture, but still does not get us anywhere close to, say, being able to predict earthquakes.
I can push a little bit back on the panacea of predicting earthquakes. You see various phenomena being predicted, and people don't respond, they don't care, because it's not here now. I think somehow, we need to realize that what we really want is to be able to have infrastructure that can withstand ground shaking and withstand fault rupture. As an example, yes, we could send everybody for the weekend to Las Vegas to have a good time, and we can have an earthquake on Sunday morning in Los Angeles, and you wouldn't feel it, even, because you're far enough away. Then we could say, "Hey, yeah, come back on Monday when everything is rubble." [laughs]
ZIERLER: [laughs] Egill, what about on the software side? What are some of the things you've worked on in computer software that improve the prospects of earthquake early warning?
HAUKSSON: For instance, collecting the data from the field, we have a dedicated software that talks to your equipment in the field, that we call data loggers, and bring the data back very fast. We have our own software that transmits these data back and forth between different computers that do various processing on the data, identifying the earthquake waves as they pass by, and measure their sizes, which then is used by earthquake early warning to formulate or associate information into an event, and then send out the information that an event has started, and we expect potential damaging ground motion to be at your place in so many seconds, or tens of seconds.
ZIERLER: The term "real-time earthquake monitoring," what does that mean? What is real time?
HAUKSSON: Data collection and processing used to be, in the old days, that there was a drum recorder with photographic paper that recorded the data. Then once a day or every two days, somebody would go into the darkroom and take off the photographic paper, take it to a processing room where they would develop the record. Then it was visible to the human eye and somebody could measure what was on the record. That was a couple of days. But scientists who were working during that time period, they maybe wanted records from half a dozen places around the world, so they would have to write letters requesting records for the earthquake that happened two or three days ago, and that letter would go via a ship or some other slow method to, say, Europe, from the US, and then the people there, who had the record, they had to make a copy of it and mail it back. That was months to collect all the data.
Today, because we have digital technology, we have the internet technology, we have computers at every site that create digital data from the sensors that of course are analog, and those data are shipped out every second from each site, and they sample the sensor 100 times a second. Those data are then collected here at Caltech without any delay, and no human intervention. It's all automatic. Then identifying the earthquake waves and associating them into an event, calculating a location and a magnitude, and figuring out the configuration of the fault—no human is involved, and the information is sent out what we call near real-time. That, on our normal earthquake catalog, is like a minute and a half to two minutes. But earthquake early warning works much faster. There, you're talking about four to five seconds to get the information out to the user.
Early Warning and Safety
ZIERLER: A very real-time question—let's say that the earthquake early warning system is detecting something scary right now. Here I am, sitting in my office in Caltech, my wife is at home, the kids are in school. We all have our cell phones. Best-case scenario, what is the system going to do so that we come out of this okay?
HAUKSSON: Best-case scenario is that the system will have a location of the event within a mile or two of the actual location, that the magnitude that it comes up with is within, say, half a unit or so. It could say it's a 6, while it could be a 6.5 in actuality. In that case, if the event is within a well-instrumented part of the network, we should have the information within five seconds or so, the first information. There may be an update somewhat later. But that would tell you right away what's happening. But it's not quite telling you exactly what is happening where your wife is, or where your kids are. You can infer that from where the location of the event is. I would hope as the system evolves; we would simply send you a map that shows you what is the expected ground shaking across Southern California from this event, and do that within five seconds. We produce such a map today, and it takes about two or three minutes to do that, which is called ShakeMap. That's for a smaller earthquake. For a magnitude 8, it would take a little longer, because that earthquake has a longer fault, and it takes time to complete the earthquake rupture.
ZIERLER: As I'm getting these alerts on my phone, is it telling me what to do? Do I stay put? Do I run outdoors? What's the public policy response?
HAUKSSON: The public policy response is to stay in place and protect yourself. Charles Richter used to say, "If you feel an earthquake shaking, stay inside for about 15 to 20 seconds, and if it keeps on shaking longer than that, run like hell out of the building." Because his thing was that quite often, older buildings have brick facades that will fall off right away, and could hit people trying to exit the building when the shaking starts.
ZIERLER: That's still the going advice today, from what Richter said?
HAUKSSON: No, I don't think so. I think the advice today is, "Stay in place. Get under a table or something sturdy, a desk, and stay away from things that might fly around in a room." In the Whittier Narrows earthquake back in 1987, there was this bank down there, I think maybe a Wells Fargo bank branch, and this guy described the effects of the shaking in an elevator that was stopped at a floor. And on the floor of the elevator was this pair of red high-heeled shoes. He described that this woman got onto the elevator, and the earthquake was like ten to 8:00 or something, in the morning, and the door closed. Suddenly everything started shaking like hell, and a lot of water started pouring on her, because there was a water pipe in the elevator shaft. She of course was extremely traumatized by this whole thing, but somebody got her out of the elevator. Her office was on an upper floor, and there were glass walls in that office space to allow people to see through, and he described that the glass broke in those walls, and in the middle was her desk, and on her left side was the glass wall, and then on her right side, there were these shards of glass that had flown across the room and penetrated the wall on the far side. So, if she had made it up to the office, she would have been in this hail of glass, flying [laughs] across her desk.
ZIERLER: So, stay put. That's the moral of the story.
HAUKSSON: Stay put. Yes, exactly. [laughs]
ZIERLER: What you do with earthquake early warning, is that all in concert, in partnership, with the USGS? Or are there aspects that are unique to you specifically or to the Seismo Lab institutionally?
HAUKSSON: No, that's in concert with the USGS and other academic partners. Because Caltech is an educational institution, and it cannot be issuing public warnings, that has to be done by a federal or a state agency. So, the California Office of Emergency Services is also heavily involved, even though they don't have the technical capability of issuing warnings. Only the USGS does have that, and the USGS wants to cover multiple states in a coherent manner. As it is today, it is California, Oregon, and Washington state that get the warnings. We at Caltech have to be a little careful here, in that we are a private institution, so we don't want to give the impression that we are able to do something special or send out warnings, which might be risky business for Caltech itself.
ZIERLER: What about SCEC? Are you involved in SCEC, or are they a resource for your work?
HAUKSSON: They are. I've been involved in SCEC since the beginning. I was actually one of the people who contributed to writing the original NSF and USGS proposal for SCEC. I had worked at USC from 1983 to 1989, and the people there started getting the community together back in 1990 to form SCEC. SCEC has provided quite a bit of funding for my research, and a lot of collaboration has come out of working with various people involved in SCEC. This is a sidebar—I don't know if you know of Keiiti Aki? He was the first director of SCEC. He and Hiroo Kanamori are both Japanese. Keiiti Aki passed away a number of years ago. They have different backgrounds, sometimes interesting for somebody on the sidelines watching the different approaches to research. [laughs]
ZIERLER: What was your takeaway from those interactions? What did you notice?
HAUKSSON: Keiiti Aki's ultimate goal with SCEC was actually to predict earthquakes, while Hiroo Kanamori would never touch that subject, because he thinks it doesn't work. The whole goal of Keiiti Aki initially with SCEC was called the Master Model. That was getting every piece of data that we could find, both seismological, geological, and geodetic, into a big model, and try to refine that model so that we could predict what was going to happen next. This included in the beginning predicting the potential damaging effects of earthquakes.
ZIERLER: Given that you were involved since the creation, what was the impetus for SCEC? In writing the proposal, what niche would SCEC fill that wasn't possible either by federal agencies like the USGS or academic institutions operating on their own?
HAUKSSON: The National Science Foundation had a program where they were asking for proposals like SCEC. They were asking for universities to get together with their state and federal partners to formulate centers, because they were tired of funding individual investigators to do a little bit on a problem over here, and a little bit on a problem over there. It was disjointed. They wanted the community to get together and have a very well-organized and thorough program to study a problem. I think that is what drove it.
To provide practical results, the SCEC focus drifted towards mapping out the effects of earthquakes. A big part of that was of course understanding how the ground shakes, or what we call ground motions. That led to various incarnations of hazard maps. Then that, in the end, sort of upset the state people with the California Geological Survey, because they felt that was theirs. Then SCEC drifted more towards basic science, of collecting data out of trenches across major faults, and analyzing those data. They were also big in the beginning on understanding how to apply geodetic data more towards the earthquake problem. At that time, GPS was becoming a thing, so they got the Keck Foundation to fund a GPS network in Southern California. Later on, Tom Jordan, who was the director for quite a while, drove the idea of studying earthquake predictability. If somebody had an algorithm that could let them predict earthquakes, well, give it to a group of SCEC scientists who would implement it and be neutral, and just run it and see if indeed it worked.
ZIERLER: As a consortium, what can SCEC do that a single academic institution cannot do?
HAUKSSON: What SCEC can do is to bring much more resources to solve a problem. Instead of having one guy, say, in rock mechanics, they can have ten. SCEC can have more seismologists working on observational data. Instead of me and maybe another one or two professors here at Caltech, you could have 10, 15 people working on that problem, and working at various datasets, and comparing and contrasting, bringing together that much detailed and bigger picture, which you can do with a much bigger effort. Similarly, SCEC was able to have staff that would put together databases. Those databases are hard to do in a place like Caltech, because people will do the first version, and then they will say, "Okay, now that is getting boring. Let's figure out something else to do." Instead, SCEC would stick with it for five or ten years, and some of it, they stuck with longer. One of the things that SCEC has done, especially under Tom Jordan, was to go more for other funding sources within the National Science Foundation, to bring in what he called special projects that would allow him to use the various supercomputers especially funded by NSF or DOE—Department of Energy—to do various calculations of how ground motions propagated through the heterogenous Earth that we have, or heterogenous crust, that in part is based on my models.
ZIERLER: An institutional question, and also a counterfactual question—if you were at a different academic institution, perhaps Berkeley or MIT or Columbia, would you pursue different things? In other things, how different is the kind of research you're doing simply by virtue of doing it from within the Seismo Lab?
HAUKSSON: I don't know. For my PhD thesis, I collected data in Iceland and analyzed those, and that was the basis of my thesis. As a postdoc at Lamont, I ran a seismic network in Alaska, in the Aleutians, and I collected those data and analyzed them, along with other people. Once I moved to Southern California, I decided to focus on Southern California. The reason I decided to focus on Southern California was that I didn't want to be all over the place. I wanted to make a mark. If I was running around to Japan, Italy, or Sumatra or what have you, I would be pretty scattered, very fast. I figured I would just stick with what I knew, the dataset I was collecting, and try to make the best of that. There's plenty of other scientists who work with our data, and they don't have the inside track that I do, but they still manage to do good work.
ZIERLER: What have been the benefits of that focus, not having a broader research purview? What have you been able to accomplish as a result?
HAUKSSON: I think what I have accomplished is writing a number of papers that have illuminated what the earthquakes are telling us about Southern California. Where are some of the faults that cannot be mapped at the surface, like blind faults beneath Los Angeles? They have told us, what is the state of stress throughout Southern California? They have told us, what is the composition of the crust in Southern California, extended down to 40-plus kilometers in some places, but shallower at other places. They have told us where earthquakes are happening, on what faults. They have told us the stories of each of the bigger earthquake sequences that have happened.
ZIERLER: In looking in your whole research history, what earthquakes have been most useful to you? What have you learned the most from any given earthquake?
HAUKSSON: I think they all are up there. For instance, the 1992 Landers earthquake, it taught us that an earthquake doesn't need to really have a very big fault nearby. What it can do is daisy-chain together a bunch of small faults. In the case of Landers, there were six or seven strands of faults that were daisy-chained together to accommodate that big earthquake. A similar thing happened nearby in the Hector Mine earthquake in 1999. The Northridge earthquake was interesting in that it slanted into the ground opposite to the San Fernando earthquake back in 1971, and that was a big surprise. People had sort of postulated from the geology, but nobody had evidence that it was actually going to happen. The 2010 El Mayor earthquake just south of the border, it actually ruptured in two different directions, what we call a bilateral rupture, which we hadn't seen so clearly in Southern California before. It's fault also slanted in two different directions. The south segment slanted to the west, and the north segment to the east, which is sort of almost like a propeller-shaped fault, which is highly unusual to see. Then of course in 2019, the Ridgecrest earthquake had this very interesting foreshock sequence at an orthogonal angle to the main shock fault. That's something we had seen before back in 1987 in the Superstition Hills earthquake, and this was a nice second example, showing how foreshocks can develop prior to a bigger earthquake.
ZIERLER: As you mentioned previously, it's so important to enhance earthquake mitigation measures, like earthquake engineering, making infrastructure more resilient. Are you involved directly in any of those efforts?
HAUKSSON: Not really. I don't have time to be involved in those things. Certainly, the data we collect here at Caltech is being probably used in those efforts.
ZIERLER: What would be an example of data that you collect and analyze that you would then turn over to the engineers, to the specialists in infrastructure, to enhance earthquake mitigation?
HAUKSSON: Going back several decades, the engineers following the San Fernando earthquake back in 1971 deployed what they called strong motion instruments, which were in a way black box recorders that had film for recording data. There was a small trigger that would set the film running if the instrument was being shaken. It would record data for a minute or two, and then somebody had to drive around and collect all the film from the different instruments, and then develop the film after the earthquake before any analysis was possible. This technology has gone out the window, with Kodak going out of the business of making film [laughs].
Since today we have digital instruments and we are able to have two sensors at each one of our sites—one for detecting the smallest earthquakes or an earthquake very far away, like a 6.5 in Japan or something similar, and we also have a second sensor that collects engineering data that is on scale for magnitude easily 8 or what we call four times the pull of gravity, which is needed to ensure we record the biggest motions on scale. Those data are provided to the engineers through our website, through the California Geological Survey website. Our data get woven in with other data that are available on an earthquake like Ridgecrest, where people are mapping out the distribution of strong ground shaking from the earthquake. Actually, our data is the majority of data that is available for that particular earthquake. Thanks to earthquake early warning, our network has more sensors than ever have been in operation for real-time monitoring in southern California.
ZIERLER: With Kodak film no longer being relevant, is there any part of your research that remains analog? In analog in any regard preferable even when there is a digital option?
HAUKSSON: Not really. We don't have any analog instruments. The last Caltech instruments that recorded data on photographic paper were turned off back in 1992. Hiroo Kanamori and I decided that it was time to do so, because at that time, we had several digital instruments called TERRAscope that were on scale and operating.
ZIERLER: A few historical, retrospective questions about the Seismo Lab, given the length of your tenure. First, very simply, how has it changed over the years? What are the questions that people are pursuing now that they weren't pursuing 30 or 40 years ago?
HAUKSSON: The big thing that is happening now is, for instance, with deployment of satellites, is called InSAR satellite, that Mark Simons, who is a professor here, is involved in. He is also the chief scientist at JPL. I think that is a big part of it. Another thing that was not thought possible was use of what's called nodal arrays. You can get a seismometer with a recorder that is about the size of a Coke can, or a little bigger, or a soda can, and you can get hundreds of those, if not thousands, and you can deploy them very densely across an earthquake fault or across a sedimentary valley to measure the shaking within the valley. That's something that was not possible then. I think also given those new instruments we talked about before that can measure very low frequencies, which means they are very long wavelengths, we have much better estimates of the magnitudes of the bigger earthquakes than we used to have. Those uncertainties have been brought down.
When Don Anderson was director of the Lab, the focus was a lot more on global seismology. That was in part driven in my mind by competition with our colleagues at Harvard University. That led to various sort of global models of how the speeds of seismic waves vary throughout the Earth. That, I see less of happening now. It is more locally focused. Don Helmberger, he worked a lot on this exciting place called the core-mantle boundary, which is not a smooth boundary. It's boundary with a lot of topography, as I understand it. We have fewer people actually focusing on that nowadays. More people are interested in another technology, which is using optical fiber to detect earthquakes, which in itself is pretty challenging. It is an instrument that changes with time, and that's always tough data to deal with, but people are up for the challenge.
ZIERLER: The idea that we have turned away from the global modeling and now it's more local suggests a cycle in the sense that the origins of the Seismo Lab were very locally based. What does that tell us about the success or not of those global models?
HAUKSSON: I think it tells us that they are pretty good, even with the new datasets that people have, it's hard to get more out of the datasets until somebody comes up with a new technique, or until the ocean floors are better instrumented. About 70% of the Earth's surface is covered by water, or oceans. Those areas of the Earth have very limited instrumentation. But there are optical fiber cables or phone cables that run every which way across the oceans. If those can be used as instruments to detect earthquake data, then I think there will be a new push towards improving those global models, but I am not quite sure exactly when that will be.
