Frank Verstraete (Postdoc '04–'06), Theoretical Physicist
January 10, 2022
Frank Verstraete's research career speaks to the fact that advances in quantum information theory and advances in fundamental physics operate to mutual benefit. However long it takes to realize scalable quantum computers, the endeavor has already yielded profound and new ways to think about quantum physics. Verstraete's work is centered broadly in quantum many body physics, and he has conducted pioneering investigations in tensor networks and quantum entanglement.
After completing his doctoral degree at KU Leuven, Verstraete focused on quantum optics at the Max Planck Institute in Munich, and he took his second postdoctoral appointment at Caltech's Institute for Quantum Information. He remembers fondly how John Preskill seemed to be the first person to be "genuinely interested" in what he was doing, and that the Caltech culture celebrated thinking about physics—even if it was over beers.
Verstraete went on to the University of Vienna, and then to Ghent University, where his research group is pushing the boundaries in nearly all aspects of quantum physics. His honors include the Lieben Prize and the Francqui Prize.
Interview Transcript
DAVID ZIERLER: This is David Zierler, Director of the Caltech Heritage Project. It is Monday, January 10, 2022. I am delighted to be here with Dr. Frank Verstraete. Frank, it's great to be with you. Thank you for joining me today.
FRANK VERSTRAETE: Thank you very much. It's a great pleasure to help you with this great initiative.
ZIERLER: To start, would you tell me your current title and institutional affiliation?
VERSTRAETE: I'm a Professor of Theoretical Physics at the University of Ghent in Belgium.
ZIERLER: What's your field? What are you currently working on, and what's the general area of physics that you specialize in?
VERSTRAETE: I work in the field of quantum many-body physics, especially trying to use ideas from quantum information theory and entanglement theory to uncover some of the most basic structures in many-body systems. If you look at the history of physics, the central problem in theoretical physics since, let's say, the 1920s, has been exactly this many-body problem. What happens if you put many, many particles together? The Schrödinger equation, the equations of motion of many particles, are understood, but they're just too complicated to calculate. The history of theoretical physics, to a large extent, has been to find efficient approximations of these equations of motion for many-body systems. One of the great visionary insights of John Preskill was to see, basically, that ideas from quantum information theory, especially entanglement theory, could really help in elucidating some central problems there. I have actually been working on this since I met John.
ZIERLER: Is it more that you use quantum many-body physics to inform questions about quantum information theory, or does it actually work the other way around?
VERSTRAETE: It actually works the other way around. I think if you look at the field of quantum information, it has exploded in recent years in terms of trying to actually build quantum computers. But if there's one single direction in which quantum information has already had a huge impact in physics, it is actually in the field of quantum many-body physics. Again, there's only one person that can be credited there with seeing this long, long before anybody else, and that's John. He was saying this to everybody that worked there, "This is the direction to go," and many of my colleagues who have become very successful have also jumped in these same directions. This is, from my point of view, the most tangible result from this whole field of quantum information at the current day.
It's impossible to think anymore of any field of theoretical physics, where these ideas of entanglement, of quantum surface has actually not permeated. It's really in all fields, whether it's high-energy physics, quantum chemistry, condensed-matter physics, statistical physics, people are rediscovering and getting a much better insight into the complicated structures of these problems by using these tools, this new language of quantum information theory that has been kind of developed, to a large extent, by people working at Caltech.
ZIERLER: As a theorist, where in the world of observation or experimentation is there data that's most useful for the questions that you're after?
VERSTRAETE: That's a very complicated question because I'm very, very far off from actual experiments. Quantum information theory has certainly inspired a lot the field of quantum optics, and especially the field of atomic physics, in which people from MIT, Immanuel Bloch, Max Planck, and many other groups in the world, are trying to manipulate cold atoms to create highly entangled states of matter. There are many people all over the world using lots of these ideas we've developed in these more theoretical approaches.
I remember, for example, that when I was at Caltech, John Preskill invited Matthew Hastings to give a talk on Lieb-Robinson bounds, which at that time was something very exotic that nobody had ever heard of. During his talk, we started seeing all kinds of connections with problems in quantum information theory, and ten years later, this is one of the central concepts that people have actually implemented experiments, and we understand many, many new things because of this. This all came because of some discussions after a beautiful talk by Matthew Hastings at Caltech.
Aiming for Fault Tolerance in Quantum Computers
ZIERLER: It's always interesting asking both theorists and experimentalists this question. For you, do we have a quantum computer? And if not, what will it take to get there?
VERSTRAETE: From my point of view, a quantum computer will only be there if you have a fault-tolerant quantum computer, and this has certainly been of the major interests and focuses of John Preskill for a long time. Indeed, you want to build a scalable quantum computer, you need fault tolerance. Actually, there's quite a nice anecdote with this. John Preskill is the most likable person that I know, but I got him angry once. We were having a dinner or something, and I like to provoke. At that time, I liked very much to provoke a bit, and I was trying to provoke John by saying, "Why are you working on this fault tolerance? It's an engineering problem. We know it's possible in principle. Now, it's for the engineers to actually do this. This is not really physics. You're supposed to work on really deep problems." And John Preskill got kind of angry and said, "No, no, no, this is actually really interesting physics." And of course, he was right. But I still remember vividly that he didn't like at all to be called an engineer. [Laugh]
ZIERLER: This is as much a cultural question as it is a scientific question. In the United States, quantum computing is often thought of as something like a horse race in terms of who will get there first. Is there a similar phenomenon in Europe?
VERSTRAETE: Certainly, I think the development of quantum computing has run in a very parallel way in Europe and the US. If you look at the very first realizations of quantum computers, I'm thinking about ion traps, the theoretical proposals actually came out of European scientists, Peter Zoller, Ignacio Cirac, these kinds of people. Then, actually, this was immediately implemented by people in both. I think these developments have always run very much in parallel, and as far as I understand, the jury is still completely out what technology will actually win. It's clear that we will be able to make machines with a few hundred qubits in the coming years, but maybe this is not enough. Maybe we need millions of qubits. Many of these technologies being pursued these days are maybe not scalable.
I'm personally not convinced that ion trap computers are actually scalable to one million qubits. The same for superconducting qubits. There are lots of technological problems that come into play when you try to scale these things up. I don't really understand the sudden huge investments that are being done in this field. I don't see any huge leap in knowledge or technological discoveries that justifies the humongous investments that are being done now relative to ten years ago. From my point of view, I think we are still very far from this scalable, fault-tolerant quantum computer. What's pretty odd, in some sense, is that I started working on quantum information theory around '98, '99, and I still remember very well that when I was speaking to experimentalists but also experts in the field, they always said, "Well, in ten years, we'll have a scalable quantum computer." Then, ten years later, they say, "In ten years, we'll have a quantum computer." Now, again, more than ten years later, it's the same.
This ten-year timeframe seems to be a constant in the field of quantum information, which might actually look a bit depressing, but on the other hand, this is only if you look at the premise of actually building quantum computers. What is beyond any doubt is that this whole field of quantum information has led to a completely new way of looking at physics, and that kind of goes back to what I was saying before, that the most tangible results or consequence of the most tangible results that have come out of the field of quantum computing is that it allows us to reformulate and look at quantum physics and quantum many-body physics in a completely different way. From my point of view, this alone already justifies this humongous interest in the field. It's a bit odd that this aspect is typically not stressed too much.
