Michael Gurnis, Computational Geophysicist and Seismology Research Leader
When geophysicists say that the theory of plate tectonics is settled science, this does not mean that the entirety of the motions and mechanics of the Earth's surface and interior are perfectly understood. As in all science, theories become accepted when phenomena are observed that accord with hypothesis, and the primary value of this interplay is that it allows researchers to pose yet new questions, often aided with advances in technology that further refine and improve the original theory. In this sense, no scientific theory is ever complete or perfect; it is at best a snapshot that represents the vanguard of scientific knowledge at a given time, and which is falsifiable in the face of compelling new evidence. The work of Mike Gurnis, who came of age professionally when plate tectonics was a mature and universally accepted field, perfectly embodies this fundamental characteristic of science: his embrace of computational geophysics, begun in the early years of supercomputing, provides a new window on geophysical processes at both the local and global scale in the pursuit of understanding the most basic questions in plate tectonics and its relation to the Earth's mantle. For Gurnis, the universal acceptance of plate tectonics is a starting point for making ever-more sophisticated models to understand the interior of the Earth and the geological composition of tectonic plates.
Gurnis came to geophysics and seismology by way of physics and planetary science as an undergraduate at the University of Arizona. For graduate school at the Australian National University, Gurnis pursued questions about the dynamical relationship between upper-mantle convection and whole-mantle convection. This work impressed upon him the centrality of Caltech's Seismo Lab in defining and answering the most fundamental questions in the field. As a postdoctoral scholar at Caltech, Gurnis became more convinced of the value of computation for geophysics at a time when parallel computing and supercomputing were proving their value across a range of scientific disciplines. At the University of Michigan, Gurnis joined the faculty and continued work on the interplay of mantle convention and plate tectonics, and he conducted important work on the initiation of subduction, motivated by one of the central mysteries in plate tectonics: how do plates form? Gurnis's recruitment to return to Caltech as a professor of geophysics allowed for his foundational collaboration with Jeroen Tromp, who preceded Gurnis as director of the Seismo Lab. In the discussions below, Gurnis reflects on Caltech's institutional embrace of cutting-edge technology and why the Seismo Lab was so well positioned to build on its historic leadership while simultaneously leading geophysics to a new computational era. In his role directing the Lab, Gurnis expresses particular pride in the successful recruitment of its newest faculty members who, he asserts, are doing some of the most impressive geophysics research anywhere.
In addition to his duties as director of the Seismo Lab, Gurnis also directs the Schmidt Academy for Software Engineering, and he is animated by a desire to encourage students to see computation's value for fundamental scientific research. His tenure directing the Lab is second only to that of Don Anderson (director from 1967-1989), and as Gurnis notes, the administrative responsibilities invariably pull time away from the research. In the future and at the right time, Gurnis looks forward, once again, to become more immersed in the research and working with a broader swathe of applied mathematicians and computer scientists, because the most fundamental scientific problems demand expertise drawn from wider and wider areas.
Interview Transcript
DAVID ZIERLER: This is David Zierler, Director of the Caltech Heritage Project. It is Friday, July 29, 2022. I am very happy to be here with Professor Michael C. Gurnis. Mike, it's great to be with you. Thank you for joining me today.
MICHAEL GURNIS: Thanks, David. It's wonderful to be here. I'm really looking forward to this conversation.
ZIERLER: To start, tell me your title and institutional affiliations here at Caltech.
GURNIS: My title is the John E. And Hazel S. Smits Professor of Geophysics. I've held this position for more than a decade. And I'm also Director of the Seismological Laboratory. But I'm also Director for the Schmidt Academy for Software Engineering, which is a new program at Caltech in software engineering. It's not part of computer science, it's an experiment at Caltech so that people who do computing, like myself or other people in geophysics, can essentially connect up with software engineers. It's sort of a training program but a science program, and it's Institute-wide.
ZIERLER: Let's start with the named professorship. I'm aware of the way the Smits have been so generous to Caltech over the years. Do you know if there's any special connection, any interest that John and Hazel had to geophysics in particular?
GURNIS: I don't know. That's very interesting. What I do know is that one of my colleagues, Jess Adkins, here in GPS, also has a Smits chair, and he has the baby one in the sense that this chair that I have is a gift that was given to Caltech, and apparently it did well enough that they were able to split it into two chairs. And this second chair is called the Smits Family Chair. Interestingly enough, Jess is somehow connected with the Smits family in some way. It's a relative of his, and I don't know exactly who, his great uncle or something like that.
ZIERLER: An administrative question. I've come to appreciate and learned to translate the idiosyncratic way Caltech organizes itself, where the division chair is, what an executive officer is. As Director of the Seismo Lab, where does that sit in terms of administrative responsibilities within GPS more broadly?
GURNIS: It almost primarily exists within the GPS division itself. It's classified as a center or an observatory underneath the Division chairman. Decision-making on who is chairman or not is done at the divisional level, although all administrative appointments, like the ones I have, were all approved by the provost and the other division chairman. The actual mechanics of who's going to figure it out comes from the chairman. The other appointment I have comes from the provost. Administratively, I talk to the chairman for this position. And it's kind of funny, because the Seismo Lab has existed for so long–we existed before the division existed–it was initially totally autonomous for a period of time. Then, when we moved to campus, the association between our academic programs and research things were totally integrated with each other. In some sense, you could never pull geophysics out from the Seismo Lab.
They were always really one and the other. These days, it's a little bit different. We do have what's called an option representative in geophysics, and all the faculty members we have in the Seismo Lab have appointments within the GPS division. It ends up being maybe 10 people or so. And there are two faculty members who also are in the EAS division. One, Nadia Lapusta, her primary appointment's in EAS, but she has an appointment within GPS, so she's in the Seismo Lab and a very active member of the Lab. Then, Jean-Philippe Avouac even sits in our division, in the geology program, and he's an active member of the Lab as well.
ZIERLER: Who would be the relevant executive officer at the department level within GPS for the Seismo Lab? Or is that really not relevant?
GURNIS: It's not relevant. That's me, the director. All functions of the Seismo Lab, in some sense, get delegated to me, then I delegate to someone else, and if I make a mistake, my head's on the chopping block by the chairman.
ZIERLER: Just to give a sense of your administrative responsibilities, where a provost might barely be able to retain a research agenda, division chair, a little more so, how much of your week is spent doing administrative stuff and not science?
GURNIS: It goes up and down, but on average, I would imagine that administratively, I probably spend up to a third of my time on administration. It seesaws between the Seismo Lab and the software. Those two compete for my time. Then, the other part of it is just my normal stuff, being a researcher, a mentor for graduate students, and teaching, as well as all the normal academic stuff that one does, committees, reviewing papers, writing letters, etc. I don't know if it really is that amount. Some days, it's just a pain. "Give me a break." Because I'm supposed to be a scientist. Even today, I have three meetings with students, and that will probably end up being almost a third of the day. Today was unusual, I was behind on a number of administrative matters, I was traveling to New York earlier in the week, and that took some of my time away.
ZIERLER: On an official basis or more unspoken, is there an understanding that there are term limits to the directorship of the Seismo Lab?
GURNIS: Well, I'm appointed by the board of trustees. Even though I say it comes from the chairman, center directors are recommended to the provost, then the provost and IACC discuss it, probably rubber-stamp it. The letter of appointment comes from the president on behalf of the board of trustees. They have set limits. If my appointment ends, there has to be a little formality. I have to either resign officially or be asked to resign. It's an appointment with a term. A typical appointment at Caltech for administration, it's almost always five years. That's what they want. Then, they'll renew, sometimes for a second five-year term. But I've been doing this more than a dozen years, so I've been asked to continue. They keep adding on.
ZIERLER: You think you have one more term in you, or this might be it?
GURNIS: No, I love the Seismo Lab. I think it's absolutely wonderful. But I've been doing this for a long time, the second-longest serving director after Don Anderson, and there's a new generation. I'll tell you, we have two young seismologists, and they're absolutely the best two young seismologists in the United States. I've never seen anything like it in my life. Being here at Caltech, being in the seismology program for most of my life here, to see that we've been able to find, recruit, and retain the two top seismologists in the country–and that's my own assessment. But I've seen a lot of people over the years, and I've never seen people with their abilities.
Where Geophysics and Software Engineering Meet
ZIERLER: Tell me how the Schmidt Academy for Software Engineering got started.
GURNIS: Interestingly enough, it got started in very different ways. It was a top-down administrative matter, where the president, Tom Rosenbaum, and Eric Schmidt, a very prominent gentleman on the national scene in matters related to American science and software, the adult in the room who made Google the behemoth it is today, were having a discussion at Caltech with the other faculty, and he has a special passion and belief that academia is going to miss the boat in computing. One of the components of that is doing software engineering really well. One of the things that happens in science, and the Seismo Lab is good at this, but in all areas of science, you've got a creative, incredibly smart person who has this idea, they put it into computer code, and they program.
Many of them are self-taught. And their software is often transformative. But that software is not sustainable, it'll break, it's brittle, no one will know how to use it in the future. And all scientists all over the world are facing this issue. Caltech has this program, and they brought me in because I've been deeply involved with software engineering, and I ran an earlier center for the National Science Foundation that did this for geophysics, so they knew that as an applications scientist, I believed in this and had the skillset to start an entirely new center from scratch and bring it up to speed. It's a whole other conversation, but it's an experiment. We're starting our fourth year on Monday, and it's going like gangbusters.
ZIERLER: If I had to guess, the Schmidt Academy, you would think, would be directed by somebody in CMS. Why you? Where's the area of expertise, where might be some of the intellectual connections with geophysics and seismology?
GURNIS: Well, it's a managerial position. I've managed large software projects before, built software centers before, been deeply involved with developing pieces of open-source software with engineers and teams of engineers, so I had all this experience. And then, there's just the passion to know, as an applications scientist, how critical this is. If you're a computer scientist anywhere, but especially at Caltech, you've got your research agenda, and just like in geophysics, there's some future thing you're aiming for. Computer scientists invent new concepts and algorithms for new ways of doing computing. Software engineering, in some sense, is to take the tried-and-true computer science and push software for it, so you can do the cutting-edge applications science. It's the applications that are the cutting edge, not necessarily the computer science. The administration decided they wanted to have an applications scientist. And this is actually run out of the provost's office, not out of CMS, and it makes things a little bit awkward.
The bottom line is, the administration apparently wanted an applications scientist to do this, and given I was probably one of the faculty members on campus who was known to be passionate about this topic, and also had the administrative skillset specifically in this area, they asked me to take this responsibility on in addition to doing the Seismo Lab. And of course, they were aware it would dilute my time spent at the Seismo Lab. But overall, it's been good for Caltech. At the Seismo Lab, we have a big team of software engineers, four or more, involved with the network operations and earthquake early warning. And all the graduate students program. For the students, the software engineering skills need to be a little bit improved within the Lab, although we did that before, but we need to start doing it all over again, I think. It's kind of odd that since Caltech being such a small place, that you have to do things like this.
ZIERLER: As an experiment that's already going so well, what do you see as some of the long-term prospects of the Schmidt Academy, and what are you hoping to see yourself?
GURNIS: The prospect is, I do want it to be sustainable. When I talk to faculty members around campus, one of the things I'm able to do with the senior staff is to identify and recruit people who have just gotten their bachelor's degrees. And at this time, we've been only recruiting from Caltech and Harvey Mudd, which is a very special little college to the east of here. Before, they would rank the different schools in terms of SAT scores. Caltech had the highest SAT scores in the country. Second was MIT. Third was Harvey Mudd College. Then, you go to the Ivy League. In any event, these kids are really smart, as are the Caltech kids. The Schmidt's wanted us to pay top dollar to get these folks. We've been able to get incredibly smart and talented people coming out of computer science programs and bring them to campus here, mentor them in software engineering, then the science teams around campus mentor them in a science problem. We do it simultaneously. It works very well.
Everyone, not only in the United States, but around the world, is talking about this major problem we have in academia and the sciences of not having enough really highly qualified people in computing and software development. This is really, really holding science back. Industry, over the last decade and a half, has been a vacuum cleaner, big tech especially, on the brightest people not only within the United States, but all the other developed countries. They're vacuuming up all the smartest people. And that's why tech has been like a rocket ship. And this has come at the expense of the most talented people leaving the universities and the scientific enterprise. This has a big impact on the overall ability for science nationally to excel. And no one knows how to solve this problem, but we've figured this out at Caltech. And this year, our goal is 12 young scholars. Next year, it expands to 14, two senior engineers, because the Carnegie Institution of Science is being brought in.
It's not gigantic, but it's substantial. But the faculty are so excited by how smart and talented these people are. I would like to see something like this continue. It has to be made in some sort of a sustainable way. It's very, very expensive. The Schmidts have been very generous. On the other hand, it's a philanthropist and a foundation, who view their mission as to disrupt things and to start new things, not to be sustainable. And they've done that. Somehow, we've got to figure out a way to sustain this. It's an Institute-wide thing. I met with a group of faculty members yesterday and threw this out there, that we've got to get the faculty talking about this because it's way more than me. Individual members of the Seismo Lab can route funding for the Seismo Lab through federal agencies and foundations when we need support for a new idea. But for this other thing, it's something that has to be done more broadly within the Institute. I think I've done my job, but on the other hand, I don't want to leave it as an orphan either. There's a catch-22 there.
ZIERLER: I wonder how much of a foundation you're already building on, given that at Caltech, undergraduates in computer science are much more likely to go into computer science, even if the numbers nationally are down.
GURNIS: No. I would've thought what you're saying is true in a historical context.
ZIERLER: Even compared to a Stanford?
GURNIS: Compared to a Stanford, it's probably worse at Stanford. But at Caltech, we're discovering that computer science, even here, is that the explosion of computer science has really corrupted, at some level, some fundamentals in academia, in my belief. Especially the Caltech beliefs in the fundamentals of science. People came here traditionally for physics, and this is great for the Earth sciences, planetary science, chemistry, geophysics. Those fundamentals are very important. And computing is really fundamental, too. But their vision, in some sense, is not so much, "I love science." More Caltech students love science compared to Stanford, Harvard, Yale. But people are going into computing because of the career opportunities, potentially business opportunities, application opportunities. I see so many résumés for graduating Caltech students, and many of them now don't do what's called a Summer Undergraduate Research Fellowship, which Caltech started around the 60s. Now, you read these résumés, and they're doing industry fellowships, financial, Google, Facebook, those kinds of things.
They're not necessarily going to work in a Caltech physics lab or JPL to do basic science. They're doing internships within computing companies, tech companies. I'm a geophysicist. One of the things I've observed and been involved with in my career was that the big driver in geophysics was oil. Ever since World War II and right after, it was finding oil and using science to get it out of the ground cheaply. Geophysics played an extraordinarily important role there. Most of geophysics–not Caltech–has been part of this field. And one of the things we saw was a seesaw. Things explode, the oil industry becomes a gigantic vacuum of talent, then there's a bust, everyone is laid off, no one can get a job. I think it's just inevitable that there will be a bust at some point in computing. People are already saying it's happening right now with the hiring freezes at big tech. We might see things turn around, and this could be really good for academia, not just within the United States, but internationally, that all of these people trained in computer science aren't going to immediately get these overpaid jobs, and they'll stay within academia and get a reasonable salary, at least for a few years. Or go to graduate school.