ZIERLER: You mentioned JPL before. Is JPL a resource for your work? Do you have collaborations at JPL?
HAUKSSON: Not a lot, because they are mostly focused on planetary seismology, and the seismometer that went as part of the InSAR mission to Mars was developed in France, and the digitizer at the Institute for Electronics at the ETH in Zurich. We were only peripherally involved. We did have discussions with JPL people about various testing and so forth, but we were not involved in that particular mission, per se.
ZIERLER: In your collaborations within the Seismo Lab, what have been some of the most fruitful partnerships, where you brought a certain area of expertise, and another professor brought a different area of expertise?
HAUKSSON: That's a good question. Can I just get back to the previous question a little bit?
ZIERLER: Yes!
HAUKSSON: JPL worked with us in that they deployed some of their prototype instruments or prototype ideas of how to shield instruments from bad storms like occur often on Mars; that was done in collaboration with us. They deployed instruments at one of our sites here in Pasadena to test them out. We also worked with them at our Goldstone site, which is one of the space antennas that is out of Goldstone in the Mojave Desert. They were trying to figure out how to shield seismometers from winds, which blows as lot on Mars, I guess, much more so than on Earth.
Getting to your second question on collaborations, I think working with Hiroo Kanamori has certainly been extremely helpful, providing me with much better basic understanding of seismology. We worked on various papers where he has applied his skills to understand the geometrical configuration of the fault, and how much did the stress in the Earth change as a result of that earthquake; what we call stress drop. I have applied my understanding of how to locate the earthquakes more precisely to get a spatial idea of how they are distributed. I worked with Joann Stock, who is a professor here, on the Salton Sea area, which is a place in Southern California where the Earth's crust is being stretched, and the two plates are drifting away from each other, so that area is kind of opening up like a rift valley, although through geologic time, there has been enough sedimentation from the Colorado River that has filled it up, so there's not a large hole. Those have been interesting processes. I've worked with Zach Ross, who is a young professor here. He was my postdoc, actually, for three years. We've worked on implementing machine learning to do more accurate analysis of our real-time data streams and identify many more small earthquakes in our data. Those are just some examples.
Next Generation Seismo Lab Research
ZIERLER: Mike Gurnis has conveyed his enthusiasm for just how important Zach Ross's research is. I wonder, since you have a special view on that, if you can comment on some of the things he is doing.
HAUKSSON: I think it's all cutting-edge, and I think it's very important. We started out on a project where we identified what we call template earthquakes. We then ran those templates through the data streams to find earthquakes with similar waveforms, which had been missed during the original data processing. This new processing provided us with a catalog that had between 5 to 10 times as many earthquakes, depending on where you were in southern California. In a different project we used measurements that we had made here in the Seismo Lab since the early 2000s on when earthquake waves arrived in the different data streams. That was all measured by hand, by humans. We took all those measurements and Zach has then developed machine learning techniques of how to detect the waves and how to associate the waves for each event automatically.
ZIERLER: To invert a previous question about the kinds of things that are happening at the Seismo Lab now, very different to when you started—to flip that around, where do we see current research that would be recognizable even to a Benioff or a Frank Press? What are the kinds of things where, if they were here today, they could understand the question, and they might be interested in how little or much progress has been made on it?
HAUKSSON: There are basic things. I think they would easily see that, yeah, we are not using a compass and some simple tools like that to locate the earthquake. We see that it just happens in the computer, and it's very accurate, right from the get-go. Unless there happen to be several earthquakes at the exact same time; then, yeah, it takes a little to sort that out, but that's pretty rare. They would see that our magnitudes are much more consistent than what they had from the few records that they were reading for each earthquake. Maybe they had five or ten records if it was a medium-sized earthquake. Maybe they had a few more if it was bigger, but then it was all clipped, so it was very hard to make heads or tails of how the theory fit that data, because the bottom had been cut off, and the top had been cut off the data, so to speak. I think they would be very impressed at how many stations we are able to operate with more or less the same number of staff members that they had in the past. We do get along fine with our colleagues in Northern California; I'm not sure how that worked out in the old days, but I think it worked out okay. We are not that competitive, so we are able to share algorithms, share data, and that works pretty well.
I think they would be impressed by optical fiber and nodal arrays, what possibilities that would have. They would also be impressed by the dynamic range for the smallest motions that can be recorded and the biggest motions, on the same instruments. Maybe they'd have some ideas on how the Earth was deforming as it shook. I don't know if, when you talked to Jeroen Tromp, he talked about how the Earth shakes like a bell, after a major earthquake, so you get these different modes around the equator, where the Earth's surface is pulled in or out. Then you get another mode that goes up and down around the poles, and then various other modes that he describes in his research—he actually wrote the book on that theory. He was detecting that in his research, for instance, from the Sumatra earthquake back in 2004, I think.
ZIERLER: You mentioned data sharing. In the olden days, data, as I understand it, was much more proprietary. People would have to come to the Seismo Lab and look at Seismo Lab data. Are those days essentially over, between the internet and the broadening culture of geophysics and seismology?
HAUKSSON: Providing open data is one of the ways that the Seismo Lab provides leadership in the field of seismology. When Hiroo Kanamori took over as director, he made the policy that all data would be open data. Then we worked under that premise, so whenever an earthquake happens, our data is as freely available to everybody else as to us. We cannot use that crutch of saying, "Hey, the data is not calibrated. It's not ready yet for prime time," so then we get to work on it first. Instead, we have to have our ideas very quickly and move ahead with writing our research paper in a timely manner. I think that benefits us.
Now, there are other places that are not as free with their data. We have a hard time convincing some of our colleagues to exchange data with us. When we do exchange data, for instance, with our colleagues in Ensenada, in Baja California, it helps us both. They ship us some of their data real-time, and we ship quite a bit of our data to them real-time. The whole point here is to—all of us, we don't want to look like fools with incomplete information in front of—the media. If there is a big earthquake down in Ensenada, yeah, we want them to have the necessary data so they can do a good job. If we don't have their data, we might be pulling the epicenter of that earthquake, into our network. It's very hard to locate an earthquake that occurred outside of a network, because the data are collected where the stations are, and the location algorithm first guess is that the quake is occurring nearby to the first station that reports detecting seismic waves. If we don't share data, we might look like fools, and similar with them. Then there are colleagues who say, "Well, we want to have our data and write out papers first." This approach has a limited benefit nowadays, because there are so many freely open stations across the globe, so in the case of an earthquake of any large size, researchers can grab those data and make a story out of it.
The Seismo Lab, as well as the seismological community at large, are part of what is called IRIS, Incorporated Institutions for Research in Seismology, which now is being merged with UNAVCO, which is a data center for geodetic data and images collected via satellites. Both groups are university/government consortiums that advocate open data exchange, and we ship some of our data directly to IRIS for their public distribution. We also have tools that if people want more of our data, they can actually automatically get more of our data from more stations. I think that's all good.
In Europe, they also are somewhat behind the times on sharing data. People feel like they have devoted too much effort to collecting their data; they want to have first dibs at the data. But then the data often never sees the light of day for other people, which I think is too bad. One way to look at this is that Caltech and USGS in Pasadena, we get all this free research done on our data, all this analysis and all this synthesis, and we don't have to do it ourselves, some stuff that we would never think of but might be interesting.
ZIERLER: I can see right on its face the scientific benefits from Hiroo's decision to make all of the data free and open. To flip that around, would the science be improved at all, or would it better serve the Seismo Lab, if the data was more proprietary, in other words if you had a chance with your colleagues to work with it first before releasing it into the wild?
HAUKSSON: No, I think the other way around. Nowadays, a lot of science is done on Twitter, after the earthquake, so people are throwing all sorts of theories and plots. I think it's better that people can use our data and at least have reasonable theories, as opposed to having very limited data and throwing around all kinds of theories that make no sense. I think the Seismo Lab has to take the high road, that we are good at our science. We are not trying to get preferential treatment by having collected the data. We make it available to everybody. Yeah, we are going to compete, on even footing, and I think we do quite well. That's what makes the Seismo Lab in a way the Seismo Lab—that we are not trying to put barriers in front of other people; we are rather just moving ahead with our own science.
ZIERLER: The last topic I'd like to touch on today, and it will serve as a bridge to our next discussion, is more of a cultural series of questions. For you, coming from Iceland, do you think that you have a unique appreciation or approach to geophysics and seismology, just given all the things that happen in Iceland?
HAUKSSON: I might, yeah. It makes sense in that I felt smaller quakes in Iceland, and I saw volcanoes and geothermal areas. There are almost no trees there, so you can see the geology, and you can understand how it forms, and you're aware of volcanic eruptions, how new material is coming up to the surface. I think that has provided me with a good foundation of understanding how these processes happen.
ZIERLER: Is it close to you? In other words, do you see the world through Icelandic eyes at this point in your life, do you think?
HAUKSSON: [laughs] No, I don't think so. I think I see it more through Southern California eyes.
ZIERLER: You've gone native, at this point.
HAUKSSON: I've gone native, and I've thawed out! I can't take the cold weather anymore.
ZIERLER: The ultimate test.
HAUKSSON: Exactly. No, I spend a lot of time around here hiking with my son and traveling throughout Southern California and seeing the geology, and all the different earthquake faults out there. I think that's fantastic and that makes it a different playground, but there are some similarities between the two places.
ZIERLER: On that note, in our next discussion, I'll look forward to going back to Iceland. We'll see how many generations back we can go, and ultimately we will bring that conversation right back to the present.
[End of Recording]
ZIERLER: This is David Zierler, Director of the Caltech Heritage Project. It is Thursday, September 15th, 2022. I am delighted to be back with Professor Egill Hauksson. Egill, once again, a great pleasure to be with you. Thank you so much.
HAUKSSON: Thank you, David.
ZIERLER: In our first conversation, we took a wonderful tour of your overall approach in geophysics and seismology. Today, we're going to go back many generations, as far back as you can go in Iceland. Let's start first with you, though. Your name, Egill Hauksson, what does both your first and last name mean or suggest?
HAUKSSON: That is a good question. My last name is a patronymic, so my father's name was Haukur, and I am his son. We have four cases (nominative, accusative, dative or genitive) for names in Iceland, in Icelandic. The genitive version is Hauks, so I'm Hauksson. My sister would be Hauksdóttir. My first name, that comes from an old Viking who was around in the ninth century. He was somewhat of a brute, so the name reflects that. He was probably a terrible person, liked to run around other countries and kill people and pillage things that were not his.
ZIERLER: Does the name mean something?
HAUKSSON: I think it means something, like "the terrible one" or something like that. [laughs]
ZIERLER: But your parents had no concern naming you that?
HAUKSSON: I don't think they were thinking along those lines. I think there may have been a person that they knew who had this name, and they may have wanted to remember that person, so that's why they picked the name.
A Millennium of Icelandic Heritage
ZIERLER: Tell me about genealogy in Iceland, how it's even possible for you to trace your family back so many generations, centuries ago.
HAUKSSON: Of course, Iceland became Christian in the year 1000 or thereabouts. The old Vikings decided that they would rather become Christian, and belong to that group of people, and that would make it easier for them to communicate and have commerce with other countries. As a side bar, at the time when they were trying to decide whether to become Christian or not, then some person came running up to that gathering, which was happening in a rift zone, which is called Thingvellir, which means "The Althing" which was the original parliament. Some person came running over there and said a couple of tens of a mile away, there was an eruption. Hot lava was pouring out of the ground. They said, "The old Norse gods must be angry now that we are going to abandon them." That's how the story goes. Then some wise guy said, "We're sitting on lava here, so what were they angry about when this lava flowed?" [laughs]
ZIERLER: [laughs] That's great.
HAUKSSON: So, on it went. Actually, Egill Skallangrímsson, who I am supposedly related to, going back 27 generations, he wanted to apparently go there [to attend Althing] and—as the story goes, his son found him with his chest of gold coins at the farm, and he was ready to take it to Althing, and his son asked him, "What are you going to do with this there?" The old Viking looked at him and said, "I'm going to stand on the highest point above the crowd, and then I'm going to throw the gold coins at them and watch them tear each other apart as they try to get a hold of those gold coins." [laughs]
ZIERLER: Have you been able to trace, throughout the generations, particular crafts or professions that your family specialized in?
HAUKSSON: To finish the other thought, the records on genealogy were kept by the church, and those were fairly accurate records, even though there may be some difficult times, like volcanic eruptions or the plague going through the country and a lot of people would wander about looking for food or a way to subsist. Still, the records are fairly accurate. Icelanders are big on writing. They wrote the sagas. They somehow liked to do that, and they wrote it all in Icelandic. They in fact had all of their church services in Icelandic, even though in Europe, all of the early Middle Ages church services were all in Latin. In Iceland, they got away with it, because even if a complaint was sent to Rome, by the time the message got to Rome and got back to Iceland that they shouldn't do such a thing, then the person who was accused of doing a sermon in Icelandic was already dead! It took so long. So, they got away with it.
Getting to your next question about my family, my family on my father's side was in the printing business. My great grandfather started a printing shop in Reykjavík, which is the capital of Iceland. He studied apparently in Europe and made his way to America to study printing and had his own print shop in Reykjavík. Then in the late 1880s, he decided he wanted to emigrate to probably Canada, where most of the Icelanders went. They went to Winnipeg. But he only made it to Scotland, because at that time, his wife was pregnant, so they got turned around and sent back to Iceland. They didn't want pregnant women on the boat, I presume because they felt they wouldn't be safe. He then died towards the end of that late 1890s. In 1929 my grandfather restarted the print shop and also bought a house in downtown Reykjavík for the print shop and his residence. It is a two story house, so they had the print shop on the first floor and they lived on the second floor. I actually lived in that house some of my teenage years. When my grandfather died in the 1931 my father took over the business, and he was 19 years old or so. He kept it going until about the 1959, when there was a huge change in technology, and he didn't feel like there was enough business to totally retool the print shop.
ZIERLER: What about your mother's side?
HAUKSSON: My mother's father was a laborer on farms. He would talk about how he had to do his work in a certain way, like he would maybe travel to Reykjavík to get various supplies, and he would have horses to carry the supplies, probably around a distance of, in some cases, up to 50 or 60 miles, but he was not allowed to ride the horses; he had to walk with them. My father talked about this, too, that fishermen who lived in Westmannaeyjar, an island to south of Iceland, would fish cod and haddock, and they would hang it up on sort of scaffolding for drying. Once the fish was dried and salted, they would tie it onto pallets, and take the pallets up to the South Coast of Iceland, which has all these black beaches, and it's very hard to land a boat on those beaches, because there's quite a bit of surf, and it's very gradual, how the coastline rises up. They would just dump these pallets overboard and have them drift on shore. People ate this fish, because that was the only good protein around for some parts of the year, but it was fish filled with sand, so that was pretty difficult to eat. Most Icelanders in those days, prior to World War II were pretty poor.
ZIERLER: Talking with your parents, the previous generation, what was their experience during World War II? Were they largely isolated?
HAUKSSON: No, not at all. Of course, the Germans came to Iceland before World War II, just before it started, and wanted to make agreements with the Icelandic government about having some kind of a military base in Iceland, but they got turned down. Then the English invaded Iceland in 1940. People were poor, they didn't have a lot of money, but they got by. Then in 1942, the US Navy replaced the English or the British Army, because the British Army went to North Africa to fight the Germans, but the US Navy wanted to maintain the base to ensure safety of ships sailing between Europe and the US.
ZIERLER: When the British invaded, is that to say that Iceland tried to maintain its neutrality and wanted nothing to do with either side?
HAUKSSON: Well, I think it's more like they were not invited. They didn't even ask to be invited. But I think Iceland was okay with it. They preferred the English over the Germans. But yes, I think the feeling was they wanted to be neutral, but it was always clear that that was not going to work because Iceland is so small. They didn't have their own navy or army or whatever.
ZIERLER: Were any men from Iceland pressed into military service?
HAUKSSON: Not that I know of. Some may have volunteered, but I never heard talk about that. There were just not that many people around. I think at that time the population may have been 100,000 or something less.
ZIERLER: Where did your parents meet? Was it in Reykjavík?
