Jeff Gelles (PhD '86), Biochemist and Research Leader in the Mechanisms of Molecular Machines
Although the metaphor is not perfect, the name stuck: the proteins, RNA and DNA, in living cells are molecular machines that perform the essential processes of life. The research lab led by Jeff Gelles at Brandeis explores these processes and their role in gene expression and regulation, and in the function of eukaryotic cells. Central to these achievements is Gelles's pioneering utilization of single-molecule optical methods, and a hallmark of his career is the interplay of new technology development, and the new science that such developments enable.
In the discussion below, Gelles recounts his long-term fascination with biochemistry, his undergraduate education at Harvard, and his graduate experience at Caltech, where the deep interdisciplinary research culture between biology and chemistry focused his interests in the fundamental chemical processes that govern cellular function. As a postdoctoral scholar, Gelles explored kinesin, a motor protein, and this remained a key interest as he built his research lab at Brandeis. Taking a deeply collaborative approach that connects his lab to engineers and condensed matter physics, Gelles's research is a reminder that the best in academic research should approach scientific disciplines as human constructs meant to be crossed with ease in the pursuit of discovery. This is an essential Caltech value that has stayed with Gelles ever since.
The end of the discussion ends on something of a philosophical note. As the technology improves and the research becomes more refined, is there an ultimate simplicity, or singularity, waiting to be uncovered at the very heart of life? Or is the complexity endless, and research and technological advances only sharpen our understanding of that complexity? While Gelles does not hazard an answer that by definition would be only conjectural, a key lesson from his career is that the science should keep going, because the discovery becomes only more exhilarating.
Interview Transcript
DAVID ZIERLER: This is David Zierler, Director of the Caltech Heritage Project. It's Thursday, June 13th, 2024. It's my great pleasure to be here with Professor Jeffrey Gelles. Jeff, wonderful to be with you. Thank you for joining me today.
JEFF GELLES: It's a pleasure. It's actually Jeff, not Jeffrey.
ZIERLER: Oh, your official name is Jeff?
GELLES: Yeah.
ZIERLER: Let's start right there. What's the story?
GELLES: We'd have to ask my parents that. [laugh]
ZIERLER: Let me see here. No. I looked on your thesis; it's Jeff there as well. My mistake. Jeff, to start, please tell me your title and institutional affiliation.
GELLES: I'm the Aron and Imre Tauber Professor of Biochemistry and Molecular Pharmacology at Brandeis University in Waltham, Massachusetts.
ZIERLER: Let's start first with the name, Aron and Imre Tauber. Who are or were they, and what's the connection to you?
GELLES: There's no direct connection to me. They were relatives in the Tauber family, the family that endowed the chair that I currently hold. I'm not the first person to hold the chair. It was originally held by my colleague Bob Abeles, who passed away a number of years ago.
ZIERLER: Now, the title, biochemistry and molecular pharmacology, there's always a story behind the titles. How are those terms encapsulating your research, the things that are most important to you?
GELLES: I think they probably more encapsulate Bob Abeles' research. I consider myself a biochemistry and a biophysicist but not really a pharmacologist.
ZIERLER: How the not really pharmacologist?
GELLES: In the sense that what I do is really basic research. I don't really develop pharmaceuticals or test their effectiveness.
ZIERLER: That's interesting. The term "pharmacology" inherently has an applied nature to it.
GELLES: Yes.
ZIERLER: Are the things that you're doing in biochemistry and biophysics ultimately relevant to what we would now call translational research, if not in your lab, taken on by others?
GELLES: I think that all basic life sciences research is eventually relevant to translational research. It's just a question of how long it takes. Sometimes takes only a few years for the relevance to become starkly apparent, and sometimes it takes decades.
The Limits of Metaphors in Biology
ZIERLER: Jeff, let's now get to the fundamentals. Going into a cell, all of the processes that are happening there, what are you focused on? What are the big questions for you?
GELLES: There's a poor metaphor that what goes on in cells is like the actions of human-engineered systems. Biochemists speak of cells as having molecular machines that do the work of the cell. Those molecular machines are what my lab works on. But the metaphor to human-designed machines is a really imperfect one because biological systems come about by a fundamentally different process than the process of human engineers designing and building things.
ZIERLER: That process would be evolution?
GELLES: Yes, evolution and natural selection. That really makes a difference, and it actually makes the biological "machines" much more challenging to understand because they're not engineered with a purpose in mind.
ZIERLER: At least insofar as we can tell?
GELLES: Yes.
ZIERLER: Tell me about gene expression. I know that's a fundamental part of your work. What are you looking at in the world of gene expression?
GELLES: For an organism to function properly, not all of its genes can be active at once. Single-cell microorganisms, as they move from different environments where different nutrients are available, they switch on and off genes as appropriate to metabolize particular nutrients. In multicellular organisms like us, regulation of genes is even more complex. Different cells have different specialized functions, and they switch on genes that, for example, are appropriate for a muscle cell, and off genes that are only appropriate for, say, a white blood cell. Gene expression is the process of switching genes on and off, and controlling which ones are switched on and off. Control of gene expression can occur through a variety of processes, but one of the main ones is switching on and off which genes are transcribed into RNA. That's one of the main things that my lab works on.
ZIERLER: Is this the work of messenger RNA?
GELLES: A lot of it is messenger RNA, but there are also non-messenger RNAs that also get switched on and off, and that do other things in the cell.
ZIERLER: What are those other RNAs?
GELLES: Probably the most common class are regulatory RNAs that control the expression of other genes or control other processes. But some of them are also components of basic cellular machinery. For example, in rapidly growing bacteria roughly half of the RNA made is just the RNA molecules that are used in the ribosome to translate other RNAs.
ZIERLER: Jeff, tell me about actin. Where does actin fit in here?
GELLES: My lab has a bit of a split personality. In addition to working on transcription and gene expression, we've also for a long time worked on the cytoskeleton of eukaryotic cells. Actin is one of the important components of the cytoskeleton.
ZIERLER: Let's start with the basics. What is a cytoskeleton?
GELLES: If you look in a microscope at a eukaryotic cell, let's say a mammalian cell, and you stain it appropriately to look at where different proteins are, you will see that if you stain for actin that actin is one of the most abundant proteins in the cell. It is the essential protein for a variety of cellular structures that perform biologically important processes. The other protein in the same category that's really important is the microtubules, which are made out of tubulin. My lab has worked on both of those systems for years. Both of those proteins sort of form filaments in the cells that, as I say, perform different biological functions.
The Connecting Point of Cytoskeletons
ZIERLER: Now, is the cytoskeleton research a subset of what you're looking at with gene expression, or are these different processes?
GELLES: They're different processes. I won't say they're entirely unrelated, but they're pretty unrelated. The reason why we work on both of them is that there's a common theme, which is a very important theme in our research, which is that biochemists are reductionists, and there's a traditional biochemist way of studying biomolecular systems. You grind up cells, you purify the particular protein you're interested in, and you study its properties. That's a good way to get started. It can be somewhat limited though because proteins interact with other proteins and, especially, proteins are regulated by other proteins.
You might say, oh, I'm going to take protein A, and I'm going to mix it in a test tube with protein B and study the two together. But there are actually many systems in biology, they're kind of legion, where even that is way too oversimplified, because there's not just two proteins involved, but there are tens or hundreds of proteins involved in the system. You might be tempted to purify all of those proteins, and mix them together, but the problem is that when you use traditional biochemical methods, it can be very hard to discern what's going on in that mixture because it's so complex. A central theme of my work has been developing single-molecule methods for studying biochemical processes. The single-molecule methods are really helpful in getting around the complexity, and still being able to discern how things work.