ZIERLER: Is there any cross-pollination with quantum computing at Caltech and all the efforts there?
GURNIS: Not within geophysics. I can't see how we can use quantum computing to do geophysics better.
ZIERLER: Is that just because earthquakes and seismology are classical physics?
GURNIS: No, I think it's because of the way we've formulated algorithms through traditional ways of solving PDEs to machine learning and other methods of optimization. They're all based upon algorithms where we do digital computing. We don't use low-level languages; we use high-level languages. Even Fortran or C are high-level languages, and then we use higher-level languages above those. They're so finely tuned to digital computing and our thought processes and ways of imagining problems, even with the revolution in machine learning. It all comes back to using these languages that exploit the digital. Now, that doesn't mean that these high-level languages down the road can't be tailored to a quantum computer. But at this point in time, I can't devote any effort intellectually as a geophysicist to thinking about quantum computing, because there's such a huge divide there, even on the compute side, between the way I can translate physics and mathematics into quantum computing.
But there could be highly specialized algorithms that could naturally fit better on a quantum computer. It was kind of interesting that my first direction in computing at Caltech was part of the Caltech Hypercube project. The parallel computer was invented at Caltech, and within a year or two, I was one of the fortunate few able to use one of these home-built computers to do geophysics. But when they first thought about using this Hypercube architecture, a lot of the thought processes, I believe, had to do with N-body problems, and there was sort of a natural mapping of that. But then, other people started to imagine how one would be able to use a parallel computer with message-passing, and the first language that allowed message-passing to work was written here at Caltech, then it eventually became the basis for the first open-source software developed at Argonne within a year or two, which is the language that's underneath our computer programs these days.
But these things happen, so you don't really know. No one knew about parallel computers before 1984. People always imagined it. People always knew there was this von Neumann machine imagined back in the late 40s and early 50s, where data and algorithmic processes happened in the same elements on a machine. But with the Hypercube project, it just exploded, and a few years later, there was an alternative architecture invented at MIT that went nowhere. I don't know where quantum computing will go. I know that Caltech is going to explode in this particular area. Many other universities within this country and others are following that lead. It's going to be interesting.
ZIERLER: In the way that you raised concerns about the impact of industry vacuuming up all this talent, between you, Schmidt, and Caltech institutionally, how much are the motivations about preserving the American competitive advantage, long term, against Europe, China, whoever? America has historically been a source of innovation. How much of this is about retaining that for the next generation?
GURNIS: That's why I think it's very important to try to imagine that we can build programs, and the Schmidt is a good example, where we really have incredibly talented people. Sometimes, Caltech, in our individual academic options, is a little bit insulated from these things because when you're at the very top of this apex, and a number of schools in the United States are, and we see so much talent–it's easier to get into Caltech as a geophysicist than it is as a computer scientist because everyone wants to become a computer scientist. Fewer people want to become geophysicists. One of our relief valves has been that we're internationally competitive, so people from around the world come here. This is exactly the reason Eric Schmidt makes these investments. I think it's absolutely important.
A place like Caltech, where people come up with new ideas, try them out, is so important. And the government has to recognize that. Hopefully, this bill, which finally got hammered out, will be passed. The one they unfortunately call the CHIP Act, even though the chips are only 20% of the bill, I think. But that's really important for the United States, and presumably, a lot of that money will go into higher-education research and our abilities for American scientists. It probably won't have a big impact on Earth scientists. They're going to build a whole new directorate at NSF for industry, I think.
From Mansion to Campus
ZIERLER: I want to shift the conversation to some historic questions about the Seismo Lab relative to where it is now. You've been at Caltech long enough that you have some historical perspective yourself. Let me start off with a fun counterfactual question. Let's imagine that the Seismo Lab never relocated to South Mudd, that it was still up in the mansion in the San Rafael Hills. What would be the advantages and disadvantages of that, circa 2022?
GURNIS: I think it was a really wise move. One of the things that was really important about geophysics at Caltech, and this was true in general, is that the Seismo Lab, over the decades, moved into two very–one of the first trends, the whole physics of the Earth, the integration of seismology with other disciplines, would've been hard to really do up there in the San Rafael Hills. I would've felt probably more out of place there, or even had no place there. When it was up there, all by itself, it was really just about earthquakes. Although Gutenberg wrote a book called Physics of the Earth. He thought all about the Earth. And Hugo Benioff thought about deep-focus earthquake and their relationship to tectonics, and he didn't get it. But they were thinking about these big things.
And I think it's important to be within a community of other Earth scientists as well as other physical scientists to sort of integrate a lot of these things. Now, with the web, it's both good and bad. The good thing is, it allows people to collaborate easily. The bad thing about the web is, it allows people to collaborate easily. [Laugh] Why is that a bad thing? It goes against this local community of people working intimately together. I've made scientific advances, and whether they're important or not, other people can judge that, side by side other people who have different skillsets here. Then, I've done things more in remote collaborations because of people's interests. And that local stuff builds up something special, whereas the other thing does advance science and allows people to communicate with one another, but it doesn't build institutional foundations. It tends to wreck all foundations that exist because things just move so easily.
That was one thing, thinking about the physics of the Earth. At some level, most of Don Anderson's career was spent on campus, and he's the guy who filled Gutenberg's shoes to think about the whole as opposed to just the earthquakes. And I think it was done better, having it here on campus. Although, most of Don Anderson's work was done within the "Lab," although he thought broadly about things. That's one big trend, thinking about the Earth. You need a lot of different people. The other thing was the physics of earthquakes, and this is something I could watch during my career. It's actually not my research at all, but I could see it in the synergistic aspects, which happened in that regard, especially between people in the EAS division and here within the Seismo Lab. I think that happened nicely, and I think that in-person collaboration on campus allowed Caltech to play a very important role in defining how earthquakes worked in the last several decades. I think it's better because Earth sciences is big, it is good for people to interact and collaborate with one another in person.
It's more effective and more satisfying. And I think we would've just become too narrow. I think it was a good thing to move. It would've been fun there, and in some sense, with the internet, it could've been fun, too. You'd work very closely with several colleagues. But just several. And now, we're seeing yet another explosion here within the Seismo Lab with Zach Ross. It's quite extraordinary because he's at the cutting edge and collaborating with people at the cutting edge in computer science and computing. And there have been other trends, too. Everything I talked about with parallel computing happened on campus. I still like things that are small. I like the fact that the Seismo Lab is small. But yet, we're easily within this larger community of individuals, both here within these several buildings in Earth sciences, then also in other disciplines. I think that's really important.
ZIERLER: As you well know, the perception 40, 50, 60 years ago, the Seismo Lab in the days of Benioff and Frank Press was really the center of the seismological universe. As director today, do you aspire to maintain that reputation, or are the trends in the field such that it really isn't a relevant metric anymore because things have changed so much?
GURNIS: It's probably not a relevant metric, and it's actually impossible because seismology now compared to then is gigantic. And we're a small program. San Diego and Stanford are bigger than we are, although the raw numbers probably don't mean much. You go to Tokyo, and they have dozens and dozens of seismology professors. Same with Paris. Internationally, these programs are very large. I think with just like many other things, all around campus, there can only be a handful of people, and that's all we are. And we have to be very, very good at what we do and figure out ways to make it exciting for very good people to be here. All we can aspire to is to do really good stuff. There's no way one can just dominate things like one could dominate things back then. No one can.
The Chinese can't, the Germans can't. No one can. You could have a gigantic institution with hundreds and hundreds of physicists and a billion-dollar budget, but that's nearly impossible to imagine for me. The world and all of science has just changed so dramatically. World War II changed everything. Science, scientific investment, scientific progress, integration with engineering, it was no longer a cottage industry. It changed the world. The only thing that got the US Congress off its duff after a year and a half was $280 billion for science and industrial policy, and they don't call it either of those things, they call it the CHIP Act because people can't buy their cars because there aren't enough chips.
The Universal Benefit of Data Sharing
ZIERLER: You emphasize the overall growth of the field in a way to understand how these things have changed over the years. What about the fact that 50 or 60 years ago, seismological data was proprietary. There were no online databases. What about the influence of the overall decentralization of information that makes it so that even at the institutional level, it's not so important to think about what programs are the best or not?
GURNIS: Absolutely. This probably goes back to the turn of the century. We had two post-docs, Jeroen Ritsema and Hendrik van Heijst, and we would go to lunch every day. We'd be over there at Chandler. Jeroen was a post-doc, so he couldn't have his own grant here at Caltech, and we had big overhead. He'd always say, "I just get my data from IRIS." IRIS is a big organization for seismology that the Seismo Lab played a key role in starting. "I just download my data. I don't need Caltech. I don't need any of this. I just download my data onto my laptop, and I'm happy. I could be at home all day." He was saying this more than 20 years ago. I was going, "Sure, that's fine. You can do all that stuff by yourself. But there are two things you're going to miss out on. One is that the scientific community needs to build big things. Astronomers need to build big telescopes, and geophysicists need to make big networks of instruments that we network to transmit high data rates into a centralized facility. Someone needs to build that and put it out. The other thing is the community of scientists."
I was in a different field having lunch with them, and we had lots of ideas, wrote a lot of papers together, but he seemed to miss out on that part. And a lot of people seem to be happy with this internet thing. I'm worried. This is historical, so I will say that this pandemic has been the most devastating period for the Seismo Lab in our entire history. It has really wrecked the sense of community. If you walk around the division, we have more open doors here, people walking around. But it's been really tough. Especially at the beginning of the pandemic, we would still have Seismo Lab coffee, and it's really important. It's been really hard, and we may have lost our momentum there. And that's devastating. Because the Seismo Lab was more social in the way it operated than your typical group, more than anything at Caltech. Then, all of a sudden, you've got the stupid pandemic, and it drops an H-bomb on social organizations on this planet. Everyone's sitting on their couch at home, Zooming with each other. The Seismo Lab is antithetical to this. We want to be here. That's what many of us want to get going again.
ZIERLER: All of the alumni I've talked to have emphasized the importance of the coffee hour at the Seismo Lab. That's where the science happened, where the ideas were exchanged, where the greatness germinated. It's a real thing.
GURNIS: And I think my whole migration from the way I thought about problems in terms of plate tectonics and Earth physics changed when I came to Caltech and got to listen and talk to people in coffee. I learned so much. Even though I didn't really do much on earthquakes, the ideas behind earthquakes, how earthquakes work, where they're located, and what we can learn seismologically just pivoted the way I would formulate problems. To me, it was transformative. Here, we're still technically not allowed to be in a room without our masks on. Even in-person, you still have to have your mask on. There are a bunch of us who know how important this is.
ZIERLER: To flip it around, as you emphasized earlier, the Seismo Lab recruited and retained, in your estimation, the top two young seismologists in the field. What's happening at the Seismo Lab to explain that?
GURNIS: Just the people. We knew them intimately when we recruited them. One was a former student who went away. Within the first semester, we knew he was going to change the world. We watched him and nurtured him. And he left Caltech. We did that on purpose so he could come back.
ZIERLER: This is Zhongwen you're talking about, I assume.
GURNIS: Yeah. Then, there's Zach Ross, but Zach found us and wanted to come here. He got his PhD across town at USC. He came here because he wanted to use the network. No one was wanting to use the network. But he did. That was interesting. Then, we started to see what was going on in his brain and what he invented. He was way ahead of the curve in machine learning. Suddenly, he totally revolutionized seismology in his ability to detect earthquakes. And he's moving in new directions now. I can't believe the stuff that guy shows me. He just drags me in and shows me what he's working on, and the things he's discovering are amazing. And he came here because he wanted to be here. Now, with these people, I think we'll be okay. We just have to keep finding really good people. But not many. That's all we can do. Things are also difficult because when I came here in the mid-80s, it was on the tail of a true revolution in the Earth sciences, plate tectonics.
There was the initial burst of plate tectonics that happened, then I did my PhD studies, and things were exciting. In my particular area, geodynamics, which is basically to try to uncover the physics of plate tectonics, it was actually quite slow. A lot of it had to do with the computing on both the mathematical side as well as computer capability. Then, in the early to mid-90s, things started exploding. There was a 20-year period where things just exploded in terms of what people could do. We did a lot of it here in the Seismo Lab.
But sometimes, bigger things unfold. If you have talented people at a particular institution, either they can perceive and take advantage of them, or they make them happen to begin with. But there are just bigger trends in sciences, and geophysics has gone through things. And the two big things, which have sort of merged together, are what Zhongwen and what Zach Ross are doing. One is creating massive pieces of data, and the other is analyzing massive pieces of data. They're both on different sides of this coin. And each has a whole bunch of students working with them, as well as the students working between them. Who knows what will happen? We just have to let it go. It's sort of the mantra of Caltech and the division, find good people. In the Seismo Lab, all we can do is follow that recipe when there's a small place with few slots. We try to have people continue to move through into geophysics, but of course, it's highly unstable, especially at a place like Caltech.
Because a whole new revolution could just explode dramatically on the peripheries of Earth sciences, and maybe geophysics won't be part of that anymore. We've always been part of these explosions, but maybe at some point, we won't be. There are always the interesting mega-questions having to do with the Earth, and we know that only geophysics can answer them. Then, there are the societal interests, which primarily these days are on the geological hazards side of things, and we contribute immensely to those kinds of things. But I just don't know. I just want to make sure it's an exciting place for young people, for the younger faculty and the post-docs.
ZIERLER: You mentioned the plate tectonic revolution. I'm sure you've heard the idea that, to some degree, the revolution passed by the Seismo Lab. I wonder if you have any insight on this and what might explain it.
GURNIS: Absolutely, it's true. But the interesting thing is, the Seismo Lab prepared for the next revolution, which was the integration of it all, and we played a huge, pivotal role in that. When plate tectonics happened, it happened primarily because of observations made at sea, and those observations were made because of World War II, primarily in secret. There's a new book by Naomi Oreskes called Science on a Mission. Fantastic book, I learned a lot by reading it. I had no idea how much, especially after World War II, was secret, which was critical for plate tectonics. And part of the reason it got held up is because it was secret. But to get back to your point, the Seismo Lab missed out on the plate tectonic revolution because the data that was integrated was actually primarily bathymetric and magnetic data.
Then, the first thing that happened to validate plate tectonics was seismology, and it was done by people at Columbia, not here. But suddenly, all the things Caltech had discovered, especially Benioff, were understood within the plate tectonics context. In some sense, because we were focused in on earthquakes, at some level, we weren't at the core of it all. We weren't an oceanographic institution doing all this top-secret research for the Navy, we were doing research on earthquakes, funded at the time by the Air Force, which wanted to detect nuclear explosions. The Navy wanted to detect submarines. Their top-secret institutions discovered it, not the Air Force's. It was no flaw of Caltech; it was just the way the dice fell. And it's really quite interesting. One of the stories of the Seismo Lab, in the mid-1960s, when the plate tectonics revolution was unfolding, Frank Press left in a huff.