HAUKSSON: They met in Reykjavík. That's where both lived at that time. With the US Navy coming there, I think there was more demand for goods and services, so the society did better. I'm sure Icelanders were hired to help raise Quonset huts and build various things that were needed to maintain an army on the island.
ZIERLER: In talking about your family, I haven't heard much in the way of science. Are you really a pioneer in your family in that regard?
HAUKSSON: I think so, yes. I'm the first one who said, "I want to go to college, and I want to go on and get a higher degree if I can." That was always my goal. My parents and even my siblings—even though my older brother ended up getting a MBA from Columbia University, there was not that much interest in science. It's something I guess I picked up in high school or what have you, and got convinced it's what I wanted to do.
ZIERLER: Growing up, what kind of opportunity is there to just be wondrous of nature, in Iceland, that might have spoken to you?
HAUKSSON: There's lots of them. For one thing, when I was there—it's maybe slightly different now—the whole island was deforested. When the Vikings got to Iceland it was forested, and they let out their sheep, and the sheep, in wintertime, when they couldn't get to the grass because there was snow on the ground, they would eat the bark of the tress, so the trees died. I presume they also cut down the forests for firewood. For me, it was always great to get out and see things, because you can see fissures in the ground in places not too far out of the capital, which is part of the rifting that goes on as the North America Plate moves away from the Eurasia Plate. You could see volcanic eruptions, and there were some major eruptions, like what was called Heimaey, which is off the south coast of Iceland, had an eruption. Then there was Surtsey which was a new island that formed off the south coast in the 1960s. When I returned from college in Norway in 1974, there was the big eruption in North Iceland, which was mostly not aerial but most of it, at that time, in 1974, was what we call intrusive activity, in that the magma flows laterally into the rift zone without coming up to the surface, which is somewhat similar to what happens sometimes in Hawaii. So, there's lots of opportunities. I also felt a number of earthquakes.
Actually when I was in high school and gymnasium, I did have some jobs—most teenagers had jobs at that time, because the construction season, for instance, is fairly short in Iceland, so there was seasonal demand for workers, and why not use the teenagers boys hanging out to do some useful work, like cleaning nails out of boards that need to be reused. That was reasonably well-paid, so I did that. Then when I went to college, I worked for what was the Iceland Energy Authority. It also conducted geothermal research, so I was out there in the field most days in the summer, pulling out long electric cables and putting an electrode in the ground. I would walk a mile in one direction, and another person a mile in an opposite direction, and then somebody in the middle would connect the wires to a machine that would put some current into the ground, and we would measure the resistivity of the ground. If there was hot water under there, the resistivity would be somewhat lower.
ZIERLER: Growing up, what were some of the major family traditions, the cultural things that you remember in your household?
HAUKSSON: The main things were Christmas and Easter, even though Icelanders are not religious people. When I was growing up, the church was part of the government, so all the clergy were employed by the government. People sort of took it for granted. They would get baptized in church and confirmed and married and buried. Many of us, including myself, wouldn't go at other occasions to church. But still, all the religious holidays were taken seriously, like Christmas was an important time to gather. There was Christmas Eve, Christmas Day, and Boxing Day.
ZIERLER: What did the feast look like for Christmas? What kind of foods were on the table?
HAUKSSON: There might be a smoked lamb, mashed potatoes, green beans, things like that, and various vegetables like carrots and so forth. There might be ham roast. Of course there was plenty of candy to go around.
ZIERLER: What about any vestiges of the Viking origins, the Pagan origins of Iceland? Did any of that translate down by the time you were growing up?
HAUKSSON: Regarding Christmas, the day before Christmas, a lot of people liked to cook stingray, which is also called skate. My mother always hated it because it smelled up the house. That's a very interesting fish. You've probably seen it in aquariums and what have you. It has cartilage for bones. I like it. Whenever I see it on a menu in a restaurant, I will order it. But regarding Pagan festivals, there is something called Thorrablot, which is usually in February. It is another food festival, that is sort of saying, "We made it through the worst of winter without consuming too much of the food we hoarded to make it through winter, now we can go ahead and eat quite a bit more of it, because we are going to make it until spring." There was all kinds of preserved food, which would be—again, smoked lamb. It might be shark that was buried in the ground to make it ferment, because shark has urine in its meat, and it needs to be fermented out before you can eat it. There might be deviled eggs. There might be herring that has been pickled, which you can buy in a store in Solvang here, in California. [laughs] A pickled herring sandwich. Also, tongue. There used to be a restaurant here on Lake in Pasadena that was of Scandinavian heritage, and they would serve tongue sandwiches. The only other place that I've noticed people eat tongue is Japan, in Hokkaido. Of course there was fish that had been preserved by drying it and salting it, and things like that.
ZIERLER: The fish was Christmas Eve and the meat was Christmas Day?
HAUKSSON: The fish was eaten the day before Christmas Eve. On Christmas Eve, there was a big meal, and then the big meal was on Christmas Day. The other tradition was Easter. Iceland would more or less close down for a week for Easter. You could hardly buy a Coke can. I never understood why, because people didn't really go to church. They just wanted time off, I guess. There, even the fishermen wouldn't go out fishing on Good Friday. If you did that, you were just asking for bad luck. So there was some superstition. Of course, there's a known superstition in Iceland that when you're building a road, and the road has to go through a big boulder, you don't go blowing that up; you just make a bend in the road, because the elves live in the rock, and if you start trying to blow it up, bad things are going to happen to you! I think that has probably come from the fact that you can read many stories of how people got lost in sudden snowstorms or sudden bad weather. So maybe a brother or a family member was lost or something, and then they needed to have some way of saying, "Yeah, that person was probably picked up by the elves," so they were okay.
ZIERLER: Do you have anything to share about gnomes and "Nei takk"?
HAUKSSON: Not really. The elves were sort of it. But "Nei takk,"means ‘no thanks'.
ZIERLER: When I was in Iceland, I was told that when you see one of the garden gnomes, you're supposed to say "Nei takk."
HAUKSSON: Oh, I see. "No thanks"? Okay. [laughs]
ZIERLER: Oh, that's what it is? "Nei takk" is "No thanks"?
HAUKSSON: Yeah. [laughs]
ZIERLER: [laughs] Because what are you saying "no thanks" to?
HAUKSSON: I'm not sure. I didn't think the gnomes were offering you anything, but maybe they were trying to offer you something or trying to trick you.
ZIERLER: Maybe something got lost in translation; no idea. Egill, I asked about the Viking roots, the Pagan roots of Iceland, and if any of that filtered down to your generation.
HAUKSSON: Not so much. Though there are people nowadays who sort of view it as being important. Like I've been to a witch's wedding in California, and I think there are some people who, instead of being Christian, view them now as being part of the Pagan tradition in Iceland. But I'm not exactly sure how that works for them.
ZIERLER: Tell me about the schools you went to growing up, starting with primary school.
HAUKSSON: With primary school, I went to a public school in Reykjavík. That's pretty similar to what is here, except we did start learning Danish fairly early on, because Iceland was part of Denmark. I don't know if you know that the independence battle of Iceland was fought by writing letters to the king of Denmark. I must make an effort at some point of trying to read some of those letters, because they must have been really nasty letters. [laughs] The king of Denmark gave up on holding onto Iceland in 1912 or so, and said, "Yeah, you guys can have your independence." In 1912, the agreement was that we would share a king with Denmark, and they would handle our foreign affairs. That was going to be valid for I guess 30 years. In 1942, the Icelanders wrote a letter to the king and said, "We don't want this anymore; we want to have our own president and have our own foreign affairs." That is when Iceland completely split away from Denmark. Now, Greenland was somewhat different. It became basically a county or a state within Denmark. They had members of Parliament in Copenhagen, capital of Denmark. Iceland never did that. Iceland always viewed itself as being somewhat a separate country from Denmark, even though they relied on Denmark for commerce.
ZIERLER: Is part of the difference—are there indigenous peoples in Greenland that are not part of the Icelandic population?
HAUKSSON: That's correct. There are the Eskimos in Greenland. Even though Greenland was settled from Iceland, then by the 12th century when people went back looking for the Nordic people who had settled, they were all gone. They couldn't be found. There were various theories about what happened to them. That may be a part of it. It may also be that the indigenous people didn't quite have the wherewithal to write letters to the king of Denmark to state their case.
ZIERLER: In middle school and high school, when did you start to gravitate academically towards math and science?
HAUKSSON: I think it was more in high school. At that time, we had something called gymnasium, which was common in Europe. That was the last two years of high school and an additional two years. There, I gravitated very much to science, and studied physics and math. Even though I was on a science track, I still had to do English, German, French, and Latin. I don't speak any of them except for Icelandic and some English. The other languages, we learned how to read and write. They didn't have language labs in those days so it was harder to teach people how to actually pronounce the language. You really had to travel to the country if you were interested in, say, German, and spend some time there to learn how to properly speak the language.
ZIERLER: What about cultural influences, either from newspapers or from television or movies? Where were cultural influences coming from in Iceland?
HAUKSSON: They were mostly coming from Europe. Iceland associated with the other Scandinavian countries, viewed themselves as being a prominent member. Denmark, Sweden, Norway, Finland, and Iceland were viewed as being the Scandinavian countries that had sort of their own set of cultures that differed from, say, Germany and England. But then the Navy base in Keflavik, which was part of the relic from World War II as well as later NATO—Iceland joined NATO, I think in 1949—it had a TV station. There was a culture and influence through that TV station, because people could buy a TV and an antenna and point it towards the Navy base. At that time, Iceland didn't have their own TV station. This was in the early 1960s, I think. By the late 1960s, Iceland had its own TV station. Icelanders are very proud of their language, in that they try to translate or use old words for new things. For instance, telephone is sími, and there are many words like that, that they have. Like a company like Google or Facebook would be called netrisi. There are lots of things that they manage to translate fairly well.
ZIERLER: When it was time to start thinking about college, first of all, what kind of support would your family be able to provide you?
HAUKSSON: Nowadays, you hear about helicopter parenting in the US, that the parents are always two steps ahead of the kids.
ZIERLER: I might be one, for that matter! [laughs]
HAUKSSON: My parents were busy with their own jobs, and they always looked at us, the kids, and said, "Well, if you want to do that, go and do it, and don't bother me with it." I wanted to make sure that I would make it into college. I was supposed to go to a nearby high school to my house, but I felt like that was not a good school. I wanted to go to a different one which was a bit further away, so I asked for an appointment with the principal and asked my father to go with me to support me. I think both he was surprised and the principal was surprised that I would do this, and the principal accepted me going there. To be accepted at the gymnasium I had to spend a whole year taking classes, and then at the end of that, there was a daylong exam that would decide whether you got to attend the gymnasium [a prerequisite for college] so to speak. The 4 year gymnasium school was an important foundation for attending college.
ZIERLER: The gymnasium accords with which grades, roughly?
HAUKSSON: It's roughly 11 and 12, plus two more years. That means that when I went to college, I was 20 years old, as opposed to in the US, a freshman in college would be 18.
ZIERLER: At 20 years old, what are your options? Do you know that you need to leave Iceland for the next stage of your education?
HAUKSSON: I didn't have to leave Iceland, but I had had this certain set of teachers at the gymnasium, in math and science, and I was looking towards having the same teachers at the University of Iceland in college. That was one concern that I had. Another one, I wanted to get out of Iceland. I looked at going to the US, but I talked to a number of people who had studied in the US, and they said, "Well, why go there? It costs a lot of money. You can go to a Scandinavian university— Denmark, Norway, or Sweden—and it doesn't cost you anything." Because the tuition was free. I decided on Norway because that was a very well-known engineering school. I wanted to do applied physics, and they offered a program in that.
ZIERLER: What kind of career were you envisioning at that point, with a degree in applied physics? What would you have been able to do?
HAUKSSON: When I finished that or was about to finish that, I realized that in Iceland at that time, most people who had a degree in physics either did geophysics or they did politics. I decided politics was probably not for me [laughs], so I ended up going to geophysics. That also allowed me to spend a lot of time outside, which I thought was great. I liked being out there, even if it was wet or cold. It was something that got me to see nature and hang out and think about things.
ZIERLER: What was it like? Was this your first time away from the country?
HAUKSSON: Well, I think we had made one trip when I was a teenager with our parents to Copenhagen for a couple weeks to just see what it was like abroad. But yes, this was my first time on my own. No, it was the second time. I went when I was in the gymnasium one summer to England, to try to improve my English at some summer school in the south of England, so this would have been my second time going abroad. It was okay, because I had studied Danish in high school, and Norwegian is similar but it's easier to pronounce. The vocabulary is similar. Slight difference in spelling, but that was nothing that was very serious.
ZIERLER: By your accent, could people tell immediately that you were from Iceland?
HAUKSSON: Yeah. There's no such thing as Norwegian; there are like 400 dialects. Norwegians with a good ear, once you have said a couple words, they will be able to tell from what fjord you are, and if you're from the mouth of the fjord, middle of the fjord, or the back of the fjord.
ZIERLER: [laughs]
HAUKSSON: So, yeah, they had no problem telling where I was from! [laughs]
ZIERLER: Going to school, did that make you feel more connected to Europe, not just obviously geographically but culturally? Did you just feel closer to things?
HAUKSSON: Yes, definitely. It opened my eyes that, yes, there was a different culture and different lifestyles. The Norwegians are very friendly people. They go out on the weekend on Saturday or Sunday for a hike in the woods, and you get to see them there with their children. I got to know some of my fellow students and meet with their families. I felt very comfortable there, and they are very accepting of Icelanders. Also, their traditions were somewhat similar to Icelandic traditions. The food wasn't too different.
ZIERLER: Did you go back for the summers? Did you take the opportunity to tour around Europe?
HAUKSSON: No, I went back for the summers, because I had a well-paying job of doing the resistivity measurements for the Energy Authority in Iceland. Most of the time I went back to Iceland to work and make money to pay for my living expenses in Norway.
ZIERLER: Tell me about the curriculum in Norway, the kinds of things you studied.
HAUKSSON: [In response to the previous question,] I did make one trip, which was driving down the West Coast of Norway all the way down to Denmark. That was quite enthralling, because they have all of these deep fjords, and you drive in and out of the fjords. They also have these very steep roads that have these like 180-degree turns, so you're coming back from where you came. That was quite exciting. What was your second question?
ZIERLER: I asked about the curriculum, the kinds of things you were studying.
HAUKSSON: It was mostly math and physics that I was studying there. That was viewed as being an essential part of physics. There were some electronics that we had to do as part of labs. I did take a course in organic chemistry, and that was probably the toughest course I took, because that's a lot of memorization. I happen to have a son whose undergraduate degree is in molecular biology and organic chemistry, and he's great at organic chemistry. I don't think that has anything to do with me. [laughs]
From Norway to New York
ZIERLER: Did you have any exposure to seismology or geophysics during your time in Norway?
HAUKSSON: I did, yes. There was one class that I took in geophysics, but not so much seismology there. It was mostly looking at what is called potential fields, like gravity, electricity and magnetism.
ZIERLER: Did that class set you on the path to pursue geophysics later on, do you think?
HAUKSSON: I don't think it was that class. It was more like the summer work that I did in Iceland, and realizing that this was fun work to do. I also worked with a group from Columbia University that was studying the rift zone in Iceland near where these recent eruptions have been happening, Fagradalsfjall.
ZIERLER: Was that the initial connection to Columbia, in Iceland?
HAUKSSON: It was, yes.
ZIERLER: Tell me about the project, how Columbia got involved in this.
HAUKSSON: They were interested in figuring out the details of plate tectonics. Iceland is unique in that it has the plate boundary on shore, just like the San Andreas is on shore in California. So, you can walk across the plate boundary, and you can put instruments on the plate boundary. They put out seismometers, and the goal was to record as many earthquakes as possible during one summer season, and then analyze those earthquake data and see how the smaller earthquakes were spatially and temporally related to [tectonic] activity along the plate boundary.
ZIERLER: Tell me about the discussions that led to your enrollment at Columbia. How did that come about?
HAUKSSON: It's mostly I applied, and I applied for a couple of other universities, and I got in at Columbia. There was not a lot of discussions, per se. I decided I wanted to go to the US to get a PhD, and Columbia was of course one obvious place because they had played such a prominent role in plate tectonics and developing all that theory. These people, of course, were part of that, so that was quite important. There was also a colleague in Iceland who had graduated about the time when I left for Columbia. I had been talking to him, too, to get an idea of what the place was like and what to expect.