ZIERLER: Now, the dynamism of all of these individual molecules, the idea that there's so many things that are happening, different processes happening at the same time, you've referred to this as the crowd noise problem. I wonder if you can explain what that metaphor means here, crowd noise.
GELLES: The crowd noise metaphor relates to exactly the problem that I was just discussing. I have some slides that I like to show in some of my talks. I have a picture of a crowd at one of the parades that was put on to celebrate the Red Sox winning the World Series. The picture just has this vast sea of ecstatic people who are shouting. I ask the audience to imagine what it would sound like if they were at this parade. Of course, it would be chaos. Different people would be saying different things at the same time, and you wouldn't be able to understand what anyone is saying. In my story, this is what it's like to look at populations of molecules, because you often have different molecules that are spontaneously forming different complexes with other molecules -- all of that's going on in your test tube at once.
If you try to study those complexes using any kind of technique which is only going to tell you about the population average behavior of the molecules, then you just get the "crowd noise", and it's very hard to distinguish what's going on. Whereas if you can do experiments where you focus in on the individual molecules or molecular complexes, and look at them one at a time, it can be very revealing. Of course, every different complex you look at will in general be doing something slightly different, but if you study many complexes one at a time, you can build up a picture of the way that the system works that you can't get from looking at a population average.
ZIERLER: Jeff, let's move now to instrumentation. I imagine that you need to be working with instruments that can capture these processes, not as frozen or dead scenes but in their natural living real-time environments. What are those instruments? How do you study this?
GELLES: All of the single-molecule work that I've done uses light microscopy, which is a centuries-old technique. But what we and other people in the field been able to do is to develop light microscope optical systems and ancillary equipment that enable us to detect signals from single molecules. I and my colleagues Larry Friedman and Johnson Chung have focused on developing light microscope optical systems for doing single molecule fluorescence microscopy that can efficiently allow us to look at behavior of multiple different colors of fluorescent dyes at the same time at high time resolution and high signal-to-noise. In our typical experiments, we proteins that we're interested in looking at in the experiment, two or three or four species of protein at a time. To each of these molecular species we attach a different color of fluorescent dye. Then we can monitor the fluorescent dyes as individual molecules come together, form complexes and perform their cellular functions.
ZIERLER: How much data is this? Is this a big data operation in your lab?
GELLES: It is not big data on the scale of experimental high energy physics or even some large-scale bioinformatics work. We might be collecting thousands of images in an experiment but not hundreds of millions of images or anything like that.
ZIERLER: Now, in terms of analysis, have you yet embraced AI? Has that become useful to you to pull out what's interesting and relevant from all the noise?
GELLES: I would hesitate to describe it as AI, but we do a lot of machine learning.
ZIERLER: What's the distinction, in your mind?
GELLES: We're not doing things like neural networks that are analyzing the data using some kind of abstract model that people don't understand the meaning of the model. Instead, what we're doing is we're using physics-based models, where all of the parameters have some physical meaning, and we understand what aspect of the data the parameter measures. That's really important because we use Bayesian machine learning techniques to analyze the model. An essential feature of the Bayesian approach is that you have to include the prior information you have about the values of the different parameters, and you can only do that if the parameters are physically meaningful.
ZIERLER: Jeff, tell me about this research in the larger framework of interdisciplinarity. Obviously there's chemistry here. There's physics at play. Where is your lab a centering point for these different disciplines, and where are you going out and collaborating with other labs?
GELLES: Pretty much every single thing that my lab does is a collaboration. We're collaborating with people who have different things to bring to the projects, and many of them are people from different disciplines. Some of them are even people from the same discipline but who have different expertise or different knowledge of the field. Collaboration, for me, it is a really important part of science, and it's something that has really been incredibly rewarding for me in my scientific work.
The Conceptual Importance of Condensed Matter Physics
ZIERLER: I can see easily enough the connections to molecular biology and physical chemistry. What about condensed matter physics? Where do the condensed matter physicists play a role here?
GELLES: Condensed matter physics brings a lot of relevant information into this kind of research. For example, we're now working on a project in which it's very important to understand the mechanical properties of a particular part of a protein that consists of a long extended chain hanging off of the protein, what the dynamics of that extended chain are, and how it affects the interaction of this protein with other proteins. Those are all questions where condensed matter physics brings a lot of theory and computational tools to the table that can help us understand how the biochemical system works.
ZIERLER: Now, are you talking about conceptually, or is there also instrument building that condensed matter physics is contributing here also?
GELLES: I'm talking mainly conceptually. There are some tools that biophysicists have borrowed from condensed matter physics. There are even some tools that have been borrowed in the other direction as well.
ZIERLER: Jeff, some institutional questions. Biochemistry at Brandeis, how far back does that go?
GELLES: Brandeis was founded in 1948, so it's very young compared to most U.S. universities. If I remember right, it was either in the late '50s or early '60s that the biochemistry department was founded.
ZIERLER: Do you see your work as part of a particular intellectual tradition at Brandeis? Did you come to Brandeis really innovating things that were not done before?
GELLES: I think it's partly true that it's part of a particular intellectual tradition. The university, when the biochemistry was founded, there were several people who were hired, who eventually ended up being absolutely the world leaders in studying the mechanisms of enzymes, particularly Bob Abeles and Bill Jenks, both of whom were still here in the department when I started at Brandeis. They built a department that was very strong in that area. The work that I do, in one of its senses, is related to that, but it's also related to biophysics and cell biology, which have also been strengths at Brandeis.
ZIERLER: Jeff, your emphasis on collaborations, being in the Greater Boston area, Harvard, MIT, all of the biotech that's around, is it geographically an asset for you where you are?
GELLES: Absolutely, no question about it.
ZIERLER: What are some of the key collaborations that you have regionally beyond Brandeis?
GELLES: My biggest collaborations right now outside of Brandeis are that my lab and Steve Buratowski's lab at Harvard Medical School have a long-standing joint project on using the single molecule methods to study the mechanisms of transcription, and transcription regulation in eukaryotic systems using budding yeast as the model system. This project has a particularly unusual feature, which is that the project is premised on the idea that eukaryotic transcription is so complex that if we try to do one of these reconstitution experiments that I discussed earlier, where you're purifying the proteins and mixing them together, you don't know whether you're getting something that mimics what's in the cell because we don't know enough about which proteins to put in. So in that project, instead of purifying proteins, we and are collaborators are just using unfractionated nuclear extracts from yeast cells. We're making a cell-free solution solution that contains all of the proteins present in the yeast nucleus, and we're using that as the basis for studying transcription. We've been able to use that system to define mechanisms of transcription regulation that were not apparent and could not easily be studied using the reconstitution approach.
My other main Boston collaboration is with Steve Bell's lab at MIT. With Steve and his students were studying mechanisms that a eukaryotic cell (again, budding yeast) uses to efficiently and accurately set up to duplicate its genome prior to cell division. This is another project that making use of our single-molecule approaches, but this time in a biochemical system that is fully reconstituted from purified proteins.
ZIERLER: Jeff, your lab as a fundamental operation, tell me about the graduate students, and what they go on to do. What's the proportion that go on to academia and industry?
GELLES: I would say more in industry than in academia, but I've had quite a few students and postdocs go on to academia as well. Another advantage of the Boston area is that we have lots of contacts with people in biotech and pharma in the Boston area, including graduates of our PhD program and former postdocs. Greater Boston is a good place for students to be when they're looking for jobs. As far as academic careers go, I have former students who are PIs at major research universities. I also have a fair number of former students and postdocs who teach at primarily undergrad institutions, where they're still doing research and can train the undergrads in doing research. Several of them have built very successful careers at those institutions. I also have some former Ph.D. students who are high school science teachers too.
