September 28, October 13, October 19, October 26 and November 1, 2021
Since graduate school, John Bercaw has tackled some of the most fundamental issues relating to catalysis, the process by which the rate of a chemical reaction increases by using a catalyst. Bercaw's contributions range from key discoveries in basic research to experiments that have led to broad social impact, and his findings have helped shape the field of organometallic chemistry.
Born in Cincinatti, Bercaw earned his undergraduate degree from North Carolina State University, and he completed his doctorate on the structure of titanocene at the University of Michigan under the direction of Hans Herbert Brintzinger. As a postdoctoral scholar with Jack Halpern at the University of Chicago, Bercaw decided that he would pursue a career in academia rather than industry.
Bercaw joined the faculty at Caltech in 1974. He took advantage of recent advances in nuclear magnetic resonance spectroscopy and pursued methods of carbon monoxide reduction before turning to fundamental questions relating to the thermodynamics of chemical reactions. Bercaw's research has led to important insights on the mechanisms of Ziegler-Natta olefin polymerization which contributed to a revolution in industry's use of polyolefins.
Bercaw is the recipient of some of the most prestigious awards in chemistry, including the American Chemical Society's Award in Pure Chemistry, and the Award in Organometallic Chemistry. He was elected to the National Academy of Sciences in 1990, and he was named Centennial Professor in 1993. Beyond his own research, Bercaw is noted for his achievements in education and mentorship. Over the past four decades, students in his research group have gone on to positions of leadership throughout academia, government, industry.
Interview Transcript
DAVID ZIERLER: This is David Zierler, Director of the Caltech Heritage Project. It's Tuesday, September 28th, 2021. I am delighted to be here with Professor John E. Bercaw. John, it's great to see you. Thank you so much for joining me today.
JOHN BERCAW: My pleasure, David.
ZIERLER: John, to start would you please tell me your title and institutional affiliation?
BERCAW: My title is Centennial Professor, Emeritus. I'm in the Department of Chemistry. Well, I was in the Department of Chemistry as an active faculty member. I still have an appointment there as an emeritus faculty member at the California Institute of Technology.
ZIERLER: Now, Centennial Professor. What is the meaning of the significance behind the word centennial? Centennial of what?
BERCAW: The professorship was offered to me as the first holder, and it was decided by the donors of the endowment to celebrate the hundredth year of Caltech's existence. They chose at that point in their lives not to make their gift public. They then remained anonymous for many years, and they finally caved in. They've given so much money to Caltech. [laugh] I think they figured that it wouldn't change if their name was available to everyone. Of course, it was known to Caltech, but not to the public, more generally.
ZIERLER: Who were the benefactors?
BERCAW: Shirley and Carl Larson.
ZIERLER: I see.
BERCAW: They've also given chairs for the provost and other projects. I'll just put it this way: They've given many other gifts to Caltech, and they've been very generous.
ZIERLER: John, what year did you go emeritus?
BERCAW: In 2015. More than six years ago.
ZIERLER: In what ways has going emeritus allowed you to shed administrative responsibilities and simply focus on the science that's more interesting to you?
BERCAW: [laugh] It's not been complete. I'll put it that way. I'm still in some demand. Although I've not been asked to serve on committees at all. I have voluntarily worked at Caltech for several years. In fact, I think for the first three or four years that I was emeritus status I maintained a laboratory. I still have a laboratory, a Noyes laboratory, and my equipment is all there. I went in the lab and did experiments myself. Something I've always wanted was to actually do chemistry, even when I was no longer able later in my career. Since I'm a synthetic chemist, and I studied reaction mechanisms, I've very much enjoyed leading the experimental aspects of our research projects. When I was a full professor, especially, I had very little time to go in the lab other than when I was at group meeting and one of my group members was presenting something that I could see that that student or postdoc wasn't doing the proper experiment or using the right technique. Then I would actually go in the lab with that person and show them how to do it correctly. I guess I've always been very good at experimental chemistry. I never had anybody tell me that having me show them the proper technique was very embarrassing for them. They were always very appreciative. By the way, other group members would always crowd around the door to the lab I was in and watch with great interest to see what was going on. [laugh] After going emeritus I had a chance to go in there and work by myself, but I also helped out some of the graduate students from Theo Agapie's lab. Theo is a professor in chemistry. He was one of my graduate students. He has a similar approach to chemistry, and his students welcomed the opportunity to use some of my equipment and to learn from me. I enjoyed that. So I guess that I was still teaching in that way. Caltech has been very kind to me and not picked on me after I went emeritus to do the things that I didn't want to do most of my career. I served on various committees, search committees for everything from the division chair to Vice Presidents to the Provost. I was very active in Institute and Divisional committees. Although I never served as division chair, I served as executive officer, and I had other roles like as academic officer that was briefly created by the division chair. We had standing committees in the division, and I chaired most of them at some point, including the one that takes the most time, the so-called staffing committee. I don't have to serve on any of those committees anymore, and I'm very grateful for that. I have spent about half of my time away from Caltech during the first three or four years of my emeritus status. After that and especially when the pandemic struck, and it was advised by the upper management of the institute that we should not go to lab unless we absolutely had to, I have minimized my presence there during the last almost two years now.
ZIERLER: But you still have an active laboratory? You have graduate students and postdocs who keep things going?
BERCAW: No. I still have a little bit of research funding, but I don't have enough to fund a grad student, nor is it permitted for an emeritus status professor. You can't have a graduate student. You can have a postdoctoral fellow, but since I have the second home in Arizona, and I've been spending quite a bit of time there, I didn't feel right about having a postdoc that I would leave for more than half of the time to work alone in the lab. But I do have a lab. The last graduate student that worked with me—although officially signed up with my colleague, Theo Agapie—Meaghan Bruening still voluntarily keeps some of my equipment going and fills liquid nitrogen traps on the large vacuum line when needed. She checks on the inert atmosphere glove box that I use. She's one of the people I trained to know how that equipment works, and she's used it regularly. She keeps saying, "You need to come back and use your laboratory. It still works fine, John, and it's a beautiful lab."
ZIERLER: [laugh]
BERCAW: When she enters the lab she says, "Your laboratory is absolutely spectacular. I have never seen any glassware so clean, and everything's so tidy. It's a wonder. It's so different than my other group." [laugh] I guess I am very… anal. I don't spend very much time at Caltech now, but I probably will increase that time when everything is open. It seems to be starting in that direction now.
ZIERLER: John, a question we've all been dealing with, particularly for you being so far away from Caltech. How have you remained active in chemistry? What have you been reading? What have you been writing? What's been interesting to you in this past year and a half?
BERCAW: Oh. I still read some articles that people send to me just for my own information. They don't really request any feedback. I don't regularly read the journals anymore. I have a full-time job here with my wife looking after our ranch. [laugh] Especially this past year because we've had a very severe monsoon season. We've had several flash floods run through the wash on our property that wiped out some fencing that helps keep our dogs contained. I've been working the last three or four months just getting those fences repaired. I've enjoyed myself doing something different. I'm also an amateur photographer. This part of the world is really beautiful, so I've been out taking pictures and enjoying the scenery. I haven't been able to keep up to speed on my chemistry. I do look at the Caltech website and all of the emails I get from various people at Caltech. I try to respond when former students and current students and postdocs ask me for letters of recommendation. I have a bunch of them, over 150. Many are in academics themselves, and that requires regular nominations and letters for promotions and various other jobs. Mostly it's been informal, not an intense, focused reading of the literature. I'm very happy, so I guess I'm doing the right thing.
The Basics of Synthetic Chemistry
ZIERLER: That works. John, before we go back and develop your personal family narrative, I'd like to ask a few very broadly conceived overall questions about your research and your approach to chemistry. First perhaps at the most fundamental level—your field of synthetic chemistry—do you see chemistry essentially broken down into two parts: organic chemistry and synthetic chemistry? Is that the proper binary from which all chemistry goes?
BERCAW: No. I wouldn't say that's the way it breaks down. People who make molecules are called synthetic chemists and people who study the reactions and the mechanisms of those reactions and explore the properties of those new molecules are from all fields of chemistry and are called other types of chemists, not only organic chemists. Most organic synthesis is conducted by organic chemists, and physical chemists study these things using modern spectroscopic techniques and increasingly, computational methods to rationalize why we see what we see and make predictions, although they're very difficult to make. Traditional ways in which chemistry is broken down is by area of chemistry, one area is physical chemistry or chemical physics. Some people like to be called physical chemists; others prefer chemical physicists. They construct new instruments and use various types of spectroscopy and theory. Organic chemistry consists of both synthetic and mechanistic people, and many organic chemists do both synthesis and mechanism. Then there's inorganic chemistry, really the closest area where to where I work. I grew up as an inorganic chemist. Inorganic chemistry is not so much what you might think from the literal definition: not organic chemistry. [laugh] Inorganic chemistry is the chemistry of all of the elements including carbon and hydrogen and oxygen and nitrogen and phosphorous and sulfur, the elements that organic chemists study a lot.
So, we're broader. We look at elements in the entire periodic table. It's a relatively new area. In fact, I think we're now celebrating our 65th year as a division of the American Chemical Society, The Inorganic Division. Harry Gray was one of the people—I don't know if you've heard of Harry Gray? He is a very distinguished chemist and a great joy to be around and one of my very, very best friends. He along with another person hired me at Caltech. We remain close. In fact, we email each other regularly. Anyway, Harry was a big promoter of forming a new division, the Division of Inorganic Chemistry. Especially early on, inorganic chemists undertook structural determinations using X-ray diffraction to get the so-called crystal structure of the novel molecules that were being synthesized. They also studied the electronic structure of these molecules that inorganic chemists were making. Unlike organic molecules transition metals have the d and f orbitals involved in bonding. Such transition metal complexes are of great interest because they display beautiful colors and have applications that often involve their photochemistry. Inorganic chemists examine the nature of the photoexcited states and what the emission spectra look like. Transition metal complexes are found in biology as well. That brings me to the next area, big area of chemistry, and that's biochemistry. Or again, chemical biology depending on who you are and what you prefer to be called. There are those four areas that make up most chemistry departments. Especially in the Midwest, but also in a few other schools, there was also an area called analytical chemistry, which is what it sounds like. It is a study of methods that are used to analyze the molecules in terms of their constituents, but also like electrochemistry where they study the oxidation and reduction properties of them and what you could learn from those properties. More recently it's not so popular to consider analytical chemistry as a separate part of chemistry, except in the few schools that have been very strong in analytical chemistry traditionally. In fact, Caltech had a couple of analytical chemists and they're gone. They retired and that was the end of the analytical area of chemistry at Caltech, and that's probably our loss. Now more recently there's a materials area of chemistry that is growing and will probably be its own area in the department in addition to the other four.
Chemistry is growing at the interface with a lot of other disciplines like physics and biology, and as I just said, materials science. More generally, some of us think chemistry is the central science and so it's only natural that scientists working at the interface would be tied to other fields through chemistry. But back to your question about synthetic chemistry. I think that people who make molecules are unique among scientists. We have that gift. We can make pretty much anything we want these days, because synthetic chemistry is so well-developed as a pursuit. We know how to make things in so many different ways now. We have that unique character among all the scientists, we can create new matter. I guess you could argue that nuclear physics can do that too, but we're talking about molecular things now, and nuclear synthesis only occurs at energies where the electrons are fully dissociated, and chemical bonds have all been broken.
ZIERLER: John, do you have a sense of when the term synthetic chemistry first came into wide use?
BERCAW: Wow. I don't. It's been a long time ago. Maybe 200 years ago. I'm just making a guess, but it grew up in Germany and England. Much of chemistry grew up in Germany, and England but it wasn't only synthesis. A lot of the early parts of chemistry were in making new molecules and seeing how elements combined with one another. More recently, I guess in the last century, synthetic chemistry has been a little different than what it had been traditionally in that we can have so-called synthetic targets. For example, we want to make a molecule that has a particular structure because it might be a model for some biological active site, for example, that in hemoglobin, where the heme iron center is where the action is. People made these molecules on purpose. It's gotten very sophisticated especially in so-called natural product synthesis. These are very complicated looking structures that are very precisely constituted, not only in the connectivity but in its stereochemistry. Commonly some of the parts of the molecule are optically active. That is, their mirror image is different from the molecule itself and thus often has different chemical reactivity with other optically active molecules. Making stereochemically pure molecules is really challenging. In fact, some of these complex molecular targets take people most of their career to complete their synthesis. The synthesis of vitamin B12 was a success story on which many research groups collaborated. It has a cobalt at its center, and vitamin B12 has a lot of remarkable reactivity that occurs at cobalt. Getting all the stereochemistry right, the connectivity right, took many research groups years and years competing with one another to be the first one there, but also working together to make the true structure.
ZIERLER: John, as I'm sure you've heard, physicists like to use the metaphor of a pyramid whereby physics is the foundational science, and then up from that is chemistry and then up from that is biology. But from how you're talking about chemistry being in the center, perhaps that's a metaphor that does not make the most sense for you.
BERCAW: You mean the pyramid metaphor?
ZIERLER: That's right.
BERCAW: Yeah. [laugh] No, it doesn't make much sense to me. [laugh] I think of the areas of science differing in the following way: Math is the "purest" science. There's just one solution to a problem and it's precise. You can apply all kinds of logical progressions to get to the answer. Physics is based a lot on math, but it also has a few things that aren't fully understood. In the very depth of the field, they're still asking questions about various states of matter, even for elements, especially in very high energy situations. Also, they don't know fully what really goes on in some of the plasmas and they're still modeling things like that. It's not quite as pure as mathematics. Then chemistry comes next, and we have more and more things that we don't fully understand. Chemists have been very clever. They build the field by doing chemistry and then using intuition formed from knowledge of many other related phenomena to guide them in understanding the new things they observe.
We can't solve the electronic structure of a complicated molecule with the precision that all would like, because the Schrödinger equation is just simply not solvable for things as complicated as molecular problems, especially when molecules are undergoing a chemical reaction. In fact, when I was teaching freshman chemistry at Caltech, I had 220 students in Chem 1. I introduced them to the field by saying, "It's a complicated field. We can't solve everything in closed form like you're used to doing in math and physics. You have to somehow become comfortable with just guessing what the correct thing is and just accept that it happens and that these are the products of a chemical reaction. For example, we can't solve in closed form why, if you pour water on anhydrous aluminum chloride, you will get hydrogen chloride and aluminum hydroxide as products. It's just not fully solvable, but we know what the products are, and we build on that observation to predict other things." Then I always ended that discussion by saying—there was a famous quote. Now I later heard that it may not be actually accurate, but I heard of a quote from Albert Einstein, it goes "The problem with chemistry is it's too complicated for chemists."
ZIERLER: [laugh]
BERCAW: I think that says a lot. [laugh]
ZIERLER: John, for you then with chemistry as the central discipline in your overall research agenda, when do you find the research taking you more into the physics realm? Where do you find it taking it into the biological realm?
BERCAW: [pause] Maybe you should say that question again because I'm not quite sure what you're asking me.
ZIERLER: In your research when are principles of physics most important? Where in your research are biological considerations most important?
BERCAW: [pause] Well, I don't think we can predict those things, but I can tell you that we don't generally choose to work, for example, at the interface with biology unless we have some special interest in that interfacial area with chemistry. Maybe something else in a more traditionally area of chemistry is of more interest to us, for example. The same could be said about the interface with physics. On the other hand, some chemists are sufficiently interested in a problem that starts as largely chemical, and research reveals that the answers to the problem are more biological or physical than chemical in nature. Some chemists would likely choose a new problem and leave further work on the original problem to the biologists. or physicists. Other chemists might choose to pursue the underlying biology or physics of the original problem, even if they must teach themselves the basic biology or physics needed to do so. For example, it's hard to say what shining light on an inorganic solid is. Chemistry or physics? Because we're hoping we can see some electronic response in that solid material. Some people would choose to study the chemistry side of those problems, but they have more physics application. By the same token, sometimes we don't anticipate but guess that the molecules we are making are might have spectacular physical properties that physicists would be fascinated by. For example, especially with the so-called f-block and d-block metals, their molecules often have very high magnetic moments and are of great interest because they have so many unpaired spins in them. There's as many as seven unpaired spins on each metal or even more when you start adding the possible unpaired spins on the ligand systems, the groups that are bonded around them. So, we make these molecules. Sometimes we're building on something that's similar to what we're ultimately targeting, but sometimes we just come across something by serendipity and this one has an amazing magnetic moment. At that point, we bring in physicists or they get interested hearing a lecture by one of us and they approach us and say, "We'd really like to have some of that material and study it." In that way we interact with each other, the physicists and the biologists with the chemists. The chemists choose or not choose to study something because of their own interest and their own expertise.
I think the one area we haven't discussed is catalysis. I don't know if you remember the definition of a catalyst, but in chemistry it's something that makes a chemical reaction proceed more rapidly without undergoing net change in itself. Those are the kinds of molecules that I've targeted in much of my work, especially in my latter career where we were trying to make a catalyst that would perform something of interest to industrial chemists, for example. Or even to people in industry trying to make money from these chemical reactions. Their chemists get involved, but also their chemical engineers get involved using traditional approaches. Many of the molecules of interest to inorganic chemists are involved in enzymatic transformations which are catalytic reactions that occur in life. Catalysis is a sort of all-encompassing interest to many fields, including my own. When I discover a catalyst, industrial people will come to me and say, "Hey, that's really interesting. Can we collaborate with you and see if we could understand it more and maybe make some changes in it that might make it more practical or work better?" I'm welcoming of those kinds of interactions, and I learn from people who have experience. Much of catalysis was discovered by accident and developed by trial and error. Now we have a more molecular understanding of some areas of catalysis. We can change for the better, hopefully, the catalytic process and make the product cheaper and more plentiful and maybe even greener. Greener is a lot in the foreground for chemists these days. We're trying to dispel this negative impression of polluting the world.
Fundamentals and Applications
ZIERLER: [laugh] John, on the question of working with industry, is your research set up in a way where you're always after basic science questions? And then you think about applications or sometimes you're starting point is thinking about applications? I wonder if you can talk about the sequencing of the relationship between basic and applied science?
BERCAW: Well, there are some chemists—and I guess I'm somewhat in that group—who mostly have research funding from the federal government—but sometimes even from industry—to just make something interesting and new. New is really the most important word there. They're given a free license to pursue anything they want, but increasingly over my career I've noticed that people who give money to chemists and to scientists, generally would like us to have in the back of our minds some sort of application in mind. Increasingly it's been in the topics that are important world problems like climate change and related to that, energy and making things that aren't so polluting. I often have to justify these possible outcomes in my research proposals that I send in to get funding so I can do my science. I start out by saying, "Listen. These are the big problems we're trying to address in my group and the possible application in several areas." Then I work my way down to saying why we're doing the experiments we are. A lot of times we think that—and I'll go ahead at the very beginning and say that this is not necessarily true, —by studying in great depth what the structures of molecules are and how things work and we can improve things. We can make new things that perhaps will beat nature at doing something that we would like to do like fixing nitrogen to ammonia, or making dioxygen from water and sunlight like photosynthesis does. We think that if we understand these things in enough depth we can create something that's more efficient and therefore, better and less polluting or perhaps a lot cheaper. I almost never have the freedom to go and work on something that I find fascinating with no application in mind.
There was a time when agencies like the National Science Foundation would say, "OK. You can have"—they would even give you a percentage—maybe one day a week you can work on something you just find fascinating, but you should spend most of this money and your time on the project that you're describing that you justified as having some ultimate application." In a way, it's sort of frustrating for us because…don't forget that we're educators. We're trying to excite students to go into chemistry and do their own thing and be creative and so forth. Often that's true, if we're working on something we really love and we want to pursue no matter what the ultimate application. Increasingly students can get excited and maybe even more excited by problems that have an important application. They wish to believe that they are looking at specific problem that is part of a bigger problem and are thus having a bigger impact. I admire people for having that ultimate goal in mind when they're studying chemistry. As an educator I like to excite my students. I often say when they're coming to me to ask about joining my group and they say, "How often do you make people come to the lab?" I reply, "I don't have any schedule like that. I want you to be excited by what you're doing, and I want you to have to drag yourself out of the lab late in the evening. And I want you to get up early in the morning and charge in there because you're so excited about what you're going to do that day." Of course, that's a romantic ideal, but that has worked very well for me. Exciting students and getting excited myself by it is a great motivator. I think when we're planning our approach and so forth, excitement is a very important driver for my field. I don't like doing mundane things. I want to do something exciting and something that I really truly enjoy.
ZIERLER: John, this is as much a cultural question as a scientific one, but over the course of your career have you noticed increased appetite or willingness in academic scientists to be involved in industrial applications? Where perhaps early in your career there was a more ivory tower approach where we only do pure science, and we don't get involved in business and today perhaps that's really not the case at all. Have you detected that transition over your career?
BERCAW: Oh, yeah. When I first started, gosh, almost 50 years ago many people wanted to just work on fundamental questions. For example, in a large part of organic chemistry—physical organic as it is called—that's the study of mechanisms and all the intricacies of how bonds are broken and made in reactions—physical organic chemists liked to do very so-called fundamental research where we learn the rules and the laws—if there are laws—of how things are supposed to go together and how things won't go together in some other way. They liked very much to be in the ivory tower because there was no immediate applications to understanding why 2+2 reaction was thermally forbidden and maybe photochemically allowed. That kind of thing. It doesn't have immediate application in industry, but over time it got harder and harder to get funded to do that kind of work. People began to work more and more with industry and less with the main funders of science in academic labs, chemistry labs, like the National Science Foundation, the Department of Energy, and National Institutes of Health. They wanted to have some sort of application and often industry drove those preferred areas for funding even in the federal agencies. If you can make just any old molecule in organic chemistry, why not make a pharmaceutical or make something that can be used in health? More and more we sort of sold our souls for the research funding that we needed to do our science. It wasn't all bad that we were doing that because some people didn't like this ivory tower approach. It was much more arbitrary who gets the money if you're doing ivory tower things. Chemists like to give good scores to proposals that are doing science much like they're doing or they find more interesting than something that's going to have an application, but the funding agencies preferred the latter. As time went on I think we got more and more involved with industry. As you probably know in my last part of my funded career I worked with what was called British Petroleum. It was BP. BP bought various other chemical companies, petroleum companies, petrochemical companies, and got bigger and bigger and ultimately they just say BP means BP.
ZIERLER: Yeah.
BERCAW: It doesn't mean British Petroleum anymore. It's bigger than British. Anyway, BP came to us. They actually sought out my research group and asked us if we would like to prepare a proposal for a very large grant that would be part of a great collaboration with BP. We would just do fundamental work, but we would listen to BP about what was most interesting to them, about what we were doing and so forth. It sounded too good to be true, but we prepared a proposal at Caltech. I was leading the work they were interested in along with my colleague and my very good friend and collaborator, Jay Labinger, who is still at Caltech. He is the administrator of the Beckman Institute and a Faculty Associate in CCE. He started out in that role working in the Beckman Institute with Harry Gray, and he has continued as administrator, as the director of the Beckman Institute has changed over time. He is an organometallic chemist like I am, so it was natural that we started collaborating right after he came to Caltech. He wanted to work with graduate students. He wasn't able to formally direct his own students in his role. So he worked with my group and a few other groups as well. He is an incredible intellect and is a very creative guy. We were working in this area of carbon hydrogen bond activation, which has important applications in making liquid fuels from natural gas. Methane is a major component of that. We had already worked in that area and published a few papers that caught their eye. They wanted us to bring in some other faculty, and so Bob Grubbs and Harry Gray and Mark Davis and a couple of others, including Jonas Peters when he was first at Caltech were included. Then later Theo Agapie. We were the Caltech team. We presented our proposal to this group of reviewers that were going around the country to about 10 or 15 schools that had sent in proposals in response to their invitation. In the end Caltech chemists won this competition, but so did UC Berkeley in the chemical engineering department. The chemists were going to be Caltech and the chemical engineers were going to be at Berkeley. Then they split the money, which was still a significant amount, and supported a large number of graduate students and postdocs and a few undergraduates for an amazing—by the time it ended it was down to just Jay and me.—for 17 full years, an unheard of length of time for a specific agreement for corporate support of academic science.
Although there were some skeptical people who were coming to our joint meetings who kept saying, "Well, how is this going to help make money for BP?", we would just ignore those questions because the management of the program kept saying, "You should apply your own ideas and develop your own reactions. Don't just listen to us. We want something new." The problem they were asking us to solve initially was to convert methane into liquid fuels like methanol or gasoline. That is an amazingly tough problem. Of course, industry's been wanting to do that for decades, and they haven't figured out how to do it in a practical process. There were some hints in the literature though that we were getting close to having something that might even be practical. This is my own interpretation: I think that the BP management said, "OK. These are smart guys at Caltech. They're not going solve this problem, but if they do solve the problem it would have such a disruptive effect on all of the petrochemical industry that we need to know about it early on. Even if they don't solve it, they're going to be able to keep their ears to the ground and know who might be close. In that way they would keep us appraised of some promising looking work, then tell us to go read these papers; we think these are correct". Most of them at BP were pleased with it in the end. They kept saying how pleased they were because they knew we were trying to solve this problem. But we had our own approach, and we were also doing lots of fundamental science. They believed that we needed to know the fundamentals, if we would have a good chance to solve the problem. They never hired very many of our people, but they loved working with us, especially the scientists in industry. A lot of them are envious of academics because they like to teach and they like to educate people, and they think we have the perfect job. Well, we do.
ZIERLER: [laugh]
BERCAW: They wanted part of it, and they enjoyed talking to our students and postdocs as well as us. Therein is a case where industry really invited us to submit a proposal. They funded us for at least 10 years longer than they had funded any other project in the history of BP funding academics. We've gotten that far.
There's another funding direction that's also rather extreme: scientists work, especially in the biochemical area and medicinal chemistry areas, and make a discovery that has real value to health or some other areas. They make some sort of breakthrough that looks like it might have commercial value. So, they found a startup company. They will be involved in lots of activities with those startup companies. That'll take them away from their academic research, but maybe also in a positive way it keeps them working on the problems with an academic style. The newly founded company may even financially support the academic work. In that way they're actually helping form a company. For a while it seemed like my colleagues that were most successful had at least one startup company. Some of us, Harry Gray and I most determinedly said, "No startup companies for me." [laugh] We didn't ever pursue that, but many of my colleagues at Caltech and many people around the world were involved in various startup companies. In that way I think they got really close to working in an industrial company.
ZIERLER: John, I'd like to ask you about the role of theory in your research. In other words, when you're thinking about setting up an experiment or how you have your laboratory set up, in what way does theory orient the kinds of questions you're asking at the outset and to know if you're on the right track with what the results tell you?
BERCAW: Hmm. When you say theory do you mean computation or do you mean developing new theoretical methods…my colleagues in theory—they don't have great respect for people who do computations. They just turn the crank that somebody else has developed the methods to compute. Theorists are people like my colleague Rudy Marcus who actually conceives of how things work and then develops a new theory, a set of principles by which he can make predictions. I don't do very much of that. Speaking of Rudy Marcus, Rudy predicted that the rate of electron transfer would increase with increasing driving force until the driving force got to a maximum point. Then if the driving force got too large it would then slow the reaction down. That was the so-called inverted region where the rate went over the maximum of that curve. You know, people like Harry Gray and other people, including at Chicago—John Miller and Gerhard Closs—they actually built molecules to change the driving force over that curve and to look for the inverted region. Actually all found it. Rudy was delighted, of course, He had made this prediction based on his very fundamental ideas about electron transfer rates for which he got the Nobel Prize, by the way. I don't think I've ever done that where I've actually designed molecules with that kind of application. That would happen mainly in physics or physical chemistry.
ZIERLER: I was thinking more along the lines of when you set up an experiment, how do you pose the questions that inform the experiment and then how do you make meaning of the results if there isn't some presupposition about what you might expect the experiment to tell you? I was thinking theory in a more nuts and bolts sense.
BERCAW: OK. So, you were thinking of this as…we have an assumption based on our current understanding of how this reaction's happening, we need to design some experiments to test the validity of that assumption?
ZIERLER: Right.
BERCAW: Yeah. That is very common. We often have an idea, a hypothesis, about how something that we just observed took place. What is the mechanism; what is the stoichiometry of the reaction? That is, how many molecules of this, and how many molecules of that? Then often at a group meeting we discuss how were we going test this. What would be the outcome if it's option A. What would be the predicted result of this experiment that we think up; like how many molecules of dioxygen are consumed in the reaction if the mechanism is option A? That's sometimes not trivial to determine experimentally. We have built capability in our laboratory on a vacuum line in my group. We have the capability of determining how many equivalents of dioxygen has been consumed in this reaction. We would weigh out the reagents and measure the number of moles of dioxygen we start with, so will know how many moles of the reactants we start the reaction with. The oxygen that's remains after reaction would be transferred around the vacuum line into a calibrated volume and its pressure and temperature measured. Then we'll know how many moles of oxygen remain. So we had this special piece of equipment that was designed to answer just those kind of questions.
Sometimes we'll use different isotopes to answer questions. Again, if the mechanism goes by option B we would expect that the isotopes would scrambled completely or would be forced to just make set of isotopologs. We often establish which outcome we reach using a spectroscopic technique such as NMR or mass spectrometer. Sometimes we have a totally unexpected result. I did that very early in my career where the amount of N15 that came out in the hydrazine that we made was not as expected. It forced us to consider a mechanism different than any we had originally considered.
ZIERLER: John, some broad questions on the role of technology. First on the side of instrumentation. Over the course of your career what have been some major advances in instrumentation that have proved to be a game changer not only for the types of experiments that you do, but the answers that these instruments yielded?
BERCAW: Wow. Instrument development is still going full speed, and I don't even understand all of these new methods that are coming out. In organic chemistry NMR spectroscopy was a game changer. When I look back at how organic chemists used to have to go to great lengths to answer the question about a structure of a molecule: it was incredibly slow and painstaking compared to what we are able to do today. We put products of the reaction that makes the new molecule in an NMR spectrometer and look at the spectrum. Analyzing the spectrum is often straightforward, so we understand the structure of the product often in a few minutes. There are also computer programs that will generate the expected NMR spectrum for a target molecule, so the analysis is also now automated. X-ray crystallography is also very important because that gives you a very detailed picture of the molecule when you solve the structure. That's become easier and easier. Now anybody can use the computers to solve it. There are some pitfalls you have to be careful to avoid if you're not a pro, but graduate students regularly solve their own X-ray structures. A few decades ago it used to be a Ph.D. thesis to solve the structure of a molecule. Now it's something you do in as little as a couple of days. You have this three-dimensional structure. Because the diffractometers are now so powerful and the methodologies for solving the diffraction pattern and translating that into a three-dimensional structure are so very powerful. Most recently we do not need to grow a perfect crystal of a molecule in order to get a crystal structure. You can get a microcrystal, and you can solve it with electron diffraction. You can solve small particles, little microcrystals as they're called, that you can see with an electron microscope. Then you can do diffraction on the molecules of the microcrystals individually and solve several structures from a complex mixture of things, which was unheard of several years ago. It's just very expensive. Using the instrumentation and interpreting the electron diffraction pattern is not as easy as I made it sound, but it is coming along, and it's something that is changing the way people who make molecules get structures. You don't have to have an NMR spectrum that you have to interpret. You don't have to grow a crystal that's big enough that you can put it on a diffractometer and solve the structure. Those kinds of things are making major improvements.
I have to say, although I'm perhaps one of the biggest critics of computation, I think that theory and computation have come along and are changing the way we think about the bonding in the molecules and the spectroscopy of the molecules and the reactivity in the molecules. These people are able to predict it. They oversell it, and I can see why they do that, but it does guide the way we think. Many people who do computation—I have a colleague named Bill Goddard who is a theory and computational type. He loves to talk to experimentalists because he wants to know what are the questions we really want to answer with these techniques? Then he focuses his research on that. He is driven by the experimental findings. He likes predictions and sometimes he's actually right about things that I find amazing. Sometimes he's wrong. [laugh] He's an easy-go-lucky guy, and he's happy either way.
ZIERLER: [laugh] Now when you say, John, that you're a critic, you've been frequently a critic of the computational approach, where are you coming from? What's the framework by which you're making these critiques?
BERCAW: OK. Well, there are various theories and one that was very popular and still is very popular with people like me, organometallic chemists, is something called density functional theory. It's not intuitive to somebody who thinks about molecular orbitals and atomic orbitals mixing to make molecular orbitals. It minimizes energy using so-called density functionals. I don't understand how density functional theory works in great depth, but it has been amazingly good at predicting the electronic structure of molecules that people like me make. In other cases, they take it a little bit too far and ask questions that are a little more difficult to answer experimentally. They will apply the theory, but they have a variable that they often don't tell you about. There are different density functionals that you can use to do the computation, and these are tabulated and widely available. Places like Los Alamos have a very popular center for density functionals. More often than I like, if you use a different set you get a different answer than if you use another set. People are casual about saying, "Well, I used this one, and I tested these other ones, and got the same result."