ZIERLER: What was it like for you when you landed in New York City?
HAUKSSON: [laughs] Imagine a guy who is used to cold weather, who really has never seen a mosquito or any sort of significant number of insects. He gets to New York City, or lands at JFK, and the temperature is about 90 degrees, and 90% humidity. Where I was coming from was maybe 45 or 50 degrees and 30% humidity. Then somebody picked me up at the airport, and dropped me off at this house that was actually a barn built in the late 1800s, and the barn was sort of the central part of it, and then on both sides, somebody had added on like five or six bedrooms. I got one of those bedrooms. When I started to try to talk to people, of course they were all New Yorkers, and only used their four-letter words, so it took me a little while to figure out how to handle that!
ZIERLER: You were conversational in English already at this point?
HAUKSSON: I think so, yes. I had been talking to people who visited Iceland and only knew English. Of course, the other big thing was the sound of the insects. That was totally new. That was the huge difference.
ZIERLER: What about just the diversity of people, all of the different cultures?
HAUKSSON: Yeah, sure, that was an important thing to understand. But I've always felt comfortable with different cultures. I like people being different. If we are all the same, it's not very exciting. I think it's a much more fulfilling life if you interact with people from different cultures and get to know them and understand what their goals are, and what they have accomplished so far.
ZIERLER: Administratively, did you spend your time on campus, or were you mostly at the Observatory?
HAUKSSON: I was mostly at the Observatory. We would occasionally take a bus from the Observatory down to campus for things like registration, which was the weirdest thing for me. You would run from office to office, to finish your registration for the semester. I mostly forget what it was all about. It was about what classes you wanted to take, and you had to fill out forms. You had to go to someplace to get that approved. Then you had to go and talk to some guy who was collecting the tuition and convinced him you weren't going to pay him anything. [laughs] Stuff like that.
Columbia is on the sort of south edge of Harlem. One of the things we got told was that you never go into the Morningside Park, which is on the north side of the Columbia campus. The other thing they told us was, "If you have to take the subway up to the George Washington Bridge, don't get off anywhere in between." But we sometimes did take the subway to catch a bus. If we missed the regular Observatory bus, then we had to catch another bus, which was a public bus. That meant riding the subway up to the George Washington Bridge, getting on that public bus, and it would take us up to the Observatory and then keep on going further north.
ZIERLER: Thinking about the plate tectonic revolution that happened 10, 15 years before you got to Columbia, what aspects of it felt like a mature discipline, and what was still being debated about plate tectonics at that point?
HAUKSSON: That's a very broad question. I think people had pretty much by the time I got to Columbia worked out quite a bit of it. Like these things go, there's a kinematic description that I can provide you via this conversation. The Earth's surface is covered by a dozen plates, and we approximately know where the boundaries are. At that time maybe we knew 80% of the boundaries, but maybe 10% or 20% still needed to be refined to figure out exactly where they are.
But then the next step that people were thinking about then, and are still somewhat working on, even here at the Seismo Lab, is the dynamics. What is the energy of the system, and what drives it? How is it driven? That is still up to debate. At the time that I arrived at Columbia, people were discussing, when the ridges in Iceland open up to form the grabens, those things being pulled apart by the gravity slide of material away from the ridge, because the elevation of the ocean floor is pretty high right at the top of the ridge, and then it falls off with distance away from the ridge, as the material cools. Then there was this question about, what's the role of the slabs that go under Japan, or the Aleutians. Or on the west side of the Pacific, like in Tonga, are those slabs of materials of the Pacific Plate, are they pulling on the ridge, so as to open it up? That was still being debated at that time. What was the role of the mantle? Is the whole mantle convecting, or is just the outer shell of the mantle moving to drive this system?
ZIERLER: Administratively, was seismology considered a unique discipline within Lamont, or how did that work?
HAUKSSON: Seismology had its own building. It was viewed as an important part of things. To some extent, the strength of Lamont was to have all of these different disciplines there so people could converse and put together the bigger pictures. Lamont at that time was running two oceangoing ships, if I recall correctly, that were collecting data all the time in different parts of the world. Then the seismology group was operating networks, in the Aleutians, in Pakistan, in South America, and previously in Tonga. These were all collecting data, and people were more trying to collaborate and talk about how to put these datasets together along with the ocean surveys that were done by instruments towed by ships across the oceans.
ZIERLER: Coming from Norway, what aspects of your previous education left you well prepared at Columbia, and where were you playing catch-up?
HAUKSSON: What left me well prepared was the math and the physics that I had learned in Norway. Where I was catching up was on the geology and tectonics, because I hadn't had a geology class. Suddenly, there was the expectation that I could put my seismology research in the context of what faults were there in the area, and how did the geology come to be, and what were the processes that generated that geology that we were looking at, at the surface today. Fortunately, I was able to take classes at Columbia to fill that void in my education.
ZIERLER: What was the process for developing a. thesis topic, or perhaps first, who would be your thesis advisor?
HAUKSSON: That was an interesting thing. In 1974, the Chinese predicted a big earthquake in Manchuria. I don't know if you talked to Lucy Jones about that earthquake, because she studied it for her PhD thesis. The Chinese claimed after that they had predicted some more earthquakes. So, there was a lot of interest in the US—"Wow, the Chinese know how to do this, so let's do it." The USGS got some funding from Congress. One of the professors at Columbia who became my advisor, Lynn Sykes, got some funding along with Wally Broecker, who is the known carbon expert on carbon in the oceans that you might have heard of. They had this grant that they wanted me to work on and go to Iceland and measure radon emission in geothermal water. I sort of said, "Well, I wanted to learn more seismology; I didn't want to go into geochemistry." But since this got me back home in the summers—so I said I would do it, and I started working on it. I had monitoring stations in—had people collect for me water samples from different geothermal wells in Iceland. Then the samples were sent to the Science Institute in Reykjavík where I had a data analyst who analyzed the data.
ZIERLER: What were you finding? What did the data tell you?
HAUKSSON: I lucked out. I did find an anomaly, increased emission of radon before a couple of earthquakes of magnitude 3 or 3.5 in South Iceland. That was a nice example showing, yeah, this is actually going to work. But it's sort of one-off, so it's one of these things that once you have a one-off, you should just quit. [laughs] That's enough! But I kept measuring, and I didn't see other anomalies in other places.
ZIERLER: Which tells you what?
HAUKSSON: Which tells me that maybe that was a fluke. Maybe that place had some special plumbing in the ground where the plumbing was more sensitive than other places. Then I had also been measuring radon at this volcano in North Iceland called Krafla that had the eruption in late 1974, or intrusion. There, the caldera would subside, just like we saw in Hawaii a year or two ago. I measured radon there in a geothermal well, and it showed that the flux of radon changed when the caldera floor subsided. That was a nice confirmation that there was something in the tectonics that changed the emission of radon as tectonic events were happening. But once I graduated from Columbia, I decided, well, in order to be able to predict earthquakes, we need to either do one or the other. We need to put a lot of energy into understanding the physics of earthquakes, which we didn't understand too well at the time. Or, we need to start understanding radon a lot better—What does it do, and how does it do it?—in terms of being emitted from rock and transported through various fissures in the ground to a place where you can measure it. Since I knew seismic instrumentation and not many people were into it, I figured there was that opening there for me to get into earthquake monitoring or seismic networks, which are instruments that are collated together into a group, and the data are collected at a central place.
ZIERLER: Did this inform your thesis, ultimately?
HAUKSSON: This did not inform my thesis. There were two parts to it. One was the radon measurements showing the correlation with earthquake activity, and the other one was showing the correlation of the radon measurements with the volcanic activity. Then I had a third aspect to it, which was displacement meters which I put across some of the fissures in the rift zone in North Iceland where intrusive activity of magma was happening, and being able to show that those fissures open up at the right time and how much they open up as the magma flowed underground.
Mitigating Earthquake Unpredictability
ZIERLER: Tell me about developing what ultimately did become your thesis.
HAUKSSON: My thesis became that earthquakes are unpredictable, but we can mitigate their hazards by carefully mapping out their potentially damaging effects. Once I graduated from Columbia, Lucy got offered a postdoc there. We both graduated in 1981. We graduated in June, and we got married in September.
ZIERLER: What was Lucy working on at that point?
HAUKSSON: She was working at Lamont in the rock mechanics lab. We also had a project in China, both of us, going to look at their earthquake precursors in Chengdu. That was in April of 1983. We left Columbia in like October 1983 to relocate to the West Coast in California. She was working in the rock mechanics lab trying to understand friction and things like that, when you slide two blocks of rocks past each other. She had also started some work on foreshocks at that time. At Columbia she also continued her thesis work on foreshocks. As part of my Postdoc at Columbia I volunteered to become part of a project which was running a seismic network in Alaska, out in the Aleutians, a place called the Shumagin Islands. You fly into Anchorage, and then you get on this propeller plane, and you bump around for three to four hours or something, in the rough Alaskan weather, and then you land in this airport called Cold Bay, which is just one strip and a shack, more or less, with a couple of bathrooms. I walk into this one bathroom, and I look at the wall, and lo and behold, there's graffiti on there in Icelandic!
ZIERLER: [laughs] What did it say?
HAUKSSON: I don't remember, it was something that the person didn't like this place or whatever.
ZIERLER: [laughs] What about you? Did you like it?
HAUKSSON: I liked it, yeah. At that time, when I went up there, our project was winding down, because the NOAA-funded environmental studies were coming to an end. The oil exploration and the oil production were picking up steam. We did actually have a helicopter to help us out, so we didn't have to drive very far. This helicopter was provided by NOAA and the pilots would fly us from place to place. One of the things we did was to clean up from previous field work. If you can imagine, there's an island out in the Pacific, and 300 feet up on the top of the island, there's a very sharp ridge, which basically comes to a nice edge on top, like cliffs sometimes do, and there sits a helicopter, the blade running, and then I am there running back and forth to the helicopter with batteries that weighed like 30 pounds each and loading them on the helicopter, because we were instructed to remove all of them. [laughs]
We had seismic station on the side of this volcano, and the helicopter pilot lands, and then the two of us get out—the senior field engineer and me as a field assistant—and then the senior engineer says, "Here's the gun in case a bear shows up, because I'm going to have my head down in a hole in the ground"—[laughs]—"and it's your job to shoot up the bear if he shows up, because I don't want him to eat me." I had only one training session, the only training I've had to fire a gun, with him, a couple of days before. He had shown me how to work the gun. Then as we were trying to finish up, the helicopter pilot comes over and says, "Well, it's too windy, we have to take off, so finish up." [laughs]
ZIERLER: What came of that research project?
HAUKSSON: I published several papers on it. One of them was to describe the plate boundary as the Pacific Plate is subducted under the Aleutians. The earthquakes define that quite well. Shumagin Islands is kind of in between the rupture zones of two major earthquakes that happened decades earlier. Actually in the last year or two, there was a big earthquake there. We all hoped it would happen while we were working on that project, but it didn't. It happened 40 years later. Another aspect of it was—there's a lot of talk about slow earthquakes now in the Pacific Northwest, that the plates seem to creep a little bit at the downdip edge of the brittle interface. We actually caught such an event in the Shumagin Islands on a leveling array, myself and my colleagues. That resulted in a paper in Science Magazine that is long forgotten, but I remember it. This was the first-ever such event measured by instruments.
ZIERLER: Who was on your thesis committee?
HAUKSSON: Whoa! [laughs] Lynn Sykes was on it, and Roger Bilham, and a gentleman from an upstate New York university. I forget his name. I could look it up. Maybe I should pull out my thesis and write this down. I don't remember exactly who else. There might have been one more person there. I'm not sure if Wally Broecker was there. I doubt it, because he was always such a busy person.
ZIERLER: What would you say the main conclusions or contributions were from your thesis?
HAUKSSON: The main conclusions were that radon might work as an earthquake precursor. That if you did a careful job and you had a good plumbing system, that there might be some things that happen before earthquakes. Even though later in life, I have decided that maybe that's not the best conclusion, that it's just too complicated of a system to actually use that for anything useful, like predicting earthquakes. The other conclusion was from the rift zone, showing how radon emission changed with the caldera floor dropping as magma was injected into the rift zone, and being able to actually document and record how quickly the fissures and the rift zone opened up as the magna was intruded down below. So, understanding those processes in more detail.
ZIERLER: By the time you finished the dissertation, was the option to stay on as a research scientist at Lamont already wrapped up at that point?
HAUKSSON: Yes, it was. I had been talking to people there at Lamont. I didn't want to return to Iceland at that time. Lucy thought it maybe would be okay, but I realized that we wouldn't be able to find two jobs there, because there simply weren't enough jobs to go around in that small of an academic community. I wanted to stay in the US. I had been to California, and I think I had always wanted to go to California, and Lucy is a fourth-generation Californian. I had had discussions with Dr. Klaus Jacob, who was in charge of the Alaska network, and his colleague, with last name Davis. His colleagues had already left the project by that time, so Klaus needed somebody to work with him on wrapping it up. That was basically my main goal during my Postdoc project.
ZIERLER: When you were a research scientist, were you by this point firmly in seismology specifically, would you say?
HAUKSSON: Yes, I was.
ZIERLER: What were some of the big questions that were happening at Lamont at that point, and where did you slot in?
HAUKSSON: Some of the big questions were, how did the plates actually move in detail, and what was the energy budget of the system. Where was the energy coming from, and how was it being used to drive the plates, and what was the role of the different layers in the mantle? I slotted in where we had this great network that actually was deployed across the Shumagin Islands, which extend out over the subduction zone. Usually there's quite a bit of distance between where the oceanic plate start subducting and the island arc on the far side. In this case, we had islands that went almost out to the trench, so we had much better monitoring of the earthquakes on the plate interface where the major earthquakes originate. If you think about the motion of an oceanic plate, like the Pacific plate, it collides with the Aleutian Island arc and subducts. As part of the subduction process it has to bend to go down into the Mantle. As it subducts, it doesn't keep bending, but it straighten itself out, forming a slab. In many cases, you get what is called a double Benioff zone, which is earthquakes in the upper part and the lower part of the plate caused by the bending and unbending stresses. That's a term that comes from Caltech, which was from Professor Benioff who worked on it here. We were able to get a much more detailed picture of that, and understanding how this process takes place, and how it is related to the volcanic activity occurring along the island arc.
ZIERLER: What were some of the technological advances with instrumentation or computer that allowed you to do things obviously that Benioff never would have been able to do?
HAUKSSON: I think the big technological advantages were that we had much better data. Benioff would have been using data from a few stations placed maybe 100 kilometers apart. Here we had stations 20 kilometers apart, and the data was all transmitted real time on radios into a central hub, and at that hub, we recorded the data, so there was no question about the timing of the data, since it was all coming in real time, just like as if you're listening to several different radio stations on the radio, in the same place.
ZIERLER: How did this work contribute to some of the bigger questions in seismology at the time?
HAUKSSON: I think it contributed in that people wanted to understand how this part of the Aleutian chain accommodated earthquakes, as compared to the areas of the Aleutian chain further to the west, or how the 1964 Alaska earthquake ruptured the plate boundary further to the east. It was trying to connect these two zones of plate boundary together.
From USC to Caltech
ZIERLER: Tell me about transferring over to the University of Southern California.
HAUKSSON: At Columbia I worked quite a bit with Roger Bilham, who was later at the University of Colorado in Boulder. He was an instrumentation guy, and a very exciting, and interesting person. He always had a new gadget every day, almost. I got to work with him on carbon fiber strainmeters, and that got me to Venezuela, and got me to California.
ZIERLER: What benefit does carbon fiber play for these meters?