Of course, it may not sound that good for funding reasons or other reasons, but certainly, as of now, this is the main legacy of this whole field of quantum information. This legacy was actually pointed out or first kind of seen in a very clear way by John Preskill in a paper he wrote in, I think, '99, a paper that's very important from a historical point of view, in which he basically says, "Quantum information and quantum computing look great, but the real impact it will have is actually in other fields of physics."
ZIERLER: Is that to say that we really don't know yet on an existential level what quantum computers will be good for, even if we don't have any understanding of how we might actually get there?
VERSTRAETE: The way I look at the quantum computer is, it's effectively a universal lab. There are many, many different labs all over the world where quantum physics experiments are being done, be it condensed matter, be it atomic physics, be it pharmaceutical companies, in which they basically make chemical reactions. There are many different disguises. People are trying to understand how these chemical or nuclear processes work. What the quantum computer, in principle, would be able to do is to simulate all these possible experiments in one machine. It is, in many ways, a universal experiment. It allows you to do basically any experiment in which quantum mechanics plays an important role. And in that respect, in a very long time, it will be a huge deal. Certainly, for companies like pharmaceutical companies, for catalyzers for chemistry.
There are many possible applications of being able to simulate an arbitrary quantum process, and especially the chemical processes that are being done. I don't know the exact number, it's a bit difficult to figure out, but there's certainly a very large fraction of all supercomputers in the world simulating quantum many-body systems all the time. And these systems are actually using classical methods for simulating quantum systems, and in many cases, this leads to something like exponential slowdown. That means that, of course, with the quantum machine, this whole part of simulations, you would be able to do them with a humongous speed-up. But even many other kinds of applications we have not been able to dream of doing, we would suddenly be able to do with quantum computers because there are lots of processes and non-equilibrium physics problems, for which we don't have an answer, we don't know what happens because we cannot simulate it. There's a lack of methods for understanding how to deal with them. To a large extent, this is where my own research fits in.
By being in Caltech, but also before, and of course, many, many years after, I was still thinking about this, we were very much motivated to try to understand what you can do with a quantum computer that you cannot do with a classical computer. Where's the line? What problems are really hard for classical machines and easy for quantum computers? I was a post-doc with Guifré Vidal, and I'm sure that John recommended him to be interviewed, and we were in the same office, but we were really competing with each other. But it was the perfect environment, of course. We're really trying to push classical algorithms, new ways of understanding the quantum many-body physics from the point of view of this new language of entanglement theory.
And this has allowed us to devise classical algorithms for simulating quantum systems we thought would be impossible to do. It's very difficult. We still don't know the line. We still don't know what a quantum computer is good for versus classical computers. But the great thing of this whole field of quantum information is that it gives you tools to do these things better classically. It leads to a completely new way of understanding, I would say, the central problem in quantum physics.
ZIERLER: Perhaps to elucidate what quantum computing might be good for, when you look at classical computing, what are some of the obvious limitations for which, theoretically, quantum computing might provide answers?
VERSTRAETE: I would say that, of course, there's the obvious example of factoring. This is the problem that kickstarted the whole revolution of quantum computing by Peter Shor in '94, when he showed that with a quantum computer, you would be able to solve a problem for which you need exponential resources to do it with classical computers. Of course, this opened up a humongous interest in many, many people, trying to figure out other algorithms that would allow you to have these exponential speed-ups, but this has turned out to be an extremely frustrating endeavor, I would say. From my subjective point of view, there has not been a single other computational problem that is relevant from the point of view of computational complexity for which you have an exponential speed-up that has potentially the same impact as that factoring problem.
But that's because to find problems that are amenable to the power of quantum computers, you must find a structure in these problems. There must be a very nontrivial structure, some periodicity or some group-like structure that you uncover in them that quantum computers can exploit because you can do free transforms very efficiently, certain types of operations that figure out irregularities in the data in a very peculiar way. But it has turned out to be very complicated to come up with problems that are relevant classical problems of computer science that you can involve in that type of framework. In some sense, this factoring problem seems to me like some beautiful kind of flower out there, a very exotic thing, that's certainly not what quantum computers in practice will be used for what they will be used for, as I said before, is effectively simulating quantum systems, trying to solve the quantum many-body problem, trying to understand how, for example, high-Tc superconductors work.
How can we make materials that will be superconductive at room temperature? If this would be the only thing the quantum computer would be able to do, allow you to come up with a superconductor at room temperature, it would already basically justify the investment of trillions of dollars. It would change everything. It would be amazing. In that sense, there's this enormous potential, but of course, we don't know whether this will be realized.
ZIERLER: You mentioned high-Tc superconductivity, which the value from an applied level would be incalculable. What about more theoretical areas of physics? For example, particles that finally break the standard model or achieving a quantum theory of gravity? Do you see a role of quantum computation in some of the larger intractable theoretical challenges in physics?
VERSTRAETE: Sure. Quantum computer could be seen as some new device that the computational physicists would use. But nevertheless, this is not enough. It's not because you can simulate something. It would be more like a black box that this actually leads to understanding. Again, this would be extremely valuable, but on its own, it's not enough. It's not because you can simulate some problems in quantum gravity that you can understand them. In that sense, it's not entirely clear to what extent it's enough to have a quantum computer that we will solve quantum gravity. We'll have to have a theory that actually explains this and allows you to understand these results that come out of the quantum computer. I remember an extremely heated discussion I had with John Preskill and Das Sarma, a famous professor from Maryland. The question was, have quantum computational physics ever led to a real big breakthrough in physics? In a sense, what is the intrinsic value of computational physics for understanding things?
Again, I was provoking a little bit, especially Das Sarma, and John Preskill was like a moderator. [Laugh] It completely escalated. Das Sarma started shouting that, "Of course, computational physics is, like, the most important thing ever." But it's actually very difficult to come up with examples of things that have been discovered because of computational physics. It's not so clear-cut. Physics is about understanding things, uncovering irregularities and writing down very simple explanations of very complicated phenomena. From my point of view, this is what physics should be about. Of course, a quantum computer or any interesting classical argument that simulates quantum many-body systems is very interesting, but it's only a small piece of the puzzle. In the end, you need to have the theoretical understanding of what is going on as a whole. Many people are currently very excited, for example, about these neural networks, deep learning, these new ways of trying to do physics. To me, this is still not clear at all whether we will be able to do big discoveries in physics using such a black box method. It's not at all clear that just being able to simulate things is enough to understand things.
ZIERLER: Some technical questions. First, what are tensor networks?
VERSTRAETE: Tensor networks is the main topic of my research. It's a way of organizing or parameterizing quantum many-body systems. One of the central concepts in quantum physics is, there's something like a tensor product structure. If you take one particle, and you take two particles, three particles, the dimension of the Hilbert space is always growing exponentially. It means that if you have ten qubits, you have a Hilbert space of dimension 1,000. If you have 20 qubits, it's a Hilbert space of dimension of a million. The fact that this grows so fast is one of the main reasons that quantum physics is difficult. You cannot parameterize or write these wave functions down anymore in any meaningful way if you would have to write down all these coefficients for all possible amplitudes. It's an exponential problem.