I get a little bit impatient with people like that that don't do the controls. For example, when I was consulting with Exxon, the managers and scientists were very interested in my reaction to their new theory department. They had them present theoretical results to me. I said, "Have you applied your density functionals and your density functional theory to answering these questions that we already know the answer to. That is, have you done the controls by examining some question for which we already have the result?" Or they're always trying to solve the unknown problem that we have not yet solved. They do a calculation on what might be the reason for some phenomenon when we just had observed experimentally. I said, "Well, why do you have confidence in that if you haven't done the basic thing like the control experiment where you calculate something you already know the answer for?" Then do you get that answer that we already know is right? Then you will have some confidence that maybe for this other problem it will work just fine. If not, why should we have enough confidence to actually go out and try. Some people, in fairness, do those controls. I have a colleague named Jay Winkler who's also a member of the Beckman Institute like Jay Labinger. I think he has an even lower opinion of density functional theory than I do He prefers ab initio methods. He said to a class, "You can do density functional theory. It's sort of like looking up a question in Wikipedia. You'll get an answer. The problem is you don't know whether the answer is right or wrong." That is true of Wikipedia and it's true of density functional theory. Although Wikipedia is pretty good.
ZIERLER: [laugh]
BERCAW: Wikipedia has, I think, pretty good controls on making sure there are no bogus websites there. Anyway, that's the basis of my reluctance to put a lot of faith into calculations.
ZIERLER: John, what about the value of computation both for data analysis and simulation for your research?
BERCAW: Computation is very important for analysis. As I said earlier there are software packages that will predict the NMR spectrum of a molecule you can draw on a computer and transfer it into this program. It will predict the spectrum for many nuclei for that matter. You can get some confidence that you made the right thing, if you get that one that's predicted by the computation. I can go on and on with various methods that are used for structure determination. Theory really is important in that way, and it sometimes does stimulate me to do experiments to test the validity of it. People do that regularly and often they find it is right and often they find it is wrong in its prediction. I don't use theory to guide me very often, but sometimes I do. For example, there are some rare phenomena—not so rare, but not very common—in chemistry where you have quantum effects coming into play. Especially when hydrogen atoms are moving around. There can be tunneling phenomena that give rise to very large kinetic isotope effects. If you change hydrogen to deuterium you get the normal rather classical prediction limit of about a 7 to 9 factor is reaction rate at room temperature, the deuterium slows the rate by a factor of the kinetic isotopic effect of about that. When you get something like a factor of 100 or even higher, you often go to computation to try to explain if there is actually quantum mechanical tunneling to the barrier. That's why deuterium is so much slower than hydrogen because hydrogen tunnels so much more effectively than deuterium just based on mass. On one occasion we thought we had a way in which we could look for this anonymously large isotope effect and correlate it with the mechanism. Jay Labinger and I had a graduate student who made many measurements for different chemical systems, to test the generality of this prediction. We were doing great for a while, and then we started getting exceptions to our prediction. [laugh] We had to publish a paper saying, "Nope. We weren't right about that. What we said earlier is wrong and there is no correlation with the mechanism here." Sometimes it does stimulate us to do certain experiments, but it's not that common.
ZIERLER: John, we can now get a little more specific in terms of nomenclature. Let's start first with metal chemistry. There's structural organotransition metal chemistry and mechanistic organotransition metal chemistry. Let's start at the most basic. Would that be metal chemistry? And then organotransition modifies metal chemistry? Or how do these words work together?
BERCAW: Hmm. When you say metal chemistry, that's not very accurate. Almost nobody works with the metal, the element. When chemists first starting working with molecules that have a transition metal (an element with a partially filled d shell for example, elements from Ti to Cu have compounds where the 3d shell has 1 to 9 electrons) in it we tended to call those coordination compounds. Coordination compounds are classic compounds. For example, you coordinate six ammonia molecules around a cobalt ion. that's a "coordination compound". That field was developed early on; Alfred Werner won the Nobel Prize in 1913 for his contribution to that area of transition metal coordination chemistry. When I teach my course I start with classic coordination compounds where we have things like ammonia and chlorine ions and water ("weak field ligands") coordinated to the transition metal cation. It's almost always the metal in some higher than zero-valent oxidation state. We are able to predict something about its color and spin state that come out of understanding the bonding in these coordination compounds. Those concepts are then translated to when we start to substitute for what are called stronger field ligands, especially ligands that have carbon attached to the transition metal. When you have a metal carbon bond then you have an organometallic compound. Sometimes the ligand is neutral like carbon monoxide. You can put six carbon monoxides around a chromium atom and you get a beautiful white crystal and material that's stable even at room temperature in air. Yet, its electronic structure is very different than if you had ammonia's around the chromium. In fact, you can't even get the ammonia's to stick to the chromium if it's in its zero oxidation state. The oxidation state has to be higher to pull these low electron pairs of ammonia into the ion. Carbon monoxide molecule has very different bonding from ammonia to Cr(O).
In general all carbon-based ligands are "strong field ligands", in part because the electronegativity of carbon is lower than nitrogen or chloride or oxygen, so carbon is a stronger base and pushes its electron pairs toward the electropositive metal ion harder than the more electronegative elements. Ligands such as carbon monoxide are also strong field ligands, even though it is hard to protonate the carbon in carbon monoxide. The reason is that carbon monoxide has empty π* molecular orbitals that interact really well with the filled dp orbitals of Cr(O) and thus makes very strong Cr-to-CO π bonds. All of these concepts came from making these carbon-containing molecules and looking at their electronic spectra and other features that made us question how they are different from the classical coordination compounds.
Organometallic chemistry started out with very simple molecules like diethylzinc that was made over 180 years ago. It's very useful for making other things, but it's a very dull molecule. The big breakthrough was when chemists made ferrocene, a so-called sandwich compound where an iron atom sits between two pentagonal cyclopentadienyl groups that have five carbons and five hydrogens. The iron is sandwiched in between those two ligands. The structure of ferrocene forced consideration of different bonding than had previously used to understand molecules such as diethylzinc. The preparation of ferrocene and the establishment of its novel structure in the 1950's really marks the birth of modern organometallic chemistry. Thus, organometallic chemistry is a relatively new field. Following ferrocene's discovery all kinds of different carbon donating groups, not just cyclopentadienyl were examined as possible ligands for transition metal compounds. More elaborate molecules were made, and everyone was fascinated with what was possible. Some of these turned out to have really interesting properties. They carried out transformations on other molecules in catalytic ways, and they gave people new ways of making organic molecules. They were used as catalysts or as stoichiometric reagents to make molecules. They were found to be really important molecules in making new molecules no matter the field. Also, in industry they started to be used to make commodity chemicals like methanol and like acetic acid and perhaps most importantly, polyolefins—that's something I've been very interested in. And so, I've been fascinated by metallocenes much in my career. I started out working on titanocene (the analog of ferrocene with titanium rather than iron) when I was in graduate school. They're still fascinating. It is true that we often tend to start out with something that's simpler and make something more elaborate and find out it's more complicated than we anticipated. Many of the properties are those that you would predict by changing in the way you changed the molecule, but you when make a variation that is not readily understood, then the field grows.
ZIERLER: John, what about the role of stereochemistry? How does that fit into your research?
BERCAW: OK. I think the main place that it's fit in—although we've used stereochemistry to answer other questions in the past—but one place where you really want stereochemistry to be exact is in the structure of a polyolefin called polypropylene. That's been one of the most fascinating things in the polyolefin business. I don't know if you know what that encompasses, but includes materials like polyethylene, (there are more than one type of polyethylene), polypropylene, polystyrenes, and all kinds of polymers that have heteroatoms atoms that replace carbon and hydrogen, elements like oxygen and nitrogen in the polymer structure. Almost all of these polymers—other than polyethylene—have stereochemistry in the structure.
Propylene is what we call a prochiral molecule. There are two stereochemically different faces of the flat propylene molecule that can be used when it approaches the metal atom to increase the size of the polypropylene chain, the polymer group having individual polypropylene units linked together. Depending on which side the propylene approaches from, you get a different absolute configuration at the b-carbon (the one second from the metal) in the polypropylene chain. A propylene molecule has a methyl group and a hydrogen on one of the carbons of a C=C double bond and two hydrogens on the other. If it approaches the [M-CH2CHCH3-polymer] every time from the same face it will make something called isotactic polypropylene. That polymer has high crystallinity. It has a high molecular weight (typically tens of thousands of propylene monomers are linked together). Individual chains coil and interact very well in the lamina of the crystals. All of that leads to mechanical properties that are highly desirable. If it had no preference for which face of propylene it coordinated to [M-CH2CHCH3-polymer], it would make something that's stereorandom that is, as we say, atactic. It's just a gooey mess. You can use it to patch holes in your roof and that's about the best thing you can do with it. Nobody wants to make that kind of polypropylene. Well, maybe not nobody, but most people don't. There's an enormous market for isotactic polypropylene because it has resilience. It has lots of uses because it's tough and it melts at a high temperature, way above the boiling point of water. And yet, you can melt it without decomposition and cool it to a solid polymer; it is a thermoplastic. You can melt it then shoot it into a mold or extrude it at high temperature. When it cools it becomes tough and hard. Although polypropylene isn't particularly hard. It is much tougher than, of course, a goo. Some polymers that you make are not thermoplastic. If you heat them hot enough to melt, they actually decompose. Thermoplastics are highly desirable.
My group had to try to understand why certain kinds of molecular catalysts for making polypropylene from a propylene monomer did so in this stereoregular or stereopreferred way to make isotactic polypropylene. Some were making this worthless form. As part of these studies —to answer the questions—we had to design a molecule that was that was so-called stereopure. It had a chiral center and had ligands that directed groups around the active site, the metal. Often this stereocenter was a zirconium often used as the catalyst for this. We were able to show that it's not just the propylene approaching the chiral metal site. There are some substituents that made the methyl group interact unfavorably if it was pointing up due to crowding with the groups around zirconium, and not interact when in a more favored way—down. That direct interaction of the groups around zirconium with incoming propylene was thought to be the important feature, but less than the stronger interaction we had missed. We and a few other research groups in Italy also knew this from the experiments they did. We designed a molecule that was chiral with known absolute stereochemistry to explore what the most important interactions were. It wasn't both stereo isomers in a racemic mixture. It was just one-handed stuff and none of the mirror image stuff. We changed the group on zirconium that was being added to the propylene monomer from to a polymer chain to hydrogen or methyl. We found out that if it was hydrogen and methyl it was not very stereo selective. It didn't care which face of propylene approached, more or less, a factor of 2:1. When you had a polymer chain growing there was a large group, the rest of the polymer chain, on the last inserted propylene that was going either up or down as propylene came in and the interactions of that methyl group on the incoming propylene with that polymer chain substituent made all the difference. Suddenly it became more than a hundred times preferred one way versus the other.
It got so perfect, it totally astounded physical organic chemists when we did this, because usually you have to have some big difference between the size of the substituents. There was consensus that you had to have some sort of electrostatic interaction on the group, on the incoming molecule that was attracted by electronegativity difference with some part of the ligand substituent of opposite charge to get a large effect. Methyl and hydrogen are not different enough compared to what was thought to be needed. Thus, it was thought that you must also have an electronic interaction that was either attractive or repulsive to get numbers that high. In catalytic propylene polymerization the preference needs to be as high as 99.9% or greater for using one face of the propylene rather than the other in order to get useful isotactic polypropylene. The stereochemistry here is make or break. The desirable properties don't go up linearly with the stereopurity; they get better nonlinearly as the stereopurity goes up. That's a case where I had to learn stereochemistry. I wasn't trained as a physical organic chemist. I hadn't even learned that when I finished college. I had to learn it when I was a professor of chemistry. I have some great colleagues at Caltech that would point me in directions to learn it and I did. [laugh]
ZIERLER: Well, John, that's a great place to end for today and next session we'll take it all the way back to the beginning.
[End of recording]
DAVID ZIERLER: OK. This is David Zierler, Director of the Caltech Heritage Project. It's Wednesday, October 13th, 2021. Once again I'm delighted to be back with Professor John E. Bercaw. John, good to see you again. Thanks for joining me.
JOHN BERCAW: Thank you, David. This is fun so far.
ZIERLER: Alright! John, in our first conversation we took sort of a broad view of your approach to chemistry and teaching and science. Today I want to go all the way back to the beginning for your personal history. Let's start with your parents. Tell me about them.
BERCAW: OK. My parents grew up in a little town north of Cincinnati called Mason, Ohio. I guess it's best known for an amusement park that's near there, Kings Island, but it wasn't there then. This town was very small. They met in high school and got married. I'm the third of four children. I was born in Cincinnati, Ohio, which at the time is where my father worked as a bread delivery person. He worked for a company called Tastee Bread. I think he may also have worked inside making bread. He quickly got into sales and into delivery to grocery. My mother was an only child. My grandfather is one of my heroes. He owned a bank in this Mason. Neither my mother nor my father went to college. My mother went a couple of years to a music conservatory in Cincinnati, and she was very good at playing the piano. She played her whole life, and she taught it part-time also. Mostly she was a housewife. I was, like I said, born in Cincinnati, but I only spent four years there and then we moved to Tuscaloosa, Alabama, because my father had a much better opportunity to be in management at a Sunbeam Bread plant there. Maybe you've never heard of it?
ZIERLER: No. I have, I have.
BERCAW: Do you want to know more about my parents or…what's up?
ZIERLER: Yeah. What were their experiences during the Great Depression and World War II?
BERCAW: Well, they didn't talk that much about it, as I recall. My father did not serve in the military. I think he was in the National Guard, but he had something like broken arches, so he didn't pass the health exam. He was unable to go. They spent the time surviving in the U.S. during World War II. I don't really know of anything terrible that happened to them, but like everybody they dealt with shortages at home and got through it. When we were younger, he wasn't particularly well paid. We were sort of a lower middle class, maybe middle class family. Later, especially in Tuscaloosa , he was very successful, and we had a very comfortable upper middle class sort of existence. He suffered through the Great Depression and World War II, but he came out of it fine.
ZIERLER: John, if not your parents, was there anybody else in your family, in your extended family, who exhibited strength in the sciences?
BERCAW: Yes. The oldest child did, my older brother; I have two sisters, also. One is younger and one is older than I. He was also called Jim like our father. Jim became an electrical engineer. When we moved to Tuscaloosa, Alabama he went to school at the University of Alabama in engineering. He was very successful, and he went first to Bell Labs, and then he worked at Western Electric and eventually became much more in management than in engineering or sciences. When he was influencing me most was when we shared a bedroom in Tuscaloosa. He would regularly get up before dawn to work problem sets and read textbooks to get through his coursework. I think I'd already decided I wanted to be a scientist. Not so much by watching him, but just because I loved it. He encouraged me greatly, because he saw something in me that I guess I had. That was the ability to grasp scientific concepts pretty easily. I remember him lecturing me on how semiconductors worked, and I asked what he thought were good questions. When I was 19 years old he actually gave me a 1963 edition of what's called The Handbook of Chemistry and Physics. I don't know if you've ever seen one of these. It has about 3,000 pages, but it's printed on India paper which is very thin so it is not that cumbersome. It has data for all kinds of properties like the melting points of compounds and lots of engineering parameters about coefficients of expansion, and things like that. I used that repeatedly. I still have it! [laugh] They still publish it, but they abbreviated it over time. I've kept that book he gave me most of my life. I'm still using it to great benefit. That was evidence of how he wanted me to think about being a scientist or an engineer. I thought I wanted to be an engineer when I went to college, but I quickly learned that no, I needed to know the fundamentals of science. Not just fit models to observed phenomena.
ZIERLER: John, after those early years in Ohio, did you spend the rest of your childhood in Tuscaloosa?
BERCAW: No. I only spent eight years there. Even then I was going to the library and checking out chemistry books. [laugh] Before I was 12, I knew I wanted to be a chemist or chemical engineer. I had some romantic idea that I would have a laboratory in a very cold, snowy place because I liked snow for some reason. I dreamt about being in northern Minnesota in my own laboratory doing science. I read chemistry textbooks and biographies of scientists and went to the library and checked all these books out and read them. My mother never ceased to be amazed at the craziness I was doing. When I was in Alabama, I think it was a couple of years before Sputnik was launched, I became interested in becoming a rocketeer.
I did really foolish things in retrospect like making rocket fuel. I also had a book on pyrotechnics. I made my own pyrotechnics. I ordered chemicals from some supply house that would sent me dynamite fuse and all of these components for fireworks like sodium perchlorate and aluminum dust, antimony sulfide and other components too—if you make pyrotechnics you'll recognize them. That's what I was going to do with the items in this order. [laugh] They sent it to me anyway. I had to have my father's signature, because they had to ship it by Railway Express. They couldn't ship it in a truck. I remember asking him, "Would you please sign this order for me?" I guess I had enough money to buy it. I worked part-time during most of my early life in my father's bakery doing various jobs. Anyway, I wanted to order this stuff. He looked at the list, and he couldn't made heads or tails of it because he wasn't a scientist. I said, "Yeah. I want to fool around with some chemicals here. Would you mind signing this? They have to send it by Railway Express." He said, "Oh, alright." He agreed to do it.
ZIERLER: [laugh]
BERCAW: Yeah, I was in Alabama in my beginnings of my love of science. It's amazing because the schooling I received in Alabama was just dreadful. I was in elementary school where I began first grade and went through (I think?) about fifth grade there. It was not good. They did their best to discourage me from trying to learn things. I mean, not purposely, but their whole system was crazy. For example, when I was in the third grade, I guess the principal and some of the teachers got together and decided there were four or five of us in the third grade who were especially talented. They sent us immediately to the fifth grade after we graduated from third grade. We missed fourth grade completely, and we didn't miss very much, because we were able to do fifth grade just fine. But then after the fifth grade they quit treating us differently, and they said, "Well, we can't continue with this program because we don't have the manpower to do it. So, you'll have to stay in fifth grade for another year." [laugh] "All of you." It was amazing that we went through the fifth grade twice and not the fourth grade, but we survived. Then I moved to Greensboro, North Carolina; my father got an even better job there. After I started six grade, I remember thinking, I'm really going have to work my butt off to catch up because it turns out Greensboro—especially at that time, but I think it's still true—has a very good school system. The classes were challenging, and I really had to apply myself. Whereas in Alabama it was a real loss. I was mainly playing sports and things like that with my friends instead of working homework problems, and I was still getting through with A's. Anyway, Greensboro school system was a good thing for me because it slapped me back into reality.
ZIERLER: John, it's notable that as a young kid it wasn't just the chemistry you were interested in. You wanted to learn about the biographies, the chemists who made the science possible. Were there any specific chemists that were heroes to you as a kid? Or people that you looked up to? Or you really admired their approach to chemistry?
BERCAW: I guess it was more all kinds of scientists than just chemists. You know, Einstein and the modern physicists were being accepted. I was interested in that kind of thing, but I also read about explorers so it was more than just scientists. I did read scientific books, and I can't even remember a chemist that I read about. I don't know what it was about chemistry that was so fascinating to me. I just liked the subject. I was, of course, learning a lot about it without having a class in it. I remember when I was in junior high I ordered a glass retort. I don't know if you know what a glass retort is; it's one of these tubes that tapers and on the other end from where it comes out is this ball. It's like a flask that it's attached to. It is the alchemic piece of apparatus that you might've seen in various cartoons or movies. It had a glass stopper on it. I got this glass retort by mail, and I decided I wanted to use it for something really cool. I made bromine, elemental bromine, from potassium bromide and concentrated sulfuric acid. You had to heat those two together to get the sulfuric acid to oxidize the bromide into the elemental Br2.
That stuff is really potent! Its vapors are very heavy from its molecular weight. You collect it in a test tube and put an ice bath around the tube to try to keep the vapor pressure down. I had this experiment going on in my bedroom in the upper floor of the house. All my family were downstairs. Some of the bromine vapors came out of the test tube, and since it was so heavy it just ran along the floor until it got to the stairs, and then it ran down into the living room. My father came upstairs and yelled, "What in the hell are you doing? You're killing us all!" [laugh] I still remember that. He just shook his head. This was typical of what I was doing as a child. Reading about how to make bromine, and then ordering the glassware for it, and somehow I got hold of concentrated sulfuric acid which is now not easy to obtain. Back then you could buy things like that and have them shipped to you, or even in the pharmacy you could find some really cool chemical stuff. Anyway, I was playing with that. I guess I'll finish with a story about my mother. I decided I wanted to follow the recipe for making rocket fuel. I made mostly zinc dust mixed with sulfur which is a relatively easy thing to do. You just mix the zinc dust which is used for galvanizing iron and steel. They melt the dust and dip a piece of steel into it. That puts a zinc coating on it. Zinc dust is plentiful and flowers of sulfur you can buy anywhere. You just mix those two together and if you pack it into a rocket motor it burns and makes zinc sulfide which is solid. It's a very smoky proposition. The thing goes off and you're able to get a rocket motor actually to lift the rocket off the ground.
I wanted to try this other fuel which was made with what's called saltpeter or potassium nitrate and sucrose which is common sugar. You have to melt them I think at like 300 degrees Fahrenheit or maybe 350. I don't remember exactly, but you can't take it higher than about 450 or it'll ignite. Then you take the liquid—because it melted into a liquid—and pour it into the rocket motor. I was thinking, how am I going to make sure it's at 350 and no more degrees? Then I thought, well, put it in the oven. I mixed the components on a cookie sheet and put it in the oven. I was sort of watching, opening the door occasionally. My mother came into the kitchen and she said, "What are you doing there, John?" I said, "I'm making rocket fuel, Mom. I'm melting the sugar and potassium nitrate together." Not surprisingly, she looked very alarmed, and she ran to the phone and called my father. [laugh]
ZIERLER: [laugh]
BERCAW: She said, "Johnny's making rocket fuel in the oven! What do I do?" [laugh] My father—he claims that he said, "Get the hell out of the house!" [laugh]
ZIERLER: [laugh]
BERCAW: She got out of the house until I was finished filling the motor with fuel. It was just an aluminum tube, but it was a foolish thing to use because when I did ignite it, it just melted the aluminum tube and flowed out all over the place. It was a failed experiment, but it was OK because it didn't detonate in the oven.
ZIERLER: Oh, that's good. That's good. [laugh]
BERCAW: Anyway, my mom and my dad were very patient with this kid who was fooling around with things they didn't understand at all. They didn't discourage me. Except the one time I did get CO2 cartridges to use as rocket motors. You know those cartridges that power air rifles, and also you can carbonate water for drinking with them? Anyway, I used to take those and fill ‘em with zinc dust and sulfur and ignite the end and shoot them out of an aluminum tube and they would go like a mortar. I wanted to try a different fuel, so I got a few shot gun shells from my father's closet, cut them open, and filled a CO2 cartridge with the gunpowder. I was showing this "experiment" to my curious little sister out in the driveway with me. The rocket took off after ignition and flailed around in the air. I didn't have any fins or any kind of stabilization of its flight path. Especially if they weren't packed right they wouldn't go very far. This time the one I ignited would just spin around, then it went zooming into the garage right by the two of us. My little sister was standing next to me watching me do this craziness. It hit the wall of the garage and detonated. An enormous explosion went off and my father came out from the house and saw us standing there. The garage was full of smoke. I told him what had happened, and he said, "OK. No more chemistry for you, John. That's it. You're going to kill yourself and I'm not going to be part of that." He took my chemicals away from me for about a year or so. I eventually talked him into letting me try again. Apart from that temporary period where I wasn't allowed to fool around, I did play with chemistry all the way up through high school.
ZIERLER: John, in Tuscaloosa you were a small kid, of course. I'm curious if you have a specific memory if Tuscaloosa was more integrated or segregated when you were there?
BERCAW: It was totally segregated. Yeah. I grew up in that. It was normal to go to the movie theatre and see drinking fountains for colored and also separate ones for white people. Bathrooms were separate. Everybody just sort of accepted the system…that was how life was. That was true also in Greensboro when I first moved there. Although that was a period where the beginnings of the civil rights movement were taking place. I went to college at NC State. One of my best friends in high school, Lawrence Ross—who was also in the accelerated high school classes with me—was my roommate at NC State. He was very much more passionate about civil rights issues than I, but I would go with him sometimes to register Black people in Raleigh and the nearby towns to register and encourage them to vote. They were very receptive to people like me walking up and knocking on their door and asking them to register, and to please vote.
That was a time when segregation was starting to change, but my negative impressions about how black people were being treated also influenced my choice of where to go to graduate school. Almost all of my NC State colleagues who got a bachelor's degree in chemistry either took a job in industry or went to graduate school in the southeast. That's why I decided to go to Michigan thinking it would be much more progressive socially. I applied to several schools in the north and Midwest. At that point I first learned about Caltech, and I thought, "Wow. That's a cool place. There's no way I'd ever get into Caltech. It would be hopeless." I didn't bother to apply, but I got into Michigan which was, especially at that time, one of the best graduate schools in chemistry. My wife and I got married after my sophomore year at NC State, took off for Ann Arbor, Michigan. I was very naïve; I thought, "Well, there's a bunch of bigots and racists in the Southeast. I'm getting outta here." I found out soon after I got to Ann Arbor that it's everywhere. [laugh]
ZIERLER: Yeah. Yeah.
BERCAW: Especially with Ypsilanti being very close to Ann Arbor where were auto workers lived. They were vocal about their racism.
ZIERLER: John, in high school did you want to stay close to home? Was that the primary motivation for going to NC State?
BERCAW: You mean after high school? Yeah.
ZIERLER: Yeah.
BERCAW: Yeah. I guess I did. I thought I wanted to be a chemical engineer. I knew that University of North Carolina had a much better reputation in chemistry, but I thought I wanted to be a chemical engineer. The state school is usually the engineering and agricultural school and that was true in North Carolina as well. So I went to NC State. I started out in chemical engineering, but it didn't matter. It had pretty much the same curriculum as chemistry anyway for the first couple of years. Yeah, I guess I still wanted to stay close to home. I went back on the weekends with my wife, just as we went out before we got married. I would go back occasionally on the weekends just to see my family and friends and to spend a little time at home. I guess I had more familiarity with North Carolina schools. I didn't really apply to very many places after high school except in North Carolina. I think I might've applied to Georgia Tech because I was considering that.
ZIERLER: John, as an undergraduate did you have any labs or summer work that was really formative in your intellectual development?
BERCAW: Well, labs are very much a part of the chemistry curriculum. At least they used to be. Pretty much every subject had a lab. Freshman lab was very many hours a week. I went to those. I thought they were OK. They were mostly analytical chemistry and a little bit of physical chemistry, but early on I really liked the subject of inorganic chemistry. I didn't particularly like organic as an undergraduate. I had a tumultuous sophomore year because my roommate introduced me to a new female student—I told you my roommate, Lawrence, was my best friend in high school. His father and my future wife's father were roommates at North Carolina State. My wife's father was moving his family from Tucson, Arizona to England for a new assignment. I first met my future wife at a party at Lawrence's home near Greensboro, where her family had stopped over on the way to England. The two fathers were still good friends. After a few months in England Diane's father sent her back to NC State where he had been an undergraduate. That was a very unusual decision because at that time NC State was mostly men, and it had just gone coed a few years earlier. There were only a couple hundred women on campus. We started dating, and it was hard for me to keep working really hard in my classes while I was going out to dinner and to movies and things like that with somebody I really cared about. Especially because she was attractive, the football team and the basketball team were always hustling her and trying to get a date with her. They were puzzled by her going out for a beer with me. Walking into a bar full of men was this good looking woman with a nerd. [laugh] They wondered what she saw in me. Anyway, so I had a lot of distraction, I'll say that, in my sophomore year.
ZIERLER: [laugh]
BERCAW: Finally after I didn't do well in organic chemistry, I took her aside and I said, "You know, Diane, we're either going to have to break up or we're going to have to get married because I can't go on like this anymore and be successful at school. And school's very important to me too." She said, "Oh. I'd love to get married." She wanted to drop out of school anyway because she was in education, and she didn't like the curriculum at all. So she quit school and became a bank teller. She ultimately got her degree in accounting at Cal State, LA. I guess I should get back to your question about lab. When I realized that I was very good in lab, and I decided to go ask the lady—her name was Mrs. Manning—who was in charge of the lower the lab courses, the sophomore organic labs, and the freshman labs, whether she needed an assistant. She hired me. I would prepare all the solutions, and I would be the one who would titrate the acids with a base, and then you figure out the unknown molarity. Everybody got an A if they got my number. She had a lot of faith in me and became very fond of me and encouraged me. I was also making up samples for organic lab, even though I hadn't had organic at that point. Diane and I both worked hard because we had to. I also continued to work summers at my father bakery. When we decided to get married my parents were not happy with that decision. They thought that I was going to ruin my career by getting married so early, and that I should stick to studying hard and not be distracted by having a wife. Her father was not very happy that she was getting married either. They more or less—well, they completely did cut us off. "OK, if you're going to get married you're going to support yourselves through college." That's why she started working in the bank and why I started working in the lab. I was making pennies compared to her, but we were able to survive. Those were pretty desperate times when you have to live on whatever you're making in those kinds of jobs. We managed, and we got through, and eventually my parents realized that wasn't a great decision they made on my behalf. They bent over backwards to help me after I graduated with honors from NC State and did well enough to get accepted to graduate in a place like Michigan. I was working in the lab for upper-level courses and I was doing the other labs, structured labs too. I found that doing chemistry was really fun. You know, mixing solutions and looking at precipitation and color changes and things like that. It was something I really enjoyed.
ZIERLER: John, as a married man was the draft something that you did not have to contend with?
BERCAW: That wasn't an issue for me. Somehow I just squeaked by. When they started the draft I didn't have to get a number because I was married. I didn't have any kids, but I was married. Then when I went to Michigan. Diane agreed to have me go to graduate school. I mean, she's being very patient, right? She more or less got me through undergraduate school, now I want to go to graduate school. Graduate school was easier than undergraduate school, because you receive a stipend for bring a teaching assistant and a research assistant. Nonetheless, that decision fueled her father's impression that I was a career student. I was never going to amount to anything. But she agreed. She wanted to start a family. In my first year at Michigan we had my son. I guess about a year later the draft office said, "Well, it doesn't matter if you're married or not, you're still going to be part of the draft unless you have a kid." So I was classified 3A, where it would be a particular hardship for two people or more other people for you to go into the military. I was never part of the draft, but I watched it around me, and it was really terrible what it did to people. You know, here we are in Ann Arbor, Michigan not far from Detroit and not far from Canada. Many people made the tortuous decision to just leave and go to Canada so they wouldn't have to go to Vietnam. But back to NC State: when I was in my first two years, NC State had very strict requirements that you be part of what's called Reserve Officers' Training School. That meant you had to either choose the Army or the Air Force; I guess the Navy wasn't considered possible.
Anyway, I chose the Army. I had to go to an armory on campus and clean an M1 Rifle once a week. I also had to go for dress parade on the athletic field once every week and march around as part of the squad. Diane, she was also an undergraduate at NC State then. She was in the Air Force ROTC, and she had to do that also. She did not have to clean an M1 rifle, though. A few years after we left NC State decided that wasn't a good requirement. We were not a military school and so they did away with that requirement. The whole second year that I was in ROTC they taught me how to fight and what the sort of catchphrases were for people who were in the military. They were trying to talk us into signing up for Advanced ROTC where after graduation you would then. become a Second Lieutenant and go to Vietnam. I was smart enough to know that was not a good idea because I wasn't in love with the military then. I was much more sympathetic to those people who were objecting to the war. I got through it and I resisted going on to Advanced ROTC. That was a good decision, and Diane was happy with that too. Anyway, I watched the war heating up, and Lyndon Johnson taking over for Kennedy. I was a freshman when I recall I was shining my shoes for going out dress parading, and I was listening to the radio and heard about John Kennedy's assassination. When Lyndon Johnson ran for President after that he actually visited Raleigh. I went to see him and shook his hand. When I went to Michigan I found it was very much more conscientiously objecting. Not only about the war, but also the equal rights for Black people and so forth. There were all kinds of demonstrations going on the campus. I remember I was working in the lab and people would march through the halls wielding pipes and trying to get graduate students out of the lab to join the strike. They had all kinds of meetings with faculty and the administration trying to calm us down.
You know, I didn't want to participate in those things at that point, because I could see that I might ultimately want to do something that would require some kind of security clearance. I didn't want to have an arrest on my record at that point, and I figured that I should just keep my head down about this. When I talked to my friends I would be supportive, but they understood that I didn't want to be aconscientious objector. I remember they used to talk to me and say, "John, you're never going to be a real objector. You're just a chemist at heart, and you're going to be a chemist your whole life, so you should keep doing that." They were understanding. There was a lot of that stuff going on, especially at places like Ann Arbor, Boston, Madison, and Berkeley. And I somehow didn't really get it. I didn't get drafted, but my classmates were really nervous about that.
Organometallic Chemistry at Michigan
ZIERLER: John, by the time you got to Ann Arbor how well focused were you in terms of the kind of chemistry you wanted to pursue for your thesis research?
BERCAW: I became pretty focused at NC State. I liked chemistry a lot, and I took the chemistry curriculum. By the way, I did a senior research thesis with a physical chemist on something called Mössbauer spectroscopy, which is used to help analyze certain elements that have metastable excited states that were close to the ground state, so that they undergo a resonance X-ray absorption. It was just coming on board, this new spectroscopy invented by Mössbauer, who got the Nobel Prize for that at Munich. Anyway, I was doing that as a senior thesis. Because I was working with this cobalt source of the X-ray radiation that irradiated the iron centers in minerals, I was fooling around with radioactive things. I thought, "Wow. This is cool." I took more and more courses. I think I took three or four courses in nuclear physics even as an undergraduate, because I was fascinated by nuclear weapons and radioactivity. You know, I liked that kind of physics. I took electricity and magnetism as a freshman at University of Michigan. I almost got killed because I was thrown in there with a bunch of physics majors that were used to all of the advanced calculus that I didn't have in my background at all. I struggled so that I got a C or a B. I don't remember. Anyway, I did like the nuclear physics courses and the structure of the nucleus and all of that. Which is interesting because you may know in my later years I actually went to the weapons labs and served as a consultant for panels. I got tours there of classified things, I never would've seen if I hadn't been accepted as a panel member.