ZIERLER: Now, for the students who have gone on to industry, is the main advantage the expertise gained in your lab? Is it more about the skills and the concepts, or is there a specific connecting point between the research questions that you're after, and the drug discovery and therapies that these pharmaceutical companies are trying to achieve?
GELLES: I would say it's more of the former, it's more of the approach, and the students tend to have very good quantitative and computational skills in their toolbox that can be applied to a wide variety of problems in biochemistry. They have some methodological things that they've learned. There are some of them who are actually directly using their biological training from the lab. The lab also does a lot of work in addition to the work on eukaryotic transcription that I talked about. The lab does a lot of work on bacterial transcription. Understanding the way bacteria work is extraordinarily important for medicine, and it's extraordinarily important for a whole bunch of other fields. For instance, one of my former students is working for a company that's trying to develop ways to engineer cereal crops to reduce their dependence on nitrogen-containing fertilizers, in order to lessen the environmental impact of that kind of farming. That's very tied in with understanding exactly how bacteria regulate transcription.
ZIERLER: Now, for you personally, have you ever gotten involved in consulting or in the start-up world?
GELLES: Not at all, no.
ZIERLER: Pure academia?
GELLES: Yeah. I became an academic because I wanted to learn about new things and to have somebody pay me to do that.
ZIERLER: [laugh]
GELLES: I'm very happy being an academic.
ZIERLER: Jeff, let's go back now to pre-Caltech. Where did you do your undergrad?
GELLES: I was an undergrad at Harvard here in the Boston area, and I was a biochemistry major.
Longstanding Fascination with Biochemistry
ZIERLER: What attracted you to biochemistry at such a young age?
GELLES: I was attracted to biochemistry way before that. I don't know how I originally got interested in it, but I think it might have been partly from reading some books by Isaac Asimov, who was a biochemist. I grew up in Columbus, Ohio and when I was in high school, I actually had a job in Prof. Michael Klapper's biochemistry lab at Ohio State University.
ZIERLER: Who were some of the key professors for you at Harvard?
GELLES: I think the most important influence was this guy named Guido Guidotti, who passed away a couple of years ago. Guido was the professor in whose lab I did my undergraduate thesis, and he was a great influence on me. He was incredibly enthusiastic, and really a deep thinker about the way things work in biology. But something that was equally important was that—I'm trying to think of a diplomatic way to put this -- some of the other professors at Harvard were arrogant and unpleasant in other ways. One of the things that I really liked about Guido is that he showed me that you could become a very successful scientist without turning into a jerk.
ZIERLER: [laugh]
GELLES: I doubt that I would've gone into science without him showing me that.
ZIERLER: Oh wow.
GELLES: That was very important.
ZIERLER: Also clearly taking an interest in undergraduates as well; that must have felt unique also. Tell me about working in his lab. What were the big research questions?
GELLES: I was in Guido's lab, and I was working for Lew Cantley, who was a postdoc there at that time, and has since gone on to be a very distinguished biochemist. Both Guido and Lew were really interested in membrane proteins. These are proteins that are embedded in cell membranes, and are involved in transporting materials and information across the membranes. They're very important proteins -- probably the major class of proteins that are targets for pharmaceuticals that we use medically. Guido and Lew had a mechanistic focus. They wanted to understand how the protein works as a machine to do what it does.
ZIERLER: Did you stay on campus during the summers? Did you do research in the labs?
GELLES: Yes, absolutely.
ZIERLER: You loved it? This was where you wanted to be?
GELLES: Yeah. It was a lot of fun doing research, and it was a lot of fun living in Cambridge.
ZIERLER: Did you have a senior thesis?
GELLES: Yes.
ZIERLER: What was it on?
GELLES: It was on this protein called the sodium-potassium ATPase. In order for all cells and particularly for neurons to work in animals that have neurons, cells have to maintain a difference in ionic composition inside and outside of the cell. Outside of cells, there's a high concentration of sodium ions. For instance, there's 100-plus millimolar sodium ions in your blood serum and surrounding all the cells in your body, and much lower concentrations of potassium ions. The reverse is true inside cells. Inside the cells, there's high potassium and low sodium. In neurons, the reason why that's important is that neurons send signals down the neuron by opening sodium and potassium channels in the cell membrane, and letting the two ions flow down their concentration gradients. The sodium-potassium ATPase is the enzyme that creates the concentration gradient, and it does that by pumping sodium out of the cell, and potassium into the cell, using the free energy that's generated by hydrolyzing ATP.
ZIERLER: Did you ever think about pursuing an MD, or it was graduate school all the way for you?
GELLES: My parents thought about me pursuing an MD, but my thought about pursuing an MD was that I didn't want to do it.
ZIERLER: Had you heard about Caltech before you started thinking to apply there?
GELLES: I think so. My father was a scientist, and he has his bachelor's, master's, and PhD from MIT. I think I didn't know very much about Caltech, and I just naïvely thought of it as the California version of MIT.
ZIERLER: Sure. [laugh] Had you heard about Harry Gray?
GELLES: No, not before I joined the Caltech chemistry department.
ZIERLER: How did you first hear about Caltech, or how did you think to apply there?
GELLES: I think it's just what I said. I thought of it as the California version of MIT. When I was applying for grad school, and trying to decide where to apply, of course we did not have any internet resources. But the American Chemical Society used to publish a book called The Directory of Graduate Research. It was about the thickness of a telephone book, and it listed the professors at all research universities in the US, in chemistry and biochemistry, what they did their research on, and what papers they had published recently. It had all the information in it that applicants to grad schools nowadays get by going to the websites of the different departments they were interested in. I looked at that book, saw what kinds of things people were doing, and what I thought was interesting. There were definitely people at Caltech whose work sounded really interesting to me, including Sunney Chan, who was eventually my final advisor, Jack Roberts, Michael Raftery. I didn't know as much about Harry's work, just because I didn't know very much about metalloenzymes when I was looking.
ZIERLER: What year did you arrive in Pasadena?
GELLES: That would have been 1978.
ZIERLER: You were specifically looking in chemistry but where there were biological questions being pursued, as opposed to biology professors who were utilizing chemistry kinds of approaches?
GELLES: I was mainly looking at biochemistry. But there are schools, including Caltech, that do not have a biochemistry department, and so then you have to look. The ones that don't have a biochemistry department, typically, they have a lot of biochemistry going on in the chem department, and they also might have people in biology doing chemistry as well.
ZIERLER: What about Peter Dervan in the very early years of chemical biology? Did that register with you at all?
GELLES: It did when I was a student there. If I remember right, I think that Peter arrived at Caltech either the same year that I did as a grad student or maybe a year before.
ZIERLER: Now, when you got here, what were your impressions? Did it feel like the California version of MIT, or was it something very different?
GELLES: I was a naïve graduate student, so I didn't actually know anything about MIT, other than the fact that my [laugh] dad went there. I had applied to MIT too because there was some really interesting stuff going on there as well. I had never been to California before, and I was really looking forward to living there. I did have a lot of fun when I was living there. The environment in Pasadena, especially, it was a little bit surreal to me. Those were definitely the bad old days of Southern California air pollution, for sure.
ZIERLER: That's right.