I think if there's one nice thing that came out of this whole COVID saga, it's that everybody understands now what exponential means. [Laugh] There's this exponential explosion. This whole idea of tensor networks, how we came up with this, is trying to find new ways of parameterizing or representing these complicated many-body wave functions. How is it possible to write down a wave function of, let's say, 100 qubits, without having to specify all possible wave-function amplitudes? What we do is effectively split up these big wave functions into small chunks, and then we connect these chunks to each other. As a whole, this leads to a very efficient parameterization of your big wave function because you connect small entities to each other. The question is, why does that work? This is exactly where this whole theory of quantum information and quantum entanglement kind of comes along. The theory of quantum entanglement, and especially things like these Lieb-Robinson bounds that I talked about before, have allowed to understand–and indeed, relevant wave functions, wave functions that actually matter, have lots of structure.
There's a paper I wrote that came completely out of discussions I had with people at Caltech tries to understand what the physically relevant wave functions in the universe are. You have this exponentially big Hilbert space, and the question is, what part of that Hilbert space is actually physical, and what part of that Hilbert space can nature or quantum computers explore? There's one thing that is completely under-appreciated by people who do not have a deep understanding of quantum information theory or quantum computing is the fact that the size of your Hilbert space is not really exponential in the system size, but the right way of counting the size of Hilbert space is as something doubly exponential. What I mean by doubly exponential is that you would say, "How many states can I create that are distinguishable?" You have wave functions, but wave functions don't have to be orthogonal. Wave functions cannot just be close to each other, but you can really distinguish them.
And of course, with a quantum computer, you want to make wave functions that are close to each other but not equal to each other because you need this during your quantum computation. What you can do is something like counting. You can start counting, "How many different epsilon balls can I put in my huge Hilbert space?" If you do this, you can see that you can fill your Hilbert space with doubly exponential, an exponential of an exponential, amount of different states. Now, you can start to count how many states you can actually create with physical processes, systems that have Hamiltonians in which only two or three particles interact. Then, you see that the amount of states you could visit or ever create in any meaningful time is only exponential in the system size. That means that, actually, the whole Hilbert space is, in some sense, an illusion. We have this humongous Hilbert space, but almost everything in it is an illusion because you will never be able to make a state even anywhere close to most of this Hilbert space.
That means that to do physics–and this is very much a quantum information idea–that meaningful physics happens on a very low-dimensional manifold in this huge Hilbert space. These tensor networks are a very specific realization of that. If you go a little bit further and say, "I'm only interested in ground states of many-body wave functions," it turns out that there's actually an efficient classical description of these relevant wave functions. Trying to understand this puzzle and actually collaborate with Bruce like that was certainly one of my main works when I was at Caltech, and I've kind of continued to work on this ever since. Again, John Preskill was instrumental there to always ask the right questions. He was the one who pushed us to think further, to really think about questions like I just explained. "What does this illusion of Hilbert space mean? How do you show this? Why is it that it's possible to do this?" This is the best possible mentor, of course, I could ever have had.
ZIERLER: In the context of quantum computation, what does the term dissipation mean?
VERSTRAETE: Dissipation is actually the main source of decoherence. Dissipation effectively means that you have a quantum system, and when this quantum system is not perfectly isolated, it couples to the environment. Your quantum system becomes entangled with the environment. For example, it could be that some photon comes along and excites some atom in your system. Basically, you've coupled the electromagnetic field with your quantum system, and now you're entangled. Of course, you don't have access to the full environment, and therefore, the technical term of this is, you have to trace out the environment. The result is that the evolution of your quantum system is actually not described by a unitary evolution, a Hamiltonian evolution, but actually something called the Lindblad equation. This is what dissipation is, effectively entanglement of your system with the environment, but you don't have access to the environment. Therefore, you kind of have to throw it away, and this leads to decoherence. Instead of having a nice, pure-state, unitary evolution, you start dissipating and creating entropy. Once there's too much entropy, the whole system will become completely useless for quantum computation.
ZIERLER: When you worked on developing a quantum generalization of the metropolis algorithm, is that to say that there were classical generalizations of the metropolis algorithm?
VERSTRAETE: The quantum metropolis algorithm, I still remember very, very well. I started working on this at Caltech in, I think, 2004. It took the longest time to actually get it. I still remember vividly talking with people like Andrew Childs. We were talking, not every day, but from time to time about how to do a quantum metropolis algorithm. We couldn't figure it out. But I kept thinking about this from 2004 until 2009, 2010. Finally, somehow, we were able to crack the problem. But this is one of these problems that you have to kind of go back to that problem year, after year, after year, then suddenly, you see the light. Why do I think this quantum metropolis algorithm is relevant, and what does it actually do? If you look at quantum many-body systems, these are classical particles, statistical mechanical particles. Think about cooling down some liquid, and it will kind of crystallize in a crystalline structure.
The questions people ask and solve using classical metropolis algorithms, are, what is the equilibrium's crystalline structure? I put this and this molecule together, I cool it down, in what structure will this system crystallize? What space group will be realized by that cooling down? These turned out to be very complicated in classical statistical mechanics, unless you solve them with Monte Carlo algorithms or metropolis algorithms. This means using effectively using randomness to basically mimic a heat bath that would thermalize many, many, many orders of magnitude faster than a real system. These metropolis algorithms are so successful and have completely acquired a monopoly in solving problems in statistical mechanics of classical particles. It's basically the only tool that still is used because it's so efficient, and it works so well for simulating many-body classical systems. The problem, if you try to do this for a quantum system, is that these quantum amplitudes will give rise to something like negative amplitudes.
There's no direct generalization possible of this metropolis algorithm to simulate quantum systems, at least not quantum systems with the sign problem, because these are problems for which you would need negative probabilities or complex probabilities. And of course, that doesn't exist. A probability has to be positive. What you want to do is effectively sample eigenstates of a certain Hamiltonian. People like Peter Shor, Alexei Kitaev, and many others have developed a beautiful and extremely important formalism of phase estimation, which is effectively a way of preparing eigenstates of a certain Hamiltonian. This quantum metropolis algorithm actually works by going from one eigenstate of this Hamiltonian to another one, and then you compare if the energy is going up or down. Depending on this, you kind of make the jump or go back, just like classical algorithms work, but the big difference is that in the quantum case, these wave functions are very entangled. In the classical case, all the possible configurations are always product states, very simple states.
That's why you really need a quantum computer to be able to do this. Why did it take so long to devise or solve this problem? It's because there are many, many obstacles that have to be overcome in quantum mechanics that, at first sight, look completely overcome-able. For example, one of the big things in quantum mechanics is something called the no-cloning theorem. You have a wave function, and out of that, you make a new wave function. You compare the energy, and if the energy goes up, you don't want to accept it, you want to go back to the original one. How do you do something like that classically? You just keep the information of the state that you came from. If the energy goes up, you just go back to where you came from because you have a copy around of what you had before. In quantum mechanics, that's impossible. There's no way of going back once you do the measurement. It's irreversible. You cannot go back.
But using some tricks, especially this use entanglement to overcome these kinds of things, we were able to understand how this could be done using quantum mechanics. This is one of these problems of these lines of ideas to develop or understand this, for which you need this very inspiring environment of Caltech, where you're continuously forced to think about how to use entanglement to solve this problem. When I was there, 2004 to 2006, was the golden age of entanglement theory. Nowadays, people are a bit less concerned about this because, in some sense, all the low-hanging fruits have been kind of picked off. But at that time, this was the most inspiring and, I think, very deep direction of research we were all very much concerned with. We were also very much motivated to think about problems like that by John.