He would later become Jimmy Carter's science advisor, then later, president of the National Academy of Sciences. He loved Caltech, but he left here because he wanted to steer our program into oceanography. We're a landlocked institution that wasn't doing oceanography at all. We have a little facility now down at Corona del Mar. But the institutions which were going to define the future were already developed. It was so extraordinarily well-funded by the military that we couldn't catch up. Frank wanted to do this, and the Caltech faculty said, "No, we're not going to do that. We already know how we're going to get to the future, and it's through the planets because we're ahead of the game with JPL. Forget about oceanography, we're going to go to the planets." Frank left, and when he went to MIT, he developed [I believe] the MIT-Woods Hole joint program, which allowed Woods Hole to become a top-tier program. Caltech couldn't have done that. There was a national committee that thought in order to move forward in terms of seismology, Caltech needed to develop a seismology program back in the 1930s.
And we did that. But at the same time that was going on, the National Academy of Sciences said that they needed to invent this thing called Woods Hole Oceanographic Institution right on the water, and they invested in that. World War II happens, all this data is collected secretively, and plate tectonics happens. But what was happening in the Seismo Lab, especially with Don Anderson, with the mantle of Gutenberg, was related to the physics of the Earth. When we came around to figure out how plate tectonics, convection in the interior, and all that worked, in some sense, it was the Seismo Lab and Seismo Lab students that did that, period. That big revolution happened, and a lot of people played a role in that, but Caltech did not miss the boat. We were central to all of that.
ZIERLER: As you explained it, the Seismo Lab was simply not set up structurally to be a center of the revolution as it was happening. As you look at your tenure today, are there parallels, things that are not happening that can't happen at the Seismo Lab that might suggest there might be other revolutions that pass by Caltech?
GURNIS: There are two big revolutions that seem to be occurring within geophysics. One is big data from an instrument perspective, and the other is machine learning. We're trying to capitalize and are the leaders in both. But I don't know. We didn't consciously hire in this area, but we had good people, and they suddenly capitalized on these trends. But those are the big things unfolding right now. What's going to unfold in the future? I don't know. The other big thing that's going to explode on the big data side of things is going to happen from space with radar interferometry. It's already revolutionized Earth sciences, but it's just about to absolutely pivotally explode, and that's because there'll be a gigantic high-fidelity US mission. And Mark Simons and JPL are the leaders in that. At the Seismo Lab, we have been positioning ourselves, doing all these investments to move in that particular area.
The other thing we want to move into in the future, which we think is going to happen, and strategically, I think the Seismo Lab faculty wants to move in this direction, is the seismology of other planets. There are moves afoot in the Lab to try to play a more important role in that. We sort of potentially missed the boat on the Insight mission and stuff like that, but that's what happens. Caltech can't do everything. It's a wonderful place that plays a disproportionate role, but we can't do everything. Because of that, there will always be areas of science that will grow that we're not part of. That's the thing, the reason other universities are big is that anything that happens, they grow to be part of that. And you know how big these universities are around the country. They have more faculty members than Caltech has students.
I was at a university like this, the University of Michigan. Anything that could happen, they're going to grow the university in that direction. But we don't do that. That's just not the mindset, even within geophysics or the division. It's to figure out a few things that could be critical ahead of time and try to make the investments in them. I have views about different areas of science that I think could be quite cool, which I've articulated to my colleagues. One area that's always moved really slow within geophysics, especially in the interior of the Earth, has been to use electricity and magnetism to do imaging in particular. It's so poorly developed compared to seismology, and no one in the field has ever really integrated these fields. It's a topic I want to nurture. I've seen some really crappy ways people have tried to do this, brought in a lot of young people from around the country the last year to see if this could be an interesting area.
The science is interesting, the technology is interesting, but we haven't yet found that person. It all depends upon the person. It's funny, people will have an open search at other universities in a discipline, like in geophysics, geochemistry, something like that, then they advertise, "We have an open position in geophysics or seismology." People will apply, and they'll have a short list. "Here's our top person. Offer them a job." "Oh, they decided to go to this other university." "Go down the list and offer the position to another person." Our searches just stay open, then suddenly, a person walks in, and you listen to them, and you say, "Wow. Let's hire that person." And you didn't necessarily want to go into that field, then you see the opportunities, and you hire them. That's the way things are.
We're going to have a few open slots in geophysics, and there are areas we're definitely interested in. This electricity area. People are very interested on the planetary side of things. That's the way things sort of are. Interestingly enough, the Seismo Lab didn't get developed under that rubric at all. It's a historical thing that happened. The Seismo Lab did get developed because someone had a vision. Harry Wood had a vision. No one would listen to him. Then, the Carnegie Institution of Washington said, "A-ha. There's a person who's interesting. How can we invest in that? We'll take a little cubicle in the Mount Wilson Observatory in Pasadena because there are earthquakes there, and we'll give you a little money to see what you can do."
And he did it, and it got bigger and bigger. Suddenly, it got big enough that there was a National Academy meeting or whatever it was, which brought all the top geophysics people together in Pasadena, and they said, "Let's make something out of this." Science was small back then. There were these huge gaps in what human beings were doing in the application of science. Universities were so big, generally, these behemoth universities just vacuumed up everything in their path. Caltech will be small and tries to see interesting niches or very important things everyone else is ignoring with the creativity and intelligence of the faculty, go in that particular direction, and then suddenly, something unexpected will happen.
ZIERLER: You mentioned some of the exciting work Mark Simons is doing with JPL. Obviously, JPL is enormous, and the Seismo Lab is tiny, but I wonder if you really see it as a two-way street in terms of what the Seismo Lab can offer JPL and vice versa.
GURNIS: Absolutely. Definitely a two-way street. Not only scientifically, but one of the reasons, especially on the radar satellites, why JPL and NASA are very interested in the Seismo Lab is the whole method of Caltech working with the USGS, especially regionally on earthquakes, and our ability of monitoring, reporting, and interpreting earthquakes, especially in near-real-time and during national disasters. NASA is totally orientated around missions. They don't actually have this long-term perspective on observing and reporting things, especially in the natural hazards arena. This center Mark has is about near-real-time integration of imaging from radar satellites with our observational capability with seismology.
We have a staff besides the faculty here, and I have a research professor, several who run the seismic network, and we also have a staff seismologist looking at all the local earthquakes, and they play a very important role in both managing people and reporting on the earthquake during a local event. But we want to have the same thing from the radar. We'll be expanding this activity, especially after this NISAR satellite goes into orbit, and Mark's one of the key players there. We've positioned ourselves in that arena. It's definitely a two-way street. Even though we're really small, we have developed this expertise. Not only have we developed an expertise–NASA could develop this expertise overnight because of how big they are and how much money they have–but we've also developed a reputation. That's the cool thing about the Seismo Lab locally, the reputation that we've developed within the culture of Southern California. And because we've developed a reputation within the culture of Southern California, where else did we develop a reputation?
Hollywood. People see that, and it gets into the consciousness. Even within the consciousness of the management of JPL, and of course, the highest-level management of JPL is either involved with the Seismo Lab or knows about us. This is one area of our expertise, measuring earthquakes and interpreting local earthquakes. And of course, we don't do this by ourselves, we do it with another arm of the federal government. That's kind of the cool thing of being a small, nimble organization. And the USGS and NASA are like oil and water. NASA's a gigantic federal agency, and the USGS is a moderate-sized federal agency. They have very different outlooks and perspectives. But we work with both of them. This gives us great strength. And we're going to be continuing to capitalize in that area, especially as NISAR continues to go into operational mode in the coming years. It's definitely a two-way street, and they see it.
Straddling Observation and Theory
ZIERLER: We've been talking about community, we've been talking about policy, history. Let's talk about science for the last part of today's talk. Some technical questions that will orient us for subsequent discussions as we get more into your research. First, at a very general level, where are you on the spectrum between observation and theory?
GURNIS: I'm between the two. I'm not sure if I'm exactly between the two. I started off at one side as a PhD student. I've always been motivated by the real Earth. By the way, let me tell you, there are two kinds of geophysicists. There's the geophysicist who literally has started in applied mathematics. They became fascinated by this and said, "I'm good at this. Let me go into geophysics." Many, many good geophysicists have done this. They've changed our way of looking at the Earth. Another geophysicist is totally empirical. They see stuff. "There's an earthquake, I measure it, I get a seismogram, I can go out with a gravimeter, see what the gravity field is doing." I was always fascinated by the Earth. I only developed my skills in geophysics in doing physics and mathematics because I was fascinated by geophysics, and they were tools I needed.
But I was involved in sort of a theoretical computational topic on how convection in the Earth's interior worked. We developed and used computer code that solved the relevant sets of equations to understand how convection works and to explain observations in a generic sense. To understand the overall heat output of the Earth, how fast plates actually moved, to generically understand what the interior of the Earth could look like between the way that areas of the cold returning plates would look like versus warm upwellings and convective fluids rising in the interior. Then, thinking about how thermodynamics influences this, how the different aspects of the materials influenced these processes, then looking at that. The way I always looked at it was, "Here are these observations. Can I tailor the physics to sort of interpret them?"
I became more and more interested, not in an inverse sense, in the way a traditional person in optimization theory would approach the problem, but thinking, about, "How can I incorporate some of the things we see with our own eyes with geophysical observations? How can we integrate those better with the solutions of the equations?" I was in this community, and they're all wizzes at applied math and computing, and they were always a little bit smarter in that area than I was. They were always really good. But I was always known to be the person more interested in the observations. Even though I was on this side of the spectrum, I'd be the person really looking closely. "What about this? What about that? On this computer model, it doesn't look like that at all. It's wrong." I developed a bad reputation. I was the thorn in the whole community's side. I'd stand up at the end of someone's talk and go, "What you just said is absurd. It doesn't look like the Earth at all".
This doesn't look like the subduction zone south of Japan. You said it did, but in reality, it doesn't. It's totally opposite. Not only that, your model does this other thing, and the gravity anomalies that are predicted to be gigantically positive, and they're actually zero. It's wrong." Because I was so much more familiar with the observations but yet was in this community, I could see when their models were doing crazy things, whereas most people, when they saw this stuff, it was just a bunch of colored pictures they were getting in a presentation. But I really knew what the models were doing, the magnitudes and stuff. I was always the thorn in people's sides about that. But then, as the algorithms I was involved in generating and the compute power had improved, they started to look much more realistic, and I started to become much more interested in explicitly how the models were related to both geophysical observations, but for much of my career, to the geology.
I became incredibly interested in the stratigraphic record of the continents, sea-level change, and trying to integrate all of the many observations associated with that field of science and integrate them in with the physics of the Earth's interior. Instead of trying to do things in a generic sense, "Oh, you predict one kilometer," then think about that from a global perspective, I said, "We predict one kilometer under North America 30 million years ago, then it's going to uplift at 20 million years ago," or something. I'd try to take it from a generic statistical average to explicit. The thing about the Earth, you go out there and measure things on the surface, we have all of these things we measure in time and space explicitly as opposed to saying, "This is planet Earth, and we know on average how it's working." I wanted to connect the explicit physics in time and space to the explicit time and space observations.
Then, I started to work in great detail on developing an area of science which is akin to what they have in atmospheric sciences. Atmospheric sciences moved, too, in this area of thinking about how the physics of the atmosphere works and developing what they would call a "general circulation model" for the atmosphere. That is now a gigantic part of science, and it's the fundamental part of weather and climate predictions. But it's explicit. A lot of it is explicit between observations and the models. I've tried to do that for the whole Earth. That means a gigantic synthesis of plate tectonics as well as having the mathematical and computational abilities to compute this in a sphere with all the relevant physics, which is hard to compute, connected up with plate tectonics, and then to connect it to things like sea-level changes and how the stratigraphic records of the continents would evolve. I became very integrated with observations.
Then, over the last decade, in another direction I was taking, I was making all these predictions, and no one was testing them. I actually got heavily involved with marine geophysics as well as what's called the Ocean Drilling Program. It's kind of bizarre. There are hundreds and hundreds of people in this field, and here I am, this mathematical geophysicist. It was sort of like being the Ayatollah or something. "Whoa, you're way out in left field, man. How can this relate to anything?" But it does. Different areas in physics and the way we observe things are connected. I got heavily involved with the Ocean Drilling Program, would go to sail on drilling legs and work with other people who would actually make explicit observations on the drill cores that we would connect to the models. In fact, I just returned from New York, from the US Advisory Committee for Scientific Ocean Drilling. Most of it's about climate change, the fossils that are picked up in the cores, plus the ships and how the drilling works, all that stuff. I got way out of my comfort zone. But it's important for science. I did a lot of very interesting things scientifically.
ZIERLER: When you say that the models got more realistic as a way to explain the shift in your career, where you're sort of more in the center now, is that simply to say that the models are just better at simulating reality? Or are you pointing to something deeper?
GURNIS: That's it. They actually started to look realistic. Before, they were completely unrealistic.
ZIERLER: How do you know?
GURNIS: Because we geophysicists measure things. We measure the topography of the Earth, how thick plates are, how plates look. We literally measure them and image them with seismic imaging techniques, and we have their shapes and their morphology. We have their explicit physical characteristics measured from their seismic properties. We measure their electrical properties. We measure things with a gravitational field. We measure the state of stress and strain inside plates. The plates in the models before weren't really plates, they were like gooey fluid things. Then, when the plates returned to the interior, they were sort of convective downwellings, not plates.
Then, in the mid-90s, they started to be like plates because we started to incorporate more realistic physics, the most important being, and where the Seismo Lab played such a huge role–when I would go to coffee, I knew I had to change my models–faults. When the plates subducted, we had great earthquakes, which meant there was a huge decoupling zone. Hiroo was at coffee, Don Anderson, and we'd talk about this. I'd talk with Hiroo, go read all these papers, then come back the next day, and all of a sudden, I knew more about how these earthquakes worked and thought about them. And I said, "Ah." When I was here as a post-doc, I realized our convection models had to be completely different. Our convection models needed to have faults in them, and no one had a fault in a convection model before.
When I left here and went as an assistant professor to the University of Michigan, I took my knowledge from the Seismo Lab and said to my PhD students, "We've got to figure out how to put faults in a convection model." And we did. It was technically very challenging, but we did it. And this is an interesting thing. The way a geophysicist was putting a fault in a model, they would literally put a dislocation or discontinuity in the computational mesh itself. There were people who were doing earthquake problems who were doing this, so you could use the finite element method to do it. But then, when people were doing fluids for convection, the way it would work was, you'd have these fluid bodies, and there was no sharp discontinuity in them at all. We literally put a sharp discontinuity in there.
And the first models were quite similar to what other people were doing, including stuff that'd been done earlier at Caltech. But then, we had the bright idea, "When you have a fluid, things are transported and move around inside a system." We actually formulated a fault, which you usually think is a discontinuity between material over here and over there. And that's how most geophysicists think to this day. You go out there in the field, there's a fault, there's material over there, there's a hanging wall and a foot wall, and they slide by each other. That's it. But then, if you really think about a fluid in the longer term, things move around. Think about classical experiments done in fluid mechanics. You have a bowl of fluid, it's moving around, you've got some dye in it, the fluid moves and strains around. Typical thing someone in engineering or fluid dynamics would do. Interestingly enough, a fault is actually material.