HAUKSSON: It has a very low thermal coefficient of expansion, so even though the temperature changes where this instrument is installed, it doesn't deform or provide a spurious signal like some other materials might. It provides a much more accurate measurement. On my trips to Southern California, I had gotten to know people at University of Southern California, because they were involved in measuring accurately the wave speed across the San Andreas Fault from Bouquet Reservoir, if you know where that is, across the San Andreas, into about five miles across the San Andreas. They wanted to see if there was any change in the properties of the rocks as the plate boundary forces were loading the fault. They were doing very accurate measurements to a tenth of a percent or so, at that time, but they didn't find any changes. Here was the Icelandic guy, told to go out with this instrument, and there was this goldmine up in the hill, where we would have to shovel some dirt and pour some cement to install the instrument. Then we put in a big steel door. Of course, some of the locals found it and had to use it for target practice, but that's how it goes. [laughs]
The strainmeter installation in Venezuela was out of Lamont. There were some graduate students from Venezuela at the time at Lamont. There was one guy. He wanted us to put some instruments in Venezuela, even though he was not directly involved in the end. I was conscripted into going there. In Venezuela, there's the Boconó Fault that I'm sure Clarence Allen studied. It's a huge strike-slip fault like the San Andreas, but it goes through a mountain range. People live on the north side of the mountain range, but it rains on the south side. The Venezuelans wanted to get the water from the south side over to the north side, so they decided to dig a tunnel. They got halfway in with that tunneling machine, and then lo and behold, the tunnel has shrunk behind them. They had a lot of technical problems.
They also had what we called access tunnels near the fault zone, extending into the main tunnel. Those access tunnels were very close to the main fault. Roger's idea was that if we could get a strainmeter at depth very close to the fault, maybe it would see amplified solid Earth tides. The softer the material, the bigger the Earth tides are. If the material or the rocks are very firm, the Earth tides are smaller. This has to do with how elastic the material is. This access tunnel that I was working in was sloping into the ground at 30 degrees, and it was about eight feet wide. The trucks that would go up and down the tunnel were about seven feet wide, so whenever a truck went by, I had to step out of the way and pull in my stomach and hope that they wouldn't scrape it off. [laughs] In between the trucks passing by, I installed the strainmeter in this tunnel.
The installation worked okay, but for data recording we had a chart recorder in the security hut, and there was only one outlet in there. The guys in the security hut, they had a coffeepot that was plugged into that outlet. We had forgotten to bring an extra extension cord that would have allowed us to plug more than one thing into that outlet. I think shortly after I left, they unplugged my chart recorder that would record the data, and plugged in the coffeepot.
ZIERLER: It's tough! Which one is more important? I don't know. [laughs]
HAUKSSON: So, we never got much data out of that one! But it was an interesting experience.
ZIERLER: Tell me about how, just by being in virtue of being in Southern California, that shifted your focus to seismological issues in Southern California.
HAUKSSON: I always had the attitude that I would work with the data that I collect, because it's right on my desk, and I know most about it. I know more about it than anybody else. USC, at the time when I started there as a postdoc and later as a research professor, they had a small network in the Los Angeles Basin, which they had in part gotten funded to find possibly induced or anthropogenic seismicity in the Baldwin Hills and in the Long Beach Oil Fields. They had also added a few more stations at the time of the San Fernando Earthquake back in 1971. So, they had ad unique dataset there that I worked with. I also worked with the data that had been collected by the Caltech network at that time, for the L.A. Basin, and I studied earthquakes in and around the Newport-Inglewood Fault and out in Santa Monica Bay, at that time, and the Whittier Narrows earthquake in 1987.
I was quite excited, working in a new place. I had gone from Iceland to the Aleutians, and now I was in Southern California. There was so much known about the geology so that makes it quite exciting to be able to look at the earthquake data and figuring out the consistencies and inconsistencies and explain what's going on. One of the big things that came out of that was that I was able to map the actual configuration of the blind faults that run under Los Angeles.
ZIERLER: With all of the data you were working with, did that give you an opportunity to look at historic earthquakes, earthquakes that happened decades in the past?
HAUKSSON: Yes. One of the earthquakes that I did look at in more detail was the 1933 magnitude 6.4 Long Beach earthquake. It's rupture actually started in Huntington Beach, in the oil field down there, and ruptured up to the south boundary of the City of Long Beach. It caused quite a bit of damage within Long Beach city or Long Beach itself. Studying it was quite an experience, because all that data was recorded on photographic paper at eight different Caltech seismic stations. Along with the photographic paper seismogram, in those days they would record Morse code from a radio on the same type of drum recorder, and use the timing of the Morse code to time the seismic data, to figure out when the seismic waves arrive at each seismic station. That's necessary information for seismologists, because that's the information we use to triangulate back to find the epicenter of the earthquake.
ZIERLER: When you were named Research Professor, did that give you opportunity to mentor graduate students, teach undergraduate classes, and things like that?
HAUKSSON: Since I'm on a research track, I don't teach classes. That's the big difference at Caltech between professorial faculty and research faculty. When I was named research professor, it was more because Caltech decided to change their nomenclature for these positions. I went from being a research associate to senior research associate. At the time when I was named research professor, they simply changed those titles.
ZIERLER: But didn't you have that title at USC as well?
HAUKSSON: I did have the research professor at USC. They were more liberal. Caltech is always more conservative in how they name things. It's just internal politics that I'm talking about here. [laughs]
ZIERLER: As research professor at USC, on the research side, did that mean you also did not teach at USC?
HAUKSSON: That's correct. My role there was to run the Network and work with some graduate students. There were students that mostly were somewhat on their way, and they were floundering around, and I would pick them up and get them going on their projects, help them out, keep them moving forward.
ZIERLER: The network you were running, was that proprietary to USC? What was the consortium?
HAUKSSON: It was proprietary to USC, even though they did share some of the data with Caltech.
ZIERLER: What was the geographic reach of the network?
HAUKSSON: The reach of it was the greater L.A. Basin, so it would reach from, say, south in Orange County, say Irvine or something like that, or Laguna, all the way up to the Santa Monica Mountains, and up towards Pasadena, and out to Montebello. It's the greater metropolitan area, which is extremely important for understanding earthquakes and earthquake faults. Here is one observation for you. If you think about the L.A. Basin, it's a bowl that's filled with sediments. If you were to shovel out all the sediment, you would get down to a depth of about 30,000 feet. Then you would hike into that hole, and then you would look up to Mount Wilson, and you would see a mountain that's 36,000 feet tall. That's the height of Mount Everest or so. There's a lot of topography in the region, which people tend to forget about. How that all has come about is quite an exciting story.
ZIERLER: I asked about historic earthquakes. What about the opportunity to study earthquakes in real time, as they are happening? What was that like for you in the 1980s?
HAUKSSON: In the 1980s and even into the mid 1990s, we did pretty well on real-time reporting for smaller earthquakes. We were able to detect and report on magnitude 3's or so. But once the earthquake got any bigger, we didn't have computers big enough, and the data pipes got clogged with too much data. So, we always fell behind if we had a magnitude 5 or bigger. That made it take a longer time to get the information out. The Landers earthquake, which was a magnitude 7.3 back in 1992, it took about an hour to get information out. In Northridge, in 1994, we had been clever and put in some filters on our data, because we had big noise spikes if our microwave dropped out. When the Northridge earthquake hit, it created signals very much like one of those microwave spikes, so our computer software filtered out the actual data as a microwave noise spike. It took us another 45 minutes to figure out where the earthquake was located and how big it was.
ZIERLER: In our last conversation, I asked generally about earthquake prediction. Here, rooted in the chronology, in the 1980s, was anybody still hopeful that this was achievable, or that was pretty much done at that point?
HAUKSSON: Lucy Jones may have talked to you about this, that she put sort of the nail in the coffin of earthquake prediction research in the U.S. Because once we got back from that trip in China back in April and May of 1983, I think, she was talking to a reporter and said, "Well, the Chinese are just guessing, and if you guess that there's going to be an earthquake every day, you're eventually going to be right." That made my advisor quite mad at her, for doing this to his research program. But I think in a way, in the long run, people have come to accept that it's extremely hard to predict earthquakes. And what are you going to do? Are you going to send everybody to some safe place? If somebody predicts an earthquake in LA for Sunday then we'd send everybody to Las Vegas on Saturday, and then they come back on Monday and the whole place is a pile of rubble?
So, seismologists have gotten much more into trying to predict the effects of earthquakes. How strong will the ground shake, and how often will it shake that strong, and where? They provide maps of that, that can be used by engineers and land planners, and similarly provide maps of where earthquake rupture is going to come to the surface, and is likely to damage the house, split it in two, and what have you. That's more the focus. The whole issue of earthquake prediction is very tough in that the earthquake usually starts at a depth of six to ten miles, and even if you're standing on top of the epicenter, you're trying to measure something that's very far away from you. From all we've learned about earthquake prediction and all the efforts, the initiation zone is quite small. It may be ten meters across or something.
ZIERLER: What does "initiation zone" refer to?
HAUKSSON: Where it starts, where movement on the fault starts. It's not like the whole fault moves in one go. It has to start at a point, basically. That point, you might describe as being a few meters across, or ten meters or whatever. Then it grows and grows. One way to think of this is, say, a big earthquake on the San Andreas that runs for 150 miles, maybe 10 miles of the fault are moving at any given time. The beginning of the fault may have finished moving, but the remainder of the fault still needs to move to complete the earthquake. It's like if you have a carpet on the floor of a ballroom, and you need to move it over three feet, then what you do is you put a wave in the carpet and move the wave across. You don't pull on the carpet, because then you're just going to tear it. That's how we think of earthquakes. Some people refer to it as the Heaton pulse, invented by Professor Tom Heaton at Caltech. He was the one who first espoused this theory. Prior to that, people thought there were just these two humongous blocks, 100 miles long or whatever, and they would just jerk past each other suddenly as the earthquake happened.
ZIERLER: From within USC, were you part of the conversations, the policy considerations, that led to SCEC?
HAUKSSON: Very much so. I was there at the time, and I was part of writing the first SCEC proposal to the National Science Foundation and the US Geological Survey. That was a very interesting and exciting time, to realize that my colleagues and everybody was going to get together and have a coherent, uniform program that was coordinated amongst the different universities and the federal and state agencies.
ZIERLER: This will foreshadow to our next conversation when you move to Caltech, but did discussions with the Seismo Lab or just being at USC get you more involved with Caltech simply by proximity?
HAUKSSON: Yes. One of the things that I did while I was at USC, I would come up here to Caltech in the dead of night. I was at USC during the day, and then I would go home and have dinner, and then I would go to Caltech and go into the basement here and haul out a bunch of magnetic tapes that had Caltech data on them. Then I would sneak those down to USC and read them down there to flesh out my dataset. I think Caltech people must have known that I was doing this. [laughs] They knew of my papers, knew of my research, and had seen me at meetings. They realized they needed somebody to run the Network and I was running the USC network. It had its ups and downs, and now suddenly it was running and providing data. I think both of those things contributed to my visibility at Caltech. I refer to it as sort of breaking and entering into the data vault here. The guy who was employed by the USGS, who was mostly in charge of the network at that time, his focus was the software development, and he was very good at that. He developed one of the first relational databases that he used for the earthquake data. Such databases became very common about ten years later. He didn't really want to spend time on providing data to researchers. I figured it out.
ZIERLER: Last question for today. Was it a particular person who invited you to the Seismo Lab? Was there an open position you applied to? How did that work?
HAUKSSON: There was an open position that I applied to, but I did get encouragement from a number of people, including Hiroo Kanamori, Tom Heaton, and Rob Clayton. They all made sure that I was welcome. Also, at the time, Dave Stevenson I think may have been GPS chair, or at least an interim chair, and I had a number of conversations with him. That all worked out well. I was quite excited and enthralled.
ZIERLER: What was compelling to you? What was exciting about transferring from USC to Caltech?
HAUKSSON: If you are just running the corner store and you suddenly get invited to be a manager for Walmart— [laughs]
ZIERLER: Well put. On that note, we'll pick up next time with the start of your career at Caltech.
[End of Recording]
ZIERLER: This is David Zierler, Director of the Caltech Heritage Project. It is Friday, September 23rd, 2022. It is great to be back with Professor Egill Hauksson. Egill, once again, a great pleasure to be with you. Thank you so much.
HAUKSSON: Same thing here.
From the Analog Era to the Digital Era
ZIERLER: Today we're going to start right when you joined the Seismo Lab. Set the stage for me. The late 1980s, the early 1990s, first of all, what were some of the big projects with regard to the Network? What did you have to do? What were the priorities?
HAUKSSON: The priorities were, in a bigger picture sense, to move from analog instrumentation to digital instrumentation. You can think of analog as your old hi-fi stereo. If you turned the volume up too high, the music would be distorted. The same thing happened with our instruments. If the earthquake shaking was too big, it would be recorded as distorted data on these old analog instruments. What we wanted at that time was to acquire and install digital instruments that would always be on scale no matter how strong the ground shaking was. That started out with a project called TERRAscope. When I arrived at Caltech, the first station that acquired digital data was here in Pasadena and had been operating for a year or so. The next step was to raise funds to install additional digital stations. This new equipment also provided real-time data that required modernizing the data processing algorithms, which was important at that time as well as today.
ZIERLER: What exactly were the technological developments that prompted the shift from analog to digital?
HAUKSSON: Now I need to think back about what was happening in the real world. I think cell phones were just coming out, so that was one aspect. Another aspect was that computers were getting better and were able to process more data than previously. For example, the PC was coming online. The idea was to add a digitizer to essentially a PC to enable it to collect data that way. The internet was just starting, with email and simple things like that. The idea was, "Hey, can we use it for actual digital data?" In fact, at the time, our phone company that was then called Pacific Bell and later got bought up by AT&T again—it was a split of the old AT&T, Pacific Bell—they donated digital data communications in the 1990s to just have a project that demonstrated that yes, indeed you could do digital data communications very successfully.
ZIERLER: Within the field of seismology, either at the Seismo Lab or other institutions, were there researchers who were really clamoring for this technology? Did they recognize what the digital revolution could do for the field?
HAUKSSON: Yes. A lot of people in our field had written theories about how to interpret data from big earthquakes in near real-time, but there was very little good data from big earthquakes, because most of the regional seismic networks were tuned to catch smaller earthquakes, which were quite frequent, but a once-in-a-20-year big earthquake, they handled very poorly, because everything was off-scale. People were clamoring for these new data because they wanted to be able to prove their theories and show that these big ground motions fit the theory. We could derive a lot more information about the earthquake if we had data on scale. We could figure out its geometrical configuration, the size of the surface that moved, and how much it moved. That would better allow us to connect what happened in the earthquake with what the geologists were seeing at the surface where they would see new scarps forming because of the earthquake.
ZIERLER: For these digital instruments, what aspects were off the shelf, and what needed to be created or retrofitted specifically for the Network applications?
HAUKSSON: The sensors were off the shelf in this case, because a certain company in Switzerland had decided that this was priority, and everybody was clamoring for their sensors, because in part they would measure very long-wavelength waves very accurately. Those long-wavelength waves are only radiated by the biggest earthquakes, so to understand the biggest earthquakes, which is say magnitude 8.5 and bigger, these waves are very important. The digitizers, or what we call data loggers—it's a combination of a digitizer and a computer, in a way. The computer pre-processes the data and then sends it on near real time to a destination. That was made by a company in Boston. So, we had these pieces, but we had to marry them together, and do calibrations to make sure we were getting good data. We had to install them correctly, so we had to dig a hole in the ground down to in some cases eight feet, put in a culvert, and make sure there was a stable bottom in this culvert, which usually was a plug of concrete. Then we'd install a sensor at the bottom, and a data logger towards the top of this vault. Then we'd insulate it so that we didn't get too much disturbance from temperature changes in the atmosphere above the thing. Then we had to make sure that of course they didn't get flooded, because then we would lose our instrumentation, so many of them had sump pumps, too, if they got flooded, to pump out water.
ZIERLER: You mentioned globally why the digital technology is so much better. What memories stick out in your mind where, with this new technology, and an earthquake happens, it's just better across the board—the analysis, the real time. What are your experiences early on with this technology that demonstrated this superiority?
HAUKSSON: The first earthquake in Southern California where we were able to demonstrate the value of this technology was the Landers earthquake back in 1992. It was a magnitude 7.3 earthquake that chained together several smaller faults to form one big fault to accommodate the 7.3 for a distance of about 60 miles. In that case, Hiroo Kanamori here at Caltech was able to analyze these new data—we had data from six new TERRAscope stations—that provided a lot more detail and a comprehensive view of what happened on those faults, how much each one of them slipped, and how that energy was radiated through the ground from the fault surface. Also at that time, and in the next couple of years, until between 1992 and 1995, we started using these new data to map out the ground shaking. That led to the first innovation to build what's called ShakeMap today, which is a product that is now provided by the USGS on a routine basis for all earthquakes greater than 3.5 in the US. ShakeMap is extremely useful because it provides you much more information than, say, knowing the magnitude. It provides you the distribution of where ground shaking was strong, and where it was weak. That is very important for emergency responders to know where they should be focusing their search and rescue efforts.