That fascination with nuclear weapons went back to my affection for explosions and rockets and things. I thought, "Wow! That's the ultimate bomb right there. Let's know how it works." I had eventually got around to reading the Rhodes' books on The Making of the Atomic Bomb and then the follow-up, Dark Sun. Anyway, I took these courses as an undergraduate and also did a thesis that used radioactive material. When I decided to go to Michigan, I chose Michigan over a couple of other schools I was accepted to because they had a reactor on campus. I thought I would be doing things like neutron activation of elements which requires a reactor. I guess I didn't tell you that I worked the summer before starting at Michigan at a DuPont laboratory in Savannah River where they make the plutonium for nuclear weapons. They also make the tritium and other radioisotopes in a site called Savannah River Plant. I spent three months in Augusta, Georgia, and I drove over to the lab every weekday. I had to have a top secret clearance for that. It's good that I kept my nose clean when I was going around doing these registering of Black people and marching to some extent. Anyway, I worked with plutonium for three months. Actually I had to go in the lab and handle plutonium-239. That was the sample they had on hand. They didn't work with the less radioactive stuff. Pu-239 is really deadly. You don't want to ingest it. I had to put on these lab coats and hair nets and tape two latex gloves onto my hands and count myself when I went into and out of the lab. Because if a very small amount—well, less than a milligram is ingested—that will be lethal. The health physics people are all over you, especially if you're a recent bachelor's degree graduate. I worked in the analytical lab where they kept track of the plutonium production purities and before they send it out to Colorado to be fabricated into the nuclear weapon.
That also encouraged me to go to Michigan because I had experience now. I'd been accepted at Michigan already, so I guess they were more willing to take me at Savannah River. When I got to Michigan, and I started talking to professors who did radiochemistry research, I soon figured out that all the stuff they were doing was just fancy analytical chemistry. There wasn't any real intellectual frontier that I could see. It was just sort of building methods for detection of this and that and analyzing in great detail all of these things. Analytical chemistry never really appealed to me all that much. I used it, but I didn't think it was a frontier area for me. There was this young guy in my freshman year at Michigan who was an assistant professor from Germany. I took a course from him. He was really exciting when he taught inorganic chemistry. I also took the inorganic laboratory that he taught. After about two or three months of working in the lab he came up to me privately and said, "How would you like to work in my lab this summer? I have an opening and maybe you would like to just try out my lab." I said, "Sure. That would be great." That was the beginning of my turning around completely toward what I ultimately did, organometallic chemistry.
ZIERLER: This is Hans Herbert Brintzinger you're talking about?
BERCAW: That's correct. Yes. I guess you may know that he died about two or three years ago. He was a good friend most of my life. I saw him about a year before he died. He came to spend two months in the summer with me in Pasadena working in my labs doing some experiments he could only do at Caltech where we have the equipment to do them. We published a paper on that work right after he died. Anyway, that was the beginning. I was one of his very first students, maybe the first Ph.D. that he produced; I'm not sure. It could've been somebody else, but I was first or the second that finished with him. We spent lots of time together. I was completely in love with what I was doing.
ZIERLER: John, what was it about Brintzinger's approach or to this new area of chemistry that spoke to you so clearly?
BERCAW: I don't know why I have an affection for somebody or some field. I don't know how to define it well. He was excited, and he was as dedicated as I was. I spent long hours in the lab, even when my wife and our son, David, were home alone while I was working with Hans Brintzinger. After working as a bank teller, she would be at home in university housing with our son, and I would be working. I would go back after dinner. I would work until very late, sometimes I worked all night. I would work 24 to even more hours straight because the compound that I worked with first was very unstable at room temperature, so I had to do the planned experiment right after I made it. I wouldn't sleep for…God, I don't know…36 hours sometimes. Then I'd take the bus back to the housing where we lived in. I would sleep for 12 hours and then I would go back to work in the lab. You can tell my wife is a saint. Hans Brintzinger was equally excited about what we were doing. We would go together to use instrumentation on the north campus that was in some engineering building and run spectra on our samples. He would be in the main campus labs at night with me sometimes, also. His total love for what we were doing was just contagious. I got it even worse than he did. [laugh] I really had a lifelong friend there.
The projects that we worked on during that period did a lot towards getting him promoted. Hans and I also devised techniques for working with compounds that are very, very air-sensitive. Open a sample to the air and it would just spontaneously catch fire because it was so air sensitive. We were lucky at Michigan because there were groups that worked with things like boron hydrides, silyl hydrides, and phosphine hydrides. Although these are main group compounds, not transition metal organometallic compounds, they are also very air-sensitive. They had methodologies that we adapted to what we were working on. We required a lot of glass blowing. I learned how to blow glass myself, and I made some of my own equipment. Organometallic chemistry was thought of as the chemistry of main group element-to carbon compounds with elements with as B, Si, P, and it was as not a very exciting area. After ferrocene transition metal organometallic chemistry took off. That was in the late ‘50s, early ‘60s and I was going to grad school starting out in'67 or '68. We were right there at the beginning and everything was exciting and new. He was working in that area I wanted to be in. He also became a good friend. He and his wife would invite Diane and me to their home for dinner, because I was an unusual student. I had a wife, you know? Then after he got promoted to full professor, he got a chance to return to Germany. He was a German; his wife was Swiss. The school is Konstanz, which is on the Bodensee, which is part of the Rhine River that runs between Switzerland and Germany. He took that job in Germany and left Michigan about two or three years after I finished with him. He would invite me to go to Germany and bring Diane and my family along if I wanted. It was a lifelong friendship, as I said. We were both very dedicated to each other, and he kept working in this area on similar projects to the things I was doing at Caltech. He told me that whenever he would mention my name in his group meetings, the group would sort of chide him and say, "Oh, you mean the Great John Bercaw." [laugh]
ZIERLER: [laugh] John, what were some of the principal conclusions of your thesis? How were they responsive to some of the key questions given that the field was so young at this point?
BERCAW: Well, what we were trying to do is follow up on some work done by a Russian chemist whom I later met, a fantastic guy, Mark Vol'pin. He had published reports of these reagents with titanium that would fix nitrogen, that is take nitrogen gas and convert it into a reduced form that you could hydrolyze to ammonia. Hans had had some interest in bioinorganic chemistry when he was in Germany before he came to Michigan. I think that sort of spurred his interest in these papers and the project. We were trying to make these titanium compounds that would react with dinitrogen. Often you would need to use high nitrogen pressures to get reactions to go fast enough, but we had these high pressure reactors up in this special room in the laboratory. We devised a way to make something called titanocene that's like ferrocene. We made a hydride of titanium that we thought might be a precursor, but we couldn't crystallize it. This was the thing that decomposed so quickly. We would do all kinds of experiments that would prove that it was a titanium hydride using vacuum lines. We concluded that it had an unusual dimeric structure like the boron hydride, B2H6; it formed a diamond in the same way with double hydrogen bridges. That was our first paper. Then we showed that treating this hydride with dinitrogen would form a dinitrogen species that somehow got reduced. We tried to figure out how that worked, but along the way we did all kinds of other chemistry with it. Then I had the idea.
The reason that this compound was so unstable was that it kept reacting with the CH bonds of its cyclopentadienyl ligands and breaking the carbon hydrogen bonds to decompose. I said, "Well, let's just get rid of those hydrogens and put methyl groups here." We had these molecular model kits, and I built a model of one having methylated cyclopentadienyl ("pentamethylcyclopentadienyl, C5(CH3)5") ligands that I later abbreviated Cp* ligands. Hans looked at my model and said, "Don't even bother, John. It will never work because it's too crowded to fit around the titanium with all those methyl groups banging into each other. It just won't stay together." I remember I said, "Watch me." [laugh] I figured out how to make Cp*2TiCl2, and it turned out it was much very stable. Everything having that bulky Cp* ligand was crystalline and beautiful and wonderful. I made Cp*Ti compounds at the end of my thesis work with Hans. I remember when I decided to go off and work with Jack Halpern as a postdoc, I told him I probably would want to be an academic. He said, "Well, why don't you continue working on the Cp* stuff that you insisted on making?" I said, "OK."
The first time I prepared Cp*2TiCl2, I planned the synthesis, and consulted with a very good colleague who was on my thesis committee who made main group compounds that were part of a ring with methyl groups. They weren't cyclopentadienyl ligands, but he had made this precursor in relatively large quantity. I think like 50 milliliters of this precursor to the cyclopentadienide, C5(CH3)5H. I went to see him, and I said, "I'm really excited about making this titanium compound that has these methyl groups all over the cyclopentadienyls, and I understand you have some the cyclopentadienide precursor in your refrigerator." He said, "Yes. I have some." I said, "OK. I'll make you a deal. I'm so excited that I want to make it right away. So, if you give me that 50 milliliters, I'll give you a hundred milliliters when I repeat the prep and make it on a bigger scale." He said, "OK." He was very generous. When I gave him the hundred milliliters later. He said, "You didn't really have to do that." I said, "No. That was the deal." I was able to get going on that project really quickly. His name is Arthur Ashe. He still remembers me coming in his office and selling him on giving me 50 milliliters of that compound.
Anyway, I think we published some really nice work on the Cp*Ti system. People who heard Hans talk were very impressed. My colleague now, Bob Grubbs, who is a couple of years older than I was just starting out at Michigan State then. He said he still remembers Hans coming and giving a seminar at Michigan State on the work that we were doing. He mentioned this young man from the southeastern part of the United States who was working with him and was really very good and mentioned my name. Bob told me about this first meeting with Hans many years later when he became my colleague at Caltech. He said, "Oh, I remember Hans. We used to call him Hans, Hans the Sandwich Man, because he spoke about so many sandwich compounds." [laugh]
ZIERLER: Yeah.
BERCAW: He said he gave a great lecture at Michigan State that everybody was so impressed by him. He certainly taught me a great deal when I was at Michigan.
ZIERLER: John, did you know Jack Halpern before you got to Chicago?
BERCAW: No. [laugh] I was ready to graduate. I'd only been at Michigan for three years and I started thinking about postdocs. I actually finished in three and a half years because I had to get out of there. Diane was ready to move on. She had had enough of this stuff. [laugh] Actually before I went to postdoc, I decided I'd better get a job now. A real job in the chemical industry. I applied to several places. I got an interview at General Electric. They have a chemistry lab in Schenectady, New York. There was only one other interviewee. He was a guy from MIT who had worked for this famous inorganic chemist, Deitmar Seyferth. They hired him, and they didn't hire me, so I didn't have a chance to work in industry. There were no jobs in the early ‘70s for chemists. I've never seen it as bad as it was then. I decided maybe I'd better go on and do a postdoc. I asked Hans Brintzinger, who I should think about going to work for, and he said, "You should go to work for somebody who does something totally different than you. Don't go work for somebody who does synthesis. You know more than anybody I've ever seen about synthesis already. Learn something new."
He recommended that I apply to Roald Hoffmann at Cornell and a couple of other people, like Jack Halpern. Roald Hoffmann was somebody who applied theory to organometallic chemistry. Hans was very taken by that. It was just becoming popular to do that. Roald was very good at it. Even though I had no experience at all in doing theory, I think Roald would've taken me as a postdoc. He told me much later that he still remembered my application. I said, "But I had no experience at all with theory. All I did was experiments." He said, "That's fine. In fact, I prefer people like that because they don't have these biases towards something wrong." [laugh] Anyway, we became good friends, actually. Jack Halpern called me on the phone after I had written him a letter and sent him an application. It was a very funny conversation, because he said, "I have a postdoc opening." I said, "Well, that's good to hear because I've applied for that position." He said, "If I offer you this position, will you take it?" [laugh] I said, "Well, I haven't talked to you before this so it's sort of off the cuff. But, yeah, sure. I'll take it." I took the job. The first time I ever met him I had already accepted the position, and I had driven to the University of Chicago, and finally went in and met him in his office. It wasn't at all like it is now where you have to pretty much go interview at places that you're interested in. When you go to graduate school you have to go visit the schools. Most applicants want to do that so they can make the choice. The schools actually pay for you to go, at least in chemistry, and look ‘em over. They put on a show for you, but I didn't do any of that for even going to Michigan. I just read the brochures and some of the faculty wrote me letters of encouragement to come. Hans Brintzinger wasn't one of ‘em. I told Michigan I was interested in nuclear chemistry. So were most people who wrote me.
I knew of Jack Halpern and his work. He was one of the pioneers of organometallic chemistry, and he did mechanistic studies. He studied how reactions occur doing a lot of kinetics and labeling studies. He developed analogies between the mechanisms of reactions of organotransiion metal compounds and well known organic mechanisms—he and Roald Hoffmann, and a guy named Jim Collman had proposed general principles that they were espousing. He was a very famous guy. I read Jack Halpern's papers very carefully. I knew I wanted to do mechanism, but when I got to work for Jack that first day, I said, "What kinds of projects are you interested in having me think about?" He told me about a project. He said, "We've been trying to make this compound and isolate it in a pure form for three years. We've been unsuccessful. I understand from your letters that you're very good at synthesis. I want you to go in the lab and make this compound and crystallize it." [laugh] I was sort of crestfallen, but I didn't show it—I don't think. I said to myself, "Well, I'd better do what he says to start with." I soon figured out I had to actually make some new equipment in order to handle this compound.
It was very, very sensitive. That's why people were having so much trouble with it. I made the needed equipment, while Jack's students and postdocs were looking at me asking, "What is that that you're making?" "Well, I have to have this equipment called a Schlenk line in order to work with this compound because it's very sensitive." "Wow. OK." I made a Schlenk line, made the compound, and finally got it to crystallize. The first time was really hard. After that it crystallized easily every time for me. It's very sensitive, so it makes no sense. I still don't understand that today. Some people claim that there are seed crystals that get in the glassware you're working with so that the next time you try to crystallize a compound those seed crystals make it easier to crystallize later. For example, Fischer made crystals of sugars, and he had to have special glassware that he thought retained very small amounts of seed crystals on the walls. He is a famous example of that. When he moved labs, he had to move all of his glassware to the new lab to get things to work again. That couldn't have been true of my compound because it reacts with air, so the seed crystals would be decomposed when I washed the glassware.
Anyway, I made this compound and got it isolated. Meanwhile I was watching the group and going to group meetings. Then I decided without Jack telling me, OK, I'm going to study some mechanism for this compound. For some reason I started oxidizing it with ferricyanide. It was a beautifully clean reaction. I started following it by the color changes and isolating the products. The compound turned out to be a catalyst for oxidation of carbon monoxide to carbon dioxide by ferricyanide in water.
Jack Halpern was a very interesting guy. He was very, very famous, very busy. He travelled a lot, but when he came back he would go down to the lab which was laid out linearly. He would go from blackboard to blackboard, right next to where the postdocs or the students were sitting. He came to the my blackboard, and he said, "Well, John, how are things going?" I said, "They're going great, Jack." He was silent. He didn't say anything. He just looked at me. I kept looking at him, and then he walked on to the next blackboard. I realized, wait a minute. I was supposed to say a lot more than, "They're going great, Jack." I was supposed to explain why they're going great. The next month when he came, I was ready. He was just fascinated. He stayed with me for at least an hour and told me a lot of things about how I that could do things better if I tried this instead of that. He was very supportive of me doing this work independently. He thought it was reasonably important. I've always been very talkative, interested in group meetings and asked questions. So I would be a vocal participant in the group meetings that we had. Too vocal, I guess because the other postdocs who were mostly from Europe and Asia took me aside after a group meeting and said, "John, in group meeting Jack talks. You don't talk. You shut up." [laugh]
ZIERLER: [laugh]
BERCAW: I just shook my head, and I said, "I'm sorry if I'm annoying you guys, and I'm not trying to embarrass you by asking a question. I'm truly interested in what you're doing and trying to clarify it." They just thought I was a pain in the ass. I said, "I'm trying to learn something here, and Jack has got a lot to teach us all. So, you should be asking questions too." They just gave up on me. Some others seemed to think I was a nice guy, and I wasn't being mean to people.
From Chicago to Caltech
ZIERLER: John, would you say that your experience at Chicago sealed the deal that you would pursue a career in academia and not go into industry?
BERCAW: Yeah. I would say that. Yeah. I think Hans Brintzinger really got me into the field that I pursued ultimately. Jack was, he was a tough critic. If he thought that I should try academics, that meant a lot to me. People were afraid of him in meetings. He was so quick and was pretty aggressive in the way he asked questions. When he wrote letters for people—I didn't really read my letter—people took notice when he was complimentary. When I said I was thinking about interviewing in academics for a faculty position, he was supportive. That was a pretty whirlwind affair, because again there were still not a lot of jobs, and especially at that time not a lot of positions in academics. I applied to about three or four schools including Caltech even though the job wasn't a real tenure track position. It was called the Noyes Research Fellow, and it was supposed to be a two or three year appointment and paid from an endowment to honor Arthur Amos Noyes. A lot of people at Caltech had started on something called the Noyes Instructor, but this was a new position they called a Fellow. I think it was sort of modeled after the Harvard…they have these Harvard Fellows. I don't know if you've heard of them?
ZIERLER: Sure.
BERCAW: They're sort of independent, but they're not really on the tenure track to be an assistant professor. Many times, especially at Harvard in chemistry, the Fellow didn't ultimately end up at Harvard. They went somewhere else. It wasn't that they didn't get accepted as a faculty member at Harvard—a real faculty member—but they had to move on because it was known that most people didn't stay at Harvard. It was an OK position. I interviewed for this position at Caltech on a Thursday. On Monday Harry Gray called me with an offer. He explained to me very carefully that, "You have to understand, this is not a tenure track position. It's not like the Noyes Instructors, many of whom went on to become famous chemists at Caltech. This is different. You're going to have to leave after three years. This is just a temporary position." I really was taken by Caltech. I'd never met Jack Roberts. I didn't even know who he was. [laugh] I didn't know any of the inorganic chemists before I interviewed. I remember asking Jack Halpern when I got an interview. I said, "So, who should I talk to when I go? They're asking me who I might want to see." He said, "Oh, you should definitely talk to Harry Gray. He's a delightful fellow." [laugh] I loved the way he said it because that's what Harry is. Dead on, he's a delightful fellow. Anyway, I hadn't met Harry; I hadn't met Bob Bergman who became my lifelong friend. I hadn't met any of the people I spoke with. I was talking to Jack Roberts, and he said—I don't know if you were fortunate enough to know Jack before he passed on, but he was a very rough sounding guy, but he was a real teddy bear. [He asked me in an exaggerated voice] "Well, you made titanocene, you say?" I said, "Yeah. We did, Jack." He said, "Oh. I remember when ferrocene was made. I named ferrocene." [laugh]
ZIERLER: [laugh]
BERCAW: I said, "Wow! Alright." Then I looked around his office, and there was this whole series of books on a shelf: The Life of John D. Roberts—several volumes. I thought, man, these guys are important. Anyway, I gave my seminar that was different most of the seminars given at Caltech. Harry's done some synthesis especially in later years, but he was a very famous physical inorganic chemist and the physical chemists ran the department. There were very few inorganic chemists. I think two or three at the time. All the synthesis was being done in the organic chemists' laboratories. When I interviewed, Harry and Bob Bergman were sitting in the back row. Bob told me later that Harry leaned over halfway through my talk and said, "This guy can make compounds." [laugh] Bob said, "Yeah. We need that." [laugh] They liked me for that reason. Anyway, when I went to Caltech to do my science at Noyes Laboratory taking over a lab that was used previously by George Hammond. He was chairman right as I was arriving, and he soon left and went up to Santa Cruz. I was making compounds. I had to go down to the stockroom to get some glassware. They didn't have anything called a three neck round bottom flask, a commonly used flask for synthetic chemistry. I had to go over to the stockroom in the organic labs to get my equipment.
I remember Bob Ireland who was a senior organic chemist at Caltech at that time doing synthesis. He said to me early on, "I love you, John. You're a synthetic chemist. You know how to make molecules." He was also from Michigan. He was at U of M before he came to Caltech. He liked me for that reason too, but he was always a good friend, and supported the fact that I was a synthetic chemist. That was my opening and after a couple of years of building my equipment and doing some experiments that were impressive; I actually made a dinitrogen complex of titanium that was crystalline, and I got a structure of it by X-ray methods. That was so much fun, those experiments. After another year John Baldeschweiler, chairman of the Division at the time came to my office and said, "Alright, we're going to make you an assistant professor." [laugh] Then I was off and running, even though they told me that I would only have two or three years to be at Caltech and I should already be planning where I was going after that.
ZIERLER: John, from those initial impressions, besides the individuals that you interacted with, what did you find special about Caltech generally? Beyond the few people that you talked with before you made this decision to join?
BERCAW: Well, remember I had less than three days to start thinking about joining. [laugh] I guess I got back on Saturday or something. It doesn't matter. I guess I remember walking around campus. I was put up in a room at the The Athenaeum…the old Athenaeum where a room was like a YMCA room. It had a bed, and a telephone, and a desk. That was it! [laugh] You could barely move around in that room. Anyway, they put me up in this room, and I thought, boy, people come here to work. Right away, just from the room at The Ath, I thought, man, they're serious. Since I didn't know anything about California—I'd never been to California; I'd never been to Pasadena. Never been to Caltech. I decided to take a walk that evening. I walked up the Olive Walk, and I walked around looking at some of the buildings that had lights on in the windows. I saw people working at night. I just got the feeling this was a place people were driven. They loved what they were doing. Gee, maybe I'll get a job here. That would be good. I liked that. You know, I liked the people that I talked to during the interview. I liked the students and postdocs because they were like I was at Chicago. They were asking questions, and they were excited, and they were obviously very bright. I was right that Caltech was a place I would never have been accepted to as an undergrad. [laugh] I thought, wow, I may be lucky. I don't have to leave like an undergrad would! [laugh]
ZIERLER: [laugh]
BERCAW: I felt like it was a great place for me to start my career. Everybody was just so supportive in everything I did. I was right. My wife, Diane, I told you she grew up in Tucson. She was born in Colorado, but her father was in the Air Force. He was based at Davis-Monthan Air Force Base in Tucson, Arizona for like 10 or 15 years, which is unheard of. I mean, they rotate out of a place in the military pretty quickly, but for whatever reason he was there for a long time, so she went to high school there. She was just in love with the West. I got tired of hearing about the West, "it's so great". Maybe I ought to go see it sometime. When I had an offer at Caltech she said, "Take it."
ZIERLER: [laugh]
BERCAW: [laugh] She wanted to go.
ZIERLER: John, was the situation any different if you had joined as an assistant faculty, tenure track, in terms of setting up the lab, in terms of your interactions with students, teaching responsibilities? Besides the term, the soft money basis for this initial position, was your research in teaching life any different than it would've been if you had joined as a regular tenure track faculty?
BERCAW: That was pretty confusing to me. I didn't know what I had to do. I knew I didn't have any responsibilities to teach a class. I was just supposed to do my science and not be bothered with the committee assignments, and teaching. It was funny because they let me talk to the first year graduate students. My very first student was a fellow from Chile who is spectacular. He told the people that he wanted to join my group, and they let him. Even though I said, "You know, I'm not sure I'm going to be here after three years." His name is Juan Manuel Manriquez. Juan said, "I don't care. I like what you're doing and I want to do that." He and I were like Hans and I working in the lab all the time. I was working with him, showing him everything I knew. He became a better synthetic chemist than I am. He is amazing. Anyway, they let me have a grad student. I remember I needed a glovebox. A commercial glovebox which you have filled with helium or more commonly nitrogen gas to keep air and water away from your compounds. I went to John Baldeschweiler who was the chairman of the division at the time and I said, "I have a need for this glovebox." He said, "How much does it cost?" I told him and he said, "OK. Buy it." With my position I had some expenses for small equipment and chemicals and other things. But a glovebox was a big purchase at the time. Fifteen thousand dollars was my annual salary. [laugh] He didn't blink. He just said, "Yeah, OK. Buy it."
They were acting like I was a regular faculty. They expected me to do really well. Even though they were expecting to get rid of me in three years. They wanted me to do well so I could be like a Harvard Fellow and go get a good job somewhere else. I guess I had a break because, although nowadays when we hire a new faculty member, certainly in chemistry, we don't expect them to teach a full teaching load for the first year. We give ‘em maybe half or even give them a whole year off to get their research operation in place and get it running before they start teaching—especially a large class. I really liked teaching as a teaching fellow at Michigan. I liked doing that. I'll tell you a funny story, just an aside. When I went to Michigan I had a very strong southern accent. [in southern accent] "I didn't go ta Chappa Hee'll." You know? "I went to Rahlee." I was speaking like that. I can still do it when I go back there. I still have a twang. At Michigan they have first year chemistry broken up into different tracks, and all the engineers take this one class that I was teaching a recitation section as a graduate student. When I went to my first teaching session as a teaching assistant in chemistry, the first chemistry recitation, there were like 30 of these people sitting in my first session. I walked in and I said [with southern accent], "Hey ya'll! Ima be yer TA." [laugh]
ZIERLER: [laugh]
BERCAW: They all looked at each other, and some of them started laughing. I soon realized I'm not going to have any credibility unless I get rid of most of this accent. I worked very hard in the next couple of months to get rid of my southern accent. People after that didn't really believe I was from the Southeast. They asked me where I met my wife (who was from Tucson) came from because she had a southern accent (from being with me). Anyway, I like teaching. That's when my son was born, and the students from my second freshman chemistry section came with knit booties and things like that for my baby boy that had just arrived in University of Michigan hospital. They all came to see Diane, too. I guess I had a good rapport with students. I enjoyed it, but I didn't have that opportunity as a Noyes Fellow. I did have the chance to go to other people's classes. I remember sitting in on Aaron Kupperman's class, sitting in on Bill Goddard's class, Harry Gray's class, some of the organic chemistry classes. Just to see how people taught at Caltech because I was an outsider. You would think that these students should know everything. [laugh] They're bright, even brilliant, but I didn't realize as a beginner that they don't know things they haven't been exposed to. You have to slow down and teach them in a sort of normal curriculum way. You can't just sort of say, "Why don't we go right to the advanced concepts?" I had some things to learn about teaching at Caltech, but everybody that comes does. They're initially intimidated by the high average intellect of the students. Anyway, I was treated as the Noyes Research Fellow pretty much like a faculty member except for the teaching and the faculty committee assignments. I didn't do those either. I just had to do my chemistry. Which I guess is a romantic situation in a way.
ZIERLER: John, last question for today. As you were developing your expertise relative to your new colleagues, relative to the exciting things that were happening in chemistry at Caltech, what did you see as your niche in terms of the projects to work on and where you slotted in in the overall research effort of the faculty at that time?
BERCAW: Well, I don't know. I've sort of hinted at it already. They didn't have a synthetic effort in inorganic chemistry at Caltech until I arrived. There were people making things, but they were just following recipes other people created and published. I was in devising synthetic methodology and making compounds that had never been made before. In inorganic chemistry that was new. I was also asking questions about mechanism. I had a mechanistic ability too. I collaborated right away with Bob Bergman on a new area. In fact, we shared graduate students for a while. He was a very famous physical organic chemist, but at that time he didn't know much about working with air sensitive compounds and working with organometallic compounds.
He was curious about it because physical organic chemistry was becoming a mature subject, as they say. It was just a bad word. "Your field is mature." It means it's had a number of opportunities for discovering fundamentals and there are not a lot more to discover. Bob was looking for a new area, and he soon went on to become a very accomplished and famous and impactful organometallic chemist. He started working with me and sharing students, and even his new postdoc had come over and worked with these cobalt compounds on my vacuum lines too. I was teaching my colleagues how to do this organometallic stuff that some of them ultimately switched to and became famous, like Bob Bergman. I guess I was teaching my colleagues, and they were teaching me a lot more. [laugh]
ZIERLER: Well, John, that's a great place to pick up for next time.
[End of Recording]
DAVID ZIERLER: OK. This is David Zierler, Director of the Caltech Heritage Project. It's Tuesday, October 19th, 2021. Once again it's my great pleasure to be back with Professor John E. Bercaw. John, great to see you again. Thanks for joining me.
JOHN BERCAW: My pleasure. I'm enjoying this.
ZIERLER: John, to go back to the time during your fellowship in the early 1970s and to the extent that you got the message early in that position not to get too comfortable. This was not a tenure-line position. At what point during that fellowship did that narrative begin to change? And what was some of the research that you were doing that surely drove you toward the tenure line offer?
BERCAW: OK. I'm not sure I can answer the first question accurately. It sort of gradually evolved when my colleagues were listening informally to what I was doing. Then they even asked me to give a lecture as part of a Caltech symposium. I've forgotten exactly what the purpose of this symposium was, but I think it was for what we called Industrial Associates, where in our division we had a lot of industrial support that was discretionary more or less to the Chairman to distribute among the faculty. In return, we were supposed to talk to representatives from those companies that were members. In any case, I gave one of those lectures and people were very impressed. I think I only had like a half an hour, and I was talking about what I had done in the first year or two. I remember Bob Bergman introduced me and said, "In a very short period he has established a laboratory that contains some of the most sophisticated glassware I've ever seen. And he's done some amazing things with it." I thought, "Wow. That's nice of you, Bob." [laugh] I was getting little hints like that. The research involved studies of dinitrogen complexes of these metallocenes of group 4 elements. We were working—we meaning, I guess it was solely me for about a year and a half—I was working with titanium complexes. I managed to crystallize the titanium complex that had a dinitrogen ligand coordinated to it and you could reversibly pump the dinitrogen off of it, put fresh dinitrogen on it, and it would reform and so forth. It actually crystallized. One of the beautiful things about my pentamethylcyclopentadienyl ligand (Cp*)—I think I told you about Brintzinger's skepticism that I could ever make a metallocene having two of those, it would be too crowded—is that it was often observed that when you tried to crystallize something, even if it wasn't pure, you could get crystals, and you would get different colored crystals if there was more than one component there. These compounds had for whatever reason a real propensity to form beautiful crystals that you could then X-ray and establish the molecular structure. I had managed to do that even before my first student joined while I was the Noyes Research Fellow. This student, Juan Manríquez. was obsessed like I was with working in the lab, so much that his wife eventually left him. I wasn't so unfortunate as he. [laugh] I asked her why she was leaving him, and she said, "John, chemistry is his first love and his second love." She kept going down until she got to ninth. She said, "Then I think he loves me." "And I can't stand it. I have to leave."
ZIERLER: [laugh]
BERCAW: [laugh] He started working on some zirconium complexes that were analogs of my titanium complex—titanium and zirconium, in the first and the second row, respectively, of the transition series—are expected to have similar chemistry, although zirconium being bigger in radius can accommodate more ligands around it. Thus, we anticipated there might be some differences. Sure enough, when we made these pentamethylcyclopentadienyl zirconium analogs of the titanium compounds, they made different kinds of complexes, but both made dinitrogen complexes. I had found that the titanium compound made a dimer Cp*2Ti(µ-N2)TiCp*2 with a bridging dinitrogen (µ-N2). For zirconium it turned out two different complexes with dinitrogen were observed. One had two dinitrogens on the zirconium, Cp*2Zr(N2)2, and one had a bridging dinitrogen, thus it was also dimer, i.e. having two zirconiums Cp*2(N2)Zr (µ-N2)Zr(N2)Cp*2. Each of the two zirconiums had also its own so-called terminal dinitrogen. The dimeric zirconium compound readily crystallized, so we were able to readily isolate pure samples of it. We were making and isolating these really amazing compounds, and our hope was that we would be able to promote the reduction of the dinitrogen ligand. Dinitrogen complexes were a novelty in the ‘60s before I started working on them. There was the feeling that dinitrogen would just be normally chemically inert. I mean, it makes up about 80% of our atmosphere and it mostly doesn't react. The rest of it is mostly dioxygen. Dinitrogen doesn't react with most reductants, except metallic lithium metal, and metallic magnesium. Actually binding something so tightly to a metal that you could actually isolate a complex with it was considered novel about 10 years or so before I started looking for my early transition metal analogs. Most of the dinitrogen complexes had metal centers of the so-called later transition metals, those are on the right side of the transition metals in the Periodic Table. When chemists tried to reduce the dinitrogen ligand on cobalt or on ruthenium in these complexes all that happened was the dinitrogen fell off the metal. It never came off as the stable reduced form of nitrogen like hydrazine or ammonia as was desired. We were hoping that these lower valence Cp*2Ti(II) and Cp*2Zr(II) complexes being more reducing might promote the true reduction of the dinitrogen ligand. We began adding things like hydrogen chloride gas to the solutions of these dinitrogen complexes. Right away we would see, even at low temperatures— minus 80 degrees Celsius—it would immediately react with hydrogen chloride and cleanly make dichloride of titanium or zirconium, Cp*2TiCl2 or Cp*2ZrCl2. Thus, the Ti or Zr was being oxidized from divalent to teravalent, meaning that something must have been reduced, most likely H+ to H2 or N2 to N2H4 (hydrazine) or NH3 (ammonia). We were doing these experiments on a vacuum line that I had built when I first started at Caltech. This was what Bob Bergman was referring to. It had a Toepler pump, a really cool feature used to collect gases that were evolved in reactions. We had added the hydrogen chloride on the vacuum line by condensing in the gaseous HCl into a solution of the zirconium or titanium complex. After the reaction we could separate the excess hydrogen chloride and solvent vapors from more volatile gases like dihydrogen or dinitrogen using liquid nitrogen cooled traps and collect the dinitrogen or the dihydrogen using the Toepler pump. Because I also had an added feature to my Toepler pump: a tube having heated copper oxide. If gases are passed through this tube in a circular fashion using the Toepler pump to move the gases, heated copper oxide reacts with hydrogen to make water (which is trapped out cryogenically), and would not react with dinitrogen.