ZIERLER: It's not the absence of a material, you mean.
GURNIS: You can think of a fault as literally material. If we go out there, and we keep having earthquakes on the San Andreas Fault, and you go there, and the reason why there are earthquakes that keep happening on faults over and over again is because that material is damaged. Because it's damaged, it's got a different composition and different material properties. But that San Andreas Fault is actually connected to two plates, the North America and the Pacific. And those plates are moving. And the interesting thing is, the fault is being translated. That material is moving around. If you think of the Earth as a continuum, and everything is moving around in a continuum sense, the fault itself is a property being transported. We then came up with a whole idea of having a fault, which is a material entity and can actually be transported and advected.
What does it do when it gets advected? It remains a source of localization. That was one big area of science I took off with, which was a direct outcome of listening to people at coffee. It was just after Hiroo had made these really monumental advances in thinking about earthquakes, subduction-zone earthquakes, things like that. That was one of the things people were talking about. But people in my community of geodynamics and mantle convection didn't think about that kind of stuff. I was just sitting there, listening to this, arguing with them, reading their papers, talking with them. That was one of the big things I took away with me. By the time I came back to Caltech several years later, we had really–actually, the whole idea of transporting them was a Caltech paper. That's an interesting example of what happens when you have a bunch of colleagues that like to talk to and learn from one another. You're not just in the same community of people in the same field.
ZIERLER: To further develop this idea, the models are more realistic, the measurements bear it out. If we can concentrate specifically on one area in which you've done so much work and innovation, using computation to better understand the dynamics of the Earth's interior. If we can imagine a scenario where, best-case scenario, we could drill as far as we wanted to, and we could have direct observation, how far away are the computational methods you've developed from literally having sensors in the Earth's interior to see what's happening directly?
GURNIS: First of all, we're never going to be able to do that with drilling, not in our way of conceptualizing science. The Earth's interior is going to have to be a remote-sensing kind of thing. It will always be remote sensing, I think.
ZIERLER: Just because technologically, we'll never be able to do it?
GURNIS: Not in any of the ways we understand physics operates. It doesn't seem to be feasible. Maybe you could do something on a different planet and wreck things pretty bad. Within rocks, you can only drill down five kilometers maybe. We can drill deeper in sediment and things like that. But it's just so hot, the pressures are so high. And it's so expensive, even to go a little bit down. The oil industry drills in easy places. It's easy because they're drilling through sediment.
ZIERLER: Let's just say if we could drill that far down, wouldn't that be the gold standard of direct observation? Or is that not the right way to frame it?
GURNIS: No, it's naive. Are we going to put a sensor on the surface of a black hole? It's that kind of a question. What we do today is like magic compared to what Isaac Newton thought we could do. Maybe things will change. But I don't view any of that as really helpful as a scientist, trying to do things realistically now. But I think the tone of your question is how realistic our models are that we can be really confident.
ZIERLER: Exactly.
GURNIS: The way we do things, and this is the interesting thing, and what makes geophysics and other areas of Earth science hard, we literally have to be detectives and bring so many different things together. In order for us to know about the interior of the Earth at some great level, a pressure thousands of kilometers down inside the planet, so much information from mechanics and models is required that realistically compute the solid and fluid mechanical processes. But then, all of the thermodynamics and properties of the material have to be known. Here's something really important. Sometimes I just give up on actually doing this, but it was something I had read in Physics Today I think when I was a graduate student. This guy thought there's something called computation, and something called simulation. And you can only really use the word simulation if you really know all of the thermodynamics, chemical properties, all the properties in the CRC handbook. And they're all known. And all the equations are known.
And then, you just turn the switch. We think people can simulate what happens inside the cylinder of a car. At GM and Ford, they have physicists who will do simulations of a piston, and they have all thermodynamics of what goes on in a chamber and the reactions. And that's a simulation. But they know all of that. Therefore, they can optimize. "What should the diameter and length of a cylinder be? If I change the composition of the gasoline or the temperature of the sparks, I can optimize things." That's a simulation. People use this word simulation all the time, and I just cross it out. "It's a computation. Give me a break. You have no idea what the properties of this material are." That doesn't mean it's not science. It's still science because you have the conservation equations, we can argue about them.
Even in geophysics, we don't know all the equations. We know the conservation equations, but we actually don't know the rheological equations. That's the equation that connects how the stress and strain rate connect to one another. We think we know, we do experiments, and we do a six-orders-of-magnitude extrapolation for known material. But now, we're dealing with unknown materials, but we know generally what they are, and we know what the candidates are, so it's not a mystery there. Just by common sense, we know what the materials have to be because it's things we pick up and we think are down there. But then, the material properties of this alloy–and they're all alloys of unknown composition. And in terms of stress and strain rate, we're extrapolating them over six orders of magnitude. It's not pseudoscience. We have to be very careful about it. Getting back to the point, because we don't know these things, because they're computations, not simulations, we have to bring all these different things together.
We have to bring in all of our seismic observations specifically, then the other geophysical observations, then it's a complex, time-dependent thing, so we need observations from geochemistry and geology to validate those particular models. You integrate them together, and it's not like they're in a model in which you've added everything together, you turn it on, and you see what comes to, but rather these are usually highly tuned to one set of observations versus another, and you change the outcomes, you see how well they fit the observations, then you look for other predictions that may actually occur. Those are some of the things I often look for. I look for things we haven't yet measured the same. And it's usually in time and space as opposed to the kind of measurement. It's like, "If we go to this place of the Earth, we can expect to see these kinds of characteristics." It makes it a very exciting area of science because it's like being a detective, and you have to bring a lot of different things in, so there are a lot of discussions and controversies.
People need to dig down and keep talking about these things so they know the reason they're saying X is because they assume Y. People are going to get upset because one group is predicting X-sub-1, another's predicting X-sub-2, then they argue. "Let's go back. You've made different assumptions. Let's discuss those things, the pros and cons." One of the things I'm doing in my own area is, I'm working much more intimately with applied mathematicians–and they're actually not at Caltech, they're outside–to more formally do inverse models for geodynamics. And I've learned a lot by them. But it's really, really hard, what we're doing. But it goes back to this whole point, that the models seem to look more like the Earth.
We're trying to infer some of these unknown mechanical properties that are usually only measured in the laboratory. We're trying to actually set up these inverse models, in which we try to extract the nonlinear characteristics of the material properties. And that's the reason we have plate tectonics as opposed to fluid mechanics, because the material properties of the system are so nonlinear. It's some of the hardest things that are measured in the laboratory. They were always traditionally really hard to put into computations. We've now tried to take it to the next level of trying to put them into optimization models instead. It's slow and hard. The science is fun. It's hard, there are a lot of different components to it. I'm actually not a fan of the word interdisciplinary.
ZIERLER: It's a buzzword, for sure.
GURNIS: Yeah, but it's because they're actually all different flavors of Earth scientists. I just say, "You just use different methods." I have a very interesting comment. I don't know why it just came to my mind. For many years, I was part of something called CACR, the Center for Advanced Computer Research. That was when the Hypercube computer was invented in 1983 at Caltech. It was invented by someone in computer science and astronomy. Then, Caltech had this leading edge in parallel computing. Then, quickly, we developed CACR, and it went through different incarnations. Eventually, instead of having a faculty director, which was a mistake, they had an executive director who was non-faculty. Always a mistake at Caltech because there aren't many faculty, and then they don't get anything done. You need to be a faculty member. But we had an executive director, and he was a computer scientist we brought in from industry.
We were trying to excite and interest different people in our center for faculty around the campus. And he wondered why people in Earth science were so much more willing to work with us than people in physics. I made the observation, "I think it's because Earth science is a derivative science. Physics is a primary science. Anything to do with physics, physicists are the smartest people in the world. For physics, that's true. They know and invent physics. But Earth scientists aren't like that. We're a derivative science, the next level up. There are things which are fundamental to Earth science. Earth history. Actually, geology is a fundamental part of science, which is part of Earth science. But in Earth science overall, we're a derivative science. We use math, applied math, applied physics, computer science, physical chemistry, nuclear chemistry, genetics and genetic engineering, all those things. All these things in the primary sciences get invented. I'm not the smartest physical chemist in the world.
You invented a new physical chemistry thing? Let's bring it over to Earth science and use it." Same with computing. That's why we were very, very open. "You've got all these new ideas in computing, and you think you can do this very well? We open our doors. Come on in." This guy was an engineer and making this observation. He said, "You guys seem to be so open and easy to work with." I said, "It's because we're a derivative science. Everything we do, we didn't invent." The only thing that probably got invented is stuff that John Eiler in geochemistry did. He invented clumped isotopes, and that's one of the fundamentals of science, period. Nothing to do with geology, geochemistry, or whatever. He happened to be lucky enough to invent one of the fundamentals. But that's really rare, almost nonexistent in the Earth sciences. We're derivative. Even at Caltech, it's all derivative. Physical chemistry, nuclear chemistry, applied math, computer science.
ZIERLER: We'll cut it there. We'll come back next time and develop your personal narrative.
[End of Recording]
ZIERLER: This is David Zierler, Director of the Caltech Heritage Project. It's Friday, August 19, 2022. It is great to be back with Professor Michael Gurnis. Mike, once again, it's great to be with you.
GURNIS: Thanks, David. It's wonderful to be here.
ZIERLER: Today, we're going to go back all the way to the beginning. In our first conversation, we took a great tour of your approach to the research, your institutional responsibilities, sort of a wide-ranging view of all things geophysics and seismology. Today, let's start way back with your parents. Tell me about them.
GURNIS: They both were born in eastern Massachusetts, to the south of Boston. My mother's Irish from the Dempsey clan. Lot of Irish people in Massachusetts in the east. She grew up during the Great Depression. My father was a first-generation American from Lithuania. We think the name actually is Girnus. Because my grandparents were illiterate, it didn't translate right. My father's parents were from Lithuania, and they came about the time of World War I. They fled so my grandfather wouldn't be conscripted into the Russian army. They came and both grew up in eastern Massachusetts. My father grew up on a farm, and he was 16 when Pearl Harbor happened. But he actually had a little problem with his ear, so he couldn't actually serve directly. He ended up in the Coast Guard Reserve, and he used to defend the beaches in that way, but he also worked on the farm. Then, he also worked in the shipyard. He had three full-time jobs. [Laugh] And he did that well, and he worked really hard.
He was a really good baseball player, and he was detected by a scout. He ended up being recruited by the University of Alabama, which is weird because he's a Yankee. Well, I grew up in Massachusetts, so he's not a Yankee because he was an immigrant. But if you're in the South, he was a Yankee. But he was recruited to go to the University of Alabama. He went down there maybe in '44 or so, played on the baseball team. In his senior year, after World War II finished, he took a class in nuclear engineering, the first year the class was taught. He was getting a degree in mechanical engineering, but he took this one class in nuclear. He got out and came back to Massachusetts, and he was immediately hired– [during the War] he worked in the shipyard building Higgins boats. But then, he almost immediately was hired by the same shipbuilders. He was hired by what was called The Electric Boat Company.
They were called electric boats because they worked on electricity made by the generator. The first thing he did was, he got a job down in Connecticut, working on some of the first-generation nuclear subs they were building in Groton. But he wanted to be back in Massachusetts, so he got transferred to the Quincy shipyard. I forgot how he met my mother, but she was a Quincy resident with all the Irish there. In the early days of my father's work, right about the time he got married, he actually worked on the first nuclear surface ship the United States built. It was called the USS Bainbridge. It was launched in '61 or '62 in Quincy, Massachusetts. I have a photograph of me in the captain's chair of the first nuclear warship. [Laugh] Then, he worked on the USS Long Beach, which was the first guided missile cruiser, also nuclear-powered. Eventually, the Navy decided not to do that. But he spent his career working with nuclear reactors and stuff like that.
He was a Depression-era guy, very practical, mechanical engineer. Eventually, I wanted to become a scientist, and I don't think he really understood the value of science. Like, "What am I supposed to do? What kind of job am I supposed to get?" My dad grew up on a farm, which was a tough but good life in Massachusetts. My mother grew up in a very large poverty-stricken Irish family. She got a full scholarship to go to Harvard, but it fell apart after the first year, and she dropped out. She never got a degree. My mother loved books. She loved learning. Books were everywhere if you wanted to read or do anything. There were four of us, and we're baby boomers, so it's a big family. We were all going to go to college. My father brought us down to a small town in eastern Massachusetts on the south shore.
A lot of wealthy people live there, but we were middle-class folks. I had a brother and then two sisters. And it was wonderful growing up, even though it was the suburbs. And I think this had a huge influence on me as I grew up. We were next to the ocean in the most beautiful spot you could imagine. We didn't live on the beach, you have to be a millionaire to live down there, but we lived on a hill, and we could actually see the water. Growing up with my friends, we'd run around in the woods, along the ocean, explore, things like that. It was an incredible time to grow up. Like many baby boomers, our parents couldn't care what we did. [Laugh] We spent the whole day outside, then you reported back at the end of the day.
ZIERLER: What kind of schools did you go to growing up?
GURNIS: Public schools. Even though we grew up in a town of 7,000 people, there were laborers who had houses in disrepair, there was the middle class, like my father, who had a house built, but then there was all this old money. I've worked since I was 13 years old, and I had my own–at the time, I didn't view it as a business, but in some sense, it was. I did everyone's yards and things like that. I did it after school most days, a little bit on the weekends. I was always working. Everyone worked at that time. The worst insult you could ever get hailed at you back then, you'd be walking down the street, and a car would go by, and they'd yell at you, "Get a job." It's like, "What the hell are you walking around for? You should be working."
That was the ethic. My father was saving up for my siblings and I to go to college, but by the same token, everyone was expected to work and save money. My parents grew up in the Great Depression, so we had to save money all the time. But I went to public schools, and they were good schools. They were really good. It was an elementary school, middle school, then a combined junior high, which is seventh and eighth grade, and high school. I was so interested in science. I don't know what it was. I even remember in middle school, we had a science class in the sixth grade, and I just couldn't wait until I would be able to do that kind of stuff, get into a classroom where they'd actually talk about science. We had a science fair in the fifth or sixth grade, and I didn't do very well in it. I built a whole bunch of model airplanes, but it wasn't anything clever or anything like that. I had no guidance.
But I knew I was very interested in that. I would just tinker all the time with science myself. I had a telescope, I built a telescope, I had a chemistry experiment and didn't burn the house down. But I also had my own projects. Even though my high school had nothing organized, I participated remotely in some things. I did some that were more planetary-science-based, where I would order these photographs from NASA. I had my own money because I worked, and I actually had enough money that I could send away and order these photographs through these digital repositories. You've got to figure it out, write lots of letters. They'd send you this crap, and you'd figure it out. There was no internet. You'd go through all these manuals, get this dataset. And you could order things.
You could actually order photographs of the planetary surfaces. I worked on cratering, and I submitted it to several regional science fairs. And I actually did quite well. As a sophomore in high school, Boston University put on some sort of competition in astronomy, and I got third prize or honorable mention, something like that. It was a low-level kind of thing. But I did all this by myself, and I didn't have any help whatsoever from the science teachers or anything. It was all done independently. I did everything independently. My parents knew I was doing all this. And I'd mention it to friends and stuff like that.