ZIERLER: This technology, what excitement or what optimism did it induce in the beginning about really fundamentally understanding earthquakes in a better way?
HAUKSSON: I think the excitement came from being able to study events, like in the Landers earthquake in 1992, and Northridge in 1994, and Hector Mine in 1999—these were all big earthquakes that had caused significant damage in Southern California. There was a lot of excitement that we understood them a lot better than, say, previous earthquakes like the San Fernando earthquake back in 1971. There was also quite a bit of work done at the time, too, to try to understand the composition of the crust in Southern California, the wave speeds—where they were fast, and where they were slow, and how that correlated with the geology.
ZIERLER: Tell me about your responsibilities. What aspects of your work were really about the Network, and what aspects of your work were being a research fellow, a part of the Seismo Lab faculty?
HAUKSSON: My responsibilities regarding the Network was to provide leadership. We had several staff members working on the network. In the 1990s, it was more like 10, but today we have more like 24. At that time, we needed to decide where to install stations, we needed to worry about the permits, and we needed to worry about the funding. I provided leadership on all of these issues. And we needed to worry about getting the data back. As I said before, Pacific Bell provided free data circuits for a number of years in the 1990s, and I was involved in interacting with them and making sure that they felt that that it was a successful project. Regarding my work here at the Seismo Lab, I worked with postdocs and a couple students in the 1990s. We focused on research to improve understanding of the seismicity we were having at the time. For instance, studying the Landers earthquake, it was interesting that we were able to show that as a rupture went from south to north along those fault segments, that it essentially ran out of energy. It didn't have any more stress in the ground to drive it. Because we found small earthquakes that showed that the crust was moving backwards around the north tip of the main shock rupture. Regarding the Northridge earthquake then, back in 1994, the whole focus there was to understand in real time where the aftershocks are occurring, and where might they occur in the weeks and months after the main shock, and get a good picture of how did it relate to the previous 1971 San Fernando earthquake. Because of the 1987 Whittier Narrows earthquake, there was the focus on the blind faults in the Los Angeles Basin.
ZIERLER: I'm curious with these new capabilities if it rekindled any optimism over the prospects of earthquake prediction, which in the 1970s, of course all of those discussions happened within the context of analog instruments.
HAUKSSON: Here's the problem. We can measure seismic waves with these instruments, but when the earthquake happens, it does several things. The fault moves, and it heats up the rocks on either side, like if you slide your hand on the table you feel it gets hot. It also breaks rocks near the fault surface, and it radiates seismic waves. We don't know the energy budget for these three processes. I'm sure there are also other processes also happening. What we measure with seismometers are the seismic waves. That's one issue that makes it very hard to predict earthquakes. If we knew the energy budget, we knew the friction and so forth, it might possibly be done. But having said that, what we get out of these new data is, for instance, how much does stress in the ground change—and we refer to that as stress drop—as a result of the earthquake. We now have a picture that shows that both small and big earthquakes can have similar change in stress, and that it doesn't change much with depth. Interpreting that in detail is still being worked on. Once we have figured that out, maybe we'll get a little closer.
Another aspect of this, of course, is foreshocks. Like back in 1987, there was a Superstition Hill earthquake sequence that started out with a 6.0 on a southwest striking fault, and then a few hours later, there was a magnitude I think 6.5 on a fault parallel to the San Andreas, the Superstition Hills Fault. Similarly, in Ridgecrest, there was a magnitude 6.4, and a few days later, a magnitude 7.1. These were also orthogonal ruptures. They're somewhat surprising because they don't really match what we know about strength of rocks. We would expect them to be at an angle of 30 to 60 degrees, not to be orthogonal. The foreshocks at Ridgecrest were sort of obvious that, yes, something bigger could happen. Understanding those processes is important. So, in some cases, I think we are getting a bit closer to at least having some idea of exactly what goes on in such sequences. Once we have enough cases, then somebody might be able to give a higher probability than what we can give today.
Advances in Real Time Analysis
ZIERLER: You mentioned the advances for real-time analysis. Is that to say that the digital revolution in seismology got us somewhat closer at least to earthquake early warning?
HAUKSSON: It did, yes. The early warning would not have been possible without the digital data. There's a couple things there. One is that with the analog data, once everything went off scale, it was very hard to analyze the data, and the computers were not powerful enough at that time, so it took like an hour to get good information about the Landers earthquake, and similarly an hour to get good information about Northridge. In the case of Northridge, people had put in some filters that said, "If the data look like this, everything goes off scale at the same time, then it's bad data, because we've got a data dropout on our microwave links." But the Northridge earthquake was so deep that its P-waves hit a number of stations almost simultaneously, so that data got caught in that filter and was not analyzed by the computer. A human had to analyze it by hand after the fact. This doesn't happen with the digital data. The digital data streams, and maybe it gets slowed down a little bit, but it just keeps streaming. We have those data streaming all the time. They're continuous and not event-triggered. They're not dependent on an earthquake being there; we collect them all the time, seven days a week, 365 days a year, 24 hours a day, and once every 0.01 seconds. The strength here is that, yes, you're getting good data, and you're getting them fast, so you can apply seismological algorithms successfully to analyze what is happening. If you are getting data that maybe sometimes comes in too slow, and that are mangled by the equipment, then the algorithms won't work.
It was clear that when we had Landers, and especially Northridge, that the expectation from the emergency management community and from the media and everybody else was that we should have information right away, and it was not good performance to only have information an hour after the earthquake. That's one of the reasons we pushed very hard for the instrumentation that would provide data on scale and could be successfully analyzed quickly. That's big progress, and that's the foundation of earthquake early warning, that we have these high-quality data and they can successfully be analyzed near-real time.
ZIERLER: Coming to Caltech, managing the Network, were you filling an open role, or was this a new endeavor for Caltech?
HAUKSSON: Caltech had been in this business actually since way back when, when Seismo Lab was part of the Carnegie Institute in the 19-teens, the 1920s. It was the later director of the Lab, Harry Wood, who had headed up the network. I took over from Prof. Clarence Allen, who was the manager for the Seismic Network, when he retired in late 1989.
ZIERLER: Who were the key institutional partners? Between other universities, the U.S. Geological Survey, who are the most important institutional partners for this?
HAUKSSON: The most important partner is of course USGS, and they opened up an office here on campus back in 1974. That was in part a result of the 1971 San Fernando earthquake, that they recognized that the instrumentation needed to be upgraded and made more modern, at that time. The other important entities are the California Geological Survey and of course the California Office of Emergency Services. The California Office of Emergency Services provides significant part of our funding today, because they are interested in supporting earthquake early warning. At the time of the San Fernando earthquake, they and Caltrans did provide some funding for several years, which then tapered off. The California Geological Survey (CGS) operated, at that time, strong motion recorders, which were like black boxes on airplanes that would get triggered by strong ground shaking. These data were available after the fact when somebody had gone out and collected them and developed the film. We worked quite a bit with our colleagues in Northern California, too—UC Berkeley, and also USGS there, which used to be in Menlo Park but now they are at Moffett Field. We have some collaboration with other universities in the area, like UC Santa Barbara, UCLA, UC at Riverside, and UC San Diego Caltech also worked with USC, in the early 1990s, when USC operated some seismic stations in the Los Angeles Basin.
ZIERLER: Your responsibilities for the Network, were they completely bound up with your Caltech appointment both in terms of the funding and the reporting structure?
HAUKSSON: They were not quite completely. Caltech did provide a small fraction of my salary, but most of my salary came from running the Seismic Network. In that way, that kind of defined my position. But because I am on the research faculty, and my position was described in the faculty handbook as research associate in the beginning, and then later senior research associate, then it later got converted to research professor—but the faculty handbook states clearly that anybody on this track shall do research, and that's how this person should be evaluated on their performance. It should not only be research, but it should also be internationally recognized research. So, I raised some part of my salary myself, on research grants, and raised salaries for postdocs and students. Then I also managed the Seismic Network to improve its funding as we completed different upgrade projects.
ZIERLER: The Network part of your responsibilities, that was funded by the Survey?
HAUKSSON: When I arrived at Caltech, the network was funded by the USGS, and a minor contribution from the state of California. I think we had like $400,000 in annual funding in the early 1990s. Today, the funding is more like $5 million per year. The way we did that was not so much through increased funding from the USGS at that time, but we had special projects. We had the CUBE project, Caltech-USGS-Broadcast-of-Earthquakes, where we got companies to join in funding a new project which was real-time seismology. The members of this project used electronic pagers and they received near real-time earthquake information on those pagers. Of course, as time went on, pager technology went out the window, and the internet took over distributing near real-time earthquake information at no cost.
Then after Landers, we started asking, "Can we find another funding source?" We were not quite successful, but after Northridge, we asked again, and we pushed really hard for getting funding. That was the TriNet project that started in January of 1997 and lasted for five years. The TriNet project was funded by FEMA—the Federal Emergency Management Agency—through the California Office of Emergency Services. It took a lot of push on Caltech's behalf, like Frank Press who was the previous director of the Lab and Carter's science advisor, was involved in helping us get that funding. Because FEMA had to rewrite some of their regulations to be able to provide this funding. The new regulations stated that if recovery funds were coming into the state, the state was allowed to use a small percentage of that recovery funding to fund new projects that might help or mitigate impact of future events. TriNet was funded 75 cents on the dollar, and then we had to raise another 25 cents on the dollar to get the total project amount up to—it was like $12 million, for five years. Various companies provided some funding for that, including the utilities. Also there were some state programs that did provide funding, that had to do with funding technology innovation. So, we managed to make it all work out. After that, there were several other projects that followed.
Since we were able to show successful products and successfully report on various earthquakes, the California Office of Emergency Services got quite interested in taking this technology statewide. That resulted in a project called California Integrated Seismic Network, where we worked closely in our colleagues in Northern California to export our technology to them. That was successful for a couple years, but then in the early 2000s, the state budget went into a hole, so we got cut quite significantly as time went on for more than a decade. We only had like 40% left of our original budget after 12 years. But at that time, there were other projects that came along, like there was something called the American Recovery and Reinvestment Act (ARRA) project, which had to do with stimulus funds after the great recession in 2008 and 2009. That allowed us to upgrade a lot of instrumentation with USGS funding. Then we had a project that was funded by the USGS called the USGS Multi-hazards Project, it included upgrade of instrumentation along the southern San Andreas Fault, which added a number of stations to the Network of high quality. The latest project, of course, is earthquake early warning that began more than ten years ago. In my opinion that has actually been properly funded. The project is doing very well today.
ZIERLER: Coming to Caltech in 1989, did you get a sense of the very tail end of the Don Anderson directorship years?
HAUKSSON: [laughs] I don't know. Don was always nice to me, and when Lucy and I were trying to decide to stay in town or move to Oregon, he invited us over to his house for lunch, which was nice.
ZIERLER: What about when Hiroo was named director? Did you sense any new directions that the lab was going under his leadership?
HAUKSSON: Yes, clearly. He cares about both local and global earthquakes, and he cares about people who experience earthquakes, so he wants to make a difference for people who live in earthquake country. He understood real-time seismology, especially because he's very well-connected with Japan and he works with his colleagues in Japan. Of course, they had a big earthquake back in 1995, which caused a lot of damage and deaths. There, they started doing new instrumentation in Japan, and when we bought ten instruments, they would buy a thousand. We would put them on the surface; they would go out there and drill bore holes down to 300 to 600 feet for each instrument, to get very quiet data, to get away from the surface noise, away from the traffic and what have you. Hiroo really wanted to make everything digital, to make sure he had data that he could model. He provided us with these algorithms so we could make them run real-time. He wrote some of the basic software that is still used today, and provided the code, and we changed it slightly to fit within our software framework, but it didn't take a lot of changing.
He also wanted to make sure that people used the information, so he was proactive about getting involved with emergency managers, meeting with them, and talking to them about the project. He raised funding from the L.K. Whittier Foundation for the first part of TERRAscope. It was clear that he was devoted. I got the feeling with Don Anderson, a prior director, that there weren't many local earthquakes, so the focus of the lab was more towards global seismology. That had in part to do with competition, too, of course, because he was competing with his colleagues at Harvard. They were big on global seismology, too.
The Advent of Seismotectonics
ZIERLER: Tell me about your research focus in the early and mid 1990s on seismotectonics.
HAUKSSON: My focus has always been to work with the data that I have in hand, because that's what I best understand. When I first got to Caltech there where lots of small earthquakes, as well as moderate sized earthquakes, like the 1987 Whittier Narrows earthquake, the 1991 Sierra Madre earthquake, the 1988 and 1990 Upland earthquakes and so forth. I was interested in understanding all of those earthquakes here in the greater metropolitan area. What faults were they lining up? What's their systematic pattern? How would this help us to further understand earthquakes and faults, into the future? The Whittier Narrows earthquake, when we analyzed the data we realized the sequence occurred at bout 10 miles depth, and that was a surprise. Then later I analyzed all of the smaller earthquakes that had been recorded back to the early 1980s—so, that was, at that time, almost ten years of data—and lo and behold, a lot of the small earthquakes formed the same pattern and mapped these faults that extended from Whittier to downtown Los Angeles.
The other thing that we want to understand is what's the role of the Sierra Madre fault, and other faults like the Raymond fault, that runs very close to Caltech. There was the Pasadena earthquake that we studied that had an epicenter somewhere around Lake Ave. and Del Mar Blvd. intersection, but it was about ten miles down. That one lit up a piece of the Raymond fault. Then of course there were other events, for example the Sierra Madre event was further up on the far side of the Sierra Madre fault zone that runs all the way from Cucamonga and out towards San Fernando. There were other quakes and faults that we studied like the Upland earthquakes, and that allowed us to understand that, yes, some of this tectonic movement was happening further south, towards the Whittier fault, and so forth. Previously I was involved in earlier studies on understanding the Newport-Inglewood Fault, and the Long Beach earthquake that occurred back in 1933. The Newport-Inglewood fault is interesting in that it is segmented so that at the ends of the segments, the ground gets pushed up, and that forms traps for oil. That's where the oil fields are, like the Baldwin Hills Oil Field. This type of deformation is important to understand, in unravelling the relationship between the seismicity and the geological structure along that fault.
ZIERLER: Was the CUBE project part of TERRAscope or that was separate?
HAUKSSON: It was separate, but it was somewhat related, in that the CUBE project was mostly focused on the fact that we had the information in hand, and then interact with users and teaching them how to use it and provide pagers to them so that they would have the information right away. But of course, the TERRAscope and the new digital instrumentation was the basis for feeling comfortable with providing the information real-time without being checked by a human.
ZIERLER: What was the day like for you when the Northridge earthquake hit?
HAUKSSON: Now I have to fess up! [laughs] It was pretty cold, because I was in Boston!
ZIERLER: [laughs] Perfect.
HAUKSSON: I was staying in a hotel where it was so cold that the fire alarm went off.
ZIERLER: [laughs]
HAUKSSON: But then as I got up and had breakfast, of course there was continuous coverage on the TV from L.A., explaining the earthquake and what had happened. I was supposed to be at a meeting with a vendor there, so I said, "No, I'm going back to L.A." I headed back to L.A. right that morning.
ZIERLER: What was the scene like when you landed?
HAUKSSON: [laughs] It was clearly that people had been through sort of a trauma. They were all wound up in this; they were not thinking about their daily lives. They were trying to deal with it. Lucy was there with our boys and getting help from her stepmother to take care of them while she was dealing with media. I did actually fly back, and they actually gave me first class going back through Denver on United, and then I couldn't land at LAX; I had to land out at Ontario airport. But I talked to people on the phone as I flew back. The feeling was that, yes, there was something that had gone wrong but most things went right. Because when you go through something like that, you get traumatized, no matter what, and people were doing their best to sort of hold up, and make sure that data was not being lost, and that they were communicating out the information that we had. But I felt cheated, not being there when the earthquake happened.
ZIERLER: Was the network up to the task? Did it do what it was supposed to do during Northridge?