From String Theory to Quantum Information
ZIERLER: To establish some intellectual context to build up to your time at Caltech, a generational question. With John Preskill, who came from cosmology into quantum information, by the time you were in graduate school, was quantum information sufficiently mature that this was a viable field to focus on for you?
VERSTRAETE: As most people interested in theoretical physics, when I started graduate school, I thought, "I want to do string theory. I want to do the really tough stuff, and I want to be the new Einstein." But I had a very, very smart advisor who told me, "Yes, but it's very, very difficult to make an impact in a field like string theory because there are too many smart people working on it. It's already an old field. What you have to do is to start working in this completely new field." This was around '98, '99. "Start looking in this field of quantum information theory, quantum entanglement theory, and try to understand whether you can do some interesting physics there." That was the only thing my advisor ever told me, actually, but that was extremely good advice. How do you start something like that? My professor himself didn't know anything about quantum computing, but he told me, "This seems to be a new field with a huge amount of potential."
I started looking around for some lecture notes and stumbled upon the notes of John Preskill. I learned everything I know about quantum information theory from this amazing set of lecture notes that he put online by the time I started graduate school. And they are actually amazing. They're very different from other lecture notes I've ever seen about quantum information theory because it's really not just quantum information or quantum computing, it's also about physics. Really trying to understand or explain the connection with physics and trying to illustrate why all these ideas are really very deep. As far as I understand, John Preskill has a contract that probably has been prolonged for 25 years to publish these lecture notes in Cambridge University Press, but I'm not so sure when they will finally be published. But this is the way that my generation learned quantum physics or quantum information theory, by this amazing set of lecture notes from John Preskill, in which he was asking the right questions, being very visual, and making connections with different fields.
And that's what you want as a graduate student. You want to be stimulated to think about connections with other things. At that time, I was kind of lucky that I had a background that was a bit different than most physicists because I was also an engineer. I knew quite a bit of linear algebra. Linear algebra is basically trying to understand eigenfactors, eigenvalues of matrices. And superficially, linear algebra looks very simple, it actually goes very deep. Many of the most complicated things in mathematics, when they are understood, in some strange way, you can bring them back to some linear algebra problem. The problem of solving nonlinear sets of equations using Gröbner bases and all these very complicated things, in the end, the way you solve it is by bringing this back to some linear algebra problem. I was reading these lecture notes by John Preskill, and I figured out that actually, my background of linear algebra would allow me to solve problems there that people had not thought about.
This is actually how I came into contact with people at Caltech, especially Hideo Mabuchi. At that time, he was an extremely high-profile and extremely young professor. I think he was maybe only 25 years old, and he was a quantum optics professor at Caltech. He was really a big deal. He was the guy. He spoke extremely fast, also. You would talk to him, and he would immediately understand what you said and come up with new ideas. Now, he's at Stanford. But I got into contact with him, and he was also very interested in these problems of quantum information, he was attending these weekly seminars. I'm talking about when I was a graduate student in Belgium. Hideo invited me to come to Caltech. This opened my eyes completely because I was attending these group meetings of John Preskill. I still remember, it was in this high-energy physics building, Lauritsen, a big room with sofas around the room. This was amazing, this electrical atmosphere.
Everybody was asking questions, pushing each other to think about problems about error correction and entanglement. Everybody was interested in everything. There was an amazing atmosphere that I've never seen anywhere else. Somehow, every year, I was able to visit because John Preskill was extremely generous with inviting young physicists to just be there. "Come and visit me for a month, work on some problems." He invited people from all over the world to do this. For me, this is how I really started thinking about interesting problems. There are many people who can solve interesting problems, but the really difficult thing is to come up with the interesting problems. This was always very clear that this was the emphasis of John Preskill. John wanted people to work on interesting problems. Once you understood how to formulate a problem that is solvable, many people would be able to solve it. But you first have to ask the right question. This was, in some sense, what made Caltech so unique, that people were continuously pushed to think about the right questions.
John was the perfect catalyst for doing this. In all the years I was there, but also before and after, John never wrote any papers with any of his post-docs or even his students. Nevertheless, he's, of course, on all the acknowledgements. He's the guy who stimulated people. If you worked on a problem, he would ask you the right questions that would allow you to see much further and see the relevance or other directions you would have to move in. John was an amazing, amazing figure in creating this whole atmosphere such that people would kind of see new things, work on new things. At some point, I think, there was not a single professor in the field of quantum information in the US that was not, at some point, a post-doc with John.
ZIERLER: Just to clarify the chronology, did you initially visit Caltech when you were a graduate student or only as a post-doc?
VERSTRAETE: I visited as a graduate student. I was there I think two or three times for a month. Then, when I graduated, I applied to two places for a post-doc. One was Caltech, and the other was the Max Planck Institute in Munich with Ignacio Cirac, who's one of these giants in the field of quantum information. He has a very unique style of doing physics. On one hand, he's very close to experiments. On the other hand, he's very, very mathematical. He has two completely different things and basically divides his time between these things. At that time, in 2002, he had just become the Max Planck Institute director, and he was looking for people to work with. I had the feeling that this was actually the right time–Ignacio Cirac is one of these very few geniuses that is also willing to work with people.
There are other people who are geniuses, and I don't want to say anything bad about anyone, but people like Alexei Kitaev, who are amazing, but with whom it's very difficult to very exchange ideas and collaborate. Ignacio Cirac is the opposite. He's always full of ideas and ready to discuss. I remember it was very difficult for me to choose because I applied to two places, and I had two offers. I could do a post-doc at Caltech or Max Planck. I decided first to go to Max Planck because Ignacio Cirac is really somebody who knows things I have no idea about. He has knowledge about quantum optics but also about physics that's clearly very important to know. I had the feeling I would learn more, in some sense, than at Caltech. Because at Caltech, although people talk to each other, it's very individualistic. And the professors at Caltech would not really collaborate with each other because they're too smart for that. They have to be the experts in their fields, so they should not collaborate with each other.
I've never understood this attitude, but it's not just unique to Caltech, but to all the top universities in the US, that as a professor, you have to be the top of your own field and not collaborate too much with your colleagues. But Caltech is a great place to be if you already have in mind exactly what you want to work on. And this is what happened. I did a post-doc for two years at Max Planck, where I started working on these tensor networks by coincidence, actually. Then, after two years, I moved to Caltech, where I continued working on this and was able to kind of open up the scope of why this would be interesting. It was only at Caltech that we realized how relevant this could be. That's also when I was sharing an office with Guifré Vidal, who had also been working on these tensor networks from a completely different point of view.
ZIERLER: Given that your graduate advisor was not a specialist in this field, when you spent those extended periods of time at Caltech during your doctoral work, did John serve as sort of an unofficial thesis advisor to some extent?
VERSTRAETE: That would be a little bit too much, but I remember him being extremely–it was the first time somebody was genuinely interested in what I was doing. This is, of course, enormously important because I was a graduate student from a completely unknown university. My advisor was not a big-shot, and he didn't know anything about quantum mechanics even or quantum computing. He was actually a control theory person who wanted me to work on quantum control. Then, to go to Caltech and be able to talk with this person from whom I learned everything about quantum information from was a completely eye-opening experience. To see that this person was genuinely interested in what I was doing and was pushing me to go further, in some sense, you could say he was actually much more important than my advisor. But without John, I would not have been in this field at all, in some sense. I would not have been able to ask the right questions and go in the right direction. That's what Caltech taught me during my graduate studies, coming up with interesting questions.