Thus, we could establish if H2 was formed along with N2, and we could quantify each of them. The normal thing that is reduced in these low valent reactions is the hydrogen chloride, so you make a metal dichloride and a H2 molecule. Thus, we were very anxious to see whether we got any dihydrogen, if any, and how much dinitrogen we got back; all three equivalents of N2 for each equivalent of Cp*2(N2)Zr (µ.-N2)Zr(N2)Cp*2 would indicate no dinitrogen got reduced, less would suggest some reduced nitrogen compound was generated. We got essentially no dihydrogen. We got dinitrogen but it was less: two of the three we had started with. Juan and I quickly deduced that (1) we had four reducing equivalents electrons available, two for each zirconium of the dimer, and (2) one of the three N2 was missing. We were hoping that we would make hydrazine stoichiometrically which is the four electron reduction product of dinitrogen. That would mean that we would release two of the three dinitrogens, but not all three. Indeed, we got very close to two and no dihydrogen. We were then very excited, Juan Manríquez and I. We said, "Wow! Maybe we've made hydrazine, and we've protonated the dinitrogen for the first case in a well-defined precursor to make a reduced form of dinitrogen, namely hydrazine." The excess hydrogen chloride had converted the hydrazine to its hydrochloride. Indeed, we were able to show using quantitative analysis that one equivalent of hydrazine had been generated. I even isolated enough of the hydrochloride to measure melting temperature which is a very ancient way in which you characterize something; it has a well-defined melting temperature. Indeed, we had made hydrazine and that was one of our first papers.
We entitled it Stoichiometric Reduction of Dinitrogen. That is four electrons from two zirconiums produces hydrazine in essentially 100% yield. It follows the stoichiometry one would write in a chemical reaction of that kind. It would be the dimeric zirconium complex which had 3-dinitrogens plus 4 HCls gives 2-zirconium dichlorides plus 2-dinitrogens plus 1 hydrazine. We realized that stoichiometry. We wrote a paper up for the Journal of the American Chemical Society. It was accepted right away. I should note that almost simultaneously team of chemists, led by Joseph Chatt and Jeffrey Leigh in United Kingdom were reporting that tungsten or molybdenum dinitrogen complexes yielded ammonia and/or hydrazine when treated with sulfuric acid in methanol, although the metal complexes generated in these reactions was not characterized. Everybody started to take note; "who is this young guy at Caltech?" That was good. [laugh]
ZIERLER: John, just to zoom out for a second. Is that to say, obviously, you were turning heads at Caltech because you were doing quite innovative research, but in the broader world of organometallic chemistry what you were doing was innovative not just at Caltech but in the entire field?
BERCAW: Maybe that's a little flowery, but it was having impact outside of Caltech as well as inside Caltech. Here is an example of how collaborative Caltech can be. In fact, when I was working with the titanium complex Cp*2Ti(µ-N2)TiCp*2, and since I could make them from N2, it was very easy to take isotopically labeled dinitrogen; you can purchase 15N dinitrogen and make the N15 labeled titanium dimer. Nitrogen's most common isotope is 14N; 15N is interesting because it has a nuclear spin of one half, and that means it's potentially a relatively easy nucleus to detect by NMR spectroscopy. I wanted to characterize these complexes in any way I could including the crystal structure, but also its solution structure.
At the time we didn't have an NMR spectrometer that would do 15N NMR. I had a colleague, Jack Roberts, who purchased this amazing Bruker 400-megahertz NMR spectrometer. Jack wanted to examine the NMR properties of 15N for organic compounds and also for metal dinitrogen complexes, and the Bruker instrument was expected to make these experiments possible. Even before the new spectrometer was operable, I spoke with Jack about my desire to look at my dinitrogen complexes, and he was very agreeable. Jack was friendly with everybody, and he invited Diane and me to a dinner at his home that included guests from Caltech. It turned out that the Bruker NMR technician showed up. Jack had invited him as well. The guy showed up to install the spectrometer and to test it out and make sure it worked properly. I was having a beer before dinner. I introduced myself and I said, "Oh, you're installing the spectrometer." He said, "Yes. I've pretty much got it done." I said, "How would you like to run a sample of mine just to see if it works?" [laugh] He said, "Sure. I'd be happy to run your sample." I didn't even tell Jack! We ran the sample and got beautiful spectra. They fit the X-ray structures that we had later.
I actually got these 15N1 spectra before I had the X-ray structure of the compound. Later on we were able to interpret them beautifully. Jack and I published Jack's communication in Journal of the American Chemical Society. Although at that time we thought that we had only one complex, the one that was used to make the sample; however, because these complexes easily dissociates N2 and associates N2 (the sample also had excess 15N2 over the solution), three titanium complexes formed in detectable concentrations: Cp*2Ti(µ-N2)TiCp*2 (the compound we added), and Cp*2(N2)Ti(µ-N2)Ti(N2Cp*2 and Cp*2Ti(N2)2, that had formed by reactions with excess N2 in the NMR tube. It turned out to be the worst choice because we did not know there were three complexes when we were interpreting the first spectra, and we actually misinterpreted it in that paper with Jack Roberts. We later corrected all of that because the zirconium complex gives the expected spectrum for the single complex for Cp*2(N2)Zr(µ-N2)Zr(N2)Cp*2 when were able to run it later on Jack's spectrometer. Jack encouraged me to come over and run my sample, but I sort of nudged my way onto the spectrometer he had on the line before he ever ran any of his samples. [laugh] Jack was fine with that.
Later on, I worked with Sunney Chan, also my colleague—he's now also emeritus at Caltech. Sunney Chan encouraged me to obtain very careful variable temperature proton NMR spectra for Cp*2(N2)Zr(µ-N2)Zr(N2)Cp*2 with him using his spectrometer. We interpreted all those on the basis of a dynamic process that interconverted so that the magnetic environments for the two kinds of pentamethylcyclopentadienyl ligands: dissociation of a terminal N2 and reassociation after inversion at Zr. Those experiments hinted that we might be able to make a specifically labeled complex. I think this is one of the experiments that I'm most proud of. We were working with a zirconium compound at that time. We made the zirconium complex Cp*2(15N2)Zr(µ-15N2)Zr(15N2)Cp*2 from Cp*2(N2)Zr(µ-N2)Zr(N2)Cp*2 and 15N2 by exchange on the vacuum line. Now according to the mechanism we interpreted from the proton NMR data that we did with Sunney, the reason the cyclopentadienyls were interconverting was that the terminal dinitrogens were dissociating reversibly. The bridging one between the two zirconiums stayed put.
There was this process that was very fast at low temperature that involved exchange only of the terminal dinitrogens leaving the bridging dinitrogen intact. Our hypothesis was, "If treat our fully 15N labeled dinitrogen complex with excess normal 14N labeled N2 we will exchange off for the two 15N terminal dinitrogens with 14N2 and the 15N2, the middle (µ-15N2) will remain labeled." We asked the question, "Which kind of N2 is going to be reduced? Is it going to be one of the two terminal ones or is it going to be the bridging one?" Everybody bet on the bridging one, including me. I would've bet a fortune on it, because I told you we had to have four electrons. We had to have two from each of the two zirconiums in order for the hydrazine to form. What better one to reduce than the one that is between the two reducing zirconiums?
We did this experiment and we collected the N2 that was evolved and we analyzed isotopic composition of the hydrazine that was formed. What we found was indeed, the bridging dinitrogen was reduced, but it was reduced exactly half of the time, not all of the time. That was a real surprise to me, of course, but to other people, I think, when they read this paper. It wasn't just random because there were two terminal N2's and one bridging one, so if it was random we should've gotten one-third of the hydrazine, not one half of the hydrazine 15N labeled. But we got very close to exactly half. We did it with these Toepler pump techniques. You can be very precise in what you are saying, if you get a stochiometric reaction, which is what we had. That forced us to start thinking about how this might happen. Although this is very hard to establish every step in the sequence going from N2 to hydrazine, we believe what happens is that there is an initial protonation of a terminal N2, because they're more basic. There is still a lone pair on one of the two nitrogen atoms that are terminal; the bridging one has no such lone pair. Both of lone pairs are tied up with the two zirconiums. We thought well, if it's going to protonate it will go for the lone pair on dinitrogen that is terminal. That protonation is proposed to trigger a reaction whereby the electrons from the zirconium that had the protonated terminal dinitrogen would take two electrons from the other zirconium. All four electrons now end up in this broken up dimer that has all four electrons in one zirconium and a positively charged zirconium complex on the other side, but it's already Zr(IV) because it gave its two electrons to the other zirconium. It thus loses its N2 ligand, its terminal one.
Now the bridging dinitrogen and one of the two terminal N2's are contained on the Zr that has all four electrons, and if continues toward hydrazine and another N2 and those two are equivalent—at least on the reaction timescale—you would get precisely half labeled and half unlabeled hydrazine. That finding was of considerable interest, because there were other people that were wondering how you would go about reducing dinitrogen; would one metal be sufficient, would you need two or more metals? By the way, the UK team was using W or Mo complexes having 4 phosphine ligands and 2 dinitrogen ligands. It is curious that both classes of compounds that undergo protonation are bis(dinitrogen) complexes. It is not clear what, if anything, this means?
Industrial Applications
May I take an aside here and go back? Why would anybody want to reduce dinitrogen? The reasons are in the current way that we reduce dinitrogen on a massive scale in industry to make ammonia for fertilizer and other nitrogen containing compounds. We take dihydrogen (made from methane) and dinitrogen (from the atmosphere) and we mix them together and promote with a solid iron catalyst their reaction to form ammonia: if you write a balanced reaction it will be 1 N2 plus 3 H2's go to 2 NH3 (ammonia) molecules. That reaction is carried out on a very large scale, but it's imperceptibly slow. Even though it's slightly downhill. thermodynamically, the barrier for carrying out that reaction is enormous. You have to heat the reagents to very high temperature, hundreds of degrees Celsius, and you have to use a catalyst. It was a so-called heterogenous catalyst. That means it's not in the gas phase; in this case it's a solid phase catalyst that you pass the gases over. It consists of iron and it has promoters that were found by Edisonian discoveries that led to this. It's called a Haber-Bosch process. The catalyst was discovered in Germany and these guys (Haber and Bosch) got the Nobel Prize for that. It was such an important discovery, because now you can make synthetic ammonia from dinitrogen and dihydrogen and not rely solely on the biological processes like the nitrogenases perform.
In nature this process is carried out by a very elaborate enzyme. I think you've spoken with Doug Rees about this. He's one of the people who discovered the structure of one of these enzymes that does this. It's still of great interest. A lot of the features of it are still not clear, but Doug is an amazing guy. He was able to crystallize it and establish its crystal structure. Doing it synthetically was a breakthrough. You might say, "Well, why do you need a better catalyst than that?" The reason is because if you heat these gases together at 400 degrees even like you have to with this iron promoted catalyst, you get the reaction actually to proceed but the thermodynamics turn around and go back toward the reagents and not toward the product. Because you're taking four molecules, one N2 and 3 H2 to two NH3 molecules, then the entropy of the reaction is such that if you raise the temperature the overall free energy of the process and therefore the favorability of it, it goes back toward reagents and not toward products. You can overcome the unfavorable entropy term by raising the pressure, and because four molecules are going to two by raising the pressure you drive the system toward products, which is what you have to do. But it's not just raising the pressure by a factor of 2 or 3 or 10. You have to take it up to by a factor of several thousand, which requires very massive reactor walls that can withstand that pressure and not explode. The engineers figured out how to do that. These ammonia plants were built to do that, but they had to make an enormous infrastructure investment in order to start realizing profits from making synthetic ammonia. The other problem was that dihydrogen is not easy to come by. You have to make it mostly from natural gas by heating it with steam. That makes dihydrogen and carbon dioxide. Then you have to separate those, but again we do this on a massive scale. Not just for ammonia synthesis, but for all kinds of petrochemical processes where we need dihydrogen to make various petrochemicals and refine petroleum. On massive scales again. Thus, we could make ammonia synthetically by treating dinitrogen with dihydrogen, but it required these high pressures, but now we also realize how ungreen that is, because you have to heat methane with steam at very high temperatures in order to get the steam reformed product, which is dihydrogen.
You have to pay the price of heating all of these gases and compressing them, which isn't free. It takes a lot of energy to do that. We're using up a lot of petroleum and natural gas to make the electricity to compress these gases, and just to get steam and methane to the right temperature to do the steam reforming and to make dihydrogen to make ammonia. It would be much preferred to make it by using a catalyst that uses electrochemistry for reduction using solar- or hydro-generated electricity and then just use water or some source of protons to make the ammonia, avoiding going through dihydrogen and going to 400 degrees. It would be cheaper and greener and all of these good things that we want. That was the excitement. Not so much that anybody was going to make it the way we did where we used sodium metal to reduce the zirconium from oxidation state four to two to start with. You would use an electrode, a cathode possibly containing the catalyst, for example. That was the reason that we were so excited. We didn't make ammonia; we made hydrazine which is even more interesting on a small scale because hydrazine relatively hard to make: you have to actually make ammonia, then you have to oxidize ammonia back to the hydrazine, and hydrazine's not all that stable. It's not anywhere near as useful on a grand scale like ammonia, but it was still a step forward. That's why we were excited.
ZIERLER: John, was there a position that opened up? Did somebody retire or did they create a new line for you in 1974?
BERCAW: I don't really know. I think they created a new line. I had an inorganic chemist colleague when I first came, and he left about the time that they made me this offer. I guess that it could be that I filled his place on the faculty. His name was Joe Gordon. He was a wonderful friend of mine. But when he was a junior faculty member, he left voluntarily. I was never told why. He left and went to IBM where he had a fantastic career. He passed away a few years ago. When Joe left, not long after that, they told me, "We'd like to make you an assistant professor. Congratulations." [laugh]
ZIERLER: Now besides the title change, how did your day to day change at all in terms of your interaction with students, in terms of your lab, in terms of the funding?
BERCAW: Well, the good news is I was an assistant professor, and I could get promoted to tenure if I did well. It also means I have to start teaching and go do committee assignments and be a full-fledged faculty member—which in retrospect that wasn't so bad. Of course, I love my research. The other thing was my salary was paid completely by the Noyes endowment. Now I had to think about finding some funds for my research program. I had to raise part of salary, too. Foremost, I had to a be great researcher and do what every academic finds to be a difficult problem. That is, finding enough funding for your research program and increasing that amount as your program grows. I started writing grant proposals. My first grant that was funded was from the National Science Foundation. I actually had sent in a proposal when I was a Noyes Fellow. They said, "We're not going to fund you. You're not an assistant professor." At least that's what the program officer told me. "Come back to us when you're an assistant professor." And I did. This guy, Oren Williams, was the program officer who handled my proposal. For some reason he absolutely loved certain people like Harry Gray, like Jack Halpern, my postdoc mentor. He loved Hans Brintzinger, my Ph.D. mentor. He loved several other inorganic chemists. The fact that I had worked for Hans Brintzinger and worked for Jack Halpern worked in my favor. He had lots of discretion, and he was much loved and much hated in the chemistry community for the way in which he distributed his funding. He gave me a very large grant—especially for a beginner—that allowed me to increase my group size, I don't know, tenfold from one person to 10 people in short order.
I was blessed that my proposal went to Oren Williams. He funded the field of organometallic chemistry very well, because he thought it was a very easy thing to sell to Congress and people who sign off on the NSF budget. He enjoyed telling them about what people like I was doing. That was good. I managed to get to that stage fine. I had now new graduate students joining my group. They're all excited about what we're doing, about working with this glassware that I love so much—the vacuum lines. We started thinking about other problems we might be able to tackle. That was right near the beginning. We decided if reducing dinitrogen is important, what about reducing carbon monoxide and making liquid fuels, for example, gasoline and other hydrocarbons from carbon monoxide and hydrogen? You can make methane from carbon monoxide and hydrogen, but it'd be the last thing you'd want to do, because as I told you, we make dihydrogen from methane and steam. You don't want to just go right back to one of the starting materials for making your dihydrogen. You would like to make something else like methanol. That's much more difficult to make cleanly from CO and hydrogen, although we now have catalysts that do it pretty well. Making even higher carbon numbered things like ethane or ethylene—ethylene would be fantastic because it is a premier starting material in the petrochemical industry. There are all kinds of things you could think about. We said, "Well, if these zirconium complexes reduce dinitrogen maybe they would reduce carbon monoxide." We started making what are called carbonyl complexes that are analogous to dinitrogen complexes except carbon monoxide is coordinated.
These are much more common in organometallic chemistry, but you can make the zirconium and titanium compounds also very easily from carbon monoxide in the low-valent or even in a high-valent oxidation states, if the metals find a way to get reduced, if you add CO because they're so stable—these carbonyls. We started treating our dinitrogen complexes with dihydrogen just to see what would happen. H2 displaces the N2 to form the dihydrides of zirconium and titanium, Cp*2ZrH2 and Cp*2TiH2. We then treated those with CO. At least in the zirconium case there were a number of reactions that happened with carbon monoxide. It's amazing we were able to figure all this stuff out, but we still don't know everything about it. When we added CO to our dihydrogen complex we made these dimeric zirconium complexes that had hydrogens on the carbon and double bonds between carbons for these dimers that we were making. I mean, a just amazingly complicated transformation happened, clean as a whistle. You can also do it under conditions where you make cleanly under H2 the methoxyhydride of zirconium Cp*2ZrH(OCH3). We were reducing carbon monoxide now with our zirconium complexes too. Some people—especially in the petrochemical industry—thought this was at least as exciting as making reduced dinitrogen. They were very excited that we made the methoxyhydride, Cp*2ZrH(OCH3). I remember a guy who was a member of industry called me up and said, "Have you ever tried heating the methoxyhydride under H2 to see if it will release methanol?" Because we had all of the components right there on the metal. I said, "No, but I'm not confident that we'll be able to do that even under hydrogen or something that would capture the zirconium product." He said, "Well, I think you're probably right, because we've already reproduced your reactions and isolated your complex, the product that you've made. And we've heated it with dihydrogen until it started to react. And we got a product." I said, "But it wasn't methanol." He said, "No. It wasn't methanol. It was pentamethylcyclopentane." They had reduced the pentamethylcyclopentadienyl ligands with dihydrogen to make that; they saturated the two double bonds in the diene to make the cyclopentane product. The other ligands got reduced off the before methanol formed. This was again stochiometric chemistry. It wasn't catalytic chemistry yet.
ZIERLER: John, to go back to this idea that the NSF program officer saw that organometallic chemistry was an easy sell to Congress. Was that related to industry coming to you with this excitement? Is that the connection point right there?
BERCAW: Oh, yeah. Right. Because people are providing campaign funding with a congressman or senator serving in Washington, the program officers were listening to what the industrial people wanted—we talked, I think, early on about ivory tower versus applied chemistry. There was a lot of pressure especially in the early days of organometallic chemistry. It was funded primarily on go see what's possible in this field and don't worry about an immediate application. A lot of people in the early days of organometallic chemistry were just making new molecules. They would do all of the rigorous characterization of what they made, but it was mostly gee whiz sort of chemistry. They would mix some easy to get starting materials of transition metals with very reactive molecules like acetylenes or alkenes. They would heat them together and get a bunch of products, and they would pour the product mixture down a column. It would separate into bands, different colors, and they would collect the bands and evaporate the solvent and get crystals and get a structure, and they'd say, "Look at that!" [laugh] That's what I mean by gee whiz chemistry. They had no idea what they were going to make, but they knew something would happen with these very reactive molecules they were using. There were some amazing products that they made in this way. Things like a carbon atom in the center of six irons in a prismatic arrangement. Those things are transformations so complicated. nobody could know how it happens. They were making these molecules and isolating them, and it wasn't clear whether they had any relevance to something of commercial importance, but everybody in the field including I was certainly enthusiastic about what they were finding. I was in the audience going, "Gee whiz! Look at that!" [laugh]
You know, when they would lecture on what they had done, I don't think that the people in the audience from industry were thinking this is all gee whiz stuff. We should just keep doing it. This was the entry point for commercial application of organometallic chemistry. Oren Williams knew that. He was willing to fund people who were enthusiastic and working hard, but I think you can still take issue. It's a little embarrassing for me to look back on how much money he gave me every year in today's dollars, but it was a lot. I'm not sure how many million dollars a year it would be. I didn't care; I just was happy that he was happy, and he was always encouraging me to do more and apply, and he would support it.
ZIERLER: John, besides supporting more graduate students, did the funding give you opportunity to upgrade your instruments in the lab?
BERCAW: Well, yeah. I don't think I changed very much. I did buy more gloveboxes for more students. I bought an infrared sprectometer and gas and liquid chromatagraphs and the kinds of routine instrumentation that a synthetic laboratory has to characterize things. I had other people like Jack Roberts and Sunney Chan and other people who were providing NMR spectroscopy. We had an X-ray crystallography lab that was very easy to use and not that expensive. I didn't really do anything totally different from what I was doing. I just did more of it, and it was easier. I could get more research done per unit time. Yeah, I think that the biggest part of funding us was salaries for the graduate students and postdocs and for their supplies. I had no problem buying N15 labeled dinitrogen or things like that before. But later I did do a lot of chemistry that costs in terms of starting materials for what I wanted to make. There were expenses that went along with the research, but mostly it's salaries that you're raising money for. Not so much for yourself. Caltech always expected you to raise a certain percentage of your salary to offset what they were spending on you. The agencies were happy with that, with the percentage I was requesting. It paid me partially, and it paid postdocs almost completely and graduate students almost completely. Graduate students also taught courses as teaching assistants, and that was including with their stipends. It was very nice having that support early on.
After the carbon monoxide I remember I was invited—I didn't even know what the procedure was—but I was invited to give a departmental seminar on my research program. I did. I was amazed at all of the faculty that were in town showed up for the lecture, and graduate students and postdocs and people from outside chemistry were there in a very large lecture hall. I lectured enthusiastically about what I had done. I had never given the lecture before, so I didn't know how long it would go. It only went 45 minutes instead of an hour. I thought, well, all I had to say at this point, "Well, we've got a lot more stuff coming along." Everybody was fine with it. I remember after that lecture my very senior colleague—one of my dear friends who has passed away since—Aron Kuppermann came to me and he said, "John, that was an amazing lecture. I didn't understand very much of it." He was a physical chemist who did very much theory on dynamics. He said, "But it seemed to be beautiful, and I was very impressed that you were able to tell us so much in 45 minutes." [laugh] I said, "Oh, OK, Aaron. I'm glad you liked that it was short." [laugh] Anyway, that, in retrospect, was probably my tenure talk to the department.
ZIERLER: Mm-hmm.
BERCAW: Unlike what goes on in today's tenure process, that is very carefully orchestrated by all of the divisions at Caltech, this was very casual. Nobody told me I was being considered for promotion to tenure. That was associate professor with tenure at Caltech. I guess it must've been after about five years or so. I guess I started in '72 it …, so I had been at Caltech about eight years total. It was about five years after I'd become assistant professor. One day there was a knock on the office door, and there was John Baldeschweiler, who was the Division Chair at the time. He walks in my office, and he sits down and he says, "John, I'm pleased to tell you that we just voted to promote you to tenure. Congratulations." I said, "What!"
ZIERLER: [laugh]
BERCAW: [laugh] Because these days you have to go out on these so-called tenure tours where you lecture all around the United States and speak to people that will probably write a letter in support of your promotion (or not). Candidates have to prepare a package that goes out with requests for external letters. I guess they did prepare a package for me, but they did it behind the scenes, and they didn't even ask me for a description of my research and what I planned to do in the future. They just had enough confidence that they voted me to tenure without ever telling me. [laugh] After John Baldeschweiler left my office, Bob Bergman called me on the phone and said, "John, I hear you were just promoted." I said, "Yeah! That was amazing!" He said, "You should know it was totally unanimous." [laugh] I said, "Well, that's great." That was another unexpected thing that happened to me. Things were much more casual back at the beginning of my time at Caltech. You may know that at that time, especially in fields like organic or physical chemistry, there were great men of the field who would call up some university department, some friend in the department, and say, "Mr. Smith, Dr. Smith, Professor Smith, Joe, whatever…I have a graduate student or a postdoc in my group that's really spectacular, and you should hire him or her." They would usually do it. [laugh] There was no formal search, you know? No equal opportunity issues or gender issues. That was the way the departments operated. It was evolving, but it hadn't gotten to where it is now back when I was promoted to tenure.
ZIERLER: John, did the promotion influence your research? Did you feel a sense to be more adventurous, to do things that you might not otherwise have done if you were not promoted at that time?
BERCAW: Yeah. I think some of that's certainly true. I was ready for a new challenge. I'd done the dinitrogen work, and after the carbon monoxide work, and I'd made some major steps toward what I was trying to do, namely uncovering the fundamental features required. But the problems were still enormous for such extremely large volume products. We didn't come close to solving either in terms of a practical application for fixing nitrogen or converting hydrogen and carbon monoxide to methanol or high hydrocarbons. In any case, they were very hard problems, and I decided I would try something else. I got interested in other kinds of questions in organometallic chemistry, such as what I think some people would call physical organometallic chemistry, where you focus on mechanism and thermochemistry. You ask questions about the thermodynamics of the chemical reactions you're studying and those you want to do. That is, what are the relative bond strengths to metal as you compare hydrogen to methyl and other sp3 hybridized groups or a sp2 hybridized carbon or an sp hybridized carbon? Those kinds of questions were out there, and although they'd been answered in organic chemistry, but not so for organometallic chemistry.
I was just very interested in doing really fundamental work at that point and also thinking about some applications that would be a little better suited to the kinds of elements I was working with. One of the big problems with these early transition metals is that their low oxidation states are very reducing; they can reduce carbon monoxide and dinitrogen, but the products that they make have very stable heteroatom (e.g. oxygen, nitrogen) bonds to the zirconium or the titanium. Thus, closing the catalytic cycle is not practical, e.g. where you have to get that reduced group off of the metal, like the methoxide off as methanol. Those transformations were thermodynamically so difficult. As I told you when H2 at high temperatures reduced the Cp* ligand before it reduced the methoxy group. I started thinking about working with hydrocarbons; these have no hetereoatoms that would lead to a thermodynamic "sink". There were well established processes like oxidative addition and reductive elimination that are fundamental transformations that work very well for the later transition metals. We soon found that with the early transition metals, these reactions didn't work well in both directions, especially for molecules with heteroatoms such as alcohols and amines. That was my next big area: examining the details of the reactions of early transition metal alkyls and metal hydrides with alkanes, alkenes, arenes, alkynes and so forth. I got interested in the fundamentals of hydrocarbon conversion chemistry. They actually turn over very well, because we weren't making these so-called heteroatom-to-metal bonds like metal-oxygen or metal-nitrogen bonds. When we began this research focus is when we started running across processes with our early transition metals that are novel and not particularly well suited to for the later transition metals. On the other hand, we found that others proceeded readily for both later and early transition metal complexes, such as migratory insertion where some unsaturated molecule inserts into a metal ligand bond. For example, ethylene readily and reversibly inserts into a metal hydrogen bond.
I got interested in these transformations and asked questions like, "when an ethylene inserts into a metal hydrogen bond is it a polar process"? That is, does it involve hydrogen moving as hydride or as a proton to the incipient ß carbon of the resultant alkyl? Or is it a more or less neutral process where you homolytically break M-H and make C-H, in other words the process is not polarized, without charge buildup. We started to look at fundamental processes, and we had some complexes that were ideally suited to that kind of study. The ones we looked at early on were in group 5, niobium and tantalum. We could easily make an ethylene hydride complex, Cp*2NbH(C2H4) and Cp*2TaH(C2H4), and by NMR spectroscopy you can watch it reversibly insert to make an ethyl complex Cp*2NbC2H5 and Cp*2TaC2H5 and then ß hydrogen eliminate back to the starting ethylene hydride complex. We were able to show that this works not only with ethylene, but with styrene and other kinds of olefins where you could vary the electronics and steric interactions and answer other questions that we were posing. We also carried out the same type of study with group 3 metals scandium and yttrium just to the left of titanium and zirconium. These are even more electropositive. They really react like crazy with ethylene. Here we found that it not only reversibly inserts ethylene into a scandium hydrogen bond, but the ethyl compound then inserts another ethylene to make a butyl complex, and then it inserts more and more and you make polyethylene with just this hydride Cp*2ScH starting material; no promoter additive is required.
Ultimately, ß hydrogen eliminates to make an olefinic end group on this big long chain that results from many, many equivalents of ethylene inserting. This new scandium hydride then repeats ethylene insertions until another ß hydrogen eliminates to make an olefinic end group on this other big long chain, etc. Wow, that's a catalytic reaction. This was our first discovery of an organometallic complex that serves as a catalyst for converting ethylene into polyethylene. Although originally I did not anticipate that we would make this discovery, I started getting interested in the whole field of polymer chemistry, especially polyolefin chemistry, one of the big businesses for Dow, Exxon, and Shell. All of these companies make polyethylene using "heterogeneous" catalysts developed by Edisonian, try and error, methodology. They were very effective, but they were difficult to study, being powders containing aluminum, magnesium and early transition metals, like titanium or zirconium or vanadium, and unlike our soluble molecular, "single site" [Cp*2Sc] catalyst, these conventional heterogeneous catalyst are "multi-sited" with a number of different catalysts, each producing polymer with different properties (MW, polydispersity, branching). Our interest was because we had a "homogenous" catalyst that could be dissolved, exposed to ethylene, and examined by NMR spectroscopy while it reacts with ethylene. Thus, we studied the classic fundamental steps of polymerization: initiation, chain growth, and chain transfer for the [Cp*2Sc] system. Our hope was that by establishing the fundamental steps for polymerization of olefins, we might be able to improve the catalyst to make better forms of polyethylene. For other alkenes, additional possible steps might take place. For example, when propylene CH2=CHCH3 inserts into a metal hydride bond M-H it can either a normal propyl
M-CH2CH2CH3 or isopropyl M-CH(CH3)2, depending on the direction of addition of the M-H to the double bond. Normally, in particular with the bis(pentamethylcyclopentadienyl) ligand system, further propylene insertions are much slower than are further ethylene insertions, probably because of steric crowding, so we began modifying the ligands.
While we were in that field we started noticing that compounds such as scandium hydrides would react not only with olefins, like ethylene and propylene, but with methane. [laugh] We were puzzled and would say to ourselves, "Wow! How does that happen?" For the later transition metals you could get these reactions to happen, although they were in low oxidation states. The later transition metals reacted with methane by oxidative addition, that is a low valent late transition metal reacts with methane to make hydride bond to the metal and a methyl to bond to the metal. That's not on for early metals like scandium. You start with scandium in its highest stable oxidation state, (+3), and thus you're not going to make a scandium+5 product by oxidative addition, because you only have 3 valence electrons for scandium. It would be like getting a noble gas like argon to react with methane and that's not going to happen. There had to be some other process. We found early on that this bis(pentamethylcyclopentadienyl)scandium methyl compound reacted with dihydrogen to make methane and the Cp*2ScH, but it couldn't possibly do that by oxidative addition, and then reductive elimination of methane. It had to be some other process. It went at -80 degrees Celsius instantly. It was very easy for Cp*2ScCH3 to react with dihydrogen. We coined a term that's still used that's the alternative to oxidative addition and that is "sigma-bond metathesis." There's a sigma-bond that comes up to the scandium, the hydrogen-hydrogen bond of H2. It's really favorable for the strongly electrophilic scandium center to interact with the H-H bond. This reaction is similar to protonation of H2 to form triangular H3+, which you can do with super acid. We thus had a neutral scandium doing this. It smoothly breaks the hydrogen-hydrogen bond as it's making a new carbon-hydrogen bond. You end up with a scandium hydride and methane.
This process is, as I said, very easy. There was a chemist that I knew while she was a postdoc at Caltech named Patricia Watson, who was working at DuPont Central Research. She made the discovery too, I think probably a little bit earlier than we did. We published more or less at the same time on this sigma-bond metathesis process. She was looking at some of the lanthanide metals which are very much like group 3 transition (d block) metals. She was seeing the same chemistry. She was, by the way, using two bulky pentamethylcyclopentadienyl ligands on those metals too, because they keep the metal complex from reacting with itself by association, very much like borane, BH3, reacts with itself to make diborane as the stable molecule.
Anyway, this was a lot of fun early on. We were doing scandium chemistry, and there was essentially nobody else doing that. Scandium is, by the way, pretty expensive even though it's relatively plentiful. There's just not very much use for it, so it's not plentiful because there was not much use. But I had enough money to work with it. I had a guy in my group who was perfectly suited to open up this area. His name is Mark Thompson. He's now a very distinguished professor at University of Southern California. He would work with the compound, and we were puzzled by the results he was getting because it was so difficult to work with the stuff. It reacted with water like nothing you've ever seen. Everything had to be perfectly dry and free of oxygen, which was OK. We could deal with that on our vacuum lines, but he was afraid to work with very much of it because it costs so much for the starting material. We just weren't making much progress because he couldn't identify what happened in a reaction, when he started a reaction on a 50 mg scale, and he was trying to isolate 5 mg of product from a big flask. It was not easy. His nickname was Tippy. I said, "Tippy, go away, buy a bunch of scandium oxide—which is what he started with—and make enough of this bis(pentamethylcyclopentadienyl) scandium chloride that you can fill a 50-gram bottle with it. [laugh] He said, "What? That's going to be outrageously expensive!" I said, "No, it won't. It's more expensive for me to pay your salary and not have you produce any new results than to go invest in something so that we can do it on a scale that we figure out what's going on." So, he did that. He came in to my office and proudly showed me these, as I said, beautiful crystals. These pentamethylcyclopentadienyl complexes make beautiful crystals even for scandium. Glistening yellow crystals in this flask sealed very tightly, of course.