HAUKSSON: Not really. At the time, the Network was mostly a research network with the old analog instrumentation. We only had about eight or ten of the new TERRAscope stations in the ground. As I spoke about before, the earthquake happens, the P wave is radiated out from the hypocenter. Because it was deep, ten miles, it hit a number of stations at the same time, and that data was thought to be bogus, because it was the equivalent or similar to what would happen if there was a dropout on the communication lines. So, the data got put aside by the computer and not analyzed. A human had to pull that data off the acquisition computer and put it on a different computer and analyze it. That took about an hour to do. To some extent, it was good and bad for us, because with government funding, nothing succeeds like failure. We were able to demonstrate we couldn't do the job with the equipment and the resources that we had, so that made a very strong argument that, yes, we need to move forward.
ZIERLER: Was this a big jump from the initial $400,000 budget to the $5 million today?
HAUKSSON: Yes, it was. This added about $2.5 million per year, at that time, to our budget. That was quite significant.
ZIERLER: As you mentioned, you want to work with the data that you have, and that compelled you to focus really centrally on Los Angeles and Southern California. What was the value in that focus in thinking about the cyclicity of earthquakes, either generally or specifically to the Los Angeles region?
HAUKSSON: In the 1980s and early 1990s, there were all of these felt earthquakes in the L.A. area. They were all felt and talked about by the public and covered in the media. But similar things had happened in the 1960s and 1970s in Imperial Valley. Then the earthquake activity migrated more out to the desert in the late 1990s, with Landers and Hector Mine. So, it's like the seismic activity was moving around. Sometimes some areas are more active than at other times. Unfortunately, most of the time we don't have enough earthquakes to make clear patterns unless we observe for a much longer time than a few decades. But plate tectonics is a very steady engine. It never skips a beat. It's loading the crust, but the crust deforms and moves in different ways to accommodate the loading. Eventually, something has to break. Most of these earthquakes that are off the San Andreas or off the main plate boundary are accommodating that loading phase. We don't quite understand what triggers earthquakes. We know if certain size seismic waves go through an area, they may trigger earthquakes. We know if we pump water into the ground, that may trigger earthquakes. But what creates, say, a decade of higher-level activity than we had seen for quite a while before in the L.A. Basin, we don't quite understand exactly what and how that works. But that's an exciting research question.
ZIERLER: A topic that you worked on with Hiroo Kanamori—you talked about the value of digital instrumentation for real-time seismology. How does that connect to earthquake mitigation, such as saving property, saving lives?
HAUKSSON: The way it connects is that if you're able to provide information like earthquake early warning, people can protect in place—duck, cover, and hold on—and that allows us to save lives because they're not running around during the dangerous shaking. They actually know it was an earthquake; it was not something else. The other aspects of it are providing good data to land use planners and to engineers, so we don't build buildings across faults that may then—suddenly, if you build one building across a fault, after the earthquake, it's two buildings, none of which you can use because they're both damaged. Or we can map out where the ground shaking is likely to be strong, and if you do a lot of processing of the data, you can build maps that show you that every 50 years, it's so-and-so likely to have this type of ground motion in these places. Those maps show the San Andreas Fault, where it's a lot more likely than, say, along the Palos Verdes fault, to have strong ground shaking. If the engineers take that into account when designing their buildings or structures or bridges or what have you, then that saves lives.
Having data in real time I think is important, because our attention span is always growing shorter and shorter. We need to know right now, and preferably before the earthquake, that it's going to happen, and what the effects are going to be. Now we can tell you what the effects are going to be if this sort of type of scenario earthquake happens, but we can't tell you exactly the place or time. I think the other aspect is, yes, if the monitoring is done with modern technology in a very professional way, and it is provided in a consistent way to the public and emergency managers, people are much better at reacting to information and doing the right things for the right reasons, as opposed to, if they don't get good information, they will just go by rumors, and that's never a good thing.
ZIERLER: What was your approach to some of the debates surrounding the Hector Mine earthquake in 1999, relating to the idea that it may have been related to the Landers earthquake seven years earlier, and what this might tell us about stress accumulation?
HAUKSSON: We have seen this a number of times—Landers and Hector Mine; San Fernando and Northridge. We have seen it on a shorter time scale with Ridgecrest and its magnitude 6.4 foreshock, and the 6.0 Elmore Ranch and the 6.5 Superstition Hill in 1987 which had a similar setup as the foreshock in Ridgecrest. So, yes, there clearly is some relationship there, but proving it has been hard. Landers had some aftershocks that were within the area of the epicenter or hypocenter of the future Hector Mine earthquake. A number of studies have shown that, yes, it is likely that Landers had something to do with the occurrence of the Hector Mine earthquake. I agree with those studies, that there is some relationship there, but exactly what it was is kind of hard to tease out of the data that we have available today.
ZIERLER: What role did TriNet play in understanding Hector Mine?
HAUKSSON: TriNet had I think about a dozen stations, at least, working at that time. It provided data on scale that was used for understanding the earthquake. We had the closest station to the earthquake, which was on a mountain just at the north end of the rupture. We also had a station out at Goldstone which is a bit further away, and people used data from that station quite a bit. So, it provided the geometrical configuration and how deep it extended into the ground, and what kind of motion took place on each one of the different fault segments. Landers and in particular Hector Mine, that's where INSAR came in and provided more detail than we had ever seen before. When you get a map from a satellite, they take a picture before the earthquake, or a radar image, then one after the earthquake, and then take the difference, and we can really see in excruciating spatial detail how the ground moved.
ZIERLER: In what ways did Hector Mine advance our understanding of aftershock science?
HAUKSSON: Not quite as much as Landers. Landers triggered earthquakes up and down the West Coast, all the way into Canada. Hector Mine did trigger some earthquakes down into the Salton Sea. The Hector Mine was somewhat typical for what we expect. There were some off-fault aftershocks like we saw with Landers, and it sort of confirmed that those patterns can occur. But its decay of activity and so forth was similar to what we see with other earthquakes.
ZIERLER: A paper that you contributed to in 2004 with an intriguing title—"Converting Advances in Seismology Into Earthquake Science"; I thought seismology was earthquake science. What's the conversion? What's happening here?
HAUKSSON: [laughs] I haven't looked at that for decades, but the earthquake science is because people don't quite understand seismology, and they view it as being more this instrumentation that's in a seismic network and associated data. But if you step back and look at what earthquake geologists do, like Kerry Sieh and Clarence Allen used to do—digging trenches across the faults—that extensive earthquake history for the fault back in time, may be up to 1,500 years. That's another piece that gets added to seismology. Then there's a lot of research that is done, for instance, modeling ground motions from earthquakes and figuring out what period band they are in, and how strong they are, where, and how likely they are. That's another piece that gets added in. Then of course there is simulation of data added in, with high-performance computers. When you add all of these pieces together, we refer to that as earthquake science. It's much broader than seismology. Also it includes geodetic data using GPS, which if you use other countries' GPS satellites, it is referred to as GNSS. That adds to understanding of displacements of the ground or strain buildup in the ground, for the secular plate motion as well as release in sudden events. If there's an earthquake of magnitude larger than 6.0, then GPS captures that usually quite well. It tells us, for instance, that the Santa Susana Mountains north of Northridge moved up by a foot or so, as a result of that earthquake in 1994.
ZIERLER: After the Northridge earthquake, as you mentioned, the Network really wasn't up to the job. It needed more resources, and it got them. At what point, how soon after that surge of funding, did the Network really become more effective as a result?
HAUKSSON: I think we saw it in the Hector Mine earthquake in 1999. We were able to put out the location and the magnitude within minutes, and that was our big success after having gotten the new funding. There may have been other smaller earthquakes where we did quite well, but that's the big benchmark in my mind.
Geophysics in the Computational Era
ZIERLER: In the Seismo Lab, in the late 1990s, were you part of those discussions where people like Mike Gurnis and later Jeroen Tromp were really pushing for much greater computational capabilities?
HAUKSSON: I was part of the discussions where Jeroen Tromp wanted to do his own version of ShakeMap using these computational facilities. He wanted to use a 3D model of seismic velocities in the ground to generate what he called a synthetic ShakeMap. From the new digital instrumentation, he would know the configuration of the fault that broke in the earthquake and how long it was, how deep it was, and what kind of motion occurred on it. He would stick that into his model and then calculate a ShakeMap. I was quite a bit involved with that but I was not involved with the actual geodynamic modeling or any global research that Jeroen Tromp or Mike Gurnis were doing. But [laughs] the big change was, for instance, that they took an old computer room and they filled it up with PCs, and they actually did that by themselves, which was impressive, I thought, and they got that to work. That worked for a while. Then Jeroen wanted a bigger computer and more professional, not something that he had put together with his students.
Almost every big building on the Caltech campus has a basement and a sub-basement; I don't know if you realize that. The campus is bigger than it looks to the eye. In our sub-basement there was a big archive of film chips with images of seismic data, from both the worldwide seismographic network that some people have probably described to you. The U.S. Air Force used to run stations in the western U.S. for nuclear monitoring, and a lot of that data was on film chips or reals of film. Tromp wanted to use that space for a computer, so he asked to have that film chip library moved out of the building. It left the building, and then it wandered around Pasadena, but I found Emile Okal, who had been a student here—he was at Northwestern—he said he wanted to get it. This was an 18-wheeler trailer truckload [laughs] of film chips in filing cabinets. He said he would take it, but he wasn't coming forth with the money to pay for the 18-wheeler. I said, "I'll pay for the 18-wheeler to save this stuff. It's only $5K." Then I got a call from his secretary, saying, "So, how much is this? Will it fit in his office?" [laughs]
ZIERLER: [laughs] "If his office is bigger than an 18-wheeler, sure, no problem."
HAUKSSON: Jeroen Tromp had a big computer that was funded by Dell that was put in the sub-basement, in part, and by other funding sources in the long run. That was a quite successful installation and one of the cutting-edge university installations of supercomputers. We published his version of the ShakeMap based on calculations done on that cluster.
ZIERLER: Were part of your motivations, besides just preserving this for posterity, that that there was still value in this old analog information?
HAUKSSON: My motivation was: there is still value. Yes, a lot of value. But the issue is the cost of scanning all that stuff. In a way, you can scan a film chip, but often, their metadata is on an envelope that the film chip is stored in. If you lose the metadata that tells you what station it is and so forth, then you don't have anything. That makes it expensive.
ZIERLER: Ten years in or so, how had your responsibilities changed for managing the Network? How many more people were you supervising? How much more complicated was the work?
HAUKSSON: When you grow with a job, you don't realize the work is getting any more complicated; you just think it is what it is. [laughs] I think the big difference was, when I came on board, we had a couple of engineers that were doing station maintenance, and a supervising engineer who designed electronics. Then we had three data analysts and a staff seismologist, who was Dr. Kate Hutton at the time. As the project grew, we added more field engineers and we stopped building electronics ourselves and instead purchased all the equipment from vendors, which I think has been a great development for the community, because when you have scientists fidgeting with the equipment, you never know exactly how it is calibrated, because they just changed something just before the earthquake, and they didn't know exactly what they changed. The big difference is that we decided as part of TriNet to have a lot of software development, so we hired two professional software developers to write software that would match up with the needs of the digital data. The USGS provided several staff who were also involved, so we had four people from basically nobody before. That was the big change in the late 1990s, early 2000s.
ZIERLER: We've talked previously about the idea of being able to extrapolate local findings to understand planetary systems. With all of your focus on Southern California, what had to be truly unique simply by virtue of Southern California's unique location with regard to plate boundaries?
HAUKSSON: In Southern California, the plate boundary is a lot more complicated than in the Bay Area. Because the plate boundary is not straight, it doesn't follow exactly the direction of plate motion, so the San Andreas, from Cajon Pass up to Gorman, is trending more westerly than the rest of it. That leads to the plates colliding with each other, and that builds the mountains, like the San Gabriel Mountains. It also leads to our seismicity being, in my mind, more complex, in that it's more three-dimensional, as opposed to, for instance in the Bay Area. There the plate boundary deformation is dominated by earthquakes on strike-slip faults, like the San Andreas Fault, Rogers Creek Fault, or Hayward Fault, which makes it easier to understand exactly what is going on. In southern California, we still grapple with what is the current role of the San Andreas, versus, say, the San Jacinto Fault, or the Elsinore Fault. We know they are all seismically active, and from geologic data, we know that San Andreas is the most active one, but how the other ones get activated and why and when is still an unsolved question.
A big part of this is, too, that the Sierra Madre Fault Zone is an east-west fault, and it's what we call a thrust fault, with vertical motion. As you think of, say, the Peninsular Ranges block colliding with the San Gabriel Mountains, the Peninsular Ranges get pushed downward, while the San Gabriel Mountains get pushed up and around the San Andreas fault to the west. As part of this, we find earthquakes that seem to be having bigger stress drops, which means that they are more of a jerk than many other earthquakes, so that the ratio of the movement on the fault versus size of the fault surface is unusually large. Those earthquakes, we are quite interested in trying to understand their role in how these processes take place.
ZIERLER: A long review article you wrote in 2010—there's just so much even in the title that requires definition, so let me just say the whole thing: "Spatial Separation of Large Earthquakes, Aftershocks, and Background Seismicity: Analysis of Interseismic and Coseismic Seismicity Patterns in Southern California." Let's start at the beginning. What does that mean, "spatial separation of large earthquakes"?
HAUKSSON: That means trying to understand where the earthquakes are happening relative to each other. For instance, Landers and Hector Mine are separated by 10 to 20 miles. Similarly with Northridge and San Fernando, it's more like five to ten miles, depending on how you measure it. But that is what comes to mind on spatial separation. As far as I recall, that paper was about trying to understand—we have a model of the faults, and we took all the hypocenters and measured the distances from the hypocenters to the nearest fault. That's trying to understand exactly where are the earthquakes relative to the faults. Are aftershocks closer to the faults than, say other earthquakes, where there hasn't been a major earthquake recently.
ZIERLER: What is background seismicity? Is that specifically related to aftershocks?
HAUKSSON: No, that's the seismicity without aftershocks. Seismologists like to build filters and filter out the aftershocks, to determine the background rate of earthquakes. But because aftershocks are triggered by the main shock and become suddenly a lot more numerous following a main shock, the idea is that that activity doesn't really represent the steady state; it's an anomaly within the system. People like to pull those out of it, and that will give them sort of an idea of how frequent earthquakes are as part of an ongoing plate tectonic process.
ZIERLER: Finally, interseismic and coseismic; what's the difference?
HAUKSSON: Coseismic is what happens at the time of the main shock. Interseismic is the time period when you don't have a main shock and the aftershocks time period has elapsed. For San Fernando in 1971 until Northridge in 1994, there's that time period of 23 years, which I would call interseismic except for a few years of aftershock activity following the 1971 mainshock, where there was nothing else happening in the region.
ZIERLER: Which tells you what?
HAUKSSON: Which tells you more what the background rate is. What is the rate when you have no aftershocks? It tells you how the ground is adjusting as faults are being loaded, because they are being loaded at a steady rate, and there's no disturbance from a big earthquake that creates secondary features in a stress field needed to trigger aftershocks.
ZIERLER: I'm not sure if the answer is that you're still waiting, but do you have a memory of when the technology and the science had progressed to a point where earthquake early warning was actually helpful to people?
HAUKSSON: I don't think we've had a magnitude 6+ earthquake where earthquake early warning made a difference, frankly. But we have had a number of magnitude 4+ earthquakes where people did get notified, and they felt like they had a heads up information. Tell me the question again?
ZIERLER: The idea that earthquake early warning had progressed to a point where it was actually helping people.
HAUKSSON: The biggest earthquake that earthquake early warning has been operating during is the 2019 Ridgecrest earthquake. There, it worked, but it didn't provide a warning to everybody who felt the earthquake. There is this sort of tug of war; should we alert everybody who might feel it, versus should we only alert people who might have significant damage? I don't think there was anybody there using earthquake early warning that had significant damage. By the way, the Ridgecrest earthquake is always thought of as being out in the middle of nowhere and that it didn't cause much damage, but about 80% of it happened within a military base, China Lake Naval Air Weapons Station. They had several billion dollars of damage out there in the "middle of nowhere," in the desert. That's not much talked about, but they have released that number. If they had had early warning, that might have helped them; I don't know. But they were right on top of the earthquake with most of their stuff. The earthquake ruptured along the hillside close to where they had built all of their different laboratories and testing facilities.