ZIERLER: What were your initial impressions, both of Caltech and IQI when you first arrived as a graduate student?
VERSTRAETE: It may sound very strange, but if I think about Caltech–and I think this was my very first impression of Caltech–it's that it smells so nice. [Laugh] They have these flowers. I forgot exactly what they are, but every time I walked to my office, I walked past some beautiful flowers that smelled so nice. This is what I think about. For me, Caltech is like this dream place, this very elusive, small island in which you can completely focus on what you're interested in. Everybody there is completely focused on doing research, and you don't have to be embarrassed to claim that even at night or after beers, you're still talking about physics. For people like me, this was a dream. Here, in Belgium, you're looked upon as a complete nerd if you do this. But there, this was the norm. This was what was possible.
Of course, this is very general. The reason that IQI worked so phenomenally well was this one invention of John that everybody in the world has tried to replicate, and nobody really manages to do, these group meetings. You must have heard of these weekly group meetings. I think it was Wednesdays at 5 pm. This was the time that everybody, all the post-docs, PhD students would come together in one big room, and everybody would have to say in two minutes what they've done. "What are you working on? What kind of problems are you interested in?" At first, I didn't get why this was so relevant. But in retrospect, this was so ingenious of John because what you see is really how ideas mature. Everybody, every week, is talking about what they've been working on, and you see ideas evolve. You see how the different ideas people are working on start somewhere and end up in a completely different place, but much more interesting than where they started from.
To understand how this research works is one of the most interesting and amazing things you can see as a researcher, trying to understand how this creative process works. This was what happened at these meetings. Everybody talked for a few minutes, went to the blackboard, drew pictures, and explained. And John would always ask the right questions. This was unbelievable. John was always sitting in his one chair that nobody else could sit in, and he would be there taking notes on everything that was said. After people spoke for a few minutes, he would ask a question, and it would always be exactly the kind of question that the speaker would not immediately know the answer to, but let the speaker kind of think about a completely new avenue. This was the magic of these meetings, so we all loved this. Everybody at Caltech has tried to replicate this in their own groups. I also tried, of course, and I still try. In my case, it doesn't work at all as well as what John did. Why? Because John was this person who everybody looked up to.
My students all think I'm always bothering them with questions. I like to always engage them, and they don't take my questions very seriously. But John was this completely different figure. Everybody had the utmost respect for him, and when he said something, everybody was always immediately silent. I don't know exactly how it went, but this was an amazing experience. Of course, this was very stressful for the new PhD students. They would have to talk about on the spot what they had been doing. Of course, the other students and post-docs would bombard you with questions. If you would say something wrong, you would have known. What it forced people to do was to make sure that by Wednesday night, you had some interesting things to say. John was sitting there, and you didn't want to disappoint John in his big chair. [Laugh] This was like the focal moment of the week for everybody because we would learn what everybody else was working on, be bombarded with new ideas, and see how this giant in physics, John Preskill, would ask questions, give ideas, and connect people with each other. That was what he saw as his role, synthesizing what other people did.
I don't know anybody else, any other physicist, who's able to do it in such a simple way. When you explain what you're working on to John, after a few minutes, the discussion may end, and it may take a few hours, but you will always understand the problem much better than before you talked to John. He has this amazing quality that you can explain to him anything, doesn't matter what, and it's clear to him what you're working on. After a few minutes, you will understand the problem much better yourself. I don't know any other physicist with that vision or kind of generosity. Because at the time, of course, this is being extremely generous. He has always been extremely generous with his time, with connecting people, writing reference letters. All these people got positions because John generally took the time to explain what they did and why he thinks these people do interesting things.
John is certainly one of the central figures that are needed for a field to be happy. People like John connect different people who might be competing with each other, but because of his attitude somehow, now, we don't see each other as competitors but colleagues who work together. John is one of the central figures in the field of quantum information that makes the field so nice to work in. It's difficult to explain exactly why this is, but he's this giant figure who towers above everything and makes sure the field works.
ZIERLER: When you initially came to Caltech, organizationally, was IQI already in existence, or was it essentially just John at that point?
VERSTRAETE: I still remember I have a hat of IQI. [Laugh] One person who was very important to IQI is the secretary, Ann Harvey. She was extremely nice. When I came there, I had a wife and three children very small children. They were, like, 1, 2, and 3 years old. Of course, I was completely lost. I had no idea what to do. But Ann was extremely efficient at organizing things, helping around, getting stuff for us. From an organizational point of view, I think she was the secretary for IQI. IQI must've been around then in 2004 to 2006. But there were many professors already involved like Jeff Kimble, Hideo Mabuchi. I don't think anybody from condensed matter was part of it yet. There were some computer scientists. Of course, Alexei Kitaev was there, but he always remained some elusive figure.
From time to time, he'd pop up in a seminar or something, but most of the time, we didn't see him. He was thinking too deeply on different problems. John Preskill is the one who kind of saw that Alexei Kitaev was a genius. Nobody else had seen this, but John saw it very clearly and said, "He has to be at Caltech." There are these amazing anecdotes of people who were so excited because Alexei had asked them to come to his office. I think it was Norbert Schuch. He was asked to go to Alexei's office, and he thought Alexei was probably interested in his recent paper. Then, Norbert goes into Alexei's office, and Alexei is saying, "Look, I saw somehow this figure that you made in your paper. What kind of code did you use for making that figure?" That's the only thing he was interested in, not the physics, just the practical thing of how to make such a figure. [Laugh] That's the way Alexei was, this elusive figure in IQI. We all knew he was a genius, but we did not really have contact with him.
ZIERLER: When you accepted the offer at Max Planck, did you understand at some level that you would still have an opportunity to do a second post-doc at Caltech? Did that make the decision easier for you?
VERSTRAETE: I don't really remember that. I certainly didn't have a lack of self-confidence. [Laugh] I probably imagined that if I kept on doing good work, John would probably still hire me. But of course, there was no guarantee at all. If I look back at the decision I made, I think it was certainly the right decision for me. At Max Planck, I was very privileged. I had an office exactly next to Ignacio Cirac, and I think we talked every day for something like two, three hours. Really, every day, three years in a row. Every day, we were kind of discussing, for hours and hours, in his office. Actually, the other post-docs were kind of angry at some point at me because he didn't have time for them. But that was Max Planck. Then, for me, the perfect next step was to go to Caltech, where I could work and think continuously. Because then, I had many, many more problems to work on that I would have time to solve.
I would have a very clear vision, I'd talk with John, he would give me ideas, I'd talk with people like Andrew Childs, like David Poulin, like Guifré Vidal, many other kinds of extremely interesting people. That would completely make this direction much more complete and would form the basis for everything that I have been doing actually since then. For me, this was actually the perfect job. Being a post-doc at Caltech, without having a clear vision of what problems you work on, was actually hard. In some sense, you're continuously kind of bombarded with ideas, but nevertheless, it doesn't mean that everybody had it easy. There were certainly some post-docs for which there was no clear line of research that must have felt very frustrated.