GELLES: The air pollution was very apparent in Pasadena because on a third of the days, you couldn't even see the mountains just a couple of miles away. The other thing that was very surreal about it was that I ended up spending practically all of graduate school working in a windowless basement in Noyes lab. Then me and some of my friends would get tired of that, and we would have to leave the lab, and take our folding aluminum lawn chairs outside, and sit on the lawn [laugh] on the Caltech campus. Of course, maybe not quite as much anymore, the Caltech campus is very weird architecturally. I can't remember which TV show it was, but there was some TV show of the '70s or '80s that used the Caltech campus as a set because of all of the futuristic-looking buildings there.
ZIERLER: [laugh]
GELLES: It was a very unusual place for aesthetic as well as scientific research.
ZIERLER: Jeff, what were some of the classes or faculty interactions that focused your interest, what ultimately would lead to your dissertation?
GELLES: I think that my dissertation, you know, what I was interested in for my dissertation, a lot of that was sort of a logical progression from what I had worked on as an undergrad. There were some classes that I took at Caltech that really influenced what I ended up doing later. One in particular had an enormous influence on me, which was a class that Howard Berg taught. That course really opened my eyes and showed me that statistical physics had an enormous amount to offer biochemists in understanding how certain molecular systems work. It was a wonderful course. Howard was an amazing teacher. The two TAs in the course went on to be very famous academic scientists in their own right. At that time, Howard had written this book called Random Walks in Biology, which was sort of my bible. My copy is still right here next to my desk. He actually used the galley proofs of the book as the textbook for the course -- he had just finished writing it.
Meeting Harry Gray
ZIERLER: When was your first interaction with Harry?
GELLES: My first interaction with Harry, this was in the old days, and it was the chemistry PhD program. The program was run in the semi-punitive way that all chemistry PhD programs used to be run, which is that you had to show up as a graduate student, and in the first week, you had to take a qualifying exam in organic chemistry and inorganic chemistry and physical chemistry and analytical chemistry. If you didn't pass any one of those exams, you had to take the corresponding course. I was a biochemistry major as an undergrad; I had never taken an inorganic chemistry course, so I flunked out of that one. I got to take the inorganic chemistry course that Harry taught, which was a wonderful course. I don't think I necessarily use very much of what I learned in that course. But the thing that I really liked about it was that there's this semi-obscure branch of mathematics, group theory. It turns out that group theory, in this way that's sort of very roundabout, is really important to understanding complexes of inorganic ions. Harry taught all this. I'd always been really interested in math, and how it impinges on science. This just further whet my interest in that connection, as did the course that Howard Berg taught, which is very much about how statistics and probability theory are important, not just for understanding how to analyze your data but are sort of fundamental things in science that can be used to model lots of biological processes that we're interested in.
ZIERLER: What was Sunney Chan focused on during these years?
GELLES: A lot of the things that Sunney worked on seemed to come out of his interest in magnetic resonance spectroscopy, both NMR and EPR, and also some other forms of spectroscopy. It's like my lab, where there's a little bit of a methodological focus that steers us in particular directions, directing us toward looking at particular biological systems. In Sunney's lab, because of his interest in EPR and resonance Raman spectroscopy, he did a lot of work studying metalloenzymes, because those are molecules that those forms of spectroscopy are useful in studying. Because he did a lot of NMR, he was also really interested in studying the dynamics of molecules, particularly molecules in membranes, including the fluidity of lipid bilayers, and how that influences membrane proteins.
ZIERLER: Jeff, as prelude to developing your own dissertation topic, what were some of the big questions in electron transport during this period?
GELLES: As I said, the subject of my dissertation was really influenced by my undergraduate work. I already described how, as an undergrad, I had studied this ion pump that pumps ions across the membrane that's fueled by ATP hydrolysis. When I came to Caltech, and learned what Sunney was working on, I said, "Oh, this sounds really interesting." Sunney was studying an enzyme called cytochrome c oxidase. It's also a membrane protein. It's also an ion pump that pumps ions across the membrane. In this case, they're hydrogen ions. Unlike the ATPase I had studied before, cytochrome oxidase is powered by electron transfer through a series of metal ion centers that are located in the enzyme. I thought that this was an extraordinarily interesting problem, how you can have electrons moving through the enzyme, moving from a good reductant to a strong oxidant—which in this case is molecular oxygen, O2—and how can those chemical reactions be harnessed to power the movement of ions across a cell membrane many nanometers thick?
ZIERLER: Now, the windowless basement lab in Noyes, was this Sunney's lab?
GELLES: Yes.
ZIERLER: How big was his operation? How many fellow graduate students were there?
GELLES: I think there were probably about a dozen, and some postdocs. But I think Sunney really preferred to have graduate students, and that's carried over. I've had postdocs in my lab, but the vast majority of my trainees have been graduate students.
The Limits of Studying Protein Membrane Structure
ZIERLER: Jeff, let's now get to your thesis in particular. Cytochrome c oxidase, what is that?
GELLES: That's this enzyme that I was talking about. The main way that we get energy from eating food is that food has hydrocarbons. It has it has organic compounds in it. In general, these organic compounds are reducing with respect to oxygen. One of the main sources of energy that any aerobic organism uses to live is that we digest our food, and do a bunch of chemical reactions on it. Ultimately, what we're doing is we're taking electrons from the food, and using them to reduce O2 to water. Because of the difference in reduction potentials, that is very downhill energetically, and liberates a lot of free energy that can be used to power biological processes. It was a controversial area in biochemistry throughout the 1960s and early 1970s, about how that energy is stored up and used. But by the time I got to grad school, it was quite well accepted—except by a few outliers—that the central currency of that process is that you're pumping protons across the membrane of a bacterial cell or across the membrane of a mitochondrion in a eukaryotic cell. So you're creating an electrical potential across the membrane, and you're also creating a chemical potential, where the pH outside is much lower than the pH inside, so that the mitochondrial inner membrane or the bacterial cell membrane is kind of charged up like a battery. Then there are other processes that make use of the energy stored in the battery.
ZIERLER: Now, you mentioned earlier the relatively ancient technique of light microscopy. Is that relevant here? Is there spectroscopy happening?
GELLES: There was spectroscopy but not light microscopy. All the spectroscopy that we did in Sunney's lab, that was all on bulk chemical samples.
ZIERLER: What was the data telling you? What were the big conclusions?
GELLES: One of the things that made the bad old days bad was that, at that point, people had been elucidating the three-dimensional molecular structures of proteins since the late 1950s. By the time I was in graduate school, it was extremely well established that having this kind of structural data was very important in understanding how enzymes and other proteins work. As I said, these proteins that span biological membranes are very important in biology and in medicine. Unfortunately, at that point in the sort of mid-80s, it was still very, very difficult to get the structures of membrane proteins.
ZIERLER: Is this a technological problem or a conceptual problem?
GELLES: No, it's a technological problem. Basically, almost all of the structural work on proteins at that point was done by X-ray crystallography. The problem that people were having is that they couldn't figure out good ways to crystallize membrane proteins because, unlike regular proteins that just float around in essentially water, membrane proteins have two different kinds of surfaces on them, some of which are sticking out of the membrane and exposed to the highly polar aqueous environment, and some of which are exposed on the inside of the membrane to the highly nonpolar lipid tails. As a field, we've since worked out ways to do that. But, at that point, there were probably thousands of soluble proteins that we knew the structure of, and only tens of membrane proteins.
The one that I was studying was not one of those proteins, so to figure out what was going on, we had to study them in ways that were much more primitive. In particular, I was doing these spectroscopic experiments that only told us about what was going on at the metal centers inside of cytochrome oxidase and did so only somewhat crudely. The take-home lesson is that I did all this work as a grad student on this protein, and then years later, when there was finally a x-ray crystallography structure of it, it turned out that the work that I had done was basically wrong because at one key metal site inside of cytochrome oxidase, we hadn't realized that instead of there only being one copper ion there, there were two, and that changed the interpretation of the spectra.