Then we started to make progress. We did all kinds of chemistry with scandium. It was an amazing adventure. Especially, because we were able to make alkyls and hydrides that reacted cleanly and effectively with olefins and proved to be ployolefin catalysts. It was pretty clear that we were working with a neutral scandium analog of a cationic titanium. That's what we call an isoelectronic relationship. The neutral compounds were much easier to handle, because if you have a cationic titanium isoelectronic analog, you must have some kind of counter anion to balance the charge in isolating the salt. Often that counter anion was reactive and led to decomposition. Thus, we worked with very soluble neutral compounds that were isoelectronic to the cationic analogs. The scandium compounds were also analogous to the catalysts that were thought to be generated when you made commercial industrial catalysts for making polyolefins. Industry got very interested in what I was doing. Exxon called me up and wanted me to start consulting with them. I had a great consulting arrangement with Exxon in the polyolefins business.
ZIERLER: John, can you explain why polyolefins were so important to industry at this point? What was industry doing and what could your research enhance what they were doing?
BERCAW: Polyethylene was discovered by Karl Ziegler in Germany in the ‘50s. It was like many things; it was accidentally discovered. They figured out how this product melts above the boiling point of water, but melts cleanly and then cools back to the solid. It is called a thermoplastic. You melt it and put it in molds and when it cools you get the desired shape of the ultimate solid polymer. This has a sort of dark side to it. You can also blow films with the molten polymer to make polyethylene bags and polyethylene film that can used to package all kinds of things. Polyolefins were made from the monomers of ethylene and propylene and other olefins. The biggest polymers were polyethylene and polypropylene, but polyethylene's most important. This is made by taking naphtha—which is the lightest boiling component of petroleum—and cracking it in these enormous billion dollar plants called catalytic crackers that heat the naphtha. You heat it with steam and pass through a very high temperature region of the cracker at high velocities, and it cracks down to ethylene as a high component product that you then separate from the reactor, from the unreacted naphtha product. The steam dilutes the hydrocarbons, to reduce the amount of "coking", where it completely decomposes to carbon (coke) and hydrogen. The process breaks and makes carbon-carbon bonds, carbon-hydrogen bonds, and ultimately produces the olefins and hydrogen gas at this very high temperature, approaching a thousand degrees. This is another feat of the engineering, but ethylene was plentiful because we had lots of naphtha. We had lots of petroleum. You could even use—and this is still done—the ethane component of natural gas, which is the major component, use it in your cracking process, and it gives very high yields of ethylene. Countries like Qatar have lots of natural gas. They remove the gas from the well, crack it to ethylene, then catalytically make solid polyethylene pellets that they send that away in ships. All of these processes were very high volume, and the plastics industry was rapidly growing. You remember the movie The Graduate?
ZIERLER: Yeah, yeah. Plastics!
BERCAW: Right. Dustin Hoffman was at a pool party after his graduation, and a guy takes him aside and says, "Plastics. Plastics are where it's at." Or something to that effect. It was true. Plastic production was really growing at this point. The volume of polyolefins made in the world is just amazing. We're trying, of course, to deal with the inertness of these products. They don't easily go back into harmless products. In any case, industry was not thinking so much about pollution. They very much are now thinking about how to dispose of all the stuff they've made. They were very interested in making more selective catalysts, and we came across one of the catalysts in our efforts to make something less sterically hindered than Cp2ScH, something that did not have two pentamethylcyclopentadienyl groups, but it had a single methylated cyclopentadienyl group and appended other ligand—an amide. These more open catalysts are even more electrophilic than [Cp*2Sc] they reacted with ethylene to make a very special kind of polyethylene that nobody had seen before. It was something called a long-chain branched version of polyethylene.
Most of the long chains of polyethylene have ethylene monomer units all tied together, but occasionally there would be a long alkyl group coming off of the main chain. This chain alkyl occurs in only a small fraction of the polyethylene units but improves the properties of the polyethylene in a very favorable way. It is similar to a major copolymer, the one called linear low-density polyethylene (LLDPE). Although you didn't have to add any comonomers like hexene (produces a butyl branch), or octene (produces a hexyl branch) or butene (produces an ethyl branch) to obtain this new long chain-branched LLDPE. These LLDPEs have special properties, unlike high density polyethylene, which is highly crystalline and thus hard and brittle, the so-called linear low density material melts at about the same temperature as high density polyethylene and when cooled doesn't have any brittle properties, so it can be used in all kinds of applications where it has to have some flexibility. These kinds of breakthroughs were interesting to the industry. I remember I gave a lecture at Corpus Christi at a meeting of polyolefins people. I was already consulting with Exxon, but there were a lot of people from other polyolefin companies in attendance at this meeting. I told them about my scandium complex. They were amazed. Some of them—I was told; I didn't notice this—but, I was told that two or three people rushed to the back of the lecture hall to get to the telephone and call home, "You need to make this complex of titanium that Bercaw's made with scandium and see what kind of polyethylene it makes because it looks like it's going to be really interesting."
That was the beginning of a period where I developed mixed feelings about doing science that had almost immediate commercial application, rather than doing science aimed at uncovering the fundamentals. There was a big legal interference between two big polyolefin companies. It was multifaceted, and it was difficult to tell who sued who. I think it was Dow that was suing Exxon or Exxon was suing Dow for these discoveries that we had made with scandium. Because it turned out that when they put these things on titanium or zirconium it made this amazing new kind of polyethylene that was very useful. It was going to be worth a lot. [laugh] At that point I went to the patent office to explain some of these discoveries with scandium. I said, "This compound reacts with hydrocarbons in really interesting ways. I think we should consider patenting this." The patent office then consisted of a couple of guys that were pretty old. I've forgotten their names; it's just as well. I remember clearly them looking at what I'd written down as a potential patent, and they said, "Nah. This is worthless. Don't worry about patenting it." [laugh] It turned out that it was worth very, very much money.
ZIERLER: Yeah. [laugh]
BERCAW: The patent office, the Office of Tech Transfer, at Caltech is still smiling about that incident. I have to say, after that first encounter every time I went to talk to them about potentially patenting something there was, "Oh, yeah! Whatever you say, John!" [laugh]
ZIERLER: [laugh]
BERCAW: Because they were told the story about them turning down something that was worth millions, perhaps more. I mean, it was going to be worth a lot.
ZIERLER: John, this, of course, it's a microcosm for how Caltech at this point was really in its infancy in terms of thinking about intellectual property and protecting all of the research that was done at Caltech that obviously did have major consequences for industry. It was early; it was just not on their radar.
BERCAW: No. That's absolutely true. I don't fault them. They were just…they were growing just like I was. This was worth something. These companies got involved in a big patent interference. I've forgotten the name of the company that Exxon hired to represent their interests. This company had worked with Polaroid company in an interference with Kodak for the instant camera, the Land Camera. There was a big settlement that Eastman Kodak had to pay to Polaroid. It was in the billions of dollars. They were very successful, and Exxon was very anxious to have them represent them. Dow went to some other law firm. I don't remember which one it was either. I had immediately told Exxon when I was consulting about my research—I gave regular research seminars when I visited to consult—and my research group had actually developed a ligand framework that was in dispute at least in one part of the interference. Thus, they wanted me to represent their interests in these court hearings and depositions and documents that were being written. I remember saying, "I don't want to deal with this." They were willing to pay me, I'm sure, a really large amount of money for personal use, if I would consult with them and represent their interests. I said, "I don't like this, that we're getting so far away from fundamental research and doing all of this arguing with one another. I'll tell you what. I'm not going to go to the law firm and talk to them about this stuff. If they want to talk to me, come to Caltech and I'll charge you my regular consultation fee."
Which was peanuts by comparison what other academics were going to get when they represented Exxon or Dow. I didn't really care; I guess I was stupid. I didn't really care about getting a lot of money. I was disappointed by what I watched what was going on with my friends at Dow. I had a lot of friends working at Dow and a lot of friends at Exxon that I knew even better. I saw them all working in the lab trying to disprove the other company's declarations that they were sharing with each other. They would say, "This happens." Exxon wanted me to testify that the scientists at Dow were wrong and vice versa. Many of the Exxon scientists quit doing original research and started trying to make the lawyers happy. I think it was a dark period in a way, because in the end I knew nobody was going to win but the lawyers. That was actually true. There were out of court settlements. Nobody really got everything they wanted. They had to compromise. There is an amusing side note. I have a colleague named Jackie Barton, who at one point in this interference was on the Dow Board of Directors. I don't think she's still on the Board of Directors, but she was at that time. She said, "Boy, it's really hard to go to these meetings with some of my Board of Directors colleagues, because they're coming up to me saying, ‘Who is your colleague that's causing us a problem and costing us so much money with lawyers? Can't you tell him to cool it a little bit?'" [laugh]
ZIERLER: [laugh]
BERCAW: I said, "I hope you told them, ‘No way can I make him cool it!" She said, "Yes. That's what I said, John."
ZIERLER: [laugh]
BERCAW: I had all these friends from Dow, and they were trying to get me to come and give seminars, but they would have to call me back and say, "John, we can't have you here because no matter what we do we're going to get accused of tampering with witnesses. And you're going to just have to wait ‘til this over." I did eventually go to Dow and give my lectures, but this was a time when people really took sides. A lot of my friends were representing Dow, and I had to write documents to take issue with certain parts of what they were writing down.
ZIERLER: John, given the sensitivity of all of these issues in terms of intellectual property and patents —even though obviously Caltech did not have them—some of the mechanics of your consulting work, when you consulted for Exxon, did you have to agree to a noncompete so that you weren't also consulting for Exxon's competitors or how did that work?
BERCAW: Yeah. That's standard. You sign an agreement. It's one that's called a nondisclosure agreement when you consult with people, and then they can really talk to you about what they're doing, and you can have feedback and so forth. You can be sued if you break that agreement because, of course, they don't want their competitors to know all of these things. You have to have enough integrity as a consultant to not sign up and then try to consult with two companies that are so closely working on a common problem. I never consulted with Dow. I think that's very common in my area. You avoid trying to keep your brain separated into two parts where you talk to one company, put that in one half of it, then talk to another company and put that in the other half. It is impossible that there's no crosstalk between the two parts of your brain. Anyway, that was simple and very straightforward. That was never the question. It wasn't that they were worried I was going to tell them some Exxon secrets, or I was going to find out something from Dow that I would tell Exxon. They were just worried about the appearance of having me visit their labs and talk to their scientists, because somebody would challenge that as being tampering. Even though it probably wouldn't have been.
ZIERLER: Tell me about being named Shell Distinguished Professor. First of all, Shell—I assume that's the energy company Shell.
BERCAW: Yeah. That's right. They're located in Houston. Actually Exxon's polyolefins business is also just outside Houston in Baytown. That professorship was pretty straightforward. Shell was giving money to Caltech, and part of that money was used, I think most of it was used to support a professorship. I think I was the second Shell Professor of Chemistry or Chemical Engineering because I think a chemical engineer—I think it might've been Henry Weinberg—was the first Shell Professor. It went on for a short period—I forgot. Five years or something like that. They gave Caltech enough money to pay my salary probably through an endowment. That was really an honor that I got to be a Shell Distinguished Professor for a while. I didn't have to raise my salary through other grants. It was paid and also that gave me that freedom to use my funds some other way. It was quite clear-cut when it comes to—maybe this was in the back of your mind when you were asking about conflicts of interest.
I went every year to Shell in Westhollow Laboratories outside Houston to deliver a lecture and visit with their scientists. I was very clearly informed that this was not a consulting visit, and I should not disclose anything to them that I know from anywhere else. You're just supposed to listen and sort of make off the cuff suggestions. I asked them, "Why is that?" They said, "Because our gift to Caltech is tax free, and if you are seen consulting with the Shell scientists you will jeopardize our gift's tax free status. So, you really have to be careful about this." All of these companies know how they're supposed to behave. Shell does make a lot of polyolefins. I had friends there who were working in areas similar to mine. Even before I became Shell Distinguished Professor, I had gone there and talked to them and saw what they were doing. It was pretty close to some of the areas I was working at that time. But that was separate from the professorship, and it was a specific earlier timeframe.
The Polyolefin Revolution
ZIERLER: What did the research on polyolefins, in a broad sense, allow all of these corporations to accomplish? What exactly was the revolution?
BERCAW: Well, I'm not sure because I didn't work with these companies to know exactly how it all came out. There certainly was a revolution in the kinds of polyolefins you could make from the same monomers. You know, ethylene or propylene or some mixture of olefins. The catalyst development there was really the key to all of these new products that they were making. Certain catalysts made certain kinds of polyolefins. We were interested in the fundamentals. We would make molecules that had specific geometries and symmetries so that you can make all of these forms of polypropylene, especially, and even some new ones that hadn't been made before. I think I told you earlier that propylene is a lot more interesting than ethylene. Ethylene just has one possible way it can insert into a growing polymer chain, but propylene has two ways and furthermore, there is stereochemistry with propylene because depending on which face you use to coordinate to the metal you get a different so-called stereochemistry of the product. That was critical to the physical properties. For example, if propylene inserts every time the same way (the same regio- and the same stereochemistry) you get what's called isotactic polypropylene which has a very high melting temperature and very good properties. If it goes in with the same regiochemistry but stereorandomly, that is with one face or the other equally or near equally, you get something that's nearly worthless. It's just a goo. It doesn't have a high melting temperature. It's not very crystalline at all.
There were other kinds of things that I thought were even more amazing. Some of the Dow scientists developed a catalyst system that makes block copolymers using a chain transfer catalyst like diethylzinc, that shuttles the growing polymer between two different catalysts. One catalyst makes the block that can be crystalline and hard, the other catalyst makes the other block that can be amorphous and soft. These kinds of new materials challenge those scientists, experimentalists and theorists who examine the consequences of having hard and soft materials forced together, because these long blocks are in the same polymer molecule, and so they cannot phase separate.
There's something called syndiotactic polypropylene where propylene inserts first on let's say this side, and then the next one comes in from the other side, in other words it regularly alternates the so-called enantiofaces of the propylene it uses in the insertion. You obtain a polypropylene, syndiotactic polypropylene, that melts an even high temperature than isotactic polypropylene. To a chemist that's absolutely astounding! How does it know to do that? What is telling the incoming propylene how it just inserted the last one so it uses the opposite face this time? Syndiotactic is not as commercially important, but to a chemist that's even more amazing than isotactic. Then there's something even more astounding, something called hemitactic where every other time propylene approaches, it inserts in only one way, and then the intervening times it inserts stereorandomly! So, it uses either face. Then the next time it goes in the way it went the first insertion. These were principles that had general interest among chemists, especially the organic chemists. When I talked to them about this they'd go, "My God! How does it do that?" It does it not, just usually, but at least 99.5% of the time it inserts in the stereoregular way. These kinds of insights, into what is responsible for in choosing the faces are used in polymerization were worked out by many chemists, including my group. I guess I can say we did some of the clearest cut work in dictating one possibility over another. There were lots of theories out there about the way these catalysts worked and how they were so selective, but I think most people were in the school that we ultimately landed with.
The key was that the ligands do direct the polymer chain in a certain way, and the polymer chain orientation (up or down) is what dictates which face of the propylene is going to be used for the next insertion. That's how it remembers what it just did so it does either the same thing or exactly the opposite thing. Or it does this and then it's random after that. That's really harder to figure out. Anyway, all of these rules came out of people like our group working with making ligands that had certain properties, that would give certain stereochemistries to the catalyst, and that would tell us how ultimately the tacticity was established. That's a pretty complicated story, but maybe we'll talk about it next time if you want.
ZIERLER: Absolutely.
BERCAW: It's very involved, but very beautiful chemistry that goes all the way back to this guy named Natta in Italy. Natta was working with Ziegler—he was visiting from a company in Milan, Italy with Ziegler's group in Germany. While Natta was there Ziegler's group discovered by accident polyethylene. Natta, being an organist chemist, went back to Italy and began working in his own labs on propylene polymerization. He worked out—even back then in the late ‘50s—some theories for how it might work. These were not soluble catalysts; these were solids. They would take things like titanium chloride and add magnesium chloride to it, pound the two solids together using a ball mill, then activate the catalyst with aluminum alkyls. They would get very highly isotactic polypropylene from that catalyst system. Natta and his graduate students had theories about how the geometries and steric preferences for the crystal surfaces (which are chiral) enables the Ti catalyst sites to produce isotactic polypropylene. Natta's theories are very reminiscent of how these metallocene catalysts are working. Anyway, Natta had these graduate students that went into academics in Italy or Switzerland and followed on from the work they did with him as graduate students. These people were really clever. They were able to work out a lot of the rules just by looking at the structure of the polymer that was made and reading back to what the polymer structure indicated about the catalyst stereochemical preferences, also what it meant about how stereoerrors arise. It's not as direct as what we were doing, but a lot of their theories turned out to be true. [laugh] I give them a lot of credit.
ZIERLER: [laugh]
BERCAW: People like Pino and Zambelli and Corradini, these guys were really clever people. Several of them became my friends, by the way.
ZIERLER: John, two last questions for today as we round out the ‘80s, going into the ‘90s. First on the research side, the larger research world, and your collaborations both in the United States and in Europe. In 1989 and 1990 you were a visiting professor first in Germany and then up the coast in Berkeley in 1990. Then you were a Fellow for the Royal Society in Oxford. At just a general level, what was the value to you for going to these other institutions and learning what was happening there? What was valuable to you and what value did they gain from these interactions when you visited?
BERCAW: Well, I guess you didn't say, but I went to Konstanz, Germany but only for about six weeks to give a series of lectures. Because Konstanz, Germany is where Hans Brintzinger was Professor, I was going to visit him and bring him up to date on what we were doing and learn from his group. He was actually interested in this polyolefin problem too. He had made the first optically active metallocene. A few years later his group had done some very elegant studies using specifically deuterium labeled propylenes that addressed the insertion preferences and the ultimate outcomes of stereoerrors for metallocene catalysts. I began thinking about polyolefins seriously about the time I took the sabbatical. I took a sabbatical year and spent part of it at Caltech, but part of it in Germany and then went to Berkeley. I visited with Bob Bergman and talked to his group and other groups at Berkeley. The most time I spent and the most beneficial for me was to go to Oxford. At that time I had made a good friend of Malcolm Green, who just passed away about a year ago. Malcolm was a wild man. [laugh] I loved him very much. He was always very flamboyant and had great passion about everything he thought and did.
We hit it off really well from the very beginning. Regarding the polyolefin work, I really wanted to learn about what made people think about polyolefin catalysts the way they did. What was the history of it? What was the current thinking? There was a graduate student of his that was visiting from a company in Japan that makes polystyrene. He had made a discovery that led to a new kind of polystyrene, syndiotactic polystyrene. As his reward he was given the opportunity to go anywhere in the world to get his Ph.D.. He chose to go work with Malcolm Green at Oxford. He was at Oxford at this time; his name is Nobuishi Ishihara. He's very formal Japanese, very respectful. And here was this scientist that was hounding him for information at coffee and at tea every day and being very enthusiastic. I had the freedom, and I was there to go to the library and read. If I saw an article that looked like it might be interesting, I could immediately go read it and follow my interests really quickly. I taught myself quite a bit about polyolefins and how they are made. I was asking Nobu—we called him Nobu for short. I said, "Nobu, how did they come up with this theory that they have? What gave them that thought?" He was trying to answer me. He was looking very nervous, but he would answer everything very politely.
After about, I don't know, four or five times of this routine over coffee in the morning, a couple of members of Malcolm's group came to me and said, "John, you really gotta know what's going on here. Nobu is so upset from you talking to him. He thinks that you're challenging him, and he's afraid to argue with you because he's so polite, very Japanese culturally. And you need to just sort of lighten up a little bit in the way you talk to him about polyolefins." And I said, "Oh, my God. I didn't know that!" I immediately went to Nobu. and I said, "Nobu, you have to understand that I'm just a very enthusiastic guy, and the last thing I would want is to make you upset." He was so relieved. He just about melted right there. We became very good friends.
ZIERLER: [laugh]
BERCAW: I got to talk to him from then on very freely, and he was very useful at teaching me about polyolefin catalysis. I had that specific interaction that really helped out my research program.
ZIERLER: Then finally, John, last question for today. In 1990, two very special awards. The ACS recognized you for the Award in Organometallic Chemistry, a very specific award germane to your field, of course. And then you were also elected to the National Academy of Sciences. I wonder if you could just compare the honors—one very narrow, very specific to what you do and the other more general, recognizing that your specific area of research really has importance across the board—which is what the National Academy is recognizing with this selection. I wonder if you can reflect on those two awards in 1990?
BERCAW: Well, the election to the National Academy was…that was the most special award I guess I've ever got for the reasons you said. I don't really remember how many awards I had one at that time. I guess I only had one other award when I was elected to the NAS—the ACS Award in Pure Chemistry. The American Chemical Society now has a very large number of what are called National Awards. At the time when I won the Award in 1990 for organometallic chemistry, that was special. I'd already won this award in pure chemistry. It turns out Caltech's chemistry department, and especially the inorganic chemists had a very good record for winning the Pure Chemistry Award. Harry Gray won the first Pure Chemistry Award for Caltech. Oh, I guess Linus Pauling won the first award. That's right; he won the first one. He was certainly the first chemist to win. Then I guess I won the next one after Harry and then Jackie Barton and Nate Lewis all won that Pure Chemistry Award too. Theo Agapie won it most recently, another Caltech inorganic chemist, of course.
That was a very special award because it crosses all fields of chemistry and they pick from physical chemists, organic chemists, biochemists, all kinds of chemists. After the Organometallic Chemistry Award in 1990, I've won a couple of other National Awards of the American Chemical Society. It's nice to have those awards. I don't want to say anything to belittle their importance, but the National Academy is really special. Although I didn't really want to win any kind of award, I remember thinking many years before I got elected to the Academy that it would sure be nice to be an Academy Member. [laugh] That was a very special ACS meeting. It was in Boston where I found out that I got elected to the Academy, while I was at that ACS meeting getting the other Award for Organometallic Chemistry.
ZIERLER: [laugh] That's great.
BERCAW: There were not too many people who had gotten the Organometallic Chemistry Award, unlike the Pure Chemistry Award, which was the first National Award for ACS. The Organometallic Chemistry Award was only a few years old when I got it. I was one of the early recipients. That was an honor.
ZIERLER: That's great. Well, John, that's a perfect place to pick up for next time.
[End of Recording]
DAVID ZIERLER: This is David Zierler, Director of the Caltech Heritage Project. It's Tuesday, October 26th, 2021. Once again it's my great pleasure to be back with Professor John E. Bercaw. John, always great to be with you.
JOHN BERCAW: Great to be with you too, David.
ZIERLER: John, today I'd like to start with a service question. The Solar Energy Research Institute Chemical Sciences Review Panel, 1990-1993. Can you tell me a little bit about that work and what was going on in solar energy research at that point?
BERCAW: Wow. That was way back. I remember going there. It's in Golden, Colorado, as I recall. I was a member of a panel that had about six scientists; I guess I was really the only organometallic chemist. At that time solar energy was not really forefront in the big agencies and so, they had their own institute—I'm trying to think why it was I was involved. [laugh] I think they just wanted a chemist, and because I also had served in other similar roles for DOE labs, that they thought I might do a good job. As I recall, solar energy was something that was way far in the future at that time. and they were trying to change that. There were colleagues of mine especially Nate Lewis, who was doing semiconductor work with photocells and photovoltaics and trying to understand the fundamentals. Since I served on doctoral committees with some of his students I got to hear a little bit about some of that from the students and also from Nate. There were chemists at SERI doing other kinds of research like trying to reduce carbon dioxide to carbon monoxide and methanol which is not directly solar. It could be solar, I suppose, because they were using electrochemistry. One of my former postdocs is on the staff there, and I remember talking to him about his projects involving palladium catalyzed electrochemical reduction of carbon dioxide to carbon monoxide. I was fascinated by that, because that was closer to my own work. It was broader than just solar energy research even though that was the title of the institute.
ZIERLER: John, I'm curious given your work with industries that were involved, of course, in petroleum and fossil fuels, when did companies—like Exxon, for example—start to express interest in alternative energy sources, from your perspective?
BERCAW: Exxon, as I recall, was sort of a latecomer. They were very much interested in petrochemicals and polymers and, of course, fuels—mainly gasoline. They were interested in those, and I suppose they had some people doing other kinds of energy research, but I don't even recall in all my visits to Exxon talking with somebody who was really right in the middle of solar energy. BP was quite different. They had their own solar energy division. It wasn't very big. They were also very much centered on petroleum, of course. And Shell, the same way. I consulted with them as I think I told you last time. Again, I didn't hear very much about solar energy back in around 1990. I forgot, how many years was I with…I guess it was called SERI [Solar Energy Research Insitute] then. I've forgotten. It's now called NREL. Is it NREL now?
ZIERLER: I believe so.
BERCAW: Yep. OK. NREL [National Renewable Energy Laboratory]. I'm not sure how many years I worked with SERI, but I remember some of the people that were on the panel with me. I don't remember much about what we did. There was a lot of controversy going on between Nate Lewis and some people at SERI at the time about the mechanisms of solar to electricity for photovoltaics, and what the limits of efficiency were, theoretical and experimental limits. We were also not so much doing discussions with scientists, but we were talking to the management and giving them some advice on managerial issues.
That was sort of like the job I had on the panels for the DOE laboratories that I visited, like Los Alamos and Livermore, especially. Also, Lawrence Berkeley for a few years. We would sit and talk to the Director of the labs, his Principal Associate Directors, and give them some ideas about what kinds of issues they might add to their portfolio and give them some feedback on the quality of science. Sometimes we would criticize them on their decisions, try to get them to do strategic planning. I think that the same sort of thing happened at SERI. We were doing both science assessment, quality assessment, and also talking to them about their portfolio and their management.
ZIERLER: You mentioned BP. I'm curious if in 1992, when you had the Sir Edward Frankland Prize Lectureship of the Royal Society, if that was from a BP connection?
BERCAW: No. I think that prize is solely administered by the Royal Society of Chemistry. Not with any one company. It's much like a national prize from the American Chemical Society, except the British version of it. In fact, I guess I had met some BP scientists at some scientific meetings but, no. That connection was solely the RSC's, that big award that I got from them was at their own initiation. BP contacted us and asked us to prepare our proposal, and we did that. They were just reading the literature and going to scientific meetings and listening to scientific talks and reaching their own conclusions about who they might partner with in the United States.
ZIERLER: And then, John, the year following you were named Centennial Professor at Caltech. What was that like for you?
BERCAW: [laugh] It's a funny name. My friends and colleagues said, "Boy. I didn't know you were a hundred years old."
ZIERLER: [laugh]
BERCAW: I'm not that old, so I had to live with that a little bit. That was a professorship that was, I think I told you before, was paid for by an endowment that some donors who wanted to remain anonymous. I asked them why they call it that. It turned out it was given to me on the so-called centennial anniversary of Caltech. They decided to call it that rather than with their name. They continue to call it that as other people have had it. It was a nice deal, because I didn't have to worry about raising my salary anymore, and I had freedom to use that money that I would've used to offset my salary partially to do different things than I otherwise would've done with my federal support. It meant that I was worthwhile. I might also say that I think it was awarded to me when I was considering moving from Caltech. They were trying to retain me, I think in part by giving me this professorship, but also by redoing my laboratories and offices. It was part of a package deal that eventually led to me staying at Caltech. I'm glad I did, but I had a couple of opportunities that I was considering at the time.
I was entertaining a possibility of moving to UC Santa Barbara to take a chair that was just opened there. Maybe that was part of the thinking of Caltech awarding me the Centennial Professorship. After I visited Santa Barbara several times, I decided, if I was going to move to a UC campus and leave Caltech, I should probably go to the flagship UC campus, UC Berkeley. I contacted people there, especially Bob Bergman, who is my former colleague at Caltech and had been at Berkeley for many years at that point. Berkeley was something I thought a lot more about. In fact, I even went and looked at housing. A big consideration was whether my wife would want to move from Caltech. We both like Pasadena and staying in the West would be good. I got pretty far along, but I eventually decided the students at Caltech and the colleagues at Caltech were so special, I couldn't leave. So, I decided to stay. A few years later, I also got an offer from Harvard to move there, but that wasn't really a close call because Diane said, "If you go to Boston, you're going alone. I'm not going with you." I said, "Well, OK…" [laugh]
ZIERLER: [laugh] John, the offer to overhaul a laboratory, of course, gives you opportunity to think about new instruments, new ways of looking at research problems. On that point, what was moving into the mid-1990s? What was some of the work you were doing on olefin polymerization at that point?
BERCAW: I think I was winding that work down at that point. We were still interested in the origins of stereochemistry in α-olefin polymerization. At that point I was getting more interested in other kinds of projects like carbon-hydrogen bond activation and work that could ultimately lead to hydrocarbon utilization, especially methane as a feedstock for making liquid fuels and petrochemicals and so forth. For a lot of my research I'd moved on from olefin polymerization into C-H activation. The olefin polymerization work was sort of winding down. I think this is the mid-90s. I was interested in one of BP's projects. One of my former students was working at one of the U.S. laboratories of BP, and he and some of his colleagues in England discovered a chromium catalyzed process that took ethylene to a higher olefin—one higher olefin specifically—1-hexene. That's a trimer of ethylene. These catalysts did so with astounding selectively. I got interested in that kind of work, and I maintained that interest all the way up to my retirement. In fact, I went back in the lab and was working on that with a postdoc and a graduate student of Theo's when I retired. The olefin polymerization field was at that point was well populated. People understood most of the essential features. There really wasn't a lot of incentive to do something truly novel in the olefin polymerization area as far as I knew. I was thinking about other areas.
ZIERLER: Now given that this field came to maturity—olefin polymerizations—what were some of the industrial applications at that point? How had it moved into industry? What had it made better socially? Economically?
BERCAW: I don't know about socially. Well, I think that people felt they understood well enough how to engineer it, and they were practicing it on enormous scales at that point. Much of the polyolefins was still being made with the more traditional powdery catalysts. The amount of polymers that were being made worldwide continued to increase at an exceptional rate. I mean a few percent per year is a lot. They were making more and more, but I don't think that they really made any new products so much as made them more efficiently and with improved polymer properties. Because they understood the fundamentals of things, they felt like they could now predict with more confidence, when they had a problem, what were the possible reasons for a these problems and how to fix them? But I think that I should go back and tell you that at the time when people like me and others were doing olefin polymerization work with well-defined organometallic compounds as catalysts, there was a lot of skepticism in the polyolefin business.
They thought no one was going to beat the catalysts that were developed by trial and error, these heterogenous catalysts that were made by just mixing together various salts of titanium and aluminum and magnesium. These were very, very good. They didn't think there was any room for improvement. There was a group of people that thought that this field really did have something to offer other than just laying out the fundamentals. The well defined organometallic catalysts are single-sited. That is, there was a single catalytic site, rather than a group of catalyst sites that are working in parallel with one another, as is the case for traditional catalysts. That is to say each type of catalyst makes polymer chains that were more or less of the same, but with these multi-sited catalysts you would necessarily get a mixture of polymers of different properties. The single sited catalysts prepared from a single organometallic compound make polymers that all have the same statistical length distribution, and poly(α-olefins) had the same tacticity. That is, they were all equally isotactic or were syndiotactic, or even atactic. These other catalysts that they were replacing had these multi-sited problems; you couldn't get a uniform product from them. I should point out that some people noted that in some ways it's good to have a mixture of polymers. These old-fashioned catalysts actually had some advantages over these purer single-sited catalysts, mainly in having rapid crystallization kinetics. Slow crystallization kinetics can be a problem when the thermoplastic is cooling.
As I said earlier there's something called linear low-density polyethylene (LLDPE) that is a copolymer of ethylene and an a-olefin. It's typically 1-hexene or 1-octene or even 1-butene. Copolymerization with these comonomers allowed there to be the side chains that came off of the long main chain of polyethylene that changed the properties and made them softer. These old-fashioned catalysts would do that, but not with the uniformity a single-sited catalyst produces, a uniform distribution of α-olefin over the entire length of the polymer chain. The multi-sited old fashion catalysts produced a mixture of LLDPEs. Some chains had a small amount of comonomer; others had lots of comonomer. The new single-sited catalysts gave new kinds of linear low-density polyethylene, only one amount of comonomer for all chains. Especially Dow was very proud that they were able to take these new organometallic catalysts and dial in the properties of the polymer that the customer wanted. They could with confidence predict the molecular weight, the resilience and level of brittleness that the customer wanted from Dow. Right away they could make the polymer that they wanted to make. They were playing around with these new catalysts at Dow and making really fundamental breakthroughs in the kinds of polymers that you can make. Whether they had widespread use, I can't really say. You'd have to ask Dow. I do know that they were touted in the literature as having new and improved properties. Particularly interesting were the two catalyst, chain transfer agent systems that I mentioned earlier for making blocky copolymers. The companies did a lot of innovative things with single-sited catalysts that they developed there and that the organometallic community provided for them. In the end I think everyone figured out that there was a place for the old catalysts—and they still make a lot of polyolefins with those—and there was a place for the new ones.