Because of these Wednesday meetings, there's a very clear kind of hierarchy there. There are people who always have these amazing results, then there are people who struggle very, very deeply with some problems and are maybe never able to solve them. All these things also have a lot to do with luck. Nevertheless, I would say Caltech was a fantastic place if you were lucky to be good. If you were a little bit less lucky in the sense that you didn't find exactly the problems you would work on, I think it must've been a very frustrating place.
A Golden Era in the Field and at Caltech
ZIERLER: You mentioned this was a golden era, 2004 to 2006, with low-hanging fruit. What was the low-hanging fruit at that point?
VERSTRAETE: This was the very first time that you could think about entanglement, that the language was developed to understand quantum correlations in a new way. If I look at the papers I wrote at Caltech, many of those were obtained in a very quick way. Of course, if you come up with a result in a week, it must be a low-hanging fruit. You come up with a result, you write the result down, and after a week, maybe a month, it's already on the archive. That means from a technical point of view, it's not very hard, but it does not mean it's not interesting. I remember Yi-Kai Liu came and gave a talk about some problem about understanding the complexity of finding reduced density matrices. After the talk, we were discussing it with John and with Yi-Kai, and you immediately come up with a new problem. Basically, a week or two after, you already have a draft of a paper in which you've solved a new problem. This is kind of characterizing the computational complexity of one of the central problems in quantum chemistry called the N-representability problem.
I'm not saying it's a super important problem, but nevertheless, it's one of these ideas that belongs now to this canon of what is difficult, the kinds of problems you can solve efficiently with a quantum computer, the problems you cannot solve. The same thing with these Lieb-Robinson bounds. Matthew Hastings was giving a talk about Lieb-Robinson bounds and how they could be used to understand the decay of correlations in quantum many-body systems. Nobody had ever heard about this. I was sitting in the audience, and I was, at that time, thinking very hard about the complexity of preparing topological wave functions, wave functions that exhibit something called topological order. This was something Alexei Kitaev, together with John, had worked a lot on, and they have a beautiful paper on this. I was trying to understand how hard it is to create states that have such a topological order. We listened to this talk, Matthew Hastings was kind of giving ideas that fit exactly in the line of thought that I needed to solve this problem.
After that talk, we sat together with Matthew Hastings, and Sergey Bravyi joined, and we solved the problem in basically a few hours. Now, people say this is a very deep idea, but I don't know whether it's deep because we solved it in a few hours, so it cannot be that deep. But it kind of exemplifies the fact that this was the golden age. There were these completely unexplored ideas that you just had to put the puzzle pieces together, and suddenly you understand something you'd had no clue would be relevant. I had no clue that these Lieb-Robinson bounds would be relevant for understanding the complexity of preparing topological phases of matter. This was something I could never have dreamed of unless I was surrounded by this atmosphere of Caltech, in which we were always thinking about new things and new directions, people are coming and giving talks, and discussions ensue after these talks. There are many more examples I could give you like that. That's why I think it was a golden age. I think it was Dirac who said, "A golden age is when third-rate physicists can make first-rate results," or something like that. I forgot the exact quote.
ZIERLER: To clarify, are you saying this is the golden age retrospectively, or did it feel like the golden age in real time, as you were at Caltech?
VERSTRAETE: It didn't feel really special because it was just normal. It was normal that you would come up with new ideas or problems every week or two. This was the modus operandi there. The people who were there, like Sergey Bravyi, David Poulin, Guifré Vidal, they would always have new ideas. In that sense, we didn't feel that this was so special. It was completely normal to us that this happened. Indeed, I don't think we realized this was a golden age.
ZIERLER: What kinds of collaborations did you do when you were at Caltech? Who were the key people you were working with, and what publications came out as a result?
VERSTRAETE: Strangely enough, when I was at Caltech, I wrote very, very few papers with people at Caltech. I'm certainly not the only one with that profile. I think we discussed a lot with each other, but that doesn't mean we wrote papers together. We all had our own fields and visions of what we were interested in. But for one reason or another, we didn't write papers together. I've never really understood why, but it worked very well. I was sitting in the office with Guifré Vidal, both of us working basically on tensor networks, but we had our different approaches, and we didn't write a single paper together. Although, we knew very well what we were doing, and I was very much inspired by discussing with him, and he was very much inspired by what I was doing. In that sense, there must've been some atmosphere where we were maybe competitors in one way or another. It's only because you're asking that I'm thinking about this. I've never thought about this before. We all liked each other very much, but nevertheless, we were all, in some sense, competitors. There was a very healthy competition there to be the best.
ZIERLER: What did the competition look like? How do you quantify or define being the best? Is there a race? Is there a depth of understanding? How do you measure such things?
VERSTRAETE: Very good question. You feel it. One of the strange things in physics is that, especially in doing research, and certainly research in a new, novel direction, your gut feeling and intuition are so important. You don't really understand why, but you just feel that something is the right direction, and you go there. In the same way, if you discuss with somebody, you can just feel that this person really has a deep understanding of this topic and sees what nobody else sees or can solve problems that nobody else can solve. That's how you quickly see this. But of course, this might also be completely misleading. I remember, for example, when I was a post-doc, Sergey Bravyi was also a post-doc there, and I remember thinking, "Sergey is such a smart guy. Why is he working on these problems that look completely uninteresting?"
But in retrospect, the problems were extremely interesting. Ten years later, everybody was working on this magic state distillation and these kinds of things. Of course, we make lots of mistakes. You can be completely wrong. It's not that one person or the other was the best. We were all very good. I think we were all kind of aware that we were good, doing good science. It's not that one was better than the other one, but we had our own approaches. That's probably why we didn't really collaborate together. I don't know. But there was certainly an amazing atmosphere there.
ZIERLER: Was your feeling that IQI was an island? How well integrated was it within Caltech? Mathematicians, condensed-matter physicists, who else was in and out of the IQI world at Caltech?
VERSTRAETE: It was a bit of an island. There was certainly some discussion with computer scientists that were interested in quantum complexity theory. The core of IQI was certainly the post-docs. We were all together there, and we had all the time together to discuss. Basically, everybody was a physicist, and we were really on an island. For us, it didn't matter because we had extremely interesting discussions. But I remember the PhD students were quite frustrated. They were in Lauritsen, this high-energy building, all John's PhD students, and they felt they weren't in the middle of the action in this office where all the post-docs were discussing things, writing on the blackboard. It wasn't so ideal for PhD students.
ZIERLER: What did you learn at Caltech, both scientifically and just working in that environment, that was so important for your next opportunity, when it was time to move on from Caltech?
VERSTRAETE: I think the most important thing I learned at Caltech is probably the real joy of doing theoretical physics. The feeling of this amazing luxury of being able to be there to try to think deeply, solve problems, and being appreciated for doing this. Really genuinely feeling that people appreciate what you're doing. Certainly, as a student or a young post-doc, this is the most important thing you want to get. You want to see that very, very smart people like John and all the other professors are supporting you. They really want you to succeed and do interesting stuff. And you don't judge you, they don't judge whether you get results quickly or not. They leave you free, but they continuously bombard you with interesting ideas. That whole atmosphere is pretty unique. I have never seen this anywhere else.
ZIERLER: What kind of opportunities did you have following Caltech?