ZIERLER: Now, missing the other one, is that because you couldn't see it, or you didn't think to look?
GELLES: It was because there were not accurate enough measurements of how much copper was present in the inside.
ZIERLER: Jeff, as a matter of intellectual foreshadowing, your subsequent interests in cytoskeletons, messenger RNA, can you reverse engineer that back to what you were doing at Caltech?
GELLES: Yes. I got interested in the cytoskeleton at first, and that came about—there were two main reasons. The real problem with interpreting the spectra is that they're population averages, and so you have different molecules in the sample. In the case of EPR spectroscopy, it's really bad because different molecules in the sample are in different random orientations with respect to an externally applied magnetic field, and that changes the spectra. You don't see the spectra of the individual molecules; you see spectra averaged over all the possible orientations, which is harder to interpret. The interpretation of the spectra were very complicated. I thought we did a reasonably good job.
I spent part of my time as a grad student building a computer interface for the EPR spectrometer at Caltech so that we could get more accurately digitized spectra so that we could interpret them mathematically in more detail. But dealing with this population averaging problem was very frustrating, and that led immediately to my being interested in somehow doing single molecule experiments when I left. Then the other thing was taking this course that that Howard Berg taught. One of the things that Howard had worked on doing what was in essence one of the only two kinds of experiments in biology where people had previously been able to study single molecules in action. There was work from the '70s, where people had managed to study single ion channels. That was possible due to the fact that the ion channels have the useful property that when they open, they can switch on a current where millions of ions per second flow through the cell membrane. That actually turns out to be a macroscopically detectable current. If you can somehow make a preparation that only has one ion channel in it, which people worked out how to do, you can actually study the properties of an individual molecule. Then Howard had done the other thing, which is that he had been studying a flagellar motor in bacteria. This is another membrane protein, and this is one of the things that's powered by the battery that's built up by pumping the protons across the membranes.
The flagellar motor lets the protons go back through, in a downhill sense, energetically, and it uses the energy to power the bacteria spinning around a little propeller that's attached to the motor that enables them to swim. This bacterial motility is very important for the bacteria because they actually have a crude sensory system where they can sense if they're moving in a direction in which the concentration of food is getting higher, and they can swim in that direction. I said to myself, "I want to do some kind of single molecule thing." The work that Howard had done showed me that molecular motors like the flagellar motor that translate some kind of chemical thing into some kind of mechanical movement, that was a subject in which it might be possible to study single molecules. That did, in fact, turn out to be the case. When I went off to do my postdoc, I went to work with Mike Sheetz, who had just in the year or two before discovered a new molecular motor called kinesin, which is an ATP-powered motor that moves along the microtubule cytoskeleton. That was how I got interested in cytoskeletal stuff.
Caltech and the Many Bridges Between Biology and Chemistry
ZIERLER: Jeff, another foreshadowing question. We talked earlier about the interdisciplinary nature of this work; biologists and chemists and physicists working together. Did you witness that at Caltech? Was that sort of interdisciplinary collaboration already happening?
GELLES: Absolutely, it was. That aspect of being at Caltech was the thing that really, I think, made me into the kind of scientist that I am. One of the things that was so wonderful about Caltech, especially in those days, is that Caltech has always been a relatively small place, and it was really small back then. As a graduate student there, I was a grad student in a big chemistry department that had something like 30 grad students in each class. But I got to know graduate students in lots and lots of other fields too. The work that I did as a graduate student was not a formal collaboration by my advisor with somebody in some other field. But I had all these friends in grad school who were in different fields, and I just learned a tremendous amount from them about science and technology broadly.
But also I learned many things from them that were specifically useful in my research. I said that I built this computer interface for an EPR spectrometer. I didn't know anything about electronics when I came to grad school, but I had friends who were in the electrical engineering department. They taught me how to design integrated circuit-based electronics, and how to go down to the electronics shop in their department, and buy the right parts, and build the thing. I learned a tremendous amount about computation from people in the civil engineering department and from people who were staff members in the physics department who ran the computer resources there. It was just an environment where you could meet all kinds of interesting scientists. People love to talk about science, and you could pick their brains.
ZIERLER: Interdisciplinarity was not a buzzword, it was a way of life, is what you're saying?
GELLES: Yeah, that's exactly right. I think that the smallness of the institution really facilitated that. When I was looking for faculty jobs, that was one of the things that really attracted me to Brandeis. We're also a very small institution, and we're also an institution here where the different sciences are usually closely intermeshed. For most of our science departments, we don't have a separate departmental building. Instead, we just have a bunch of science buildings, and faculty from different departments are sort of intermixed in them. We have a lot of programs where we train students from different disciplines together in the same program, and that's really helped to create the same kind of environment here where there's just a lot of informal contacts and talking in the hallway with scientists who work in other areas. Sometimes you're just chatting with somebody, and you find out something that you can use to your advantage.
The Fertile Field of Kinesin Research
ZIERLER: Jeff, what postdocs were you considering?
GELLES: As I said, I thought that molecular motors were really interesting because of this potential because, again, it's an enzyme that converts one kind of energy into another kind of energy, just like these other systems that I had been studying. That's called free-energy transduction in the lingo of the field. I talked to several labs that were doing that kind of work. Then there was another less-related but important scientific development at around that time, which was that people had figured out how membrane-based transport systems work. These are the systems where cells endocytose material from outside of the cell by pinching off the cell membrane into a spherical vesicle, and then that vesicle gets transported to internal membranes in the cell. There's a whole bunch of really important biological processes that are involved. People had just really made the key discoveries to figure out the basics of how that works at that time. I applied to several labs working in that field as well.
ZIERLER: Why ultimately the decision that you made? What was compelling?
GELLES: I think that as I learned more about the vesicle thing, I learned that it was really a pretty complicated system, and it might be hard to tackle at the level of biochemical mechanism that I was interested in. I also met my eventual postdoctoral advisor Mike Sheetz, and Mike convinced me that the kinesin problem was really a problem that I could sink my teeth into, and explore my interest both in biochemistry and biophysics on that system. It didn't hurt at all that Mike was a Caltech graduate, and had done his dissertation with Sunney Chan. [laugh]
ZIERLER: Tell me about Mike Sheetz. What was he known for? What was his main research?
GELLES: Mike had done a lot of work on the membrane and the cytoskeleton of red blood cells, including studying the actin-based molecular motor myosin. But Mike had been a professor at the University of Connecticut, and he used to go to the Marine Biological Laboratory in Woods Hole, Massachusetts to do summer research there. When he was there, there was one key summer, which was I think the summer of 1985, where he teamed up with some other people who were already at Woods Hole, and who were, as it happens, involved in a very acrimonious competition with another group of people studying the same thing who were also at Woods Hole. Both groups discovered this molecular motor kinesin, which turned out to be a really important discovery, and it was important both for the biology of what kinesin does, but also kinesin turned out to be really the perfect molecular motor to do biophysics and detailed biochemistry on. The initial work had just been published when I was looking for a postdoc, and that was very exciting.
ZIERLER: Did you see that as more a continuation of what you had done at Caltech, or as the foundation of what you would pursue as a professor?
GELLES: Absolutely both. It was a continuation in the sense that it was another one of these energy transduction systems. It was the foundation of me really wanting to develop these light microscopy-based systems for studying single molecules, which, in the case of these molecular motors, we could do because what I did as a postdoc was develop methods to use light microscopy to measure the nanometer scale movements of the molecular motors as they move, enabling the study of individual motors one at a time. That really gave us a way in to study the mechanism by which they work.