ZIERLER: And there was no internet, you couldn't Google anything either.
GURNIS: Are you kidding me? There was no Google. I was very good at the mail. And that's why NASA was so incredible, because everything was dirt-cheap, even on my budget. I still had money. If I wanted to buy these things, I would. You'd order a particular frame, and they'd make a print, 8.5"x11". But with my telescope, I even started to make photographs of the moon in different colors, combine them, stuff like that. It's a very common technique in remote sensing, where they photograph the Earth, the planets, or whatever in different colors, and I tried to experiment with it myself. I don't think there was anything brilliant about it, to be honest, but I did it all myself, tried to build everything myself, put everything together myself. Then, in my senior year, there was what's called the Science Talent Search. It used to be supported by this company called Westinghouse that made nuclear reactors, dishwashers, things like that.
I was bold enough to submit my project to this in the beginning of my senior year, and I had no help from anyone. I ended up being one of the top 40 in that particular year, '77 or '78. I said, "Holy smokes." All of a sudden, I was a senior in high school, still barely any acknowledgement, and it was never really acknowledged by my high school, interestingly enough. I was selected and went to Washington in the group of 40. I was one of the winners. And that was an eye-opening experience because one thing I realized when I went was that they all seemed way smarter than I was, but then everyone else was in a program. There were, like, three from Stuyvesant and four from the Bronx High School of Science. There were at least 10 kids in the 40 from New York City from these two schools. All these other people had all this stuff in their high school curriculum, all these mentors. That was one thing I realized. I felt like a fish out of water. "What am I doing here? I have no idea what I'm doing here." It was kind of cool. I got to meet some interesting people. I got to meet Frank Press.
ZIERLER: Did that leave an impression on you?
GURNIS: It did. He totally torpedoed my project at the National Academy. And later in life, I got to meet Frank. I was very polite to him, I don't think I brought it up. I talked about these color differences on the moon, saying there were these color differences one could see between blue and red. I thought it was a large geological structure that hadn't yet been identified. But it hadn't been talked about, and he said, "I never heard of that." Most people, I don't know what they were talking about. It was mostly chemists. There was Glenn Seaborg, and he discovered a number of isotopes. I got to meet him, I got to go to the National Academy of Sciences. And because Jimmy Carter was president, for the first time ever, the group of the Science Talent Search winners were invited to the White House.
We got to walk from the hotel to the White House, and it was probably the most memorable experience in my entire life because that morning, it was 1978, the United States was in the middle of this massive coal strike. We were reaching this point that industrial production was starting to come down, and there were these rumors that the president was going to invoke this act to make all the coal miners go back to work. We walked across Lafayette Square, and it was a fortress. I've been around the White House, I know the area very well, and I've seen a lot of police and armed people, but I'd never seen anything close to this. They were so worried the coal miners would violently react. They put up so much, I couldn't believe it. There were phalanxes of horse police, streets with hundreds and hundreds of police cars, armed guards everywhere. We walked through all of this stuff and made our way into the West Wing, then we went into this room. Different people got up there.
Frank Press introduced us, Glenn was up there. Then, all of a sudden, it got really, really quiet. Jimmy Carter came through the door, and it was like an explosion in there of flash bulbs and cameras. I had never heard anything like this in my entire life. We went from dead quiet to like the 4th of July. The room exploded in light. It was weird. It had nothing to do with us or Jimmy Carter, except that literally one minute before this happened, he invoked what's called the Taft-Hartley Act. It's a very martial, imperial thing. He basically could order certain businesses back to work, period, or you'd be arrested. It was the most aggressive thing he had done in his presidency up to that point in time.
It was a very worthy event that had nothing to do with us. We were just there. Probably not even mentioned in the newspaper. We then got back to our hotel rooms and could see the big headlines on what'd happened. But I was in the front row, and Jimmy Carter took my hand, shook it, and said, "Thank you, Michael, for your contributions." But it was weird because he took your hand, and it was so limp. I couldn't believe anyone's shake could be so limp. [Laugh] I later discovered that you can read about all this stuff on the internet. You just download the minute-by-minute events the president does, and you can see what happened, what room we were in, etc. That was exciting.
ZIERLER: Between your mom's Irish heritage and your father's Lithuanian heritage, what kind of cultural traditions did you have in your family growing up?
GURNIS: It was definitely not overly Catholic. We all went to church, but I stopped. After several years of me telling her, "God doesn't exist. We came from the Big Bang," my mother gave up on me. [Laugh] And she's smart, so she knew there were these other views out there. She went to college, and she read all the time. I probably had the strongest views in the house about atheist views. But no one said, "You're going to go to hell," or anything like that. No one was oppressively religious. But yeah, there was a lot of Irish-Catholics around. It was sort of middle-of-the-road, education was important, sports was important, frugalness. The outdoors was really important. It became very important, the outlook on my siblings and myself, interestingly enough.
My dad always thought vacations should be camping, so he would take us up to primarily New Hampshire, then every alternative year, it would be something further afield, Maine, Nova Scotia, Canada. But primarily, New Hampshire in the mountains, camping, things like that. I developed, even at a very young age, this appreciation of the outside and walking. And my brother did, too. I think my brother, who's older than me by two years, was like this. My brother and I were more thinker, outdoorsy types. But the older of my two sisters became quite an accomplished sailor in high school. She was in the national championship, and she crewed with a guy her same age, and they came out here to San Diego from Massachusetts to compete in a national competition. And my youngest sister was a real sports fanatic. I'd say we were more bookish and scholastic.
ZIERLER: Despite the working-class roots.
GURNIS: Yeah, and a lot of that has to do with my mother, I believe. And the fact is, my father had this cutting-edge field of work. High tech didn't exist back then, but he was at the cutting edge of technology. And he just sort of got into it. He was practical, and the country needed a lot of engineers during and after the War. He got into one of the coolest subjects. And my mother was really smart, she could've done a lot. She always wanted us to succeed, study, do book-learning, things like that. I think my mother wanted us to achieve what she hadn't herself. Everyone went to college. Some of us went further than others. Eventually, my brother became a teacher. He's married and lives in Maine.
His wife is the headmaster of one of the big private academies in the state of Maine. She's been a headmaster in other schools in the country. And he wrote poetry and got a master's degree. Clearly, because of my mother, there was a lot of intellectuality. Lots of books. We had the World Book Encyclopedia. I had looked at every single page, all of those 5,000 pages. All of a sudden, at dinnertime, something would come up, and I'd say what the capitol was, what the principal product of a country was. "Where do you know that stuff from?" "There's the World Book. You start at A on the first page, then there's Z 5,000 pages later." I didn't read every single article. "That's an interesting article. That's not interesting. This one's interesting." There were pictures with captions. It was great. But I think a lot of it had to do with my mother.
Developing Interests in Seismology
ZIERLER: When it was time to start thinking about colleges, between geography, family finances, your grades, what was in range? What kinds of schools did you think you could get into?
GURNIS: I was not the best student. I studied hard, but I also goofed off with all these other things. I was a goof-off in the sense I'd spend so much time on all my little projects. Because of that, I wasn't going to get into Harvard. That was what everyone talked about. "You've got to get into Harvard." Not in our family, but kids in school or the neighborhood would go to Harvard. It had to be a good school academically, but something right in the middle. I went to the University of Rochester for a year and despised it. There were all these people from New York there, so I wanted to get out of there. I went to the University of Arizona. It was out west, and I knew Arizona was a big place because of astronomy and planetary science. I knew that was a thing to do. I then, on my own accord, up and went to the University of Arizona.
I didn't really consult too much with my parents about it because I was away in New York, again, just writing away, getting stuff in the mail, corresponding, things like that. [Laugh] Actually, that year, I had a very close friend, and he went to the University of Arizona as well. But he went there as a freshman, I went as a transfer student, a sophomore. I saw the bill from the University of Rochester, and I said, "I don't know about this. This is unbelievably expensive." My dad seemed to be willing, and we got some loans, and they paid the first year. But then, I went to the University of Arizona. There, I was determined to do everything myself. My dad paid maybe the first semester. Then, for the rest, I got loans. And they weren't very much. I started there in 1979, so the tuition wasn't gigantic. I would get these loans underwritten from the government. Then, I started to work immediately.
Within six months or so, I had hooked up with these faculty members in this place called the Lunar and Planetary Lab. I wanted to do planetary science. I absolutely wanted to do it. When I did my project with the Westinghouse Science Talent Search, I wanted to do planetary science. And I thought it was so cool because it was geology, but it was as astronomy at the same time. And I think that was the draw from the University of Arizona. Up until a few years ago, there were two places in the country that walked on water in planetary science. One was Caltech, and the other was the University of Arizona. And they built this Lunar and Planetary Lab, which is really one of the top programs in planetary science. There was a guy who died before I was there named Gerard Kuiper, like the Kuiper belt. He was a Dutch astronomer, and he was an oddity because he wanted to do planetary science. He built this big interdisciplinary team at the University of Arizona.
And I knew about one of the guys. There was this guy who was an old man even at the time named Ewen Whitaker. I knew about his research because when I did my Westinghouse thing, I sort of followed his papers. He was doing remote sensing, and you could only do it for the moon because that was the only big thing you could see. I corresponded with him by letters. He was Scottish, a protege of Kuiper, one of the first people Kuiper brought in to help him do all these fantastic things with the moon, taking lunar photographs, things like this. But once I got there and spent time talking with Ewen Whitaker, he really was in the team of one of these guys with the research group. His name was Robert Strom, and he became quite prominent because he sort of led all of the interpretation of the photographs of Mercury. Later in life, he may have been the top scientist on the Mercury probe. I got to work on Bob Strom's team.
I was this industrial guy, working hard. Those guys took me totally under their wing and hired me. Seemed like I worked full-time for these guys, and I published a bunch of papers with them around 1980. Because of that, between working full-time with these guys and getting these loans, I put myself through college. But along the way, I kept reading. There were two things going on that had a big influence on my career. I started to read in geophysics. How did I get there? I figured if you're going to become a planetary scientist, you've got to be a physicist. What better way to get this background that was traditional and solidly fundamental, a good background for planetary science, than geophysics? I pursued this geophysics degree and suddenly became totally fascinated by it. But simultaneously, I was beavering away in Bob Strom's group, and these guys were such downers.
This was from about 1980 to 1982. Suddenly, the NASA and planetary budgets, instead of going up, went down. These guys were still loaded with money compared to today, but these guys started to question where planetary science was going, and this really had a negative impact. I was doing all this reading in geophysics. I was already fascinated by the Earth, starting to get fascinated by physics and applied math. I said, "This is cool. That's the direction I want to go." I made wrote all these papers in planetary science, then I just ducked it. I was trying to figure out what to do. I was working with this guy, Alex Woronow. Alex was in Bob Strom's team, and he was a senior scientist, really smart. He was a compute guy. He did all his work by computer simulation. I have this single-author paper as an undergraduate, where I developed all my own Monte Carlo models and analyzed images of Mars. The whole idea of using a computer to look at these complex physical systems just fascinated me.
Then, I started to figure, "I'm interested in geophysics and the Earth, and I'm interested in computers. What should I do?" I started to read papers and books and talk to people about two topics. One, the subject I went into, mantle convection. The other was about climate change and post-glacial rebound. I was really fascinated by this whole area of thinking that the Earth was changing its orbital configuration, causing climate change. Then, the ice caps came and went. I said, "That could be really interesting." I knew there had been work that had been done on Mars on the same topic. And I could see this Keeling curve of CO2. I said, "When I'm old and dead, this world is going to be really in tough shape. But thank goodness it's all going to be when I'm dead." I did not see the urgency in 1981 when I was making these decisions about going in the direction of the climate, which is one of the two things I was going to do.
Instead, I was going to go in the direction of mantle convection because several years before, plate tectonics was discovered, and this area of trying to understand the physics of the Earth's interior looked like it was taking off. That's what happened. You'd think I would've pursued a planetary science degree. In fact, it probably would've been very successful for me because I was right there at an early stage. And it eventually totally turned around and has taken over the world. It's going to take the GPS division over. [Laugh]
ZIERLER: When exactly does seismology enter the scene for you?
GURNIS: I took classes. Seismology was not taught in the year I needed to take it. In some areas of geophysics, my classmates pissed me off royally. All my classmates were master's students. They were [in the] physics, math, and geophysics courses. It seemed like we were all in the same classes. They did it because they were all interested in going into the oil industry. I later actually did a lot of work with oil companies, but at that time, I liked the outdoors and hiking, and I knew there'd be this thing called climate change eventually, and the oil industry was doing all this drilling, destroying everything. At the time in Arizona, there was this guy called Edward Abbey who wrote The Monkey Wrench Gang. I got to go to a rally with him, which he led. It was anti-Ronald Reagan, and we did this demonstration when Reagan's Secretary of the Interior, James G. Watt, came through.
But anyway, I was so anti-oil company, but all my classmates wanted in. This was 1982. Finally, the way to get us out of the whole oil crunch that started in '73. There was so much activity in the oil business. The year I graduated, the top job in the country was a petroleum engineer and second was geophysicist. But I was fascinated. These people wanted to get the education to get the job, and I was taking the same classes, and I was mesmerized by the physics and math classes and how it all connected together. There's this whole way that physics, partial differential equations, and applied math all kind of merge together, and you can't really see it all until you've taken a lot of different things. You reach this point where it's like an epiphany. At this time, in 1981, I saw this and said, "Whoa." I wanted to go into a field where I could do this kind of stuff, yet do something about the Earth with computer modeling.
That's why I pursued this direction of mantle convection. But I was still a troublemaker because I spent all this time writing these papers as an undergraduate, so I didn't have a good undergraduate résumé either. [Laugh] I was like, "What am I doing? I just keep repeating myself, taking this direction that doesn't seem to work out." There were some geophysics faculty members, and I had an advisor at the University of Arizona named Randy Richardson. Interestingly enough, he did computer modeling, mechanics, and things like that. He had just gotten his degree a couple years beforehand from MIT. He was a student of this guy, Sean Solomon. He eventually became director of Lamont.
Randy said, "Man, you're a troublemaker. We've got to send you to another troublemaker. You've got to go work with this guy, Geoff Davies." He was a recent Caltech geophysicist graduate at Washington University in St. Louis. I was corresponding with Geoff because he was in exactly the field I wanted to be in. Randy said, "You guys are going to hit it off. Go work with him." We were corresponding, [then] on the telephone. Here I am, an undergraduate at the University of Arizona. Geoff said, "Mike, can you keep a secret?" A lot of people can't keep secrets. "You've got to keep it a secret. But if you come here and work with me, I'm going to leave, and I'm going to go to the Australian National University."
ZIERLER: I was waiting for this, how you got to Australia.
GURNIS: Interestingly enough, I knew about this school. I couldn't believe Geoff told me this because I was reading this book called Composition and Petrology of the Earth's Mantle by A. E. Ringwood, who wasn't a physicist, but a petrologist. He was a really famous Earth scientist in Australia. There was an Institute for Advanced Study at the Australian National University, and they were in this place called the Research School of Earth Sciences. I corresponded with them. They had geophysics, too, and wrote back all this material. Suddenly, it was really ambiguous how to go to graduate school. I said, "This looks really cool. They're doing all the things I'm interested in. Maybe I should go there." And I didn't know much about Australia, and I didn't know how to apply. It said to write to a professor. Well, I should've, except the ones in geophysics, I really didn't like their research.