Early on, most of the industry would not want to practice making polyethylene using a solution of an organometallic compound, because they had engineered all of their reactors, these enormous reactors, to use the solid old-fashioned catalysts that were powders that they sprayed in the reactor. They figured out early on that you could take these organometallic compounds and support them on something like silica particles. They figured out the best ways to do that. They lost some activity, but they maintained their single-sited advantages. Thus, they merged some of the old technologies with the newer catalysts. The organometallic compounds were dissolved in a solvent and added to silica that had been treated with an activator, usually an alkyl aluminum compound. There was give and take between industry and academics. Some of us academics tried to figure out what might be going on when you supported them this way or that way.
The polyolefin industry has come to the realization that they need to find out a way to make a recyclable or biodegradable version of the polyolefins. I regularly go to a series of symposia sponsored by the Polymer Division of the American Chemical held every other year; I think I probably attended five or ten of those in the past. The topics were wide and varied, and they'd talk about economics and engineering as well as the chemistry going on. This past meeting was almost totally focused on how are we going to make a biodegradable or recyclable polyolefin, so we don't continue to pollute the world with all of these polyolefins that people are throwing away? I think that that's a big focus of the polyolefin business now, to finally reconcile that their polymers are really making a mess of oceans and all these other places where polyolefins end up and are very slowly biodegrading. They don't really biodegrade; they sort of decompose by partial oxidation usually promoted by sunlight. There's a change in emphasis more recently toward making something as inexpensive as a polyolefin that has some recyclable or biodegradable properties as well, and that's a big, tall order, especially because there is so much polyolefin made. Making something that's not a polyolefin that takes its place without a big cost increase is going to be a real challenge, and they know that.
The Importance of NMR Spectroscopy
ZIERLER: John, on that topic, what were some of the advances in NMR spectroscopy that were relevant for this research?
BERCAW: Well, I think it goes back to scientists at Bell Laboratories. Early on NMR was used by chemists to look at solutions of pure, uniform molecules, not mixtures like what you often have in polymers. The NMR spectroscopists figured out how to quantify the stereochemical purity of things like polypropylene. In fact, my colleague, Jack Roberts, used to consult with some of those people. I think one of his former students was the person at Bell Labs that was involved in that research. It was a really cool application of NMR. The NMR spectrometers were getting better and better at resolving very small chemical shift differences. You could get very high-quality spectra even though these polymers at room temperature are, of course, solids. You have to dissolve them in some sort of solvent. If you run them in a solvent such as trichloroethylene at a high temperature around 150 degrees Celsius typically, they give high quality spectra that allows determination of the nature of stereoerrors and distinguishing how these come about. Maybe I should elaborate on that a little further. The 13C NMR signal for the methyl groups of the polypropylene provides a lot of information: at high molecular weight isotactic polypropylene has a very low concentration of chain ends, and thus by far the largest signal in the 13C NMR spectrum is due to middle segments having all methyl groups pointing together in the same direction off the main chain, giving a dominant very large single resonance. Nearby and upfield of this signal are much smaller resonances due to methyl groups that pointed in the wrong direction and those methyl groups pointing in the right direction that are close enough (usually 2 or 3 carbons away on either side of the steroerror) to slightly change the chemical shift. Even though the catalyst favors the correct face of the propylene to be used, there is a probability, usually less than a few percent, that the wrong face will be used, because the barriers for inserting with the correct face and that for inserting with the wrong face are similar. With high resolution the 2 (usually correct) methyls next to the one pointing in the wrong direction, and the 2 methyls just beyond those 2, along with the methyl pointing in the wrong direction give rise to a "pentad" with a characteristic upfield shift of the major 13C signal. Statistical analysis of the intensities of the 13C signals due to the 10 possible pentads of polypropylene has allowed scientists to distinguish between various possible mechanisms for stereocontrol: for example, whether the chirality of the metal catalyst or the chirality of the growing polymer chain at the second carbon away from the metal, "enantiomorphic site control" or "chain end control" dominates.
If it makes a mistake and the stereochemistry for the next insertion is dictated by the orientation of the last inserted monomer then the system will continue that mistake and all the rest of the methyls will go back instead of forward. That'll give rise to a characteristic set of resonances around those mistakes that you can, by statistics, you can quantify. Whereas if the way in which a mistake occurs is not dictated by the last inserted monomer's orientation, but by the chirality of the catalyst site, then after it makes a mistake it will go back and correct itself so the methyl group will return to going in up. We could tell early on in the single-site catalyst business for making polypropylene how it worked. It worked in almost all cases that the chirality of the metal dictates how the monomer should go in.
There's naturally going to be this background of the chiral influence of the last inserted monomer, but that's a relatively minor influence compared to the chirality of the catalyst site. That's an example of how carbon-13, especially, NMR spectroscopy has influenced the polyolefin business. We use that routinely.
We also did some experiments that established the influence of the two a-carbon-hydrogen bonds for the M-CH2(CHCH3)P) in the insertion step, the so-called a-agostic assistance for olefin insertion. We used some ideas to tests for this kind of assistance based on kinetic isotope effects that came out of the Grubbs group. They looked for isotope effects in support or not in support of this so-called a-agostic effect that was in the popular literature at the time, especially by my good friend Malcolm Green. We were able to make olefins that had deuteriums and hydrogens along the chain in various ways. We were able from that experiment to show that there was indeed an isotope effect in the right direction and of about the right magnitude to be involved in the insertion. That had a crucial role in the stereochemistry, because coordinating one of the two a-C-H bonds oriented the growing polymer chain up or down during propylene polymerizations. People have done some experiments to show that that might well be the case at least some of the time. There were people doing theory. You could calculate that coordinating one of the two a-C-H bonds would give you a favorable influence on the rate of insertion by lowering this transition state when you this alpha hydrogen coordination occurs as the olefin inserted. We did NMR experiments and used deuterium NMR for analysis. In that case, where we could tell the isotope effect and the direction of the isotope effect and the magnitude of the isotope effect. All those were in accord with α-agostic assistance. We did several experiments like that and published our results in JACS They got quite a bit of attention. NMR was crucial to us in doing these kinds of experiments not just for establishing the structure of our molecules. We used NMR to determination the purity of the chirality when we made enatiomerically pure zirconocene precatalyst. We then made some 1-pentene that was chiral at the 3-carbon by substituting deuterium for hydrogen in a sterochemically pure manner. This chirality reported the facial preference for addition of 1-pentene in polymerization and for 1-pentene in hydrogenation for comparison; NMR allowed us to establish quantitatively how stereochemically pure our products were. All of these experiments led ultimately to our conclusions concerning the important role that the growing polymer orientation, set by the a-agostic assistance, was crucial. These experiments also confirmed for the first time that our models that we were using to rationalize the absolute facial preferences were, in fact, as we had thought. The metal chirality relays to the polymer chain which of the two a-hydrogens should coordinate. All of these projects relied on NMR.
ZIERLER: John, moving into the mid-90s, the late 90s, as you were getting more interested in hydrocarbon research. What was the relevance of this to companies like BP? What was their interest in this research?
BERCAW: Well, this was one of the grand challenges that BP researchers identified. I think all of the petroleum companies were interested in carbon hydrogen bond activation. The interest to BP was, initially at least, was in taking methane and making a liquid fuel out of it. If you could partially oxidize methane to methanol, cleanly and using oxygen, methanol could be used as a fuel substitute made from methane. It's like ethanol. You could distribute it in the same way they distribute gasoline: people can pump it out of a tank and in to their car or truck at a service station. They were interested in that goal or making perhaps by oxidatively coupling methane using oxygen to make water and higher alkanes. Maybe even heptane and octane, a synthetic gasoline that would be made by partial oxidation of methane. There was a lot of thinking that there was more and more methane being found around the world. It was even what I later found was apocryphal, that was there was stranded gas in places that were hard to get to because they couldn't drill for gas easily in these locations. There was also a lot of methane hydrate, where the methane molecule is surrounded by water molecules. In fact, most of the methane is in more of a hydrated icy form in the arctic. When this ice melts, it releases gaseous methane. Moving methane around was recognized as being a very expensive proposition because it's a gas. It's the major component of natural gas.
It varies, but natural gas is always at least 80-90% methane. Getting natural gas to a location where you need to use it is expensive. You can build an expensive pipeline; we have lots of those. We also liquify methane. It doesn't easily liquify, but you can refrigerate it and compress it into a liquid. That's how methane is moved around the earth, on these ships that have liquified methane in these like giant thermos bottles that are well insulated. That's an expensive thing—to make that much liquid methane out of gaseous methane takes a lot of energy and again, it's not very green. You have to create that energy usually by burning something, namely methane, to liquify more methane. They were thinking, wow, it'd be great if we could go to the source, whether it's methane clathrate—that's what this methane in ice is—and melt it, or we could take it out of a well, and partially oxidize it on site to a liquid fuel, either synthetic gasoline or make methanol and ship these liquids around that way without having to pay a price and the pollution costs by taking methane to a liquid and then moving it. There was a lot of interest in using methane as a way to make liquid fuels rather than taking petroleum and refining it into a series of components and removing sulfur and nitrogen by hydrogenation. Of course, such a liquid fuel would be a lot cleaner burning if it was made from pure natural gas. Currently these impurities are ultimately polluting the atmosphere with things like sulfur and nitrogen oxides which form acids with atmospheric water. Thus there is a lot of impetus. The energy companies had known for a very long time that it would be great if we could do something with methane other than just burn it. The real challenge was not getting oxygen and methane to react with one another. It was getting them to react in a selective way that would make only methanol or only higher hydrocarbons and water rather than the thermodynamic products, which are carbon dioxide and water. Of course, that's what we get when we light a methane source.
You don't make detectable amounts of methanol or hydrocarbons, when you oxidize methane in a flame. It usually finds a way to go completely downhill to the unwanted products. Although when you oxidize methane only partially to methanol or higher hydrocarbons and water byproduct you do not get the maximum heat, but you do produce some energy because even partial oxidations of methane are exothermic. It had been known that it would be great to do all these things, but everybody who tried to partially oxidize methane with oxygen was frustrated. There were lots of people trying to devise catalysts. Most had tried to devise catalysts for partially oxidized products by trial and error with solid materials that they passed oxygen and methane over. They observed what appeared to be a ceiling in the yield that was very low. Only a few percent of the methane would go to things like methanol rather than all the way to carbon dioxide and water, a very frustrating finding. Most people have just given up, although some people are still playing around, trying to find these catalysts by trial and error.
This was the status of a longstanding issue, but the payoff was just so enormous. If somebody discovered a catalyst that would selectively take methane to methanol or higher hydrocarbons with oxygen, it would completely change the energy landscape. It would make everything greener that uses petrochemicals and that would be something that, of course, BP or Exxon or Chevron or Shell would love to do. They would like to be there first and reap the benefits of having a new catalyst that is a lot more selective in a good way. Anyway, that was the thinking. We need to get hydrocarbons to selectively go to liquid fuel. It was also depressing to think that all we could do (apart from steam reforming it to CO and H2) was burn methane. That's pretty much all we do. Even in the chemical industry they burn methane to furnish the energy needed for all of the processes they're performing to make petrochemicals, not only from methane, but from other things. Steam reforming of methane to hydrogen and carbon monoxide and then convert these products to methanol and hydrocarbons and water is practiced on an enormous scale, but steam reforming is energy intensive and certainly not green. You could even dream about making ethylene from methane by partial oxidation. That was the impetus that these companies, they knew it was a very important area for them to work in, but all of the prior research frustrated them in finding the right kind of catalyst.
ZIERLER: John, tell me about your visiting professorship at Harvard in 1999.
BERCAW: I think I was there for only a week. Are you sure it was…
ZIERLER: This was the Robert Burns Woodward Visiting Professor at Harvard.
BERCAW: Yeah. [laugh] Yeah. I visited for a whole week.
ZIERLER: Oh, I see. [laugh]
BERCAW: I gave a little lecture series. You know, it wasn't so grandiose. Although Woodward had passed, he was such a hero in chemistry at Harvard that they maintained his office in sparkling condition with wood paneling and beautiful furnishings. It was very, very luxurious. I got to sit in that office for the week I was there. Their main reason for giving me that lectureship was to try to attract me to Harvard. I think it was like the second or third day when I visited with my friend, Jeremy Knowles, who was the Dean of Arts and Sciences at Harvard at the time. I went into his office, and he handed me an envelope with an offer to come to Harvard and join the faculty. [laugh] I think they were making life at Harvard look like a grandiose thing for me that week. It was fun because many of the faculty there were long term friends, a number were my former Caltech colleagues who had moved there. I guess I like to think that they were pushing for Harvard to make me an offer, because they enjoyed having me as a colleague at Caltech. Harvard is a great place, no question about it, but the style is very different. It's much more, I don't know, I guess I can use the word—uptight. The faculty are less prone to collaborate, they're less prone to work together and not compete with one another. That's one of the great things about Caltech I really loved. I think my former colleagues loved it too, but they wanted to be at Harvard. Most of the people that moved from Caltech were the organic chemists, and Harvard had the best group of organic chemistry professors in the world. There wasn't a comparable effort in inorganic chemistry.
They had many people outside Harvard criticizing their chemistry department for being so focused on organic chemistry and physical chemistry as well, and biochemistry, but not so much inorganic chemistry. For two or three years I was a member of the committee for external assessment of the chemistry department for the provosts and the deans. We were charged with looking at the department and with writing a report to answer questions such as, "What's the status of our chemistry department at Harvard? Is it great? Where is it great? Where do we need to improve it?" When I was on those committees, I always pointed out that they needed to get some really outstanding faculty in inorganic chemistry. They remembered that. I told ‘em they should hire two people at the same time, because they were having so much difficulty moving somebody they really wanted in inorganic chemistry. I said that, "If you have offers to two people you'll have a better chance." It turned out while I was at Harvard they approached a young guy at MIT and said to him, "We're making Bercaw an offer. We'd like you to come with him to Harvard." We were good friends. His name is Kit Cummins. He's actually my academic grandson. He worked as an undergraduate at Cornell with one of my former students.
Anyway, the next day he came over to Harvard, and he sat down in the office, the Robert Burns Woodward office. The first thing he said was, "You're not going to Harvard, are you, John?" I said, "I don't think so, Kit." I said, "Are you?" He said, "No. I like MIT. I'm going to stay there." [laugh] Even though they were being very clever in this one week lectureship that I had there, the odds weren't very good. They weren't surprised when we both turned them down.
ZIERLER: John, on the topic of grandiosity, that same year in 1999 the American Institute of Chemists named you a Chemical Pioneer. Was that more of a lifetime achievement award or was that in recognition of a specific pioneering aspect of your research?
BERCAW: I think it's probably the former. The American Institute of Chemists is not anywhere close to the stature of the American Chemical Society. I don't even think I belong to the American Institute of Chemists. Or I ever have belonged. They recognize people through this one award and it does have some stature. I shouldn't put it down completely, but it wasn't something that made the same impression on you as getting an actual national award from the American Chemical Society. Yeah, I guess their big award was the Chemical Pioneer, and I was an inorganic chemist, and they selected an inorganic chemist that year.[laugh]
ZIERLER: Another award that year—I'm curious since we're talking about hydrocarbons—The George Olah Award for Hydrocarbon or Petroleum Energy. Was this more aspirational given—was it more about the promise of where hydrocarbon research could go? Or even as early as 1999 there was really a significant body of research at that point?
BERCAW: Yeah, I think it was based on our fundamental work in CH activation of hydrocarbons, early work on sigma bond metathesis and recent work with platinum based chemistry. I'm pretty sure that's why I got that award. George Olah was a friend of mine. He was a chemist at Dow when he did his Nobel Prize winning work. He moved Case Western and eventually to the Loker Hydrocarbon Institute at the University of Southern California. I used to visit with him occasionally; go over there for joint seminar. He and I were also part of a joint grant from DARPA that involved scientists from Caltech, JPL, MIT, Harvard, and USC. I was fond of him; he was a real character. I was pleased to get that award. The reason they gave the award his name was that he did the Nobel Prize winning award by showing you could add a proton to a hydrocarbon, a particularly amazing finding at the time. Even things like methane will take a proton and make CH5+, a cationic molecule, a carbocation as it's called. Then George did a lot of fundamental work on making and characterizing carbocations and exploring their chemistry in very acidic media solvent systems. He was always pushing hydrocarbons, and about the time that that award was created he was writing a book on methanol, all of the glorious things methanol could be used to do, and ways you convert methane and methanol. Of course, he didn't have a practical process for doing it yet, but he said if we did have a process this would be a really great thing. It got a lot of attention. George Olah and hydrocarbons are sort of synonymous. He used to call me on the phone when I'd publish a paper, and he didn't like something in the title or that he picked up on some minute part of the paper. He would try to correct me. I'd always be very patient with George because I liked him, but he would call me and he'd say, "Now, John, this paper is a very nice piece of work. But you have to get away from using this term for this process because it's not anything new. We discovered that many years ago." [laugh]
I would listen. "Yes, George. I understand, George." I was visiting USC a couple of years before he died, and as always, he was very kind to me. He made a special effort to come in. He wasn't feeling well. He died a couple of years later. After I retired it turned out my doctor I was going to had also recently retired. I asked him to suggest some people that I might take on as my new doctor. He suggested a guy named Ronald Olah. [laugh] I said, "Isn't that George Olah's son?" He said, "Yeah. He's a very good doctor." I ended up going to him; I still go to him. He has a lot of George's characteristics too. George Olah still sorta lives for me in a way from his son. Yeah, that was a very nice award. I was pleased. That usually goes to somebody doing heterogenous catalysis, not homogenous catalysis. I think George was impressed that I could do work in hydrocarbons with homogenous catalysts. I should probably close by pointing out that although the award is named for Olah, George was not involved, at least directly, in my selection. ACS demands that all national awards selections are to be made by committee.
ZIERLER: John, to drill down a bit deeper into CH bond activation, something that you wrote about in some detail. You talked about CH bond activation as something that needed to be understood before it was exploited. I wonder if you can reflect a little bit about that? What needed to be understood about CH bond activation? How did you envision that research being able to exploit it?
BERCAW: Yeah. Well, I hesitate to say that we're going to connect fundamental research so quickly to exploitation or application. Some people would argue that a lot of the catalysts, most of the catalysts even, that activate CH bonds were discovered not by understanding the fundamentals, but by just trying different things and seeing what things worked. These people are skeptical that fundamental research really has a place in catalyst development and exploitation. Fortunately, I think they're the minority. Most people think that understanding fundamentals really leads you to application. Maybe not at such a direct line. Maybe not even in a way in which you can explain very clearly and logically. It builds your intuition about what things to try and where to look for a way to get CH bonds to react. The real driver for CH activation is this partial oxidation goal. We know it can be done because Nature does it. Although she does it in a very elaborate and biochemically expensive way. For example, there are a class of enzymes called methane monooxygenases. That's exactly what you want. You want to put one oxygen of the O2 molecule, a monooxygen, into one of the CH bonds to make methanol.
I should point out that Nature must waste the other oxygen to make simultaneously water. These methane monooxygenases are catalysts based on transition metal compounds (iron and copper compounds). It can be done, but the way in which Nature does it doesn't look like it's going to be very easy for us to duplicate. For example, the reason that Nature takes methane and converts it to methanol so selectively is not so much how the detailed chemistry is selected as it is that once it makes methanol the binding site rejects the methanol and expels it from the active site before it can get recharged, if you like, for further unwanted oxidation. Building such an elaborate channel in a synthetic catalyst probably would just be prohibitively expensive. Nature's had a long time to develop these things by evolution. If it works, and it's really required for the organism, it will be duplicated. A synthetic catalyst system has never been built that I know of that has that feature where the channel expels the hydrophilic methanol from the hydrophobic site where it was created from oxygen and methane. We know there is probably some way to do that, but maybe if we keep discovering new ways to activate CH bonds that will build our intuition so that we will speed up the discovery of the catalyst that actually functions well.
They're close. There were some catalysts that actually partially oxidize methane to methanol—this goes back to how I got interested in this. The earliest way we looked at activating CH bonds was with these early transition metals, the ones that are very electropositive that coordinate the CH bond and smoothly exchange it with a hydrogen or a carbon already on the metal by sigma bond metathesis. These catalysts rapidly decompose violently when treated with water or oxygen (or methanol), so they would not be suitable for obtaining our goal. My colleague, Jay Labinger, and I decided to check a report that came from a Russian chemist named Alex Shilov, where he claimed that you could take very simple platinum salts, platinum chlorides, dissolve them in water, and heat them just above the boiling point of water in the presence of methane. This mixture makes methanol. Sorry, not with oxygen. Well, the platinum salts get reduced and not the oxygen, even if it is added with the methane. That's sort of jumping ahead where we try to couple methane oxidation to oxygen reduction. In any case, we couldn't believe that something as undistinguished as platinum halides in water would do something so elaborate as break a carbon-hydrogen bond of methane and add an OH group from water so selectively. We decided to check on that and that's how we got interested in so-called Shilov chemistry. Well, I guess we and others named it that, because he had made these claims in the Russian literature. Initially Jay and I just couldn't believe that it would be true. We thought somehow the platinum got reduced to platinum particles and on the surface there was some reaction that took place like heterogenous catalysts based on platinum metal. We weren't sure exactly what that was, but we just couldn't believe that platinum chloride would could react with methane to do the reaction. Since Shilov hadn't used NMR spectroscopy or any other more modern standard techniques, we decided to check it by NMR. We wrote a proposal for some seed money, and we got that seed money from the department to support a postdoc We decided not to work with methane right away. We'd work with it later. We just tried a CH bond of another molecule like that of a methyl group on a benzene ring. To make it water soluble we put a sulfonate group on the opposite side of the benzene ring. Thus para-toluene solfonate was dissolved in D2O and heated with platinum chlorides and any reaction would be monitored by NMR spectroscopy. We noted it did not appear to [be] precipitating platinum metal, at least not visibly. We could see by NMR sure enough, the C-H bond of the methyl group was getting converted to a carbon OH bond. We were making the alcohol from the methyl group and that was amazing enough.
But even more amazing to a chemist was that if you kept heating it some of the alcohol, the para-hydroxytoluene sulfonate, would be converted to the benzaldehyde. That was a little slower than the methyl group going to the hydroxyl group. It was completely backwards from everything organic chemists thought because it was well known that it's much easier to oxidize an alcohol than it is an alkane. The aldehyde was forming, but very slowly. Then a next level of amazement was that the aldehyde was not further oxidizing to the benzoic acid. We know from organic chemistry it's even easier to oxidatize an aldehyde to a carboxylic acid. There were some really novel things going on here, and we began to be believers that Shilov was correct. We eventually did look at methane and indeed it is oxidized to methanol. We were funded to look at it. I then shifted some of my National Science Foundation funding to support some grad students. We started doing fundamental studies on how exactly the Shilov chemistry took place on all kinds of hydrocarbons. We devised something called the Shilov cycle, which was based on some suggestions in the literature by Shilov. There were three stages of the overall reaction that took place. We studied the detailed mechanism of each of those three stages and ultimately found out why Shilov chemistry works whereas related things don't work so well. I guess we could go into the details, but I think I'll just say that over the years we laid out the relative rates for alkane versus alcohol versus aldehyde oxidation and got quantified data on them.
The surprising thing was compared to the traditional way in which you oxidize hydrocarbons or alcohols, the Shilov chemistry was not very selective. We had oxidized a methyl group at about the same rate you oxidized an alcohol. An aldehyde was much slower. That meant that there was fundamentally something very different about the way in which this platinum chloride precursor was converted into a platinum species that was soluble, but liked carbon hydrogen bonds of alkanes and activated them. We spent a lot of time and money [laugh] and tried to understand the Shilov system. Ultimately we did find a way in which to replace one component of the platinum chlorides; it turned out you needed a mixture of Pt(II) and Pt(IV) chlorides. The Pt(II) activated the C-H bond to yield a Pt(II) alkyl and a proton, and the Pt(IV) oxidized that Pt(II) alkyl product to a Pt(IV) alkyl releasing one Pt(II), and finally the Pt(IV) alkyl reacts with water to form another Pt(II), alkyl-OH and another proton. That's how the cycle worked. The Pt(II) is a catalyst for the alkyl oxidation by Pt(IV) to form alkyl-OH and Pt(II) and two protons. We figured out a way in which we could replace the Pt(IV) chloride by two Cu(II) chlorides, taking some note from a former postdocat Penn State, Ayusman Sen. Here the two Cu(II) chlorides oxidize the Pt(II) alkyl to Pt(IV) alkyl, releasing two Cu(I) chlorides as the oxidation cycle. The advantage of that is that you can reoxidize Cu(I) chloride with dioxygen. Also, Cu(II) chloride does not oxidize Pt(II) chloride to Pt(IV) chloride, which would then deplete the system of the required Pt(II) chloride catalyst; Cu(II) chloride waits for the formation of Pt(II) alkyl from the first step; the alkyl group makes Pt(II) thermodynamically possible. Thus, you ultimately are consuming dioxygen in the water as you're making the methane into methanol. We got several turnovers in that way. We got maybe a hundred or a couple of hundred turnovers of para-toluene sulfonate to para-hydroxylbenzyl sulfonate, before it all shut down. At least in principle, we demonstrated that you could do these reactions catalytically with O2 as the oxidant. You could do them in a way that yields much more alcohol than aldehyde and carboxylic acid, than you would obtain with a traditional organic oxidant system (with which you would obtain little or no alcohol or aldehyde). We were doing something practical on a very small scale.
The problems remains so enormous, because nobody wants to use platinum as an oxidant, because it's a very expensive element; we would need many, many millions of turnovers to approach being practical. Everybody wanted to use oxygen, ultimately, and if we could catalytically do it, great. But doing it on a scale where you would need so much platinum even with this very efficient catalyst to do this is on a scale that would have an impact on how we currently make methanol—it's hard to imagine. I think we have the fundamentals, but the really difficult thing is taking those fundamentals and having a very creative person somehow devise a way to make it work on such a scale and with such efficiency that we would actually replace the way we make methanol now. We take methane—methane is a precursor—and we heat it up with steam and we make carbon monoxide and hydrogen out of that. Then we take the hydrogen and the carbon monoxide, and we pass it over a catalyst. It's actually a zinc catalyst with a copper promoter, a heterogenous catalysts. That catalyst selectively makes methanol in high yield. We make all of our methanol that way, oxidizing methane. We steam reform it first and so we have to heat it really hot and drive that reaction that would go back the other direction at lower temperatures. We drive it at really high temperatures to CO and hydrogen. Then we take it partially back downhill to methanol using a catalyst. It's a very expensive—energy wise—process, but it works really well. Replacing it with something that only turns over a couple hundred times is not on. Nobody in industry is going to do that.
ZIERLER: John, moving into the early 2000s. Tell me about the origins of the MC2 program at Caltech.
BERCAW: Yeah, we called it MC2. [i.e., MC-"Squared"]
ZIERLER: Oh, MC-Squared. [laugh]
BERCAW: The same equation Einstein used to relate energy and mass (C is the speed of light). Here the M stood for methane. Conversion Consortium. That's what the CC was, C2 for short. It was methane conversion. It didn't have to be converted to methanol, but something more useful such as a liquid fuel or a petrochemical. Anyway, it was their name. A group of scientists and managers from BP were told by the CEO at that time, Lord John Browne, who is a physicist, had this vision for supporting academic research that would be through these grand consortia involving BP and a university. Some of them were in physics, some of them other areas, but there was going to be one in chemistry. Lord Browne decided that it was going to be in methane conversion technologies. I think they had in mind that they would identify some university that had people working in the area of methane chemistry, and by working with BP scientists and engineers this consortium would stimulate the development of some of these technologies we've talked about. They came up with a list. I don't remember how many universities they invited, but two of them were Caltech and Berkeley. There were also Illinois and Texas A&M, and others. These universities all had people working in the area of methane chemistry in some way. They came to me and to Jay Labinger and said, "You've been publishing on this platinum work. We're very interested in you. We would like to encourage you to write a proposal to attract these funds to Caltech. We'd like you to identify, at Caltech, people in your department that would be part of this consortium with BP. We organized a group of people; we discussed what we would put in the proposal. We submitted a written proposal to BP. I guess we survived the first cut because they sent a group of about five people to Caltech to listen to our presentations of what we would do with the money. In the end they decided that they wanted to support two schools. One was Caltech and one was Berkeley. The Caltech people were to be the chemists working on the problem. The Berkeley team was to take the more chemical engineering approach to the problem. Berkeley, Caltech, and BP would be the MC2 consortium. We met with BP scientists regularly. They alternatively came to Caltech or Berkeley once a year. We went to a BP site once a year. We talked about our results, and we talked about where we might go next and so forth. That was a wonderful thing for Caltech. It involved not only methane conversion, but at the outset they liked Harry Gray so much they decided that they wanted to do something in photochemistry. Solar energy conversion, I guess I should call it. BP had a small effort in that area, but they thought Harry was making great progress.
We had not only people working on methane, but also on solar energy conversion. That was how it started. It was supposed to last 10 years, and it had very generous funding for 10 years and then it would end. At about year five, we went to BP, and we reported on our first five years of progress. They had a clause in the agreement that they could terminate, if they didn't think we were making sufficient progress. Interestingly, the person at BP that was in charge of the MC2 consortium was my former provost, Steve Koonin, BP's Chief Scientist. Steve's a physicist, and he and I are contemporaries at Caltech. We were on a lot of institute committees together. I knew Steve very well, even before he was my provost. He was the one who was sitting in the audience when we were proving that we deserved the next five years. He agreed with us and agreed that we should have an inflationary increase, so he gave us even more money. Steve was very good to us. Then after 10 years they decided they liked it so much that, rather than terminating the program, they reduced the scope of our problems; Harry's project was shuttled off to NSF support because Harry got a lot of support for his solar energy research program from NSF. BP stopped funding Harry, continued funding people like Jay and me and also Bob Grubbs and my young colleague at Caltech, Theo Agapie. We had about half as many people as we started with, and we got a reduced amount of funding. They continued for another seven years before they ultimately said, "OK. This is the end." I think in part it was because I had decided I was going to retire at about that time. [laugh] I don't think that BP ever had a university program that lasted that long. On reflection I think it was such a hard problem they didn't expect that we would solve it even after 17 years. We certainly made progress, and I think they saw that.
They didn't really hire very many of our people, so they weren't really trying to get Caltech graduates and postdocs to come and work at BP. They were hiring Caltech and Berkeley students and postdocs at the same rate they were before. I think what they wanted to do was sort of outsource their fundamental research efforts in this area so that they would have some group of people working in the area and watching the area closely. If somebody made a breakthrough—whether it was us or some other group—we would know about it early. We would have an opinion of how likely the breakthrough was to be correct. They sort of kept us as their ear to the ground for fundamental research in the area of methane and natural gas conversion to liquid fuels and petrochemicals. I think they felt good about it. They were somewhat torn between whether we should be doing something practical for all this money. "What are they getting for their money?" was what they would occasionally say at one of these meetings. Fortunately, the managers of the program kept saying, "We're going to let you work on these problems that are close to application. But we want you to use your own creativity in taking the program in different directions. We don't want you to just do what we already are doing." I think in the end both the managers and the scientists were quite happy with it. I'm still very good friends with some of the chemists that work at BP and are working in organometallic chemistry. Although they're not supporting me anymore I still keep in touch with them. I'm certain that they were very happy with it.
ZIERLER: John, what would you say at the end of the day were some of the achievements of this collaboration?
BERCAW: Well, essentially all of our fundamental work on the Shilov cycle was done with BP funding. It started out with my NSF grant, as I said, and this little seed money that we got from the Division of Chemistry and Chemical Engineering. Most of the research was done with BP funding. It started from using methane as a substrate. I told you about how we worked out the Shilov cycle. Then we decided that we should really look at ways to make higher hydrocarbons from carbon monoxide and hydrogen. This is a longstanding problem also. I told you that we could make methanol with zinc copper catalysts selectively. If you try to make gasoline from this carbon monoxide/hydrogen mixture there are messy product mixtures, you get a mixture of messy products. It's essentially crude oil. They were interested in making catalysts for more selective conversions. Making something maybe more than just higher alkanes, which is what this process called Fischer-Tropsch does, but making olefins in higher yields and more valuable petrochemicals. We stepped back and said, "OK. We're going to have to use methane and steam reform it into CO and hydrogen. Now what kinds of fundamental processes can we envision there?" We did a lot of fundamental work in that area as well. Toward the end they were also funding work that I was doing with a postdoc or two in this area of olefin trimerization catalysis. I think some of the breakthroughs we did there were of great interest to the BP scientists who were interested in lubricants. Because we showed that we could come up with a catalyst that would selectively trimerize not only ethylene to make 1-hexene, but it would even trimerize things like 1-hexene and make a C18 product, and it would do it very selectively. Or you could make C15 from C5 and so forth. These turn out to be made in industry, but again, you get ugly mixtures by using the way they do it now. Synthetic lubricants like Mobil 1(Exxon Mobil) or Castrol Edge (BP), synthetic motor oils that we put in our automobiles and trucks, are really created by very nonselective processes. Here we were making selectively a C18, which is one of the many components. C18's (octadecanes and octadecenes) are a major component; our C18 is a 3:1 mixture of two octadecenes is minor component. The way they're doing it now you get many, many higher alkanes and alkenes. In the end they were very interested in that work as well, and we've actually written patents in that area. The BP scientists were very interested in having pure samples of these products that we were making to try to figure out what component of synthetic oil was the most desirable, and how we might make only that and not these other things that cause problems. Those were the scope of the projects that we were looking at. They were quite happy to look at almost all of those processes that we thought were worth dedicating a grad student or a postdoc to. That was good enough for them.