So far, there hasn't been an urban earthquake, except for instance there was an M4.5 earthquake near El Monte, in 2020—where the warning went out about the same time the P wave hit the surface at the epicenter, and a lot of people got warning and realized it was an earthquake, but they didn't have to do anything because it wasn't big enough to cause damage. But that demonstrated that the system is working and gave people a heads-up so they could practice how to respond.
ZIERLER: The Sierra Nevada microplate is a topic you've returned to repeatedly. What is so interesting to you about it?
HAUKSSON: I don't know whether you spend time in the mountains or not, but [laughs]—
ZIERLER: It's a nice place to be!
HAUKSSON: It is. The interesting thing about it is that this is shown through various data, that the Sierra Nevada is a microplate by itself, and it's moving at its own speed, and it's not attached to the North America Plate. So, as the two places, the Pacific and North America Plates pass by each other, the Sierra Nevada sort of has its own speed, so it can have earthquakes both on the San Andreas side as well as on the east side along the frontal faults or in Owens Valley. That is one aspect of it that is quite interesting.
Another aspect that is exciting about it is we see in the earthquake data that the southern part of the Sierra Nevada is in what we call gravitational collapse, that the mountain is extending both east-west and north-south as it decreases in altitude. The elevation of it is coming down and the earthquakes are reflecting that. Of course, it's a slow process; if you look at it today or ten years ago, it will look the same. But [laughs] that's the interesting thing about these small earthquakes; they can detect these significant processes that are happening that we can't see with our bare eyes.
ZIERLER: Back on the administrative side, when you were named research professor in 2014, was that seen as a promotion, or was that something where Caltech had turned all of these previous titles into this new research professor position?
HAUKSSON: That was seen as simply that Caltech was adjusting its nomenclature for these research positions. It was not a promotion at the time, because I had already made senior research fellow, and this was just changing that title over to research professor.
ZIERLER: Having the professor title, that didn't change the kinds of things you did, the interactions you had on campus?
HAUKSSON: No, it didn't.
ZIERLER: Have you been involved in—I don't know what the right term is—discriminating seismic data, earthquakes, from other kinds of shocks to plates?
HAUKSSON: We have earthquakes that have to do with tectonics and the movement of things along the plate boundary. Then we have earthquakes that are more related to, say—the ones in Southern Sierra, they don't extend all the way through the seismogenic crust. They cease occurring before they get through the brittle crust, so they're not related to accommodating plate boundary motion. Also Hiroo Kanamori studied an earthquake in Santa Maria Basin that was unusual because it was in an oil field, and the movement on the earthquake fault seemed to be very slow, and that was a big surprise to us. I have looked at earthquakes in the different geothermal areas, like Coso, just east of Owens Valley, or north of Ridgecrest, and the Salton Sea geothermal area. Some of those earthquakes are certainly triggered by human activity, but then again, there is also a lot of natural seismicity in those areas.
ZIERLER: That is what I was asking about, in terms of discriminating. How do you know what occurs naturally and what is caused by human activity?
HAUKSSON: That's a good question. If you look at the seismograms themselves, they look the same. There is no difference depending if the earthquake was natural or caused by human activity. But usually if somebody is drilling a hole in an area where there are no earthquakes and they start pumping in a lot of water, and then suddenly there are earthquakes, people infer that the two are related. It's the same thing in the geothermal fields. The entities that are doing the production of power there often pump in water because there's lots of hot rock, but there's usually a lack of water to harness that heat out of the rock and bring it up to the surface. In a geothermal field, having small earthquakes is a good thing, because that creates cracks, and that allows the water more easily to flow back up to the surface, and increases the surface area, so increases transmission of heat out of the rock matrix into the water.
There are some thoughts that some of the earthquakes that are near oil fields may have been triggered by activity in the oil fields, but again, that's always a circumstantial thing that is hard exactly to prove. Sometimes the distance is greater than we would like between the oil field and where the earthquake happened. I did look at the L.A. Basin, looked at the rate of earthquakes near all the different oil fields. I didn't feel like I had a case to say that, yes, there were more earthquakes because of the oil fields, where the oil fields are actually located, as compared to other areas of the L.A. Basin. Other people have looked at that data and said, "No, in the early 1900s, when they started oil production in the L.A. Basin, there were more earthquakes than we have seen since then." The jury is still out on some of those issues. I think as people study them more, we will get a better handle on it.
ZIERLER: When did you start thinking that machine learning or artificial intelligence might be useful tools in seismology?
HAUKSSON: That was with my postdoc, Zach Ross, who is now a professor in the Seismo Lab. That was like 2018, we started thinking about that. We had all of those hand-labeled data, that our analysts had labeled back to the 1930s, with the digital data starting in 1981, and then high-quality digital broadband data in the 1990s. We could use all of those labeled data to train machine learning codes to do the same thing as the humans were doing, and then use that to run through all of our data that we had saved back to about 2000 and see if it could find more earthquakes in the data. And we did; between five to ten times more. Of course, that opens the door for, "Hey, can we just do this all with machine learning and no human interaction?" We're working on that, but we're not quite there yet.
ZIERLER: Is that more a limitation of seismology, or a limitation of machine learning, would you say?
HAUKSSON: I think it's a limitation of resources. You have to remember, when JPL puts up a satellite, that costs one or two or three billion dollars. We're working in the millions. [laughs] We can have maybe two or three people, but for us getting 30, it's out of reach. If we had 30, then yeah, it would be easy. It could be done much more quickly. [laughs]
Learning from Old Earthquakes
ZIERLER: A specific question relating to a general issue: in 2018, for example, you returned to the 1992 Landers earthquake. The general question there is, when do you find it valuable to return to old earthquakes? What new things have happened in the field, observationally or technologically, where there might be new things to learn from old data?
HAUKSSON: I think whenever you have a new earthquake, so to speak—something like the 2019 Ridgecrest earthquake happens—then you have a better understanding of what is going on with that earthquake, and then sometimes you may want to go back to an older earthquake that behaved in a similar manner and ask the same questions that you were able to answer for Ridgecrest because you had better data and you had better understanding of how to interpret that data. In the case of returning back to Landers, I have a colleague in Japan, and she had a better model of interpreting the state of stress in the ground. We applied that model to get a better understanding of how much the state of stress in the ground changed as a result of the Landers earthquake, and we were able to get a quantitative answer that we didn't have before. We knew it changed, but we didn't know by how much.
ZIERLER: Moving the conversation closer to the present, when did you start thinking about stepping down from your responsibilities managing the Network?
HAUKSSON: About two or three years ago, I told Mike Gurnis that I would like to step down at some point. It took him a couple of years to find somebody. [laughs] Allen Husker has been on board here for a year or so.
ZIERLER: You were thinking about this before COVID?
HAUKSSON: Yes. COVID was a curveball for all of us, including myself, but COVID didn't really affect me that much, because I was able to work from home, and we did all our work from home during that time. I've been working in the office now for more than a year or since September 2021. Since I live close to campus, I can't have the excuse of a long commute.
ZIERLER: What have been some of the benefits and liabilities of remote work, not seeing your colleagues?
HAUKSSON: If I was trying to plan a new project, if I was dealing with issues of funding and agencies being weird about funding and I needed to talk to my colleagues; if I have a new idea about research and I would like to talk to my colleagues, that's not so easy now. Now, you have to set up an appointment; you can't walk into somebody's office. There's not this sort of spur of the moment collaboration that used to happen. It seems to have gone by the wayside for the time being. I think that's a huge issue, in a way, for something like the Seismo Lab that relies on a coffee hour every day in the morning to educate the students about how to discuss their work and have discussions with other colleagues about their research.
ZIERLER: Did working from home, not seeing other people, provide some extra time to work on some projects that you might have intended to, for a long time?
HAUKSSON: Not really. [laughs] I did work on a project of analyzing earthquake sequences in the Salton Sea, or at the south end of the San Andreas Fault. That was an important project that I suddenly had time to do. It gives us a good overview of what has happened there over the last 40 years, and good context for interpreting any future sequences that might happen.
Then I had some weird side projects. Imagine some Caltech guys in the early 1960s decided they wanted a seismic station about 60 miles east of Palm Springs, which was on land that is owned by Metropolitan Water District (MWD). The MWD decided in the middle of COVID that they wanted to get rid of our hut that was built in 1962 and hadn't been maintained, but we had moved the instrumentation out of it and put it in a vault in the ground. Here was this shed filled with killer bees, so we had to find a bee exterminator—communicate with him on the phone, and ask him to go out there and kill the bees. [laughs] Then we had to find a contractor who decided, "Well, I can't really tear it down, because it's all asbestos." So we had to get an asbestos test guy out there, and he said, "No, there's no asbestos. They probably didn't want to spend the money on asbestos when they built this thing." Then we had to have a contractor tear it down, clean it up. This project took a fair amount of effort, more than I liked. [laughs] There are these weird things that crop up sometimes, because of all the history here, that Caltech Seismo Lab has built these things and is responsible for various buildings and installations around Southern California, and they do take time.
ZIERLER: Bringing our conversation right up to the present, you mentioned you were quoted in the Los Angeles Times this morning. What's the project, and what was your comment?
HAUKSSON: Our colleagues at Harvard University have been mapping the Palos Verdes Fault. They claim it's about 60 miles long. Previously, we had thought of it as a number of small segments that would only accommodate 6+ magnitude earthquakes. But the 60-mile-long fault they claim will accommodate a magnitude 7.8. My comment was that this earthquake was extremely unlikely and would not happen for thousands of years. The L.A. Times changed that to "it wouldn't happen in our lifetime." It would be quite devastating for L.A. if that 7.8 magnitude earthquake were to happen, and even all of southern California. It could even put the US gross national product down by a percent or so and knock the US right into a recession, the way the economy is going today. [laughs] But that earthquake, it cuts across the L.A. and Long Beach Harbors, so they wouldn't be useful for quite a while, and it cuts across a number of oil pipelines in the area, so there would be a lot of spillages. It would knock down a high-rise or two in downtown L.A. So, it's a pretty serious thing. I was a bit disappointed in my colleagues, however, to say, "Here you go. It's a 7.8 magnitude earthquake." Which is about the same size as we expect on the San Andreas. It's not an inconsequential earthquake.
ZIERLER: Now that we've worked right up to the present, for the last part of our talk, a few retrospective questions and then we'll end looking to the future. First, over the course of your careers—at Caltech, before Caltech—when you started in this business, what were some of the big open, unanswered questions, what has been resolved, and what remains perhaps as open as when you first thought about it?
HAUKSSON: Wow, that's a huge thing. [laughs] I think the big open questions were, we have all these earthquakes; what do they have to do with the geology and the geophysics of the crust? We now understand much better where they are occurring and what faults are actively accommodating them, and what faults are totally dormant and are probably not playing much role in loading of the San Andreas Fault. Now, the big open question is—if you look back 40 years, we have had earthquakes north in Coalinga, earthquakes in the south—the El Mayor earthquake just south of the border. We have earthquakes to the east and west of the San Andreas—Northridge and Landers and Hector Mine. But nothing on the San Andreas. The question is, "Why?" You can go all the way back to Harry Wood in the 19-teens and 1920s; he was looking at, when was the next San Andreas earthquake going to be? And hey, here we are, more than 100 years later, scratching our head, and it hasn't happened yet. So, what is going on? How does the San Andreas work? Why isn't it producing all these earthquakes that earthquake geologists tell us that it has produced over time? What was the second half to your question?
ZIERLER: The questions that have been resolved, and the ones that are still open.
HAUKSSON: The issue with San Andreas is, why hasn't it produced a big earthquake in the last 100 years? That is a huge unresolved question. And how is it being loaded that allows it to get away with that? But what we resolved is a lot about the other faults that have had earthquakes. We resolved where the blind faults run under L.A. Recently our colleagues at Harvard have provided a more detailed picture of the Palos Verdes Fault. We understand I think better how the earthquakes occur in the Salton Sea that might trigger an earthquake on the southern San Andreas. We understand better how the Sierra Nevada is deforming, which is reflected in the earthquake data today. We understand much better how the bend in the San Andreas, where it's more westerly, to the north of L.A., as opposed to, say, up by Coalinga, where it's more northwest, how that bend affects and creates our geography and creates the mountains and the basins.
ZIERLER: In all of your years managing the Network, what are you most proud of?
HAUKSSON: I think what I'm most proud of is that I pushed really hard for getting the new funding and building these different projects to make sure we got a state-of-the-art network out of it. This is one of the few networks, and probably the only regional network in the U.S., where we have more than 22 million people living within the footprint of it. Most places, you've got a million or two, and Alaska, maybe a few hundred thousand, living within the network. I'm proud of the fact that we are able to, with modern instrumentation, provide real-time earthquake information and earthquake early warning in a timely manner, and actually provide the public and the media and the emergency managers with accurate information that they can use to respond to when the earthquake happens.
ZIERLER: On the research side, what have you been able to accomplish in your over 30 years at Caltech that might not have been possible if you were conducting research at another institution, had you stayed at USC for example?
HAUKSSON: With all the data being open, other researchers who are at other institutions can do similar research as I do, and some of them do. But I think the strength of being at Caltech is the interaction with my colleagues, as well as having a fundamental understanding of the data which is always, when you do something yourself, you feel like, yes, I have some inside track here, because I've thought about it, and I was involved in building this, and I know what the data is good for and not good for. Then having my colleagues like Hiroo Kanamori and others to collaborate with and talk to and throw ideas back and forth is something I wouldn't have been able to do at another university.
ZIERLER: Finally, looking to the future, for however long you want to remain active, what do you want to accomplish that you haven't done yet? What is on the agenda?
HAUKSSON: I think one thing that I'm actually working on these days is trying to understand if there are places on earthquake faults where there was an earthquake, say, 20 years ago, and recently there was another one. If these quakes moved the same patch of fault, that would tell us something about how the fault is being loaded, and how fast it is moving at depth, without having to do GPS measurements on the surface. In addition, I would like to understand better this dilemma with no large earthquakes occurring on the San Andreas for the last 100 years. I think that's something that I need to take a much broader look at, not look exactly along the San Andreas Fault, but look at all of Southern California, and see if I can assemble all those puzzle pieces so that I can come up with a model of how it works. A lot of the pieces of this puzzle I have worked on myself, and other people have worked on some of them, so I want to put it all together and come up with a holistic picture of why this is the case. In other words, if we have a big earthquake, what else will have to jostle around to allow that earthquake to happen?
ZIERLER: You're optimistic?
HAUKSSON: Yeah, I'm always optimistic! [laughs] Earthquakes are a natural phenomenon. One generation experiences a really big earthquake, and then you go several generations, and there is no big earthquake on the San Andreas. I would like to do that research study and then have the earthquake, but it may not occur in my lifetime.
ZIERLER: [laughs] We'll see! This has been a terrific series of discussions. I'm so glad we were able to do this for the Seismo Lab history project. I'd like to thank you so much.
HAUKSSON: Thank you so much.
[END]
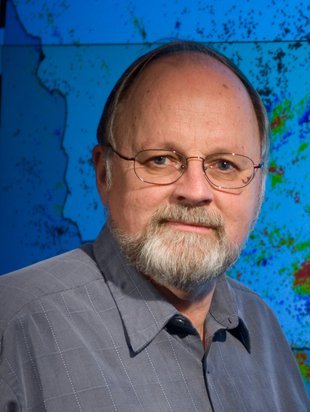
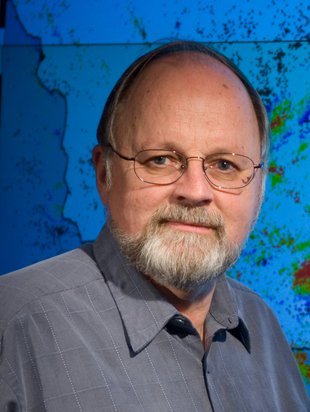
Interview Highlights
- The Historical Seismic Record
- Plate Tectonics and Continental Drift
- Early Warning and Safety
- Next Generation Seismo Lab Research
- A Millennium of Icelandic Heritage
- From Norway to New York
- Mitigating Earthquake Unpredictability
- From USC to Caltech
- From the Analog Era to the Digital Era
- Advances in Real Time Analysis
- The Advent of Seismotectonics
- Geophysics in the Computational Era
- Learning from Old Earthquakes