VERSTRAETE: In my second year at Caltech, I started applying for jobs at various places, the US, Europe. I actually also applied to a hedge fund because I didn't know if I would get an offer at a university. For whatever reason, I was very lucky. Once you get one offer, people know, and you get offers from everywhere. I had the luxury of choice. But because I had a wife and three children, we decided that we would go back to Europe. Not that we didn't like it in the US. I think the US is an amazing place where you immediately feel welcome. But nevertheless, we, at that time, thought it was a bit too materialistic. It did not completely resonate with the way we were raised in the sense that being rich in the US allows you to do things that you cannot do if you're not rich. The differences between rich and poor people are so huge. Also, there are some strange dynamics in the US that made us feel like, "Let's go back to Europe, where we'll be able to raise our children in a more normal climate."
I remember stories of colleagues who stayed in the US for a few years, then came back to Europe, and these children could not adapt to Europe. They ran back to the US because they couldn't adapt to it. We didn't quite understand this, we were quite puzzled, and that's why we decided to go back to Europe. Of course, also, I got an extremely interesting offer from the University of Vienna. From being a post-doc, I would immediately become a full professor with a very nice salary, a chair, post-docs. This was also very nice. Vienna is an amazing city with a humongous history of doing fundamental research on physics, quantum physics, with people like Anton Zeilinger who are very interesting. There was a very interesting atmosphere there for doing things. I just accepted that offer. But I think if I would not have had a family or children, I would have stayed in the US, for sure.
ZIERLER: When it was time to start thinking about building your own group, essentially from scratch, in what ways did IQI and even John Preskill serve as a model for how to do it in your own department?
VERSTRAETE: Well, the first thing you try is to have these group meetings. [Laugh] It kind of works, but you can never replicate it. Still, there's nothing I value more than discussions among my students, among the post-docs. I especially kind of built an office in the University of Ghent that's one big, open office in such a way that the post-docs and PhD students are all sitting together, and there's a big, big blackboard. Any time people are discussing, I encourage other people, even if they don't understand or they're new, to just sit there and listen so that everybody has the chance to be inundated with the way people discuss, the way people think. This is very much inspired or in the spirit in which physics was done at IQI. Discussions, challenges. "Oh, this is stupid. You don't have to do it like this, you can do it like this," and then people react. A very open atmosphere. Also, there's no hierarchy. John would never pretend to be smarter or know better than the students. He would just ask questions. And he wouldn't mind being wrong. Although he was the starring figure, he was very much part of all the discussion, and you wouldn't mind saying things that were wrong to John. He wouldn't mind, but he would correct you and say, "No, it's probably like this." This is the atmosphere I try very much to replicate in my group. I certainly have been a little bit successful in this.
ZIERLER: Did you see the low-hanging fruit becoming less low, so to speak, when you were setting up your own shop?
VERSTRAETE: Yes, for sure. Yes, and no. I think I was very, very lucky with these tensor networks that it took a very long time before other people really started working in this field. It was 2010 or something like that before many other people were working on this. I had this almost eight-year stretch of being one of the only people in the world working on the theoretical and numerical aspects of tensor networks. For me, this golden age certainly kind of stretched all the way to something like 2010. In that sense, I was very lucky. I have to think about the golden age of quantum computing, quantum information. Certainly, many of the problems that people are working on now are problems people already started working on again in this time until 2010. I think, also, in quantum computing and quantum information, I think it's not just tensor networks, but the golden age, I would say, was between 2000 and 2010.
This is where the people of my generation made their careers and came up with original and beautiful ideas. After that, I believe it became harder for new students to come up with problems that had a big impact. Of course, there were still amazing and beautiful results. But one of the witnesses of this is that papers became much longer. If you look at the average size of a paper before 2010, most of the papers were like Physical Review Letters, like, four pages, in which you have a very good idea, you write it down, and you immediately jump to the next idea. You don't spend too much time writing it down, but four pages is this perfect format in which you can really kind of explain everything on this one great idea.
Also, John has amazing papers that are four pages. Four pages is, in some sense, this perfect format. If you have one very good idea, you can condense it to four pages, and people will really read this. It's very easy to read. But after 2010, papers became longer and longer. They became much more technical. The papers became much more mathematical, much more technical, the proofs became very, very long. Of course, this is also very valuable and very important for a field to mature. It's difficult to say whether the golden age is when lots of new ideas come out or when all these many ideas are completely worked through in a beautiful general framework. You could also call this a golden age. But from my point of view, the most important thing in physics are the original ideas. In that sense, the IQI age was the time.
ZIERLER: Leaving Caltech, what were some of the big questions you wanted to pursue next?
VERSTRAETE: It's not that I had the feeling, "Now, I have a tenure position. I have to start working on something very deep. I want to spend my time now on a very important problem." Because my experience, and this is also what I experienced at Caltech, was that working on relatively minor problems is very important to come up with big ideas. I think it's very important to even work on problems that at first don't look very interesting but might be just very technical. You work on them, and only by working on small problems, you, from time to time, see big ideas popping up. I kept on doing physics like that because it just worked. Of course, I had laid this foundation of these tensor networks, both at Max Planck and Caltech, and to me, it was clear that this was an amazing opportunity. These tensor networks are a new type of field that seems to give a new language for tackling some of the hardest problems in quantum many-body or theoretical physics.
Still, as of today, we are really in this process of trying to understand many ideas, like conformal field theory, but also in topological phases of matter, in the language of these tensor networks. And they really keep on giving nontrivial insights. In that sense, I have been extremely luck and fortunate that I just was lucky to be there in the right place at the right time, having had the right mentors to ask the right questions. Then, in some sense, everything rolls on its own. Of course, you have to keep on working very hard. But the world is very unfair. Once you're kind of on a roll and understand things that very few people understand, you keep understanding more, and more, and more things that nobody understands, and you keep on having a head start on all new projects you start. That's how the academic world works, and it's very unfair, and it's very nice if you're one of the lucky few for which this happens, and it's the opposite if you're not lucky like that. But again, to remain being lucky, the important thing is there to continuously be interested in other results and trying to understand all the problems that people have and trying to reformulate this new language to continuously reach out, and this was the one thing that was extremely crucial and central at IQI.
John invited all kinds of people that, even if they didn't work on quantum information or entanglement theory, they were giving talks about problems that were very relevant for broadening our horizons. This has led to these many insights. Maybe John was not my advisor officially, but he was kind of much more important than my advisor. He's the one who taught me about both the joy of doing physics, but also the person who made me think about what kind of physics I wanted to do, what kinds of problems I wanted to pursue. How do I come up with new and original ideas? This is what I learned at IQI, and this is, of course, the most important thing as a researcher for the rest of your life, to be able to go up with interesting problems.
ZIERLER: From the outside looking in, what did you take away as the significance when IQI became IQIM? What did the M mean for the field?
VERSTRAETE: This was very much in the direction of the problems we were working on. These tensor networks were exactly showing that this whole idea of entanglement theory was relevant for problems in condensed matter, in many-body physics. This was very nice. I didn't pay any attention to this name change, though. It remained IQI for me. But this whole idea of the fact that entanglement theory is relevant for these different things, especially for condensed matter and atomic physics, as I said in the beginning, is probably, as of now, the most important legacy of the whole field of quantum information.
ZIERLER: As the field has developed and similar centers of quantum information have grown throughout the world, where do you see IQI in the development of this global effort to expand the field?