ZIERLER: John, tell me about being a Seaborg Scholar at Los Alamos. Was that more like a Harvard one week thing or was that a long engagement?
BERCAW: No, that was a longer engagement. I think I may have been the first Seaborg Scholar. Anyway, you probably have heard of Glenn Seaborg.
ZIERLER: Of course.
BERCAW: He was a chemist who really understood the essentials of chemistry that goes on in a weapons lab where you have to work with radioactive elements like plutonium and related actinides made in nuclear reactors. Glenn Seaborg was involved in the Manhattan Project and was the real chemist in that project. Chemistry has been maintained at Los Alamos National Lab as a discipline that truly is central to maintaining the stockpile and improving processes that are used for maintenance of nuclear weapons, as well as other types of chemistry and biochemistry projects. They're an energy lab as well, so they call themselves a national security laboratory. Thus they research all things related to national security. They look not only nuclear weapons related technologies, but those related to secure energy sources and having independence in energy and having first rate intelligence communities as well as climate predictions, etc. Some of the things that my group was working on were of interest to the people—the scientists, the chemists, especially—at the weapons lab. I was ready for a sabbatical again. I forgot exactly when it was. Was it 2004?
ZIERLER: That's right. 2004.
BERCAW: Yeah. Anyway, I decided I would go for six months. The scientists there, the people who were in the management of the chemistry division—as they call it—were interested in having me there and they said, "We have this money from the Seaborg fund, and we would like to support you." They paid my living expenses. I went over there and worked in an office, talked to the scientists a lot, and worked on some papers of my own. It was a real sabbatical, once I was in familiar territory. The sad thing about it was that after I'd been there about a month—this was at a time when safety was of even greater importance to the national labs than it normally was—there were a couple of accidents that happened in the chemistry division. The director of the laboratory was a retired admiral in the Navy, and he was very determined to make sure that safety was recognized as being really important. He did the amazing thing of shutting down the entire laboratory as a demonstration of his commitment. He made all the scientists and technicians think about safety and write protocols for how to make the lab safer so that no more of these accidents would occur. I was already on site. I had an office. I was not an employee of Los Alamos, but having this appointment I had to sit and watch all the videos at these so-called all hands meetings that the director would call occasionally. He would express his anger with the chemistry division and others that had had security accidents. In the end that I know that the chemists were really unhappy with that situation. I think the accidents were not insignificant. A laser accident with an undergraduate occurred at that time, and they had some stupid, mostly silly accidents in the chemistry labs; their occurrence was magnified. They also had security problems where they thought they'd lost some disks that had classified information on them. It turns out the disks never really existed, so it was just cataloging errors that led somehow to the thinking that the discs were missing.
Everything quieted down eventually, but some of the people that were good friends of mine, including the leader of the chemistry division, was expelled from the lab during that period. When the lab was shut down, the leader of the chemistry division had his security clearance suspended, and his admittance badge to the lab had to be returned. He had to just sit around at home during this time. I played the role as a job counselor in a way because everybody was thinking, "This really stinks. I'm going to go somewhere else." We would talk about things, and I would try to get them toned down a little. It was not as enjoyable as I had hoped. Plus, I had some experiments that we were going to do using a special reactor that they had at Los Alamos built to be explosion proof, so that if something went off inside of it, it would be contained completely. We were thinking about heating to high temperatures and pressures methane and oxygen with our platinum catalysts to see how much conversion we could get under conditions of high concentration of both of those reagents. It was going to be carried out in that reactor which was remotely controlled. Of course, when the lab was shut down nobody could do any experiments. It was shut down for a long time. You don't sort of casually shut down a nuclear facility, because restarting has so many procedures that have to be implemented. It takes months and months and even years to get everything back up fully running again. Which is probably one of the reasons that the director of the laboratory was moved to a desk in Washington and a new director of the lab was put in place. There were other reasons too. Part of the reason was also because the uproar over safety and security at the lab led Congress to decide they needed to have a competition for which organization would be the contractor. The University of California, which used to be the sole contractor for Los Alamos—just like Caltech is for JPL would have to compete.
That's how they ended up adding some industrial supporting management in addition to UC. UC still got at least half of that contract, but some industrial organizations got the other part of it. There was a whole reorganization that came about starting at the time I was there. It never does continue to be exactly like it was, but this was a very major disruption in the management and the stockpile stewardship at Los Alamos. I happened to be there right as it was taking place. They knew I was a member of the Science and Technology Panel, so they asked me to serve on some of the selection committees for some management positions, including Principal Associate Director, and other safety planning committees. Because I was now performing duties very much like what I had left, I returned to Caltech and went back to my labs.
ZIERLER: John, last question for today, a technical question. One of your most significant research areas during this time—Mechanistic Studies of the Ethylene Trimerization Reaction with Chromium. Tell me a little bit about that research.
BERCAW: Yeah. This is what I was talking about earlier. Actually it started out with a report right at the time we were getting this Methane Conversion Consortium in place. BP reported a discovery that chromium catalyzed ethylene trimerization could give one 1-hexene in high yield. They were very interested in that because it was so selective that this might be of some commercial interest. I started consulting with them on that project too, in addition to the consortium we were just establishing. They gave us some funding to do our mechanistic studies of their process. Again, Jay Labinger and I were working with students, one of which was this fellow who is now a professor at Caltech, Theo Agapie. He was doing the fundamental work on how this catalyst might work by identifying what the precatalyst is. We did a lot of mechanistic studies that supported one of the two mechanisms we had been thinking about. Anyway, we worked on that area for quite a while. That was a good half of Theo Agapie's Ph.D. thesis with me. We also had postdocs working on it. It became a very interesting problem.
In the end we were trying to figure out what the true catalyst was, so we did some very careful studies where we showed that when you add the cocatalysts to the chromium complex, there is a series of reactions that occur. On warming from low temperatures beautiful color changes occur—from dark blue to red to green and then to a pale blue color, as it warms up to room temperature. At the time nobody really knew which one of those (if any) was the active species. It had to be beyond the blue one, because that's the color of what we started with. When you added a cocatalyst it immediately went red. Is that the true catalyst? Or does it have to go to the green thing or...? We were doing not only the visible studies of this system, we were also performing what is called Electron Paramagnetic Resonance studies. EPR studies, and importantly carrying out simultaneous ethylene trimerization rate measurements. Ultimately, we came to the conclusion that the chromium system was very complicated, but we could say with confidence that neither the blue nor the red nor the green nor the pale blue species was the catalyst. [laugh] It was there in at most only a few percent, and it was not visible by any of those spectroscopic methods we were employing. In other words, that catalyst activity started at low temperature and was maintained all through all of those color changes and EPR changes that we saw.
The conclusion is the true catalyst was doing all the "heavy lifting", and it is never present in more than small percentage of the chromium concentration. There had always been some thinking in the catalytic community that if you see the compound by some technique it's probably not the catalyst, because the catalyst is so active it's probably a very minor component of the whole thing. That was certainly true in our system. We were totally frustrated at that point with the chromium system because we could not observe by any technique the true catalyst. Thus, we decided to follow up on a study that was done in Japan at the chemical company called Mitsui. We studied their titanium catalyst, so we're back in titanium world again, like in olefin polymerization. I'm sure the Mitsui people found by accident that a certain ligand system around titanium gave only only 1-hexene and not polyethylene out of ethylene. 1-Hexene is a lot more valuable than polyethylene, so making 1-hexene very selectively was attractive. In fact, their catalyst system does makes a little bit of polymer, depending on how pure the specific titanium catalyst is; you don't want polyethylene. You want to minimize the polyethylene and maximize the 1-hexene that you get. We were studying that catalyst in great detail, and we made some real progress for that system. We found that you could support it on silica, and if you supported it, it gave much higher activity and made much less polyethylene. The chromium catalyst led us to study a titanium catalyst for which we could follow the process of trimerization in an NMR tube, the catalyst itself. We could actually see the precatalyst, and we could see what things were formed from the precatalysts and follow the reaction by NMR. That was very nice.
ZIERLER: John, that's a great place to pick up for next time where we'll talk about your work with the DOE on catalysis.
[End of Recording]
DAVID ZIERLER: OK. This is David Zierler, Director of the Caltech Heritage Project. It's Monday, November 1st, 2021. Once again it's my great pleasure to be back with Professor John Bercaw. John, as always, it's great to be with you.
JOHN BERCAW: Nice talking with you too, David.
ZIERLER: John, today I'd like to talk about your work with the Department of Energy and the Basic Energy Sciences Advisory Committee or BESAC. Just by way of context, can you talk a little bit about what BESAC is and how far back your work with them goes?
BERCAW: I'm not sure I was ever officially a member of BESAC. Is that in my CV somewhere?
ZIERLER: No, but you did the work with catalysis with the Department of Energy—in catalysis.
BERCAW: Right. What does the acronym stand for? The Advisory Committee, right?
ZIERLER: That's right. Basic Energy Sciences Advisory Committee.
BERCAW: Right. I guess I was a member for a couple years. There's also a science board that's appointed. It's separate from the Advisory Committee though. Yeah. I only served for a couple of years. I think it's a rotating business. I remember now having some meetings where we tried to encourage formation of symposia to highlight progress in areas that we thought were important such as alternate energy basic research. We also discussed among ourselves where we thought there were opportunities for directing funding and so forth in the portfolio. I was also a member of some sort of review committee for the Basic Energy Sciences, and I thought that's what you were talking about. That was separate. We went into the offices of the program directors and looked at various, sort of at random to look at their programs and to see how the program officers were performing and reported to the secretary. I guess it was not really the Secretary of Energy. It was the person who was in charge of Basic Energy Sciences at the DOE. I've had several advisory roles, but the BESAC really wasn't, in my opinion, a big highlight. It was just a group of about ten people who got together once a year for about three years and then the committee changed. Sorry.
Catalysis and National Energy Policy
ZIERLER: John, can you talk a little bit about catalysis and its relevance for energy policy in the United States?
BERCAW: Yeah, I can. I was on some of those workshops or symposia that we organized. We had to write—and I helped write—a very large document that pointed out where catalysis was benefitting basic energy sciences, goals, and so forth. We pointed out that catalysis could be the way you improve a lot of very polluting processes that are practiced on enormous scales. We studied what—we somehow got data from—I think from the Department of Energy—which processes were especially polluting. These are very high energy processes like making ammonia, doing methane steam reforming into carbon monoxide and hydrogen, and then all these other processes that use heterogenous catalysts, especially petroleum refining. All of these processes require very high temperatures and pressures and therefore consume a lot of fossil fuel just to make the processes go thermodynamically or kinetically. Some of these processes are thermodynamically favorable. For example, fixing nitrogen. We talked about that. Fixing it to ammonia using dihydrogen. It's downhill, and if we didn't have to heat it so hot and raise the pressure so high it would be a lot greener process. The same can be said for Fischer-Tropsch process where CO and hydrogen are combined to make hydrocarbons, but we were encouraging Basic Sciences to lead the way to new and improved catalysts.
Of course, we should be realists because the energy companies are very motivated to do the same kind of research. Although they generally don't do basic science underlying the development of new processes as we've talked about previously. These companies generally invent catalysts by trial and error. They have some idea of what to try, but exactly the conditions and so forth—they have to work out by trial and error, not only by applying fundamental principles of basic science for each of the steps. There is sort of a complimentary role of academics, especially, and government labs and others who are doing the fundamental science and not necessarily the science that's close to development into a practical process. I might go back a ways and talk about my NSF program director because he was very much hands on and liked to distribute his funding to places where people were working on fundamental steps, mostly in academics, especially supporting those who were working on these kinds of projects that involved catalysis with the ultimate goal of making practical, less polluting catalysts. Even back when I started getting funded from NSF, he would sponsor workshops where we all got together to talk about these same issues.
This was a commonly done thing back then. Not so much these days, but we do occasionally have these kinds of workshops where we talk about what are the promising avenues to pursue that will lead to processes that are much less polluting and much more attractive to society in general? I remember talking with him and I said, "Why do you have so much of your funding going into projects that are all related? In my view, people are sort of competing with one another to make the big breakthrough and to get more funding, or have the satisfaction of developing a practical catalyst." He said, "I like to have people in my program competing with another." It's, in my view, a way to get progress to go forward is having people talk to one another, not competing in secret, but openly talking about their results and where they're going with a program and so forth, so that we can get the maximum progress toward these catalysts." The program officers themselves actually had a very powerful influence on how basic science was performed in academic labs and that was true in the Department of Energy as well.
ZIERLER: John, what were some of the political backdrops at the Department of Energy at this time with regard to catalysis? Was there a recognition that we needed to be as smart as possible with our remaining fossil fuel supplies?
BERCAW: I think that opinion was relatively quietly expressed. It wasn't the main driver. One of the areas was the nitrogen fixation area. There were international symposia I attended where people went from other countries as well, would get together, and discuss progress for understanding how nature fixes nitrogen and how synthetic catalysts were being developed and how they might work and so forth. Although they were far from practical. There was funding going in and these program officers from DOE and NSF were going to these international symposia and listening to talks and digesting how that might translate the conclusions to their own administrative role in distributing funds. It wasn't as nearly so much in the minds of the researchers and the funding agencies that we needed desperately to do this because of issues like climate change and fossil fuel limitations. In the nitrogen fixation area, I remember that people commonly had first used slides that always pointed out how many people were starving on the planet and how much we needed to provide fertilizer for improved crop yields. It was more feeding people and not so much aware that we were using our fossil fuels at a phenomenal rate, that they weren't going to go on forever, and that we needed to quit polluting.
Those things weren't really discussed in the 70s and early 80s, at least. I think everybody in industry realized that we had limited fossil fuel reserves, but they would sort of begrudgingly say, "Well, we have very large amounts of natural gas. Enough for 200 more years, and then it'll all be gone." Then in another five years you'd hear them talk again and say, "Well, we have enough natural gas reserves for another thousand years. Then it'll all be gone." People would raise their hand and say, "Wait a minute. Why do we have five times more at this point, whereas, we should be using up what you said was 20 years' worth." He would just laugh and say, "Well, we're really good at discovering new gas wells." The same is true of petroleum. We've been close to running out of fossil fuels for a long time. I think that people think that's not going to be the problem. We're not going to run out. We're going to lose the planet due to climate change while we still have fossil fuels sitting around. There's sort of a complicated role that society and the public play in raising awareness to these issues. I think most of us in science believe that if we really wanted to fix a problem badly enough, we would have to prove that it was something that was worth the money to do it. That's a hard one to prove. We pretty much think that we can do anything we want. We put two guys on the moon a long time ago, and that was something people just wouldn't believe could be possible prior to them actually achieving it.
I think we can fix these problems that have to do with better energy sources and saving the planet, if we really put our mind to it, but I don't think the public are ready to pay the price for that. This means the increased costs of fossil fuels, so that we can do the research that's needed and the engineering that's needed to do all this. We still have gas to bridge the transition to other energy sources, and the fundamental science for developing some way toward longer range solutions. It's not like going to the moon. That was more of an engineering feat than it was a fundamental science feat, although the fundamental science provided the instrumentation and all of the rocket engines and so forth that they used to get there. It's some combination again of fundamental science and engineering. Energy has become, especially in the last 10 years, I think, something that everybody is really getting very concerned about.
ZIERLER: John, around this time you're spending a lot of work with advisory committees at National Labs like Berkeley, Argonne, Livermore, Los Alamos. Just at a broad level, what was the expertise that the National Labs were looking for? What was your input on the things that were happening at the National Labs at this time?
BERCAW: Well, I think it was multiple in the sense that they wanted some distinguished scientists and engineers to come and listen to their own lab members who were giving research talks about what they were doing. They also wanted us to listen to the management tell us what their strategic plans were for pursuing the projects that they were pursuing and giving them some feedback on the quality of the science and the appropriateness of the science that they were employing to try to address the problems that they identified. Most importantly, they were worried that they were missing something at the lab, that there was some science going on somewhere that they should be a part of, because it really could be crucial for making progress in the areas they identified. In all of those areas we were given a lot of respect. We were a panel of about 12 people from most areas of science and some areas of engineering. The directors of those laboratories came and welcomed us, told us, "Come on. Give us some candid feedback on how we're doing and what we need to do better."
They would stay with us for two or three days. Sometimes they wouldn't sit through all of the scientific presentations, but they would be available to answer our questions. They would tell us what they were emphasizing and so forth, what the status of the lab is, how the funding of the lab was changing for the better or for the worse and so forth. I think fortunately, they didn't want us to go stomping into Washington and try to convince Congress or their staffers that they should be funding this or that laboratory at a higher level. It would've been a conflict of interest, in my view. Occasionally, they sort of hinted that they wanted us to be advocates for their labs when we talked to people in Washington for other reasons, but I told them that I thought that was a conflict. I personally didn't like that, but it came pretty close to happening at one of the labs. I won't mention what lab it was, but I remember we were hearing from the principle associate director that advocacy should be one of the roles for these advisory committees. I remember my stomach sinking when I heard him say that. That was just so inappropriate. When the committee got into private discussions among ourselves, they all agreed with me; they had the same opinion. That issue went away fortunately.
Although at the very end of my service on that lab's committee, we had a couple of years where we went to Washington to have discussions with the people who were providing the funding. We were careful not to advocate, but we wanted to hear their side, because we always heard the lab's side of the problems they were having with funding certain projects or areas. We wanted to talk to who we called the customers. The customers were largely the Department of Energy. People would come and sit and talk with our committee and tell us what they thought of the labs. [laugh] The labs weren't real happy about that, but they went along with it, and I thought that was a very nice compliment to the possibly biased view that we got at the labs. They didn't want to put their average scientist or engineer in front of us. They would have their very best people talking to us, and we had to seek out the other side of activities. There were a lot of roles that we played. Sometimes we got in arguments with the lab management, even the Director.
We would say, "You are making a big mistake here by changing the lab in this way." Sometimes they would get pretty put out with the committee, and especially a couple of us members that were most outspoken. To their credit they didn't say, "Well, you're not going to be a member of this committee anymore. We're tired of hearing you." They listened to us, and they even put up with us when we got angry with them. I think it's a very good thing. I did it for essentially no money. They paid my travel expenses, and for most of the meetings I got a minor honorarium for serving, but it was something that I felt obligated to do, because it was something I thought I was pretty good at. The lab scientists would tell me they really liked having me on the committee, and I told them that I enjoyed serving. I felt that that was a way I could serve my country, and I think most of the people on the committee felt the same way.
ZIERLER: John, I'm curious with Los Alamos and Livermore…it makes sense why with Berkeley and Argonne they would be working on energy issues for which your research would be relevant. With Los Alamos and Livermore was there a national security dimension at all to what they were doing that you were providing input on?
BERCAW: Yes. Los Alamos and Livermore made the point very clearly at the very beginning of all their presentations that they were one of the national security labs. They weren't just maintaining the nuclear stockpile. Although that certainly was where most of the funding went, to make it possible for the lab director to send a letter once a year to the POTUS, the President of the United States, that says, "These weapons are functional and will be available should you need them." That letter was mandated by the NNSA to give the President confidence if he needed them. I was very impressed that they really took that charge to heart, but it grew over the years to be more than just nuclear weapons security. It was energy security because we can see from our dependence on certain countries, especially in the Middle East, that if we continue to use petroleum in the ways that we're using it, and if they cut it off it's going to be a major national emergency and threaten our national security. I think that case can be made very easily. They expanded their research portfolio and started calling themselves a national security lab, not a national weapons lab. That did cause occasional friction between DOE Basic Energy Sciences and DOE National Nuclear Security Administration funding of projects in fundamental areas of energy. Occasionally the scientists from the weapons labs that tried to attract DOE BES funds for their projects were rejected by program officers from BES that felt there should be plenty of funding from DOE NNSA overhead (Laboratory Directed Research and Development). The NNSA labs received tens of times the amount that BES labs did. Occasionally they were successful in attracting funding for programs that looked very much like BES ones in the BES labs, and some chemists, especially at Los Alamos historically received their major funding from BES.
Also, Los Alamos and Livermore thought it didn't sound very good to the public that you're a site for weapons labs. When the committee got into discussions about individual projects, you immediately got into secret, limited access topics. We all had to have Q clearances to maintain our position on these advisory boards. All of the discussions were not secret, but when they did go classified they need you to have the secret clearance, and if you did not it was a real pain. Dealing with a renewal every few years took pages and pages of documentation that I had to provide. For every place I went to deliver a lecture, I had to provide a letter of invitation and answer questions about why was I going there and so forth—even when they weren't really what we call sensitive countries. That was the downside. The good side was I quickly found at Los Alamos and Livermore that the best science was in the classified areas, and I would never have heard about those projects, if I didn't have clearance and serve on these committees. It really made a very positive impression on me when I listen to what they were doing in the classified arenas. Not just in nuclear weapons research, but in the intelligence areas and the surveillance areas that this country is involved with. I enjoyed my time there. I think I told you early on in these discussions that I was pretty much the only chemist on the boards. They really relied on me when they presented chemistry projects. They asked me what I thought of that science and how it compared with best outside Los Alamos and Livermore.
I guess I was on it for a very long time. I think when I left Los Alamos, I was the longest serving member of the committee. I had a lot of experience. When you first start going there you're just overwhelmed with the complexity, and you can imagine all of the areas that you're not really expert in. You have to learn about enough and how all of the projects are connected to each other to actually have an impression. It takes several years to get into that mode where you have some confidence. We have these review committees that came in, and one of the roles at Livermore and Los Alamos was for the members of these advisory committees to work with the lab management in putting together the membership of these visiting committees, to suggest possible members, and to try to convince people to serve in that role. I remember some of my friends that came in and looked at the chemistry division at Los Alamos. They would say, "I can't serve in this role. I don't understand what they're talking about." They're very prone to using acronyms for everything, and if you don't know what an acronym specifies you don't know what the heck the topic is that you're supposed to be listening to. I said, "Well, we don't expect you to know all that. We want you to learn as you go along, so for your first year you're not going to be very much help to us, but after a few years you're going to be a wiser person and everybody has to work their way through that." It is a complicated role that the external committees play to the lab science, but I think it's crucial that they have this open view to a committee, that all the committee has top secret clearance so they can talk to them pretty broadly. Although I should point out that they never tell you more than you really need to know to do your job. I think that's a very good position, but I never felt like they were holding back. If we had a question they would go deeper. They might go and ask their boss to see if it's OK they talk about something, but they seemed pretty responsive. I was impressed. I think the national labs are a gem in our research programs.
ZIERLER: John, I'm curious at Argonne, or more broadly at the University of Chicago, if you ever interacted with Tom Rosenbaum before he came to Caltech?
BERCAW: No. I did not. I didn't meet with the physicists at Argonne nor did I talk to them at Chicago. Of course, I did my postdoc at Chicago, so I knew about the University of Chicago, and I returned there a couple times on academic visiting committees for the Department of Chemistry there. I was familiar with Chicago, but I think I was not made familiar with the types of projects that Tom was involved with when he was there. I talked to him at Caltech when I was on some committee, and he was sitting next to me. When we had a break I remember talking to him, and he asked me where I was from and I told him, "Chicago." He asked me who I worked with and I said, "I was a postdoc with Jack Halpern." He said, "I know Jack." Jack was still living at that point. He said, "I know Jack very well. He's a special guy." [laugh] I said, "Yeah." When talking about it he asked me where I lived; I lived right across the street from where Tom's labs were. I'm not sure I ever overlapped with him when he was still just a professor there before he started moving up the administrative ladder to provost. Anyway, Tom and his wife, Katherine, are very friendly and very easy to talk to. I would've been happy to talk to Tom when he was at Chicago, but I didn't have that opportunity.
ZIERLER: John, you served in an advisory role for external committees in the Department of Chemistry at top-flight programs like North Carolina, Yale, and Harvard. What did that give you, sort of with a bird's eye view, of what was important in academic departments in chemistry at this period—the most important lines of research, the most important issues in terms of hiring and graduate students? What did you get in terms of understanding what was going on in the world of chemistry at this point?
BERCAW: Well, you're right. I served on many, many visiting committees. The one at North Carolina I chaired. It was a big committee of about 15 people. We were concerned about their department and the fact that like many of them, the young people were fewer in number than they should've been. The department was therefore aging. We made some recommendations that the Provost took very seriously. They made a whole bunch of hires of young people after that. One of them is a former student of mine who's very successful, and he's tenured there. Viewing it from the other side at Caltech, for example, we were a little bit intimidated by the visiting committees, because they were meeting with the President and the Provost at Caltech and getting their marching orders more or less about what the administration at Caltech wanted to hear about in their report to the Provost and President. We worked very hard at making presentations that would show that we were aware of a lot of the issues in the department, and that we were working on solutions and so forth. When I was on the other side, when I was a member of these visiting committees, generally we were impressed by the quality of the science that we saw. We talked to the students. They knew that we wanted to talk to the students and get their impressions, see about how happy they were with how things were, whether they were missing something being at that department, or whether they were feeling like they had a really good opportunity to do well in the department and so forth. We got a lot of feedback. We got feedback from the untenured faculty. There is always an issue about how much mentoring is appropriate from senior faculty to the young faculty, that will get them going down the correct path toward promotion but not guide their programs?
We got a lot of inside information, and I guess I felt like I had my own opinions about how things ought to be. Caltech's chemistry department is either the best or tied as the best in the country and the world. I felt like I had made a contribution toward building that department, and so that's why they wanted me to be on the committees, because they wanted to know what Caltech was doing and why they were so successful. It was nice in the sense that I saw friends and made friends and saw young people when they were just beginning, and all of those acquaintances really make you feel like you're part of a big community of scientists and academics. I enjoyed being in that community, and that was the way I could get out of my office and away from my research group for a couple days and mix it up with some other people.
ZIERLER: John, a question I've been looking forward to asking you for some time now. Tell me about your visiting professorship in Saudi Arabia at King Fahd University. First, how did that opportunity come about?
BERCAW: [laugh] OK. I'll be perfectly candid. It was frustrating. [laugh] That's my general impression. It came about because King Abdullah decided to pour a lot of money that they had from all of their petroleum resources into building a new western style university, KAUST (King Abdullah University of Science and Technology). It's in the west of the country on the Red Sea. He also poured a lot of money into other universities. One of them was this King Fahd University of Petroleum and Minerals, where they started well-funded programs to look more like the western countries, like in the U.S. and Europe. One of the programs was at this—KFUPM is the acronym for it. They wanted some university in the West to collaborate with KFUPM that would be a model for how they should develop their new program that he was encouraging. They had a lot of money. It was incredible. KAUST was given so much money, they just couldn't spend it all. They were buying instruments; they bought many NMR spectrometers that cost $2-$3 million. Places like Caltech would have one or two or three of these, but they had about 10 or 15 of them, and initially they didn't have anybody who knew how to run them. They bought tens of these inert atmosphere glove boxes that I had two or three of, and most of my colleagues around the country had two or three of them. They had as many as 50 of them. They didn't have any use for all of them, but they had to spend their budget, so they bought a bunch of them.
It didn't seem like they were being very wise with their money to me. They looked for young scientists around the world that they could fund. One of my colleagues, Brian Stoltz, was made some sort of KAUST scientist or a research fellow or professor. He had enough money in this reward to fund his whole program. It was supposed to be, I think, for 10 years, but it turned out after a few years they started to come to their senses and realize these people didn't need that much money. In the beginning—being at Caltech—I was part of the university that KFUPM decided they wanted to be partners with. A member of the advisory committee for the KAUST board, the university that was going to be the hub of all of these various research programs that were supported through KAUST as the hub, was my colleague, David Tirrell. Being at Caltech, he convinced them to consider Caltech's chemistry and chemical engineering department as being the place that King Fahd University ought to partner with. He convinced Bob Grubbs and me and a couple of other scientists at Caltech and a couple of engineers to consider participating in his collaboration. It provided a lot of funding, more than I really needed for the projects we were doing. We spread it around amongst various people, but part of the agreement— and I think sensibly, was a requirement—that we would go to King Fahd University, and we would look at what they were doing and try to get them aware of how they could do better. We flew for the first time, Bob Grubbs and Theo Agapie and Zhen-Gang Wang from Chemical Engineering and I flew together. I'm not sure Zhen-Gang went on the first trip.
The rest of us went to King Fahd University, and we were met there by the person who was in charge of the Institute for Petroleum Research that we were going to collaborate with. He was our main organizer. Anyway, we discussed what we could do, what fields that we could work in, and it turns out that one of the areas they were quite interested in was polyolefin research because, of course, places like Aramco and many of the petrochemical companies in the Kingdom of Saudi Arabia were making lots of polyolefins, and so they were very interested in doing some fundamental studies in that area. That was my field, of course, and a collaborator was selected to work with me. He and, it turned out, his technician were doing experiments that I watched and then made suggestions for improving things. We had three or four years of the program before we eventually lost this funding for what was called the Center of Development at King Fahd University. KAUST was cutting back everywhere. My colleague, Brian Stoltz, lost his fellowship. I think he only had like a year to go back to regular funding, so it was not very easy for him to transition back to what he was doing before KAUST. They didn't really care much about that. They didn't really seem genuinely interested in changing very much at King Fahd University, in my view. Although I should point out that every time we went there, the Rector and the Vice Rector spent at least an hour or two during the visit talking to us about how things are going, how they were doing, were they really improving? I think they genuinely wanted to do the right things, but the next level down was where I have a lot of issues with them.
I actually went over there in the second year and spent about two weeks there; I also took one of my graduate students. I think I spent two weeks, and he spent three weeks there living in the apartment buildings that they put their faculty in. This was in the middle of the summer which was really hot as hell. [laugh] I remember we had to have a driver pick us up and take us to the lab and take us back to our apartment. There wasn't really very much to do ever. The country is just not a happy place, because it's so conservative, and they just don't seem to be having a lot of fun. [laugh] It was so hot that they told us right away, "If your air conditioning breaks you need to call. We have 24-hour service. They will fix it immediately because it's a matter of life and death." It got above 45 degrees Celsius there and that's just incredibly hot. The scientists were not really willing to keep the equipment running that we agreed we would use in collaboration. I don't know what they were doing, but I sensed that they weren't doing very much other than teaching their courses. We were so different. I wanted to work in the lab, and these people didn't wanna really be in the lab all the time. I said, "Is it OK if I take a key and I let myself in and go work in the lab with my grad student?" They said, "Yeah." We had to walk from our apartment six blocks when it was ungodly hot. The labs were air conditioned, but anyway… Their research programs were not really of upmost importance to them. After several frustrating experiences KAUST discontinued KFUPM as a Center of Development. It turned out that King Fahd University of Petroleum and Minerals was very happy with what Grubbs and I and Theo Agapie and Zhen-Gang Wang did. So they continued our collaborations using Ministry of Education funding. We would report to the Vice Rector, who was also the Director of the Research Institute at KFUPM.
Most of the work that was going on was going on at Caltech with my postdoc who was, being paid from our grant. Not very much was going on in Saudi Arabia. We had success at Caltech, and I think my collaborator was very happy. The first time around my collaborator was very happy, and even the second time another collaborator seemed happy. I would coauthor papers, and we even co-invented a US patent, that was quickly allowed by the patent office. The Vice Rector of the Research Institute where all these experiments were conducted, was always trying to talk Bob Grubbs and me into becoming adjunct professors—I'm not sure what the title implied, but that's effectively what it was—so that they could take credit for all of our publications and the so-called h-index in the citation database, that would increase their standing in the international community.
This got to be a very hot button item, especially in the US, because some people would agree to be adjunct professors, and they wouldn't even visit the universities in Saudi Arabia. Some of the Saudi universities would take credit for all of their research programs because they were adjunct professors. They would pay a reasonable portion of your salary to do that. After a few years the apparent ploy by the Saudis came to light in a Science article that got a lot of attention. Bob and I resisted doing that all along, and I didn't want any more involvement than I already had. Things began to come to an end for me when I sent many samples that were prepared in my lab, some of the them, when I was working in the lab by myself. I would send samples, and I would be very interested in the results. Saudi Arabia had instrumentation for characterizing these polymers that I made, but these instruments were always broken. They kept saying, "Well, we're going to get it fixed in the next couple of weeks." Six months later it would still be broken, and again I would complain bitterly. I told them that we're not making any progress, because there's a big waiting line for you guys to run these samples and to interpret the data and give us some of your feedback to the program. I was very frank. I told my collaborator, "You know, I don't think you're really part of this program because you don't think about it; you don't correspond with me." I think he was shocked that somebody would be that frank with him, but he didn't run away. We wrote a couple of papers together, and he was happy with that, but I had reached my limit. I think I must've gone to Saudi Arabia five or six times for regular meetings that were invariably held in Saudi Arabia. They did not want to come to Caltech. The main reason, at least what they expressed to us, was they were afraid to get on an airplane as a Saudi citizen. They would be harassed and discriminated against, they thought. I didn't see that happening, but maybe it did. In any case they wouldn't come to even some middle ground country like the UK. They didn't want to do that. They wanted us to make the effort to come and do this over and over again.
I eventually resigned my part of the program. I couldn't in clear conscience continue to participate. Theo Agapie continued; Bob Grubbs continued. After that, our main contact resigned from King Fahd University and went to work at a petrochemical company in Saudi Arabia. He didn't even tell us. [laugh] We had to hear it through the rumor mill, and when we tried to contact him he wouldn't respond. It finally ended.