But I was drawn more by this other guy, Ringwood, who I later became an enemy with. He was one of the most controversial figures. Brilliant scientist. Geoff tells me, "I'm going to Australian National University, and you can come. But you've got to keep it a secret." Then, we finished up the stuff with Wash U, they admitted me, and even though I didn't do very well, they gave me this top fellowship where I got paid more than the other students who were named. I got to work with Geoff. And I totally kept this a secret. We started to work together, and we worked very well. Of course, he's extremely independent and did his own stuff, and he let me be independent. I'd get help from him and bounce ideas off him. Then, halfway through the year of being at St. Louis, Geoff said, "I got this offer, so you're going to hear around the department that I'm going to be leaving. Are you still interested in going to Australia?" I said, "You bet." I still didn't know anything about Australia.
They put all the first-year students in one big room. I was studying away, working hard in there, then all of a sudden, the door opened, and all the graduate students came in, roaring, "Hey, Mike, do you know Geoff's leaving?" Suddenly, the department was in an uproar because their star faculty member was leaving the department. "Mike, what are you going to do?" I said, "I don't know." [Laugh] That's how I ended up going to ANU. And I loved it. That place was in its golden age when I went there. It was like a self-contained institute. They had so much money for Earth science. You didn't write grants or anything, you just went there, and everything was covered. They built all the top instruments for mass spectrometry in the world. Even when I was a graduate student there, they got so far ahead of the curve that no one could even approach them. They were dating the oldest rocks in the world, doing all these other high-pressure experiments in Ringwood's lab, doing all of this geophysics and seismology. We were working on plate tectonics, and we were having this discussion.
Geoff goes, "Mike, you don't know much about plate tectonics or seismology, do you?" He had a seismology degree from Caltech. He gave me this book and said, "First, you read this." It was written by Bruce Bolt, and it was more like a monograph for scientists, but it wasn't super technical. I was reading it, and I got to this whole section about the interior of the Earth, and the narrative that this guy then portrayed–he was talking about seismic waves piercing into the interior of the Earth and being used as a remote sensing technique. And you'd be almost like a detective with this wave going down and seeing the unseen as a detective. Just the way he wrote about it, I said, "This is incredible." Simultaneously, there was a group here in the Seismo Lab with Don Helmberger, who I later became very close with scientifically, and one of his students, Thorne Lay, who became very prominent in the field. Don and Thorne wrote this paper and had discovered this new part of the deep interior. It had already been named, but they finally put some clues to it.
It was called [D"] D double-prime. But this paper they wrote–I wasn't yet excited by earthquakes and seismic waves, but this paper Lay and Helmberger wrote came out shortly after I'd read Bruce Bolt's book, and I was like, "Oh my God, this is incredible." Because we didn't know what this layer was that Helmberger and Lay had discovered. They thought it was the crust. We, then, started to write papers and work on this problem right away, and it was like continents, except you'd put them on the bottom of the mantle. In fact, Geoff and I almost wanted to call it the anti-crust. We said, "We better not call it that because the religious fanatics will think it's called the anti-Christ." [Laugh] Basically, we argued that they were like the opposite of continents. Later, we determined that this model was totally wrong. But then, I suddenly became fascinated with Earth's structure and Caltech. Because I realized that all the discoveries of the interior of the Earth, every single one of them, pointed to one place, which was here. Then, all I wanted to do once I was finishing my degree was come to Caltech.
Geoff was an alumnus, and there were a bunch of other people at the ANU who were from the division or the Seismo Lab. There was a very strong connection. Then, when I decided to become a post-doc, I got a lot of offers, but there was only one place I wanted to come, which was here. Even someone I thought I was doing intellectual battle with, Don Anderson, in reality, a lot of it was because of his ideas, even though I disagreed with him, and what he had done with students here at Caltech. That's the great thing about academic discourse. Even when you have contrarian views, the best thing to do is to get in a room, discuss, and compete with one another. And Don was always incredibly supportive of me, even though I was advancing a vision of the Earth's interior that was very different from his own. He was very supportive in talking to me and sending me off in directions that were very helpful.
Debates Over the Interior of the Earth
ZIERLER: Before we leave Australia, tell me a little bit more about how you developed your dissertation topic.
GURNIS: The big debate that started here at Caltech and other places in the 1970s, largely because of Don Anderson here in the Seismo Lab, but also because of geochemistry at Caltech, there was this view of the interior of the Earth that the whole interior of the Earth was stratified. Plate tectonics, subduction, but then this region in the upper mantle all the way down to the transition zone, down to 660. And then, below that, we have no earthquakes. That's the lower mantle. It's the largest part of the Earth by volume. This debate between upper-mantle convection and whole-mantle convection, either there was this strict layering at 660, or there wasn't. That was the big holy grail of geophysics, between upper-mantle and whole-mantle convection. And Geoff Davies and Tom Jordan at USC, another very prominent Seismo Lab graduate, were on one side of the debate, the whole-mantle convection.
But 95% of the community, most of the physicists, and all the geochemists were on the layered convection side. But there was a lot of stuff that just didn't piece together. And Geoff then started to formulate the hypothesis that you could reinterpret the geochemistry in terms of whole-mantle convection, but it required one to put this on a physics basis, understanding how convection would work and how geochemical anomalies would be transported in the mantle. Then, I developed the fluid-dynamic basis and the geophysical basis by which to do that. That was specifically my thesis topic, to bridge this gap of taking the whole-mantle model and seeing if I could connect it with geochemistry, with different studies I did under the mentorship of Geoff, trying to interpret the Earth in terms of whole-mantle convection. Even by the time I finished my thesis and came to Caltech in '86, the consensus was still with layered convection. But I stopped that immediately and started to work on other topics.
That topic eventually got resolved by the Seismo Lab, primarily, I think, the best work was done by Seismo Lab graduate Steve Grand, a professor of geophysics at the University of Texas. You could just see in his PhD thesis all of the plates returned to the interior of the lower mantle very clearly, just like Geoff's model. Geoff was vindicated by the seismologist in a famous paper that was written in the mid-1990s, and Steve Grand coauthored it with Rob van der Hilst, who came from a different academic tree than Caltech. That was sort of the nail in the coffin.
ZIERLER: I'm curious, in graduate school, were you following any of the debates and some of the optimism about earthquake prediction that was happening?
GURNIS: None at all. In fact, I keep hearing things. Lucy Jones keeps telling me these very interesting stories. Exactly at the same time when a lot of this deep Earth-stuff was happening in 1970, '71, '72, '73, there was a lot of work that was done, even at Caltech by Don Anderson, which I only discovered this last year, about how they could use some sort of anomalies in P- and S-wave speeds to predict some sort of dilatation. One thing I was familiar with, though, and it also feeds into that, in the 1970s and into the 80s, there was this thing called the Palmdale Bulge. Based upon some work that I believe USGS scientists were doing, they used these two-color light beams, and they thought near the San Andreas Fault that the Earth was being tilted one way or another. Eventually, this guy, David Jackson, who became a very prominent graduate of Caltech and professor at UCLA, long since retired, was the real person behind that.
Then, there were several other graduate students. In fact, the other interesting thing, and part of the reason this came up with Lucy Jones, is, we're doing this public thing for the centennial called Shaking in Your Seats. It's a big outreach event Caltech is going to do with movies and stuff like that. But we discovered that some of the comments that were made in this 1974 movie called Earthquake. It had Caltech graduate students in it with a Caltech Seismo Lab director. But it sort of, in an indirect way, hints toward this idea of the wave speeds. But it's interesting, it's part of the Seismo Lab history. I think Bob Geller authored these papers. Bob's a smart guy. He worked on earthquake prediction, but then he became the biggest thorn in the side for the Japanese because he believes it's something we should strive for, if I understand him correctly, but on the other hand, it has to be put on a fundamental science basis.
If you can prove everything on a fundamental level, you can take it to the next level. I believe his concern was that a lot of the work never panned out, so he couldn't put it on a fundamental basis, so all the other stuff we're doing about earthquakes, there's nothing fundamental about. I was clueless about that. In fact, a lot of my work over the years came from a lot of the images of the structure of the Earth that came out of the Seismo Lab. The other redirection I took in my own science is, when I arrived in the Seismo Lab, I was nominally supposed to be connected with the Hypercube project after parallel computers were discovered at Caltech. I was going to run the computer codes and write the software for these computers. But in parallel, I was going to put tectonic plates into convection models, which really hadn't been done up to that point.
As I was thinking about all of this–and I didn't do this when I was a post-doc here, but I did it when I was an assistant professor with a graduate student. But it all came out of sitting in coffee, listening about earthquakes, and then reading Hiroo Kanamori's papers about great earthquakes. Then, I would be simultaneously reading these papers about mantle convection. They're totally on different topics and different ways of approaching problems. I decided I needed to bring them together. And that was what I did, one of the accomplishments I've made as a truly independent scientist. The Seismo Lab, the students, all the work they did subsequently around the country and world played a huge role in my thinking.
ZIERLER: When you were thinking about post-docs, was Caltech the be-all and end-all? Is that really where you wanted to be?
GURNIS: No. There were competing ideas that were working in my head. It turned out, there were three of them. One, from a geophysics perspective and the perspective of the interior of the Earth, I wanted to come to the Seismo Lab because of its intellectual atmosphere. And mostly, that was just people interacting with me at the ANU, telling me about Caltech. I spent most of my time reading papers from Caltech. [Laugh] I wanted to come here. But it turned out there were two other intellectual threads that played a big role in my thinking at the time. The need for supercomputing in the mid-80s had just exploded. The whole debate was about how to get access to the fastest computers in the world if you're not building an atomic weapon. I wanted access to the fastest computers in the world and wanted to be involved with teams that were involved with this work.
And I didn't know what to do. In addition to writing to Caltech, I also was pursuing the possibility of going to Los Alamos. I could've gone probably through connections through Gerry Schubert at UCLA, started to develop very strong linkages with Los Alamos. In hindsight, I'm glad this happened, but I narrowed my sight. They only had a few open fellowships, like the Oppenheimer Fellowship, and those things are very competitive. There are hundreds and thousands of physicists competing for these things. Luckily, in hindsight, I didn't get it. But I was still really worried about this connection with supercomputing. We had the geophysics intellectual one, then there was the part of supercomputing, then, it turns out, there was a third intellectual thread, and that was plate tectonics. That's ultimately one of the big things I did as a scientist, merge the observational basis of plate tectonics with a theoretical one.
I was reading all these papers from this other place called Lamont-Doherty at Columbia University. Then, someone had photographed this four-issue article in the New Yorker, put it in a binder, then put it in the library. It was about this guy named Doc Ewing. He was a seismologist at Lamont, and he built Lamont. He made Lamont. But a totally different story was portrayed in this article. It was all about science and about this really hardworking, driven scientist, Doc Ewing, who'd take these boats around the world, make all these measurements, and assemble all of this data. The other weird thing I was doing at this time, besides reading all of these papers, I was reading all these books about sailing and exploration. I said, "Oh, man, maybe I want to go to Lamont." I also applied for Lamont. People would later say, "Why didn't you come to Lamont?" and I'd say, "I applied, and I was rejected." I realized in hindsight, it was because the year before, they gave this fellowship to a person in my same field, someone who did computer modeling.
They weren't going to give it to a person in the same field. I only did computer modeling. I had no basis for making a measurement. I was a little bit disappointed. But then, they wanted me to come to Caltech. And they wanted me. I met with this guy, Brad Hager, at the AGU, and he came up to me, "I want you to come to Caltech. You've got to come to Caltech." I said, "Whoa, I've got to go." I looked up to him enormously. He was just a little older than I am and was making great breakthroughs at the time. He left for MIT shortly thereafter. Other places offered me jobs, and a lot of people wanted me, but it was Caltech. Then, there was all this commentary in the pages of science magazines about, "Academic scientists don't have any access to this computing". What are we supposed to do? NSF is trying to get their own supercomputers that are not connected to Los Alamos. But because they'd just discovered the parallel computer, and it was going to revolutionize computing, and it was at Caltech, and I could be involved, suddenly, two of those three pieces came together, and there was no question. As this unfolded, there was no question what I needed to do.
The Impact of Parallel Computing
ZIERLER: Tell me specifically what was so revolutionary about parallel computing for you at that time.
GURNIS: Because I was a person who thought with numerical methods, we did the finite difference method at the time, which people still widely use to solve partial differential equations. But the whole basis for these numerical methods is to imagine physical space is broken up into a whole bunch of smaller pieces. The whole idea is that you can now, with hardware, represent the physical situation. You have a big computer, and different parts are in demand. It played such a huge role for me. One of my first pictures I made when I was at Caltech back in the mid-80s was this, and it'll hammer home the point. This calculation can be done on my iPhone in about a billionth of a second. [Laugh] But this was the first geophysics calculation that was done on a parallel computer. We're looking at a picture of thermal convection. Yellow is hot, and you see along the bottom, there's a yellow thermal boundary layer. Then, on the top, there's this blue cold thermal boundary layer. This is your cartoon view of normal thermal convection, in which this goes up, comes around, and goes back down.
On a much finer scale, with the numerical method, there's a grid. And the equations of conservation of momentum and conservation of energy are solved on this very fine grid. It turns out that this was literally done on a 32-processor computer that was built here at Caltech. Each one of these little squares was done on a different computer. They were all actually done on the same computer, but with different processors, literally different chips, the same chips that were in the first IBM PC. The computing was done on one processor, which communicated with other processors. I even made pictures like this. This is a time dependence to watch a classical problem, convective instability. Here, it's going convectively unstable, then at a different time, it overshoots, then we reach steady-state convection.
But this is why I was so possessed by parallel computing. And I was one of few people in geophysics who was doing this, and the only one in my field for a long time. This was actually not that state-of-the-art, this calculation. I did somewhat bigger calculations, but I demonstrated that it could be done. It turns out there are lots of applied math problems that explain why this didn't work very well either. But in the same year I did that, I did these calculations. This was 1988, I was doing this as a post-doc. But I did this because after I got to Caltech, the National Science Foundation built these big supercomputers, and they bought one -- down in San Diego -- from Cray Research. I got on this system, and we were able to communicate. We put a radio antenna over at the computer building that we could use to communicate through an early version of NSF Net.
I could work here in my office and send jobs down there. But I would go down to San Diego, too, and I made one of the first convection movies, and it was on the front page of Science News. I could do interesting geophysics, but I had to use the NSF supercomputer, and it was the same couple years we were building these new algorithms. But it was more like a proof of concept. Over the last several years, we've gone to astronomically large number of compute nodes, but at the time, I bought into it. I read these news articles and Scientific American articles about what parallel computing could do. And I imagined I could do it at Caltech and wouldn't have to go to Los Alamos. I was worried about coming to Caltech since Los Alamos was a fascinating place with a long, storied history. That was the good thing about coming to Caltech, it was this intellectual thing, and I thought I could do the computing thing. Through the National Science Foundation facilities and just being clever, I was able to also do some geophysics and some pie-in-the-sky stuff that wouldn't have big payoffs until decades later.