VERSTRAETE: This is still the place all the best people want to go to. If you're a post-doc doing quantum information, Caltech is still the place you want to be. It is really the center, the place with the biggest history, but also where the best post-docs are. It has kind of retained that amazing aura. If you want to become a professor, you must've been a post-doc with John Preskill. This is not a coincidence. It is, indeed, this extremely inspiring place. In the field of quantum information theory, there's no place like it. There's nothing that can even compare to it. There are places like MIT with Peter Shor, Berkeley with Vazirani, but these places are much more specialized. In the history of the development of quantum information, I think they've had a little bit less of an impact. They didn't have this completely open and welcoming attitude to different communities coming together.
This has been always one of the central mantras of John, that he wanted condensed-matter physicists to be part of it, atomic physicists to be part of it. He wanted to join all these communities because he realized that this whole language of–high-energy physics, of course, the It from Qubit kind of people. He realized that quantum information is a perfect new language for doing quantum many-body physics. In that sense, it has always remained a very special place because Caltech is not about one person, it's really about the whole community. The other places, you go there because you want to work with one person or something. Caltech, you go there because that's where things happen.
ZIERLER: What you're saying, then, is that from the beginning, when John is after the most fundamental questions, it requires all of the people, all of the different kinds of specialists, to contribute to those questions.
VERSTRAETE: That's true. Nevertheless, I don't think that John–this is my own feeling, and I could be completely wrong–chose post-docs because they were coming from certain fields. He just chose people because he believed in them and thought they had original takes on problems, that they'd do interesting work. That's the way it went. I might be wrong. But he was amazing in attracting the right people who were all very independent. He put these people together, and the magic came out of it. Again, the discussions. This is what I remember from Caltech. Every day, I was so happy to go to the office and stand in front of the blackboard, discussing with people, saying they were wrong, hearing that I was wrong, striving to solve anything. We were very lucky to be there in the golden age. But that's pure luck.
How to Measure Progress in Quantum Information
ZIERLER: Two last questions, looking to the future. One, how do we understand developments in the field? Looking back when you initially got to Caltech, to now in 2022, how do we measure progress? What does it look like to you?
VERSTRAETE: That's a very interesting question. It's difficult to measure. You could try to measure progress in the sense that there are so many more papers and people working on it, so many more citations. But this is certainly not what people from the field care about. We care about new fundamental ideas. One thing I would say is very frustrating is the complete lack of quantum algorithms for solving interesting real-world problems that have nothing to do with quantum mechanics, like factoring. This is certainly something that is worrying as a community. We don't really talk about this too much. I don't think we've fulfilled that promise. When I started in this field of quantum computing, there was still this amazing idea that with quantum computers, you would be able to solve lots of problems exponentially faster than with classical computers. That premise has never been realized. It could still be realized, but that's certainly, I think, one of the things that put our feet back on the ground, that we have to be a little bit careful in thinking that quantum computing is the biggest thing ever.
It's extremely interesting and allows for new ways to do physics, but that doesn't mean that it revolutionizes everything. For me, this has certainly been a reason that my work has more and more involved the direction of understanding quantum many-body physics as opposed to working on quantum information or quantum computing problems, which are more and more becoming engineering problems. What are the big issues? An extremely interesting technological problem is, how do you make a quantum computer? How do you make something fault-tolerant? I think the focal point in the world of research on that topic is Caltech, especially now with this whole Amazon cluster that's built there that's completely focused on this. If a quantum computer has to be built, it has to be fault-tolerant. This is now a technological problem, an engineering problem, and that's why you need new means or methods for doing that. That's exactly what Amazon can provide.
I don't know what budgets they have, but it's something that's not as much in the spirit of what I remember about IQI. IQI was about fundamental, original new ideas. What the field needs now is not fundamental new ideas but very good engineers who get things done and scaled up. And that's what is being done now. In some sense, the golden age for experimentalists is–I'm not sure if it's here already, or if it'll be there in a few years because I'm not really familiar with the current state-of-the-art experimentalists. But that's the way quantum information is evolving. That's one aspect of it. The other aspect is, papers are getting longer and longer. Mathematics ideas that are needed to make progress are getting deeper and deeper. In that sense, this is also a very interesting development. Also at Caltech, there is this prime example of Thomas Vidick, who obtained a revolutionary insight into some of the biggest open problems in mathematics using quantum information tools. This is another direction in which the field has evolved. It's really evolving. This language has matured in such a way that it allows now to solve deep problems in mathematics. This is, from my point of view, equally valuable to the problem of building a quantum computer.
This is also a very valuable thing. The third pillar of research that I find extremely interesting is trying to understand entanglement in many-body physics. This is not quantum information per se, but all top universities in the world, whether it be MIT, Harvard, whatever, people are reading the lecture notes of John Preskill. Whether they do high-energy physics, quantum chemistry, whatever problem that has to do with [the] many-body problem, people have to know about entanglement theory. People have to understand this. Because without this, you cannot follow any of the current literature or state-of-the-art research. In that sense, research has completely changed.
You can't follow the language in which research is done anymore if you don't have a background in quantum information. I think the credit for that really almost uniquely has to be given to John. He's the one who pushed his students and post-docs to work on these problems, to explore connections between physics and entanglement theory, physics and quantum information theory, and he did this starting in '99, 2000, and all the way somehow until now. This is certainly a big part of his legacy, that he gave us problems to work on for the rest of our lives, basically.
ZIERLER: Finally, last question, this has been a theme that's permeated our discussion. In thinking about the upcoming 25th anniversary of IQI/IQIM and John's directorship of it, how do you understand his legacy in historical terms, both for you personally, for the field in his capacity as a mentor, and perhaps most importantly in his capacity as a visionary?
VERSTRAETE: I think I'll have to repeat myself here in saying that John is the one who understood the relevance of quantum information long before anybody else in the sense that he understood that this gives you a new language for doing physics, for solving the big open problems in physics, and he really pushed all his students and post-docs to explore this. This is very subjective, but from my point of view, this alone makes him great. But he was much more than that. He was always extremely passionate in sharing ideas. He was the one who let us think further, who made sure we were not just happy with the latest result. Once we had the result, we should explore and go deeper. He's the one who created this whole fantastic atmosphere in which we kind of competed, but on the other hand, we all worked together and were pushing each other such that we would be able to shine during his group meetings. [Laugh]
It's difficult to say, but John had an amazing influence on all of us. I've said this many times, but he made sure we had joy in doing physics, and I have tried to give this to my students by mimicking how John does that. It's really kind of seeing what kind of questions to ask people or engage people in such that they will discover new things themselves. I'm sure many of the things John was asking, he already knew the answers to, but he wanted us to discover them and come up with something new in the meantime. He was always extremely happy to share all his ideas. There's nothing more you can want from an advisor than that.
ZIERLER: Frank, on that note, this has been a tremendous pleasure spending this time with you. What a wonderful addition as we work towards 2025 and the anniversary. I'd like to thank you so much.
[END]
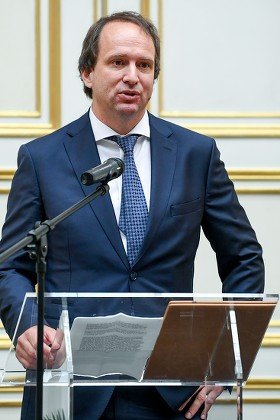