That's what I mean by frustrating. I remember when I was thinking of participating in this first stage of it, this Center in Development. It was when my colleague, Ahmed Zewail, was still alive, and I talked to him about it because he was an Egyptian, and he was very familiar with the Middle East and the countries there. He was also a main advisor to KAUST. I said, "Maybe I'm a total romantic, Ahmed, but it seems like I could go there and make some real difference, at least for one person. If I could just make one person excited by opportunities in chemistry and get him working on something important, it would be worth it to me." His reply was not very optimistic. He said, "I admire you for wanting to do that, John, but I think you're going to be very frustrated." [laugh] He was right, of course. Ultimately, at three or four years later, I realized that it was very sad situation, because there were some Saudis that were very excited about their projects, but they too were very frustrated. They couldn't order chemicals because the Saudi government was worried about drugs, starting materials for making drugs, alcohol and things like that. They were worried about everything to a fault, and the bureaucracy was just incredibly cumbersome. The scientists that were trying to do some experiments could not get the reagents to make the molecules they needed. You had to order them a year ahead of time. If you have that kind of lag time for when you conceive some experiment and when you can actually perform it, it's just not going to be possible to make good progress. There were a lot of reasons why I was frustrated. Plus, I thought some of the Saudis, when they took us out to dinner, were interested in very strange topics like talking about women and race matters and what would I do if my daughter married a Black man? These questions were just totally inappropriate. It was like they were in a boarding school, and they never really grew out of that and became real adults.
ZIERLER: [laugh]
BERCAW: [laugh] Grubbs came to that same conclusion. It was a very awkward thing. I think that it's sad because I felt like when I worked closely with my first collaborator's technician and showed him how to do experiments that he didn't know how to do, he was very appreciative. I knew how to do it with my hands, and they were amazed! What is this professor from Caltech doing in the laboratory working with a technician showing him how to do experiments? I didn't care about their reaction, but it was an astounding show that the rest of the laboratory was pretty much amazed by. The technician thought I was a magician, because I could tell him what was wrong with his glove box, why it wasn't working properly. I could show him how to handle other air-sensitive things using the proper techniques. His experiments started working, and he was very happy and so was his boss. I felt like I was making progress, but it was not possible for me to spend a lot of time over there showing everybody how to do experiments. They needed to have some visitors come to Caltech, as Bob and I repeatedly told the Rector. They actually did send a couple of postdocs to our labs for a few months. But culturally it was a disaster because the one that came to my lab was immature. He brought his wife along because he couldn't have lived without his wife looking after him. He didn't work well with my lab members because he was special, you know? People should wait on him and do experiments for him and things like that. In addition, some of his work areas were so cluttered, making for an unsafe working environment, that I had to go to him and threaten to ban him from the lab until he cleaned un his messes. There were cultural issues that just got in the way with everything. I'm glad that stage of my life has ended. I'm sorry that I didn't have the patience, I guess, to stay with it a little longer, but I didn't. I don't know. I guess I'm too aware when I might be taking something that I haven't really earned. I didn't feel like I should be getting any more funding from them, so I ended it.
ZIERLER: John, meanwhile back in the United States the American Chemical Society recognized you with a string of awards—2013, 2014, 2016, and 2017, in that order. The Tolman Medal, the Gibbs Medal, the Kosolapoff Award, and then the Gábor A. Somorjai Award for creative research in catalysis. Tell me a little bit about, from a broad perspective, what it was that the American Chemical Society was recognizing you at this latter point in your career, right at the point in which you decided to go emeritus?
BERCAW: Yeah. That's a hard one for me to answer. I think there are a lot of senior people that get multiple National Awards from the American Chemical Society. Some of those awards like the Tolman Medal is not a National Award; it's a so-called Section Award. That was true of the Gibbs Medal too. That was the University of Chicago's section that gives that award. They're very prestigious awards and previous winners were certainly people I admired. I think there's an inevitable tendency for these selection committees to choose people who are distinguished and have accomplished a lot. That usually comes with age. I think it was just sort of my time for a lot of these awards to come on. I've won a several ACS National Awards, and I can't tell you why. [laugh] There are certain people, like Harry Gray and Bob Grubbs, that have won more than I have. Part of the reason is that ACS has established a large number of new National Awards in the last 20 or so years. So those of who have already won the long-standing National Awards are now being considered for the newer ones, too. Winning one award was a big, big deal for most people in the United States, and winning two was unusual. Winning five or six National Awards, is just a rather rare thing. I guess I'm happy with it. But now the number of people winning more than one or two is growing, too. Maybe people think I'm a nice guy, so they don't have a hard time picking me for another award. I don't know. [laugh]
ZIERLER: [laugh]
BERCAW: I can't help but think that it's something like that. [laugh]
ZIERLER: John, around this time one of the more historical or historically minded articles that you wrote with Jay Labinger—Mechanistic Studies on the Shilov System: A Retrospective— I wonder if you can talk about the Shilov system and why you were motivated to take a historical or retrospective approach at this time, in 2015?
BERCAW: Well, back when we were interested in activating carbon hydrogen bonds, you might recall, Jay and I were sitting around trying to think up a project that we could submit a proposal to our divisional funding for getting started on. We both decided that the Shilov system that Alex Shilov had published on—it was several decades before we were thinking about it—had seemed too good to be true. We decided to check it, and when we found out his observations were reproduceable, and after we had a lot better opportunity to investigate the mechanism than he did, we concluded that he did pretty well with the techniques he had. I knew Alex Shilov before we started looking at the platinum chemistry. When I was just starting at Caltech, we had a collaborative program with the Soviet Union. [laugh] This was in the ‘70s. In fact, I spent July 4th, 1976—that was our bicentennial celebration as a country, riding on a train from Moscow to Kiev with some other Americans going to a joint symposium with Soviet scientists. [laugh] I was supposed to collaborate with Alex Shilov. Shilov not only invented this platinum system for activating CH bonds, he was also interested in nitrogen fixation. I think it was the National Academies of Sciences that encouraged the establishment of this collaboration with some Russians in the area of catalysis. John Baldeschwieler, who was the chairman of the department at the time, asked me to collaborate with Alex Shilov, and the kickoff was to be at a symposium in USSR. That was where I first met him. We flew to the Soviet Union. We started in Moscow. I met with Alex and his wife at dinner in the Sputnik Hotel, and I remember we were talking science on the napkins. [laugh] Alex Shilov's wife, I think her name was Alexa Shilova. was a very good chemist that was working in Alex's labs. Alex had published in the area of nitrogen fixation, using some titanocene compounds. I'm always someone who just speaks out without thinking, and I said, "I remember this paper that you published, Alex." I don't know if I had a photocopy with me or not. I said, "But this one equation can't possibly be true, because I know from my own work that the reaction would not work as you describe." Alex was able to speak English very well, but his wife could not speak English at all. She could speak French as well as Russian. There we were, there's three languages and three of us without a common language. Since she couldn't speak English, I would tell Alex these things, and then he would ask his wife to explain that equation, because I guess it was based on work that she had done that they had published jointly. I remember she listened to his Russian version of what I just said, and she was very animated when she replied. Alex translated her Russian to English: "No! It is not correct!" "This is stupid. How could we have published that? That's not right; he's correct." [laugh] Alex was put in this awkward situation, but he handled himself really well. He was quite cordial throughout the rest of the evening.
He came along with some other Russians to Caltech as part of this program. I remember going out to dinner with him. They were all very nervous coming to the U.S., and especially going out to dinner and to a nightclub with us. Harry Gray was there; some of the other Russians were there. Alex was convinced that there were KGB officers walking around watching what we were doing, reporting back; he was so nervous. None of that ever happened so far as I ever heard, anyway. Even before Jay and I started reproducing Alex's work, we both were aware of Alex. Jay was not yet at Caltech; he was a postdoc at Princeton. He was aware, as you might recall reading the article, that Alex had come to Princeton as part of this collaboration. Alex and I both spoke. Alex spoke about his CH activation chemistry at that symposium. That was many years before we started working on his system. We both ran into him at a couple of other meetings, because he was apparently a secure enough member that they would let him visit the western countries to go to scientific symposia regularly. Jay and I had met him after that encounter at a meeting somewhere in the Armagnac region of France, I remember that. We had met him a couple of other times. When he passed away we felt like we had lost a friend, not only a peer scientist.
We agreed to write an article for a special issue of a journal that one of his Russian colleagues organized, and we wrote a remembrance. Jay and I were often asked to contribute a scientific article, and we would rewrite some of our papers on the Shilov system, try to include some more recent experiments. That got to be harder and harder as time passed. In the last few years we've written joint remembrances of some of our friends. We have begged off from sending in a scientific article, and explained, "We'd be happy to submit an article in this case where we talk about the Shilov system and its importance and the amazing reactions that are promoted by this system." They agreed, "Sure." That's how we ended up honoring Alex. I think he was 84 when he died. He was about ten years older than I.
ZIERLER: John, around this time you came to the decision to go emeritus in 2015. Tell me about the timing of that decision. Why then?
BERCAW: Well, there were a couple of motivators. My wife has been interested in rescuing dogs and placing them in forever homes over many years. We had been looking for a second home for about five years, and we finally located a ranch in northern Arizona in 2007; we named it Red Dog Ranch. Increasingly, we spent time with our pack over at RDR, and it was taking me away from my labs when I spent time there. I thought that wasn't going to work in the long term. That was one motivator. Another was I was just reaching a point where I was ready to stop the parts of my job I didn't enjoy. You know, dealing with committees and funding and all of the things that go with those committees where you have to write reports. At this point I had worked with about like 150 graduate students and postdoctoral fellows. Most of these people were writing me asking me for letters of recommendation. They needed multiple letters, and sometimes they would want to relocate, and I'd have to look at what they'd done and write a new letter. I've written so many letters; my administrative assistant (of about 50 years!) told me, "If you are ever at a meeting and someone walks up to you and introduces themselves and you say one word to them, they're going to ask you for a letter."
ZIERLER: [laugh]
BERCAW: [laugh] Now, she and I have to sit down together and go through all of the letters that I've written for so many people, and mostly throw them out. We have hard copies in filing cabinets. There are five or six drawers in each one and there must be 10 of those, at least, sitting in Noyes Laboratory locked up. She's not exaggerating that. I still have several letters I have to write for people. Some of them I never worked with directly, but they're in the same field with me and they need an award, or even another award. I try to support them. Other people are trying to relocate. I still do a little letter writing, but I was doing a lot at the end of my career with all of that buildup of people that have worked with me. The people that were working with my former students and postdocs who were in academics were also writing me and asking for letters. It just went on and on. That kind of thing was sort of burning me out, I guess is the word. It was also getting to be time when I should think about whether I was going to retire at Caltech, using one of the incentive programs that Caltech has in place. It was when Ed Stolper was Provost. He used to regularly invite especially senior colleagues—from all divisions around Caltech—to have coffee with him once a year. I had coffee with him when I was thinking about retiring. [laugh]
I remember clearly when I said, "You know, Ed, I'm thinking I might want to retire in the next couple of years." He said, "That's terrific! Great! I'm glad to hear that." I said, "Well, that's not very nice to say, Ed." [laugh] He said, "No, no, no. I don't mean it that way. I mean you have a lot of respect around the Institute, and if somebody as respected as you retires that would make other people think, well, it's OK to retire. You don't have die in the saddle, as they say." He was very happy, and he said, "You should take advantage of these programs. You can learn about them in human resources." I sought out some of that information from them, and I decided that the optimum time for me to retire was in 2015 when I was officially 71. There were some financial incentives, so everything came together. I warned my students far enough away, and I quit taking new graduate students so that I would not have any students when I retired. I told my postdoc fellows that they would have to be thinking about finishing up. Although I could have a postdoc, that wasn't so much a problem with timing. In fact, I had postdocs still working with me when I had retired, and I was in the lab working with them alongside me. I'd always wanted to go back to the lab and do some experiments again, because I knew how to do a lot of things in terms of procedures, and I had a lot of anecdotal information. We had these special techniques that I was teaching to new people when I was active, but I wouldn't really have the opportunity to do very much of that if I didn't have any graduate students. I like to go back in the lab and get things working and perform experiments, the aspects of research that made me love chemistry. Experimental science is a lot of fun.
That's why it all sort of came together. I never really thought about whether it was a good idea or not. I think it was. I've sort of evolved. The pandemic made it more difficult for me to do experiments in the lab. That part of it was a disincentive about being in Pasadena, because I wasn't encouraged to go to the lab. I wasn't told you can't go in the lab, but—and I did go into the lab occasionally following all the protocols that were in place at the time. It just wasn't the same place for the last year and a half or two years. I went to lunch three or four weeks ago with my colleagues in inorganic chemistry, and everybody's teaching again. They were commenting on how it seems like the old Caltech again; it's coming back. Maybe I'll go do some more experiments. Who knows?
ZIERLER: [laugh] John, of course—
BERCAW: I still have a lab.
ZIERLER: As you say, going emeritus does not necessarily mean going inactive. In fact, one of your most significant papers came out a year after you retired was on co-supported tandem catalysts. On that point, what were the topics after you pulled back a little in research that was most important for you to stay connected with? To continue writing to maintain your collaborations even in retirement?
BERCAW: Yeah. Well, like you said, the tandem catalysis was a new process for making linear low-density polyethylene from ethylene only. This was a combination of polymerization catalysis and trimerization catalysis that took place by two separate catalysts that were supported on the same particle. I guess I liked that work because it was that kind of area that required vacuum lines. I liked working on my vacuum lines. It required that we work in glove boxes also. I still think the trimerization catalysts and the polymerization catalysts are some of the most amazing catalysts I've ever worked with. They have turnover numbers, the number of monomers that will be turned over in the lifetime of a catalyst site, that are just mind boggling— approaching a million. There aren't too many catalytic processes in the world that operate with that kind of lifetime. If you think about it, one of the amazing things about olefin polymerization catalysts is that they're so active that you don't have to remove the spent catalyst from the product. It's something innocuous like titanium and aluminum. It's there at the part per million level, which means it's just not concentrated enough to have any consequences on the properties of the polymer or the safety of the polymer.
Anyway, I was still amazed by the activity. I mean, you can just watch it working by the pressure change that occurs when it is exposed to ethylene. It's just amazing. I still remember working with Theo Agapie's student, a lady named Meaghan. She was absolutely ecstatic when her first polymerization was going on my vac line, and we could watch the manometer, with mercury column rising up a glass tube in real time? She was saying, "That is incredible!" She was screaming, and I said, "That's what's got me hooked too." That's why I went back and started working with her, because I had something to teach my coworkers, and it was an area that I really loved.
A New World in Chemistry
ZIERLER: Well, John, now that we've worked up to the present in all of our discussions, I'd like to ask now some broadly retrospective questions about your career, your activities as a professor and mentor to students, and asking you to reflect on some of the broad trends in chemistry over the course of your research career. Let's start first with the teaching. You know, working with 150 graduate students is an amazing thing to have accomplished, all of the letters that you've written for these students. I wonder if you could reflect on a broad level thinking about some of your true standout graduate students and all that they've gone on to accomplish? What are some of the similar character traits that you see in your most successful graduate student? What does it take to succeed nowadays in a career in academic chemistry?
BERCAW: Well, it's a different world than when I started out. I think I made it clear that I was very fortunate in that I got funding, and I got support from my colleagues, and I got promoted without any kind of hassle. None of that's true anymore. Many of the chemistry faculty, but especially Harry Gray, Bob Grubbs, Peter Dervan, Jackie Barton, and I have been very fortunate at Caltech to have so many graduate students and postdocs go into academics. It's also true in chemical engineering. Dave Tirrell told me that Caltech places professors in chemical engineering like no other school in the country by a factor of 10, I think, on a per faculty member basis, People may ask, "Well, why is that true?" All of us have often wondered why this is true? I have concluded that it is because Caltech has a special atmosphere where it's truly fun to be an academic at this institution. Nobody would dispute that. I think it's the best job in the world. Harry Gray says it is so—and I don't dispute that. I think that it's only natural that our students and postdocs, when they're at Caltech working with us, see that we're having a lot of fun. It's not easy to hide that you're having fun, and for that matter when you're not having fun. You know, we laugh at each other. We all have big egos, but we're able to laugh at each other and have fun with each other. It looks attractive, I think, to students and postdocs. So, they aspire to be in a profession like a Caltech professor. Especially in our fields of organometallic chemistry and inorganic chemistry as well as bioinorganic and bioorganic chemistry. Those areas were very lively and continue to be very lively areas in terms of research efforts. It was nice that they had jobs to go to because people wanted to add faculty in those areas. I look back and a lot of my former students, they won national and international awards. One of these days they'll be members of the National Academy of Science; I'm confident.
But it's a different world. When I was starting I supported my entire group on one NSF grant. When I talk to my students and postdocs who are going into academics I say, "You know, you're going to have to do something different than I did when I started out in terms of getting funding." The system has changed. I had what's called an Individual Investigator Research Grant from the NSF, and those are not very common anymore. The National Science Foundation, for various politically motivated reasons, has put many Individual Investigators into a common research thrust. They call them various things. Centers for Chemical Innovation, Chemical Process Clusters, and many other terms for joint grants that the various agencies have. They have a key lead person and lead institution, and then there are professors from universities around the country, and in some cases even from other countries, that are all part of the same research thrust. The funding goes through the principle investigator out to all of the various other universities. It ends up that an individual would get more or less what they would get in terms of dollars if they had an individual investigator award. But they have the added responsibilities that take time away from performing their research programs: justifying that what they're doing is benefitting the program to all of the rest of the members in that joint program. They have to all work together. There are some advantages; I can see that. There's no longer an individual investigator atmosphere because the individual investigator grants are much less than they used to be. They have to become affiliated with several of these, and thus they have to be part of lots of different areas.
I look around for example, the guy at Caltech that worked with me, Theo Agapie. He's got funding from several sources within Caltech—the Beckman Institute, the Resnick Institute, and it goes on and on and on. Some of them are outside of Caltech. He has to juggle all of these things and keep lots of principle investigators and program officers happy. It's especially burdensome now for somebody who's working in organometallic chemistry, because the area's been around now for more than 50 years. I didn't have any difficulty at all in attracting lots of graduate students and postdocs to my program. Some of them were the very best people that we brought in those years. Thus, I was getting the best students, and in many cases, they were smarter than I and they were working at a very high level.
I just had some wisdom on ‘em and a little bit of maturity. I benefitted from all of those things, a big advantage that's no longer true. I look at the research proposals when one of my students or postdocs is sending out to apply for jobs. When I see some of their research projects are in organometallic chemistry, and I say, "I can see that the review committees are not going to look very favorably upon that. You might have one of those research proposals, but you'd better have something that's more in the areas that are getting lots of funding so that you can run a program wherever you're going." It's sad that they can't work in the area they are so in love with, organometallic chemistry. They have to figure out some way to sell it to the applicant review committee and to the funding agencies as being related to something that's green or sustainable research, especially something that's related to medicine and biology. Because those are the areas that are really getting funding. You can just look at Caltech and see where we're spending lots of money on new buildings and enhancing existing or new departments within Caltech. Most are in the bio areas, especially, and not so much in the catalysis and organometallic chemistry areas. We're happy to have those people, and I recognize the need to pursue timely new areas. But I also think that we can justifiably say that professors working in organometallic chemistry train scientists to think beyond organometallic chemistry, to look at an area and identify opportunities and formulate an approach that has impact if you succeed at it. That doesn't depend on the area; that's really something that you teach by example and by working in a research group where that activity goes on and you can identify with it.
So, I was really fortunate having this magnificent program in an area that was really hot when I started. It was still hot when I left, but other areas have surpassed it. I'll say it that way. [laugh] I know some of my students would go to seminars and listen to all of this salesman type lecturing from people who were working in areas described with catchphrases that really irritated them, like "bioinspired". That was the one that repeatedly set off one of my students. He came out of there just fuming, saying, "That's complete nonsense." [laugh] It's a reality you have to live with. You can have your own opinion, but don't let sales people affect you too badly.
ZIERLER: John, what opportunities have you had to interact with undergraduates over the course of your career at Caltech? Either through teaching undergraduate courses or including some of the really talented undergraduates in your laboratory?
BERCAW: Yeah, I've had a lot of opportunity. I did work with undergraduates to a fairly large extent. Early in my career I taught freshman chemistry to Caltech students. I saw them the day they walked in. I taught the various laboratory courses in chemistry for many years, and I got to see students there as well. I would visit the labs—they had a teaching assistant, but I would visit as the professor and talk to them individually, and in that role I could recognize really talented people. I would encourage them to apply for a SURF Award in the labs. My commitment was a modest amount. These students would then go on to work during the academic year with my group, and attend a graduate school usually outside Caltech. Especially in chemistry, we encouraged students who were undergraduates at Caltech to go somewhere else to pursue a Ph.D.. They'll broaden their perspective on chemistry working with faculty outside of Caltech. I had undergrads in my lab. Most of them turned out to be quite good. One of them is the wife of Theo Agapie who he met working in my lab and was her mentor. She's now a professor at University of Southern California. She's just an example of how people who were undergraduates at Caltech end up somehow interacting with Caltech forever. [laugh]
Not all undergraduates, I've had in my labs were cut out for experimental chemistry. For example. I remember one person in particular. I won't say who he is. He was supposed to do an experiment in my lab which was relatively straightforward. I told him to go order this chemical from a commercial vendor, and he did and when it came. I said, "I want you to go in the lab and distill it and take the center cut—the center cut is a very large fraction in the case of a commercial sample—the part of it that boils at the right temperature and the impurities will then be decreased in concentration and so forth, so we can go forward with it. He came back to my office and said, "Uh. There's none of that compound in there. It's supposed to boil at 120 degrees Celsius. It all boiled at about 35 degrees, so there's none in there." I said, "Wait a minute. Go try it again. I think something's wrong with your setup." He came back and said, "The same thing happened when I did it again." We went down to the lab, and I looked at his distillation glassware. He had a Vigreux column attached, and it was all safely assembled. It was distilling over while we watched, and so I looked at the thermometer and sure enough it was reading about 35 degrees. Yet when I was leaning in the fume hood, I could feel the infrared radiation coming off of the glassware saying to me that it was hot, sort of like hot coffee. I looked, and he had taken one of these thermometers that had a ground glass fitting that went into the distilling head. You can buy a short stem and a longer stem thermometer. He had taken from the drawer a short stemmed one and stuck it into the ground glass fitting on the distilling head. He should've had a long stemmed one. The mercury bulb of the thermometer was not in the stream of hot chemical vapor going into the condenser part of glassware. It's like it was just barely getting warm from all of the 120 degree vapor going into the condenser. I said, "Look at that thermometer bulb. Where do you think it is?" He said, "Oh. Why it's not down where the vapor is…" I said, "Alright, put your hand on the Vigreux column"—a column where the distillate vapor goes up and it condenses and it revaporizes several times, making it more efficient.
He touched it—"Ow!" He burned his hand (not badly). I said, "Do you think that's 35 degrees? He said, "No." I said—I forgot his first name, but I said, "You know, you're really trying, but you have no common sense at all. You can look at this apparatus and not ask yourself questions that are important. I'm afraid you might hurt yourself in the lab. You should try some area other than experimental chemistry." He was sort of crestfallen, and I didn't see him again. He left my group. He wrote me a letter about 10 years later, and he said, "I just wanted to tell you how important your advice was to me when I was frustrated and trying to do experimental chemistry. I switched to physics. I was very successful in that and I've built a wonderful career in physics. And it probably wouldn't have happened as quickly or easily if you hadn't been in my life." Although not commonly I did head off some people that were in the wrong area, and I guess I felt strongly enough to tell them something that they needed to know about their possible future in chemistry. [laugh] Most of the time they were really talented and they worked really hard and they fit in with the graduate students and postdocs and went to all the parties and the group backpacking trips and so forth, like all the rest of group. The distinction between graduate and undergraduate once they got in your research lab was not so obvious. They were just a member of the group.
ZIERLER: John, a really important point you made earlier in reflecting about your external advisory work for other chemistry departments. You noted that there was something special about Caltech's Department of Chemistry. I wonder if you can reflect on exactly what was the secret sauce of the department that made it so successful? How much of that was unique to the department itself? How much of that was because this department was in Caltech and Caltech is primed for success more generally?
BERCAW: Well, all those things are true. I think the most important part of Caltech's special sauce—I think the special sauce has all these ingredients you mentioned—is the people. I'm not talking only about the President and the Provost necessarily. I'm talking about everybody, and the respect that they all have for each other. Also, the informality of the place is just unique. There are a couple of other chemistry departments that have an informal atmosphere, but because Caltech has all these other advantages of being small and select, everybody respects everybody else. Because everybody's good. Talking about Saudi Arabia…like before I ever heard about Saudi Arabia and their chemistry and so forth, there was a delegation that came to look at Caltech, the buildings and the equipment and talked to the people to some extent, especially the President and the Provost. Several of us were invited to lunch with them so they could meet a couple of the current faculty. This delegation's leader said in some remarks after lunch, "We like Caltech very much. We want to buy one." [laugh] "We want to build a Caltech campus in Saudi Arabia." Actually and amusingly, this King Fahd University of Petroleum and Minerals looks a lot like Caltech. In fact, it was designed and built by someone who had interacted with a JPL employee. I forgot who it was, but they did build a Caltech. My mouth fell open and someone said—"You can build the buildings and buy all the equipment you want, but establishing this group of people is not something you can buy.
You have to nurture it and do things that aren't related directly to money. You have to realize that Caltech is great because Caltech has great personnel. It has great leadership. It has great students and great faculty and great staff. All of these things make Caltech very special." Going someplace as a member of a visiting committee and recommending to them what they can do to improve—it is easy to say, but it's difficult to implement, because getting them to change culture is almost impossible. They try to do it, but some of them enjoy being in a very stuffy and scholarly place like some of the Oxford college campuses, you know? Oxford is great. It has great traditions. I enjoyed being there and going to High Table, but it was a little bit too much. [laugh] That's not Caltech. Some of the Caltech faculty go sit on the lawn and eat a paper bag lunch with the students and mix it up that way. That's the great part. It really is the people, the most important thing.
ZIERLER: John, we've engaged in great detail on a technical level of all of the research that you've been involved in. I wonder if you can reflect at the broadest possible level all the research that you've been involved in? What has given you the most satisfaction in terms of your contributions? I'll ask you to think about that in two ways: 1) your contributions as they relate to basic science, simply understanding how the chemistry works and 2) your contributions in applying that knowledge to all kinds of issues in modern society—in industry, in technology, in energy. What has given you the most satisfaction of all that you've worked on?
BERCAW: Wow. Well, I don't think that I can point to one process or one product that I can claim enough credit to single out. I mean, these things develop with a lot of different contributions from a lot of different people, and I don't think I was the key person on anything like that. I think that my contribution to the machine of research and development has been the students. Training students has been the most satisfying thing for me and seeing them go out and succeed the way the students and postdocs have succeeded is wonderful. I'm amazed at how clever they are. Anyway, I just get more and more proud of them, when I see how successful they are, doing different things than what they were doing with me. Training people to go out and be creative and to realize their full potential is something I've aspired to. In some cases I think I've done pretty well at that.
ZIERLER: And to flip that—
BERCAW: But there's not a Bercaw process out there; it is probably fair to say that I've contributed mostly to uncovering some of the fundamentals of organometallic chemistry. I was at a meeting recently and listening to some people that are quite senior in my area. I have a couple of good friends of mine. One is Bob Bergman at Berkeley. I've mentioned him. There is also another is a guy named Maurice Brookhart who is a good friend of mine at University of North Carolina. In their lectures at the meeting they referred to the Bercaw-Bergman-Brookhart trio as "The Three B's". They explained some aspect of a reaction mechanism as being part of "The Three B's" of fundamentals. They were complementing us as a legacy, "The Three B's." We brought this stuff to the rest of the world of organometallic chemists, these fundamental areas of organometallic chemistry and the individual parts of it that they can point to as being part of "The Three B's." Being one of the "The Three B's" is very satisfying. I am pleased that I've made enough fundamental contributions that I could be included with those other two guys that I have such great respect for.
ZIERLER: John, to flip that question around, what are some of the great unanswered questions in organometallic chemistry? What are some of the things that you've spent so much time on and yet there's still so much foundational formative work to be done?
BERCAW: You know, I left areas because I didn't have any new ideas that I thought would make a breakthrough. Areas like nitrogen fixation, like Fischer-Tropsch chemistry, like methane conversion technologies. All of these areas have very difficult challenges in terms of finding ways to get over a major part of the process. I'd move on to another area where I thought I could make progress on until I come up with a better idea. I think it's good that you have enough sense to know when it's time to fold ‘em or to throw in the towel, and move on to some other area where you can make more progress. If I had persevered and managed to get over those difficult barriers, it would still take a lot of work from scientists outside organometallic chemistry to make it just an absolute slam dunk killer technology and change the way the world really operates. But all of these areas that I've worked in are still important areas that need to be worked on, and that there's going to be a way to do this, I think. We figured how to perform some of the needed chemical transformations, but what we did was not very practical on the enormous scales required, but that's the best I can do, I guess.
ZIERLER: John, one last question looking to the future. It's been a theme in these discussions that you've expressed a sense of both good fortune and even wonderment that for your research, just in terms of the chronology, you were so lucky to be there right at the beginning of all of this research. For the next generation of chemists—graduates, undergraduates, even postdocs—what are the things that you're looking at today in the field for which 40, 50 years in the future they might look back and say they were so fortunate to be at the origins of the field circa 2021? What's going on in chemistry today that you think are going to make for really tremendous research for the next generation of chemists?
BERCAW: That's the million-dollar question. I'm not sure I have an answer. I admire the courage that some of the graduate students and postdocs have when they leave my group or some group where they work in an area similar to what I do. Some go into something totally new, and they make a major contribution. One of the areas that one of my students got into when he left for a postdoc—he was a grad student with me—he went into the area of light emitting diode displays, like computer screens and smartphone screens and so forth. He went into the fundamental areas of a totally different thing than he worked on with me or his postdoc. Now most of the android smartphones use his chemistry in the display. He is Mark Thompson, a world class scientist at USC, University of Southern California. With me he developed the scandium organometallic chemistry I have mentioned. It's hard for me to guess what the next area like organometallic chemistry is going to be.
But the basic ideas were all his. He took some inorganic chemistry concepts and applied it to this issue of light emitting technologies and increased the efficiency in a very simple way that wasn't obvious to anybody before he actually did it. Then everybody goes, "Oh! That's what we should've been working on." I'm sure there are lots of areas like that that are out there, but I don't claim to be the person that can see that broadly and with that much judgment to know which one's going to be like organometallic chemistry.
ZIERLER: But you can say with confidence that you might not be sure what it is, but whatever it is it's certainly out there to be discovered.
BERCAW: [laugh] OK. I guess I can agree with that.
ZIERLER: [laugh] Well, John, on that note it has been a great pleasure to spend all this time with you to get your insight and perspective in the fields of chemistry and Caltech. I'm so glad we were able to do this, so I'd like to thank you so much.
BERCAW: Well, thank you for taking time to do this.
[END]
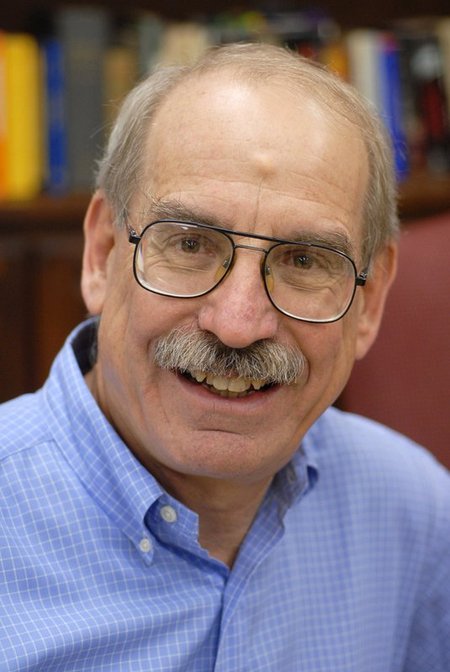
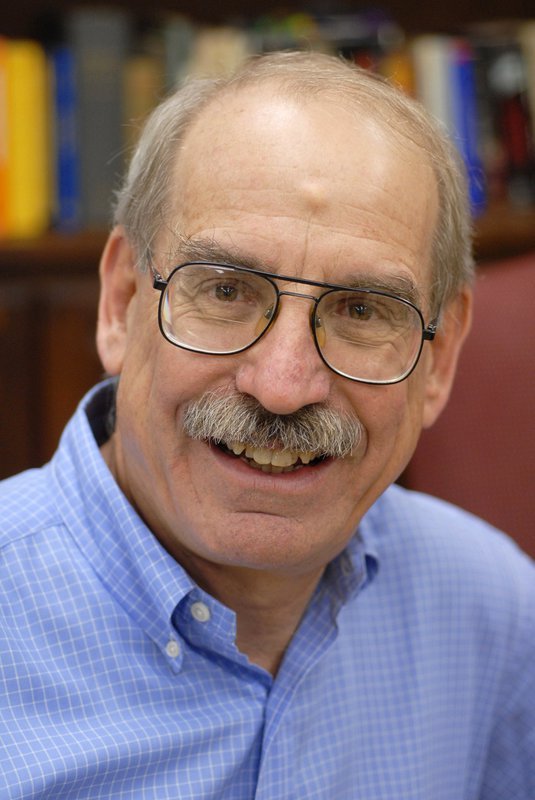