ZIERLER: When you got to the Seismo Lab as a post-doc, what were some of the big ideas?
GURNIS: There were a lot of arguments about [D"] D double-prime, the very bottom of the mantle. Ultra-low velocity zones were getting discovered. It was just everything. The way earthquakes were working, what earthquakes looked like, what all these layers were like in the mantle. It was interesting, there was a period of time in there, and I think it was about this period of time, from about 1990 to 1995, about the time I left the Seismo Lab, it was kind of quiet. I could tell it even in my own field. It was like this in seismology and whatnot. The reason was, with the simple computers, a lot of people did cool stuff. But to make the next big jump, you needed even bigger, more algorithms, more compute power. Then, from the observational perspective, in terms of seismology, they were deploying all these new seismic networks, but they hadn't yet been totally crystallized.
One of the things that was endlessly debated is what convection looked like inside the mantle from seismology. Forget about what the computer models I was doing said. I think the robust things we were arguing about there ended up being the robust things. The powerful person at the time was Don Anderson, who was director of the Seismo Lab, and he was Mr. Layered Convection. He was going to absolutely have layered convection no matter what, even though all his students, by the mid-90s, had come together. In this period of time, there was lots and lots of debate, but there was lots of argument because the data just couldn't convince people one way or the other what these structures were like. The other thing that added friction into the system was the fact that just before I came, Hiroo Kanamori had just made this big intellectual leap on the nature of great earthquakes.
Mostly, it had to do with his ability to get the moment magnitude scale that didn't saturate so we could see how big earthquakes were. There was a lot of discussion in coffee about the great earthquakes and whatnot. Yet we hadn't seen any. It was debate about all these older earthquakes. They were deploying all these seismometers around the world. The Global Seismic Network had started to be put in. These were very expensive broadband instruments they were putting in around the world. They weren't putting them in locally, like here in California. And we didn't have any great earthquakes. The Earth went quiet. This is the best way to stop an earthquake, give geophysicists a lot of money to put instruments in. The Earth will just shut up. That's a joke, of course, tongue-in-cheek.
We'd put this big observational system in, and just nothing. When we finished our last conversation, I said that we had just finished up a golden age of earthquake science, and that golden age of earthquake science sort of started in the late aughts, '07 or whatever. Then, the Earth lit up again with great earthquakes. But of course, we had already figured out the depth of mantle convection, or we at least knew it wasn't upper-mantle convection anymore. Those came in by the mid-90s, the Global Seismic Network had resolved. It's interesting, things definitely go up and down. Things get really exciting for a while, new instruments go up there, sometimes there's a little bit of friction in the system because the data is not there yet. Then, all of a sudden, things explode, the paradigm shifts. But at that time, there was basically a lot of arguing in the Seismo Lab. I would argue with Don Anderson about the depth of mantle convection and what the seismic images showed. We were discussing, but probably not arguing about, what any of this fine-scale structure was like. Those debates heated up more several years later when the observations got better.
ZIERLER: Hearing the stories about the old Seismo Lab up in the Hills, we talked a little bit about this last time, when you joined as a post-doc, was it still a place that would attract the top people in the field with proprietary data that drew people in because the internet simply wasn't around yet?
GURNIS: Absolutely. That's actually quite interesting. When I came, in terms of datasets, I think the draw was just the people. But it is interesting, it comes and goes, the draw of the Seismo Lab. When we brought Zach Ross in as a post-doc, we didn't have to recruit him. He wanted to come here because he had this vision. Even though he could get the data on the internet, by being here–we didn't understand what he was up to. But I think he wanted to come here because he would be here with the data itself. But it comes and goes. The big thing that played a really important role for me scientifically was this network called the Global Seismic Network, which IRIS put in, and anyone could get that data. Before that came online and it became easier to get the data around the late 1990s, you still had to order magnetic tapes through the mail. Before that, there was a previous network called the World-Wide Seismic Network [WWSSN] World-Wide Standardized Seismograph Network.
All of this data existed only on microfiche chips, and there were just three open places in the country. One here in the Seismo Lab, the other one at Lamont, and somewhere else. Of course, a lot of people are pissed off at the Seismo Lab because we got rid of ours, but that's their problem. It wasn't a unique dataset, no one was using it anymore, and the people who used it said to get rid of it. But it comes and goes. The places like Caltech and the Seismo Lab, the whole internet works against some of the things that make them unique. There's all this openness of science. Being able to freely move information around, you lose the propriety of certain inside groups where science can really unfold in ways that are quite transformative by a small group of people. And as a historian of science, I'm sure this is a big thing you see. I hate the internet, by the way. [Laugh]
ZIERLER: Being at the Seismo Lab as a post-doc, relative to the other positions you were considering that might've been more broadly geophysical-based, did you get more involved in earthquakes simply as a result of being here than you might otherwise have been?
GURNIS: Yeah, just listening to people, it was just amazing. I just developed an appreciation like I would never have by osmosis. That's it. That's why by the time I left, I was determined to make mantle convection models mechanically closer to earthquakes. That's why one of my first big accomplishments with a student was to put faults in. All my information on faults was coming from earthquake seismologists, not geodynamics. I said, "That's what I want." The way your science unfolds is how the people around you look at problems. I just have developed this appreciation of the perspective of my colleagues and what they've accomplished. Despite people not liking a lot of the stuff that comes out of Caltech or some of the people from here, I say, "So what? I don't care." Maybe it's brainwashing.
But it's definitely the way my science is. But my science is not even close to a cookie cutter of what other people in the lab ever did. I haven't even really talked about what I consider to be my most important scientific contributions, which are more on the geology side of things, sea-level change and long-term evolution. The Seismo Lab really only played a small role there. When I was finishing up my post-doc here, when I wasn't working on these sea-level problems and whatnot, it was Don Anderson who steered me in the direction I went. I was already going to go in this direction anyways, and I was already doing reading, but he sent some papers to me and said, "Mike, you've got to look at these papers, man." It was like, "Holy smokes, I can't believe what Don gave me here." And that played a huge role.
ZIERLER: Which faculty were most important to you at the Seismo Lab during your post-doc?
GURNIS: Well, my mentor was Brad Hager. He clearly played a huge role. Interestingly enough, the role that Brad played was one of computational rigor. We get the equations, we think we're smart, we write a computer program, we solve them, this is the answer. But Brad really pushed me in the direction, the way he would interact with other people in the Seismo Lab. The intensity level here was way higher, the self-criticism was way higher here. A lot of self-criticism about the numerics, the quality of your solutions, and things like that. He instilled in me a huge perspective in this regard. In hindsight, Hiroo Kanamori had a huge influence on me as a post-doc, but I hadn't realized it yet, and it had all to do with connecting geodynamics to great earthquakes.
Reading his papers and listening to him played a huge role. And just watching the way these guys worked played a huge role. They were all geophysicists instead of being theoreticians, even though they were just as good as anyone else in this area of using physics, computing, math. But it was all about what the Earth was saying, too. This is totally empirical. The simultaneity of getting both–sometimes you go and find people, and they're so narrow. And I mentioned before how I annoyed so many people in my field because they were so good at running all these computer models and endlessly making pretty pictures. Then, I'm telling them, "The Earth does that. What do you say?" But in the Seismo Lab, it was always the data first, then, "What does it mean?" But they were from people who were always interested in making sure that the physics was right. Suddenly, the bar was so high, and I learned that bar here. People were tough and competitive, but highly interactive and generous at the same time.
It was just an amazing time. Even though I didn't do the same research everyone else in the lab did. But that was okay, they had a huge impact on me and the ways my science unfolded. And then, I think Don Anderson had a big impact on me but kind of in a contrarian way. Every day, having to go spar with him in coffee, cartoons on the board, observations. He knew everything. Some days, I'd be really quiet because I didn't know anywhere close to what he knew. And at the time, Don Helmberger had zero impact on me personally in working with him. His papers and his students' papers did, but he didn't. It was only when I came back as a faculty member. Then, he became the closest one. Of course, by the time, Anderson was already incredibly famous, but I think his most productive phase had already passed by the time I came back.
ZIERLER: For our next talk, we'll pick up on the opportunities you considered after your post-doc. Last question for today. Was it discussed at all about you getting a faculty position at Caltech during your post-doc?
GURNIS: No, because at the time, they didn't need me because Brad Hager was here. It only happened almost immediately after I left. In fact, the only discussion we ever had here had nothing to do with Caltech, it had to do with MIT. Brad Hager came to me, my post-doc advisor, and said, "I know you're interviewing at MIT. But just so you know, I'm very supportive of you, but they're also looking at me, too." [Laugh] And I'm so happy it worked out the way it did. I visited MIT a bunch of times, and in the end, they decided to hire Brad. Brad became very famous right at the same time, and there was some tension in the division. MIT took advantage of that and got him a chair. Nafi Toksoz, who was a student of Frank Press, took me aside at MIT and said, "I think we're going to try to hire you because it's going to be really hard to hire Brad because we're going to have to give him a named chair." But then, everyone was such a jerk to me at MIT, it was like, "Holy mackerel, who are all these jerks?" All the other universities I'd visited, they all worked hard to be nice to me, even at Caltech. I went to MIT, and they were all jerks.
ZIERLER: And that was next stop after the post-doc?
GURNIS: No, I went to the University of Michigan. Boy, were they nice to me there. There was a whole cadre of Caltech people there. They knew I was here, and they immediately latched onto me. They worked the hardest, and they got me. But MIT never came around in the end. They went for Brad. But a position opened up at Caltech, and they had to figure out who to hire. There were some high-flying people, including myself. I figured out things, not from Caltech but from other people. It was interesting.
ZIERLER: We'll pick up for next time on that opportunity.
[End of Recording]
ZIERLER: This is David Zierler, Director of the Caltech Heritage Project. It's Monday, August 29, 2022. It's great to be back with Professor Mike Gurnis. Mike, once again, thanks so much for joining me. It's great to be with you.
GURNIS: It's wonderful to be back, continuing to talk about the history of the Seismo Lab.
Old and New Questions on the Origins of Rocks
ZIERLER: We're going to pick up in the narrative in your career when you arrive at Michigan. In terms of your own research, what were you working on at that stage? What was most interesting to you?
GURNIS: I think this was 1988. I'd just finished my post-doc at Caltech, and when I was a post-doc, I had figured out a way to study, in a dynamically self-consistent way, mantle convection and tectonic plates simultaneously. One of the things these models allowed was that one could see and study not just the horizontal motions of plate tectonics. The great triumph of plate tectonics was our ability to explain horizontal motion for most of the surface of the planet. It also allowed to explain some but not all of the vertical motions of plates. What are vertical motions? Those are things like when mountain ranges go up, but in addition, there are also many subtle variations in which the surface of the Earth goes up and down. Different continents are at different heights. Some are very low, some are very high. It turned out that most of classical geology before plate tectonics, over the centuries, really sort of worked on three different big things about the Earth.
Of course, it dealt with the origin of rocks, which I won't deal with here, but it also dealt with structure, where some things get squished in a mountain range, and you have folds, and things get pulled apart, and you have other faults. Of course, you have strike-slip faults, too. But another big occupation of geology, besides those things, was the vertical motions. And we see those classically in geology manifested in several different ways. The classic thing is that you find, over vast expanses of the Earth, let's say within a continent, marine rocks. And marine rocks form near sea level, near the seashore, or in a submarine environment. But you can find, of course, fossils that are way up that are at substantial differences from present-day sea levels. Sometimes, kilometers.
In fact, Leonardo da Vinci pointed this out and mentioned that "These marine fossils are way up in mountains. How did they get up there?" Anyone on the street can understand that this is kind of a baffling thing, to have marine fossils at that elevation. And of course, one of the ways we get fossils up at great elevations is, you compress the rocks, and the crust gets thick. We can go into many mountain ranges and find previous critters that were at the shoreline. But within the vast areas of continents, you don't usually find these very spectacular mountain ranges. What you find is just endless. You go over hundreds or thousands of miles from one outcrop to another outcrop, and you see these flat-lying sedimentary rocks. All over the planet. You drive thousands of miles, and you see this over and over again. Then, you find rocks that are way up in the air. Sometimes you can follow these sedimentary horizons, and they're still all flat.
Yet, they're way up in the air. Geologists, starting hundreds of years ago, but more focused about 10 years ago, there were sort of two ideas that emerged in geology to explain the existence of these features. One is that sea level just goes all the way up and down all around the planet. For example, you pour more water into the oceans, and sea level rises. Or the physiography of the ocean floor changes, so they have less carrying capacity, and sea level rises and then falls. That was one idea that existed. Then, another idea was that individual continents moved up and down by themselves. Like, North America went up, Europe went down, things like this. And that has a very technical term within geology, it's called epeirogeny. Geologists were at their throats for hundreds of years that, "You have epeirogeny, or you have this sea-level process called eustasy." And it was like, "You have one or the other." And if you don't, there was arguing.
Even when I was a graduate student, I remember the fiercest debate unfolding was from a set of geologists at Exxon Research Laboratory who developed a methodology of looking at sediments offshore, and they claimed we would see all these simple cycles all over the planet. They were called Vail cycles. Vail actually was a famous geologist at Exxon. One of the reasons it was controversial was that Exxon could never release the underlying data. They felt this gave them a gigantic competitive advantage over oil companies around the world in their ability to explore the continental margins. But within the pages of the journals, this was debated endlessly, the nature of this process. Some people said, "Oh, it was sea level." Others said, "No, the continents were all moving up and down." I'd always been fascinated by this problem because when I was an undergraduate, I went for a year at the University of Rochester, and there was this brand-new geologist–he was a paleontologist.
The classical education of a geologist, in their [first] semester, you take a class called physical geology, like volcanoes, the origin of rocks, plate tectonics, things like that. Then, there's another class, which is very classical, and it's called historical geology. This is the interesting thing about the Earth sciences. It's not just like physics and chemistry. Of course, most of our process-based phenomenology comes about through an understanding of physics and chemistry. But there's this whole other historical realm, like in anthropology and history. You have this historical component. And all of these stories were put together about how the seas came and went over the continents. It was the defining way to look at historical geology because even North America at times was completely covered by water. Even after the Cambrian period, there were different periods of time that this was almost a submarine place.
This was very baffling. I learned about this when I was an undergraduate. I had not really known about this at all. And we went on field trips in both upper state New York and throughout the United States. And over and over again, we'd drive the cars all day long, and we'd get these endless marine sediments everywhere. I'll never forget, in class one time, a student raised their hand and asked the professor, Carlton Brett, "What causes all of this?" And he said, "We don't really know. Maybe it's sea-level rise from plate tectonics or other processes." I just filed that away, that it was a big thing. I couldn't let go of it. After that semester, I decided physics, applied mathematics, and geophysics was the direction I was going to take. And this was a totally different realm than historical geology, paleontology, and sediments. I decided to take this mathematical route to understanding Earth physics.