ZIERLER: Now the term "molecular machines or motors," was that in use back then, or this is a more recent phenomenon?
GELLES: No, definitely in use.
ZIERLER: You were unsatisfied with the metaphor even then? You recognized that it wasn't exactly a machine?
GELLES: I don't think I thought about it at the time. I came later to the belief that the metaphor can lead one into non-productive ways to think about how these biological systems work.
ZIERLER: Jeff, what was the primary value of your postdoc?
GELLES: The primary value? One value was that it got me working on kinesin, which I worked on for many years after that, and there was a lot of interesting stuff to come out of that.
It also continued my interest that I had started as a high school student in developing instrumentation. Especially in that period of time, it was quite an amazing thing, the idea that you could have a minicomputer in your biochemistry laboratory to run instrumentation and to analyze data. Before the mid-1970s, that was relatively uncommon. I was able to do instrumentation work that really facilitated what we were doing, and I was able to not only use what I knew about biochemistry but also the statistical physics that I learned from Howard Berg, because those statistical approaches are absolutely essential for analyzing single-molecule data.
ZIERLER: When did you feel ready to go on the job market? When did your postdoc feel like there was a sense of completion, or you were ready to articulate a research agenda as an assistant professor?
GELLES: I published one paper in Mike's lab, and I was thinking that maybe I needed to spend another year with Mike. Mike got invited to Brandeis to give a seminar and talk about my work. He got a call from Chris Miller, who was a professor here. I talked before about the ACS Guide to Chemical Research. When I was looking for graduate schools, Chris had just started at Brandeis, and I remember reading about his research and being really interested in it, because Chris worked on ion channels, and his was one of the early labs who did single-channel experiments. Chris called up Mike and said, "That postdoc, is he applying for jobs?" Mike said no. Chris said, "We have a job opening, and he should apply for it," and so I did, and they offered me the job. I was very fortunate that I got offered this job at Brandeis, because Brandeis was a particularly good place for me. Not only was it the kind of small school that I liked, it was a teaching-oriented place, which was what I was really looking for. It had Chris, who was working on the one other main system where you could study single molecules, and it had a bunch of people, a really world-famous set of professors who were working on myosin, which is one of the only two types of molecular motors other than kinesins that work in the cytoskeleton. It was really the ideal combination of colleagues and the type of school that I wanted to be at.
ZIERLER: Now, the emphasis on teaching at Brandeis, were you hearkening back to your experiences as a Harvard undergraduate, that this was important to you on the other side of this relationship?
GELLES: I think it was actually my experiences as a grad student. When I was a grad student, I really taught a lot. I think I was a teaching assistant for more than half of my years in grad school. A lot of what I taught was just freshman chemistry. I thought that teaching it was very rewarding personally, but it also made me realize how valuable it is intellectually, and how you can learn about science in ways that I personally aren't really able to achieve unless it's something that I've actually taught about. In a way, teaching about something just imposes a kind of self-discipline about learning the science, a self-discipline that is hard for some of us to achieve in other ways.
ZIERLER: Jeff, tell me about Brandeis support for assistant faculty. What was your start-up package like, and what were the most important external funding agencies?
GELLES: The funding agency was clearly NIH. Other than some junior faculty start-up grants from various foundations, my research throughout my entire career has been funded by NIH. I don't even remember how much my start-up package was, but it was enough to get going.
ZIERLER: Now, in the '90s, the famous doubling of the budget at NIH, did that register with you? Were you a beneficiary of that?
GELLES: I'm sure I was a beneficiary of it in the sense that I don't remember what my scores on grants were in those days, but it certainly made it easier to get funding. I don't think it really changed the amount of funding very much for individual labs, at least not for mine.
ZIERLER: Now, in building the lab, was the emphasis on light microscopy from the beginning?
GELLES: Absolutely, yeah.
ZIERLER: Were there advances, the opportunity to buy new instruments, where there were innovations that made the research better?
GELLES: When I started as a postdoc, I really didn't know anything about optics or microscopy, so I had to learn all that stuff. When I was a postdoc, I built a microscope in Mike's lab that was specifically designed to do these nanometer-scale movement measurements. That's the work that I continued when I started in my new lab. All of the microscopes that we use in our research are either completely home-built or substantial parts of them are home-built. The microscopes that we do most of our work on nowadays, there are almost no commercial microscope parts in them at all, except for the objective lens.
ZIERLER: Tell me about the early years of the Gelles lab. What were the things that the graduate students were focused on?
GELLES: We did lots of experiments trying to understand how kinesin works. How does it hydrolyze ATP, and use the energy from that to move unidirectionally along a microtubule filament? How does it move without letting go and falling off? The answer turns out to be that kinesin has two structures that are called heads, and it walks along the microtubule, alternately stepping the two heads forward. The steps are eight nanometers in length, which is the spacing between tubulin dimers in the lattice of the microtubule. It's basically just stepping from one binding site to the next, to the next, as it moves along. It does that in a way that is coupled to ATP hydrolysis, which in these energy transduction systems basically is tantamount to saying that it can't go through the whole cycle of hydrolyzing ATP unless that cycle is accompanied by the stereotypic movements of stepping along.
All of my students were working on questions like that, and every single student in the lab was doing these experiments where we were monitoring the movement of single kinesin molecules as they moved on the nanometer scale. This was something that was definitely physically possible, but there was a sizable number of people in the community who were convinced that it was impossible because there's a stereotypic orientation toward light microscopy, which is that it can only be used to study things that are larger than the wavelength of light that can be resolved by the microscope, which of course is the micrometer scale, not the nanometer scale. Biologists tended to think that if you want to look at the nanometer scale, use an electron microscope. If you want to look at something big like a cell, you use a light microscope. Here, what we were doing is we were studying single molecules, nanometer-sized objects, and measuring their nanometer-sized movements. The reason that you can do that is because if you're just looking at a single isolated object that is far away from other objects on the micrometer-sized scale, you can detect very small movements. Some people had to think about that for a while before they got it.
ZIERLER: Jeff, what was the tenure process like at Brandeis? Was there an opportunity to summarize what you had done so far?
GELLES: Oh, absolutely. The tenure process back then was, I think, very similar to what we do now. You had to write a summary of what research you had done, and what your intended future directions were. But, of course, probably what people write for their tenure committee is not as important as just what papers they've published. The department solicits recommendations from other people who are experts in your particular field, because want to know what people in the field think about the candidate's work.
ZIERLER: As a composite of the key papers, as an assistant professor, what were the contributions? What could you point to?
GELLES: My lab was mainly working on kinesin. But even before I got to Brandeis, I had collaborated with another professor at Washington University, Bob Landick, who worked on DNA transcription. He knew about the work that was going on in Mike's lab. I met him, and we got to talking. I was explaining how kinesin uses the energy from ATP hydrolysis to drive its movement along the microtubule. We realized that even though people hadn't really thought about it that way before, that RNA polymerase, the enzyme that makes RNA transcripts of genes and DNA, is also a molecular motor in the sense that it catalyzes an energetically downhill chemical reaction, which is taking nucleotides, and stringing them together to make an RNA. It uses the free energy that's liberated from that reaction to power its movement along the DNA molecule so that it can read one base and the next and the next. Again, it's another system where we have this enzyme, and it's moving along a track, and it's taking constant size steps, and each step is coupled to a single cycle of an enzymatic reaction. Bob and I and Dorothy Schafer and Mike Sheetz were working on this project to see if we could take the nanometer-scale tracking technology that I had developed to study kinesin, and apply it to movements of RNA polymerase along DNA. That was something that we hadn't quite gotten to work when I was in St. Louis, and so I brought the project with me to Brandeis, and I and the same Washing University collaborators eventually got it to work. Doing subsequent single molecule experiments on transcription was also a big part of the work that I did as an assistant professor. Fast-forward to the present, now that's much more of the bulk of what I do, although we have better single molecule techniques now where we can study processes that don't necessarily involve movement.
ZIERLER: Jeff, you mentioned, as part of the tenure process, there was a future-looking opportunity to extrapolate what you had done and where you were headed. I wonder if you can reflect on that, and if you've stayed more or less true to that plan.
New Technology for New Research Pursuits
GELLES: I'm trying to remember. I would have been up for tenure in like 1995 or '96. I think the answer to that is probably yes. I'd hate for this to sound like it's—
ZIERLER: Overly linear?
GELLES: I'd hate for it to sound like it's all planned out years in advance. But, really, a lot of the work that I've done as a scientist, it's been motivated by saying, here's a problem where there are things that we'd really like to study, and we can't currently study them very well. Then here's a tool which really sounds like it has great potential to study these things, if we could just develop it and get it to the point where we can do the experiments. In some cases, the developing and getting to the tool to point where we could do the experiments has taken more than a decade. But we've been able to do that, and the tools have just turned out to be even more valuable than I originally thought that they would be, because there are ways to studying biologically important molecular systems at a level of insight that we really couldn't get from the methods that existed before.
ZIERLER: Jeff, was the Human Genome Project, as it was ramping up, were you following that? Were you part of that at all?
GELLES: That's a very interesting question. The Human Genome Project, and particularly the instrumentation and methods that made it possible, for instance, things that made it possible to do high-throughput DNA sequencing, that was really a big deal at Caltech in the '80s, particularly from the work of Leroy Hood and other people. That had an enormous influence on scientific life on the Caltech campus, and on the field as a whole, of course, later on. People were very excited about that. I knew lots of people who worked in Lee's lab who were working on that stuff. I have to say that I did not find it interesting. [laugh] It was clearly something that was going to be very valuable and important, but it was not something that I thought that I personally would like to work on.
ZIERLER: Because it was too applied or because it was too big science-y?
GELLES: No. It was because it was not thinking about biology and biochemistry at the level that I wanted to understand it, and it just was not the sort of problem that I really find engaging in science. It was more like a methodological development that enabled you to do interesting things, but the methodology wasn't really tied into the biology. It was just kind of a means to an end.
ZIERLER: Jeff, what about biotech around Cambridge and the Greater Boston area? When did that start to register as a significant force? When did you start having students who thought about biotech as a viable career path?
GELLES: I'm trying to think. I think in my early years as a professor, I didn't have much visibility into what was going on in the industry at all. I don't know whether there were biotech companies around here, and I just wasn't aware of them. Very few of my early students ended up doing biotech. I think the industry has, especially in this neighborhood, it's really expanded a lot, in the last two decades especially.
ZIERLER: What about biotech as a place where students with fundamental interests can carve out a research agenda that might not be entirely directed towards some particular business goal? What have you seen in that regard from your students?
GELLES: I'm not very aware of that. Famously in the early years of Google, and maybe still, for all I know, there was this understanding that they would let people spend 20% of their time working on their own projects. I have never heard of a biotech company that did something like that.
ZIERLER: The broader question is, and I'm just generally curious why Bell Labs as a model has never quite caught on for biology? Why not?
GELLES: That's a good question. Of course, Bell Labs as a model eventually didn't catch on even as a model for Bell Labs.
ZIERLER: Fair enough. [laugh]
GELLES: I had lots of friends at Caltech who were really involved in the early days of Unix, and some of them eventually left Caltech and went to work at Bell Labs. Then they all got fired when Bell Labs shut down. [laugh] Maybe the biotech companies just decided early on that that model wasn't tenable. I would love to think that it's in the economic interest of companies to devote some of their resources toward basic research, but I think the verdict is in, and the companies don't think that. When I graduated from Caltech in 1986, many, many of my classmates in the chemistry department who graduated the same year went off to work for Bell Lab-like research operations that were run by different chemical and petroleum companies.
Many of those people who took those jobs, they graduated with their PhD, they went to work with the company, and they were out of work within a year, because that was right when all of those basic research labs shut down. Actually, a similar thing happened to my dad during his scientific career. He's a materials scientist, a metallurgist, and he started out working at a basic research lab that the Kennecott Copper Corporation ran in the Boston area. At some point, they just decided no more basic research, and they closed the whole thing down.
ZIERLER: Jeff, in moving our conversation closer to the present, this is going to be a very general question, but I wonder if you could historicize it in the narrative. Your discoveries, your interest in all of the dynamism that's happening at the molecular level, is that really—and, again, not to overemphasize the linearity or the preplanned nature of it—but is that with you the entire time in your research career? Is there a certain phase, conceptually, with advances in the instrumentation, where it moves from a hunch, and you're really studying it as a concept in and of itself?
GELLES: It's definitely something that's been known since before I was a scientist. But I think—
ZIERLER: I mean, studying it at the level that you're now working in?
GELLES: I think that it's the losing your watch under the lamppost story. You know that one?
ZIERLER: Of course.
GELLES: I think people have always thought that, in principle, it's important, but in practice, the tools we had, like this X-ray crystallography technique that we use to study protein structures, by its nature, that tool tells you about the static structure of the protein where nearly all the movement is frozen out. That's the lamppost under which we could look as a field, and so people tended to do that. When I started working on motor proteins, it's very natural to think about the dynamics because the dynamics are essential to how motors function. Then as the field has moved along over the decades, there have been more and more revelations that make it clear that dynamics are really key to the way most or all proteins function in the cell.
ZIERLER: Jeff, when COVID hit, and all the avalanche of interest and research on mRNA, had some of your work, was it relevant in the specific application of studying mRNA for the purpose of making a COVID vaccine?
GELLES: I don't really think so. The sort of aspects of mRNA biochemistry that are important for the COVID vaccine, a lot of it goes back to the early days of molecular biology in the 1960s. The key discoveries that made the COVID vaccine possible is actually nothing to do with how mRNA works. It just has to do with some really key discoveries that were made to understand how you can actually inject mRNA into people, and not have it be attacked as a foreign molecule and degraded. But the basic science behind the vaccine, it's really old stuff, and it's an example of something where people were studying something just for the pure basic interest in understanding how biology works, but with the confidence that the information would be valuable in many, many different ways, as it has proven to be many times.
ZIERLER: Were you able to get an exemption to keep your lab open because of your work in mRNA?
GELLES: Not really, no. We were closed down for a while. Brandeis was particularly good at getting the labs started back up, and working under conditions where people could be safe. That was very challenging, but the university was pretty flexible, and managed to get us back up and working after only a few months. Bringing the research back online went pretty well. Getting the teaching part working effectively, that was very challenging.
Current Focus on Eukaryotic Transcription
ZIERLER: Sure. Jeff, we'll bring the story right up to the present. What are you currently focused on? What are some of the new directions in your lab?
GELLES: I told you about this project on eukaryotic transcription. We're really excited about that. It is an infernally complex process, and we really think that the approach that we've developed to study it—which we've now done enough times to show that it works well—we think that it's going to make a valuable contribution to the field. It's certainly not by itself going to answer all of the questions. But we've developed a way to study these processes that other people haven't really been doing or been able to do that we think fills an important gap between studying transcription processes in live cells, and studying them in the test tube in reconstituted systems. These extract systems are somewhere in the middle. As I said before, they've given us insights that I'm very excited about. These systems, there's so much to learn about them that — if I were 30 again — I think I could have a whole second scientific career just working on that one thing. In addition to that, I have another big collaboration in the Boston area that I didn't talk in much detail about, which is with Stephen Bell's lab at MIT. This is a collaboration to study the replication of DNA molecules. We're studying the machinery that makes a copy of the genome to put in a daughter cell in eukaryotes. That system and the transcription system and the cytoskeletal systems, the thing that they really have in common is there's this complex regulation that involves multiple proteins that's been very hard to study in bulk samples, but where the single molecule approaches have really given us a lot of insight. In all of these systems, there's [laugh] just a lot more work to do. We've really been fortunate in having really fantastic collaborators, and I've really enjoyed this work because not only are we successfully doing science, but, by working with these great collaborators, I've been able to learn from excellent teachers about new things in the field, which I really enjoy a lot.
ZIERLER: Jeff, to close this conversation, I'd like to ask a few retrospective questions about your career, and then we can end looking to the future. Of course, what brings us together is Caltech. It's been implicit throughout the conversation, but let me ask you straight on now. What has stayed with you from Caltech, both in your approach to the science, your approach to collaborating widely, your approach to technology?
GELLES: I think that collaboration and the value of interacting with people from different fields, those are some of the most important things. As I think I've indicated, those have been central themes in my scientific career. Caltech was just such an exciting place. I think that people's enthusiasm for science really infected me as a grad student, and that continues to this day.
ZIERLER: It's as much a philosophical and it is a scientific question, but given your dual interests in fundamental research and instrumentation and building technology, where do you see technology driving discovery, and where do you see discovery driving technology?
GELLES: In my field, they're really inextricably intertwined. There's a lot of things that people do because they can do those things now. I would even go so far to say that a some of the things that people do just because they can do, those things may not be so interesting. The technology has enabled key developments but probably also has enabled some things that were a waste of time. On the other hand, there are many examples in my field where there was a clear need to develop a technology to do a particular thing, and people went out and did it, and it's made a big difference. I hope that the kind of technology development that my lab has done is mostly in that category.
ZIERLER: What discovery or line of research feels most complete to you, where you approach something where something was very poorly understood or not understood at all, and now is understood well in the context of what that means in science?
GELLES: I think that a lot of the things that we've worked on were just things where we went and we saw something in the field that people kind of accepted as true, but the data supporting it wasn't very good. We said, "Oh, this looks like something that maybe the single molecule techniques would be valuable to study." A lot of times when we looked into those things, we found out that what the field thought was partially or completely wrong, and the single molecule stuff really has enabled us to correct our view of what's going on in the system. Then there are other examples like the early work on kinesin, and it was really clear from the outset that there were well-defined questions to which we didn't know the answers, and we set off and figured them out.
The Endless Complexity of Biology
ZIERLER: In elucidating all of the complexity that we now understand in a cell, what is the end goal of this research? Is it ultimately to find a simplicity at the most constituent basic parts, or is the complexity endless?
GELLES: [laugh] I think that there are some things in biochemistry and biophysics where there really is a more universal underlying story behind what you see in the biology. I think that's particularly true in the physical understanding of some of these molecular processes. There is definitely also endless complexity in the details, and I think that that complexity is one of the big challenges of being a biologist. The challenge is, how do we learn something that's useful and humanly understandable, based on the complexity that we see? I think there are definitely ways to do that. But it's sort of a different intellectual process than it is to study human-made systems where there's some underlying designed purpose.
ZIERLER: Finally, Jeff, one more question, looking to the future. You mused earlier, you know, if you were 30 again, you could see a brand-new scientific career in one, in many areas. Of course, in the absence of that, you have your students. What do you see them doing that is going to continue all of the lines of inquiry that you've opened up?
GELLES: Some of my students who are academics who are PIs of labs, some of them are doing things that continue the work that they did when they were in my lab. Some of them are working on completely new things, that may or may not use little parts of the approaches that they learned in my lab, and the approaches that they learned in the rest of their training, and putting it together to be their own unique kind of scientist.
ZIERLER: I imagine it doesn't get more satisfying than that, actually.
GELLES: A lot of them have done really very well, and I really enjoy talking with them about what they're doing.
ZIERLER: Well, Jeff, this has been a wonderful conversation. I want to thank you so much for sharing your perspective with me.
[End]
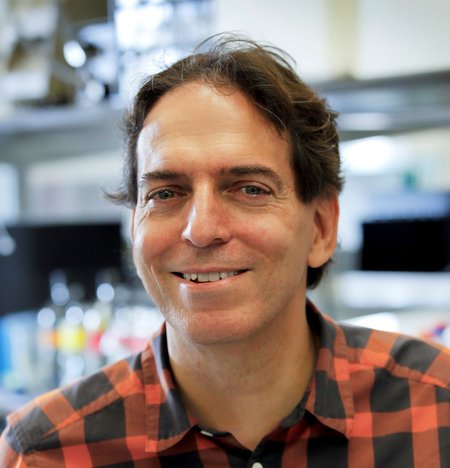
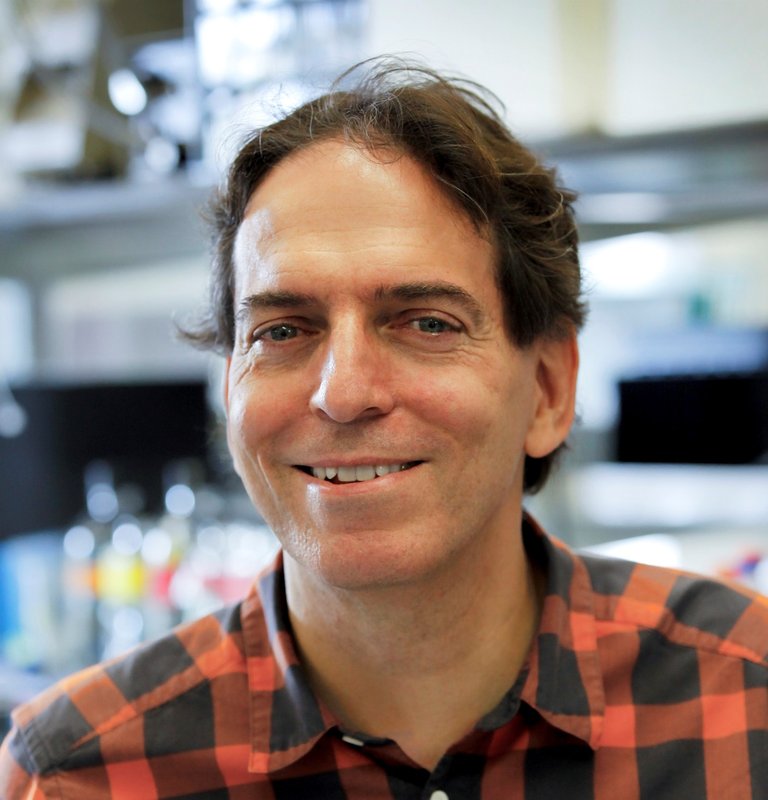
Interview Highlights
- The Limits of Metaphors in Biology
- The Connecting Point of Cytoskeletons
- The Conceptual Importance of Condensed Matter Physics
- Longstanding Fascination with Biochemistry
- Meeting Harry Gray
- The Limits of Studying Protein Membrane Structure
- Caltech and the Many Bridges Between Biology and Chemistry
- The Fertile Field of Kinesin Research
- New Technology for New Research Pursuits
- Current Focus on Eukaryotic Transcription
- The Endless Complexity of Biology