But then, there was starting to be developed an idea, although the concept first emerged in the 1930s, of something called dynamic topography, the idea that inside the Earth, when you have convection, it pushes the Earth's surface up and pulls it down. Folks sort of came up with the idea that this existed for the Earth today, and it was actually a fundamental breakthrough in understanding the Earth's gravity field. Up to the early 1980s, the understanding of the Earth's long wavelength gravity, which reflects the mass distribution of the planet, was a mystery. In order to resolve this, convection actually had to change the shape of the Earth. Now, all of a sudden, I had invented this method when I was a post-doc at Caltech where I could study the time dependence of convection with tectonic plates, and therefore, see how tectonic plates, as they moved around the surface of the Earth, would go up and down.
Immediately, I said, "That's what I want to focus in on." It had been in my head for six years, and I was fascinated by this historical geology thing. I would bring this up with Don Anderson in coffee, and Don gave me a set of these papers from this guy named Gerard Bond. He was a classical geologist, and he was at Lamont. He wrote a set of papers when he was an assistant professor at UC Davis. Basically, he was able to figure out, by looking at the thickness of sediments, that the continents went through these different patterns of going up and down. And it was so straightforward, his argument, that it was irrefutable. But everyone ignored it. First of all, there was no mechanism to explain it. He actually didn't get tenure, and people attributed him not getting tenure due to him not toeing the line as Peter Vail at Exxon-Mobile. The whole community mostly liked this idea of sea-level change, but then there were these crazies out there, like Gerard Bond.
But he wasn't crazy. He was rigorous, he was smart, he was a field geologist, he measured out in the field. I read these papers, and I went, "Holy smokes. Convection can explain this thing." Then, I got to Michigan, and I immediately started to use these same methods I had applied to understand how continents could give rise to these vertical motions. I did several things, but perhaps one of the most important early things is that the classical model that people were using for sea-level change, essentially, they were applying plate tectonics. In plate tectonics, you have mid-ocean ridges that spread apart, then you have material returning to the Earth. Essentially, these plates subside. It's the top of a convection cell. The faster you move it, the less time they have to cool. If they have less time to cool, they don't subside enough. People had worked out very quickly after plate tectonics was discovered that if you change the spreading rate of the oceans, you change the carrying capacity of the ocean basins themselves. And this was the hypothesis to explain sea level change on the surface of the Earth. It explained global sea-level changes.
It was called the Pittman hypothesis, though he wasn't the first one to invent it. But he did this, then I started working on the convection models, and I discovered, "Wait a minute, this doesn't conserve mass." Basically, I figured out a simple way to demonstrate this with simple computer models. There was a whole series of papers in Science and Nature at this time, but one of them was to address the fact that the classical model doesn't conserve mass. Then, if you made it conserve mass, you'd discover that individual continents go up and down. All of a sudden, by trying to solve a physics problem, mass conservation, I discovered that suddenly, the global sea-level changes this was used to explain also explained the individual continents. Through a series of papers, I then demonstrated that on a dynamic Earth, if you take all of what we know about plate tectonics and mantle convection and put it together, you get both processes. You get both signals that were observed and argued about. One, you get these nice global sea-level variations, then you also get these individual ups and downs.
From a mechanistic standpoint, and what I would call a generic standpoint, that is, in terms of just the basic physics of the process, I could explain both of these. That was one of the big things I really focused in on and it was known for at the time. The next step was, "Okay, I've shown that generically. Really, all geological problems get solved if you do things in the explicit time and space." That's how plate tectonics was discovered, by piecing everything together. Not generically, but it's taught in a generic kind of way. But the solution of it was that we could piece all these different observations and parts of geophysics together in a time and space fashion. And it's not the only thing. Many other major problems in the Earth sciences were solved by this. For example, the whole post-glacial rebound problem, another classical area that had been known from the 1930s and debated 100 years ago in the same epeirogeny and eustasy perspective. But in the 1960s, Canadian geologists had resolved it.
In fact, I think it had been resolved even before then, that both global sea level and places of the Earth, like Hudson's Bay, which was bowed down when you have the big ice caps, then when the ice caps went away, it went up locally, but it also went in synchrony with global sea level. That's an example of piecing together things in time and space. All kinds of observations were put together to do that. The next big thing to do was to try to piece this together in time and space, which would mean having a major push in our ability to look at this physics that I could do in a little box, and we sort of had the computational tools available. But now, all of a sudden, we had to do convection in a sphere, on the one hand, and we also needed a digital representation of plate tectonics. You've probably seen many examples in science documents and whatnot of the continents moving on the surface of the Earth.
In some sense, you would imagine any tool that does that would be the major tool you'd use in Earth science to organize things, the organizing principle. I attempted to do this very crudely before I left Michigan, yet I quickly discovered that two things were not possible. In a kind of realistic physics way, we couldn't yet do convection in a sphere. People were doing it, but it was all a simple fluid with constant properties, which doesn't give you plate tectonics at all. Doesn't even get close. On the one hand, we didn't have those computational tools to solve all the equations on a big computer, but I quickly discovered that one of the big problems in all the tools which existed for plate tectonics, they are left vast areas of the surface of the Earth out. As a function of time, all these plates are going back into the Earth's interior.
We generally can piece together that there were plates going down, yet if you had a plate tectonic reconstruction, to the first order, you move the continents around, and that was it. Then, there were sort of vacuums between all the plates. Or you had ocean plates, like North America breaking apart from Africa, and it created all this seafloor between the two. Or you fill it in there, but then the Pacific is left blank. We needed some sort of a way to represent the whole surface of the Earth, and I had started to recognize that existed, and I didn't know what to do. I tried to put together several big teams of individuals to solve this with different existing tools and modifying those existing tools. Yet, luckily, none of those got funded, then I eventually came to Caltech. I worked on several other problems when I was at Michigan, but the most important one was the ability to better represent computational models of plate tectonics in a convection model. And I think obviously, that's a key ingredient of the sea-level problem I mentioned. I think those were sort of the two big things I had worked on when I was there. Then, I came here to Caltech.
ZIERLER: When you talk about classical models of plate tectonics, are you talking about classical in the historical perspective of the development of plate tectonics in the 60s and 70s, or are you talking about classical, Newtonian physics?
GURNIS: I've probably intermixed a lot of uses of the world classical. All the physics we use are classical, Newtonian mechanics. All of the solid mechanics we use, primarily work done by engineers over the last 200 years, is all classical, Newtonian physics.
ZIERLER: There's no quantum in seismology?
GURNIS: Except in some of the mathematical methods long-period seismologists use. They've used a few things. Other than that, no. Then, of course, microphysics of minerals and things like that are quantum. On the mechanics side of things, it's all classical. Jennifer Jackson across the hallway is dealing with modern physics. Most other people in the building are dealing with classical physics.
ZIERLER: On the interpersonal side, you spoke so warmly of Michigan as a nice and supportive place. Tell me about your interpersonal experience there, what the department at Michigan was like.
GURNIS: Well, it was a very interactive, modern department. Highly respected classical field geology. There were several field geologists there. There was a small group of seismologists. In fact, the whole geophysics faculty had come from Caltech. There were two seismologists, Larry Ruff and Thorne Lay. Thorne actually was the one who recruited me to go to Michigan. Thorne worked on the deep interior, and Larry worked on earthquakes. Both of them and their work influenced me. Simultaneously with that, there was faculty there, especially Bruce Wilkinson, a field geologist very interested in sea-level change. He was mostly interested in geochemistry of the seas, sedimentary rocks, cycles, things like that. I talked with him a lot. Then, there was another gentleman named Henry Pollack. He was a heat flow person. He was already very senior, but with a post-doc, he was able to invert, for the temperature of the Earth's surface through the heat flux.
Because you measure the thermal profiles by drilling down. You have a nice profile of the geotherm. And he was able to invert for what the surface temperature was on the Earth. This thing played a huge role in the IPCC reports. He was part of one of the IPCC panels. They had this surface-temperature plot that mimics exactly what the measurements made in weather stations around the planet, then it zooms upwards. I talked a lot with Henry Pollack about heat flow and the nature of convection. It was a very personable place. A lot of the exchanges happened almost every night down at the bar. [Laugh] It was kind of an old-school Midwestern place where everyone left work at 5 and headed to the bar. And something that played a huge role in my subsequent career, and I didn't really realize this, over the last 15 years, as sort of a second career, I got heavily involved with the Ocean Drilling Program.
And Michigan had this mafia of ocean-drilling people, believe it or not, even though it was squarely set in the mid-continent. And they would talk about the Ocean Drilling Program, expeditions they were on, and in the back of my mind, I think that played a big role. Although, in reality, the biggest role the Ocean Drilling Program had was not so much my interpersonal relationships but reading these books. After every expedition, they would produce these books, during and right after the cruise. There was lots of stuff in them that never ended up being in the normal literature. I pieced together various stories, not about the sea level, but another one that was very much related to it called the initiation of subduction, which is something I worked on in the last several decades.
ZIERLER: What does initiation of subduction mean?
GURNIS: One of the big, baffling things about plate tectonics, which no one was able to figure out, is how a new plate was able to form. People thought they'd form at the margin of, let's say, the Atlantic Ocean. Just in terms of conservation of mass, imagine that it has to happen. Here, we have conservation of surface area on the surface of the Earth, then we have Pangaea, the super continent. And it's all one big continent. When it breaks apart, it only makes a mid-ocean ridge on the surface. Now, all of a sudden, all this seafloor forms, and we have a perfect record of it. But eventually, the continents are going to have to come back together again, so the plate's going to have to break somewhere. People assumed–and subsequently, it's been recognized that this does not occur–that in the Atlantic Ocean, the plate gets very heavy and old, then it suddenly goes bloop. Most geophysicists actually use this cartoon, even though there's no geological evidence to suggest that it's correct. A few of us have bashed our heads against the table trying to convince people there's no geological evidence for this. I don't know if other areas of science have this problem, but in geology, we often do, where a cartoon gets created for whatever reason, then people try to tailor the chemistry and physics around it.
ZIERLER: The way you're discussing plate tectonics in the early 90s, what was settled science that was resolved in the 60s and 70s, and what were the big, outstanding questions you were contributing to at that time?
GURNIS: At that time, it was the overall kinematics of the plates. From the mid-80s to even today, the first-order kinematics that we're very interested in haven't changed. It's been refined and [made] more accurate, and there are way better digital representations of it. But in some sense, that was all sort of worked out. The only major kinematic thing that hadn't been worked out was the initiation of subduction. We knew how new plate boundaries formed at rifting and stuff like that, but from the kinematic perspective, that was not worked out. And it's not completely settled, and that's something I've more recently contributed to, the last 15 years. Then, in the late 80s and early 90s, it had to do with the mechanics of the problem. That's what I do, work on the mechanics. What was not settled at all back then, partly settled now but not completely, was how to dynamically get plates that evolve as a function of time.
One of the things, which was not settled at that time, was when two plates come together, we have a subduction zone, sometimes what happens is that the plate goes under another continent, like South America, and it forms the Andes. But in the Western Pacific, we have a different kind of margin. It's one with what's called a back-arc basin. The plate actually goes underneath it, yet there's a little plate on top that actually sort of rolls back. Initially, after plate tectonics was discovered, people thought there was active convection going on in a subduction zone to push that apart, although some people said it was passive. I did the first modeling in which we could actually have the plate margins evolve as a function of time. We did this paper in which we were able to figure out how to put faults in models, then the faults could advect. When we were able to do that, we could actually see that the shape of the plates, was able to change. The ocean basins could grow in size, and the ones with subduction zones could shrink in size.
Then, we could have different characteristics on the overriding plate. This happened when I first arrived at Caltech in 1995, we wrote the first paper to show we could actually get these plate boundaries to roll back. But we didn't get the back-arc basin yet, that happened several years later when we were trying to figure out the initiation of subduction. We figured out that when a new subduction zone forms, it falls into the Earth's interior, and when it does that, it creates space. When it creates space, this other plate moves to take up, and it breaks on the top. No one knew how the mechanics of any of this worked. I used to explain to people that we were trying to explain the time dependence of the mechanics. What was worked out quickly, in my opinion, although not to consensus, was that the plates were basically driven by the slabs. Subsequently, we were able to produce some of the nicest models of this process. But nevertheless, it was sort of like, "Well, the slab pulls the plate, and that's how the plate moves."
ZIERLER: How did you model that process? What did that look like?
GURNIS: We were always able to do it in 2D. It's a combination of various things, but it's a combination of two different things. One is that you need to have the rheology. This is basically a description of how materials behave under different stress and strain-rate conditions. You could have elastic and fluid, but you can also have what are called non-Newtonian fluids. The physics of ketchup is totally different because it's a long-chain hydrocarbon, but it's a classical nonlinear fluid. Before they put it in squirt bottles, you have your all-glass Heinz ketchup, and you're trying to get that ketchup onto your burger. You hit it, and nothing comes out, then you hit it again, and it comes out. When the stress goes up, the viscosity of the fluid goes down. Then, it also starts moving, and there's strain weakening. All of a sudden, in this highly dynamic, high-strain, high-stress environment, the viscosity drops, and it all comes out. There are all kinds of nonlinear materials in our everyday lives. The Earth has this.
And the materials depend upon temperature. When you first take ketchup out of the fridge, it's not good for a barbecue. You want to keep it out there for a while so it warms up and comes out more easily. That's because ketchup is also temperature dependent in its viscosity. Ketchup is temperature and stress dependent. It's called a thixotropic material. Part of what makes plate tectonics plate tectonics is that the silicates, which make up the solid, actually have a temperature- and stress-dependent viscosity. In addition, they also have faults in them. I was able to combine faults with nonlinearity and temperature dependence, and you could start to see how mechanically a tectonic plate could work. And we could do this in 2D, and eventually lots of people were able to do this, but in 3D, it remained this huge challenge.
When I was at Caltech after some time, I was able to convince a bunch of computational scientists that this was still a relevant problem to work on, and we wrote this paper in Science in 2010, and we were able to resolve individual faults on a global scale as well as have the plates weaken, have strain weakening, have temperature-dependent viscosity, on a global scale. Not only could we explain the large-scale motion of the tectonic plates and subduction zones, but we could have little microplates suddenly emerge as well.
And we're still working on that problem but switched it around to going from a forward to an inverse problem. But that's still for the future, solving that problem. There were lots of things which got discovered from the early 1970s through the late 80s, yet we didn't know the mechanics. That was mostly what I focused in on, besides the sea-level problem, the mechanics of the time-dependent system of plate tectonics. That eventually led me into, when I got to Caltech, a lot of theoretical models of the initiation of subduction. I realized I needed to energize the community to make more observations because no one else seemed to be doing that. And that's what got me heavily involved with the Ocean Drilling Program.
ZIERLER: While you were at Michigan?
GURNIS: No. It's one of these things, you learn about certain things, compartmentalize it, but suddenly, you realize, "Oh my gosh, there's this other direction for this body of knowledge I've had." Other things get pieced together. It was kind of funny, at Michigan, I had done the bulk of my reading in the Ocean Drilling Program when I was a post-doc here, believe it or not. I'd pieced together some interesting things about how long subduction zones existed for. I didn't fully appreciate it. The prevailing view was that no one knew how a new subduction zone could form. All the mechanicians, a decade or so before I thought about this problem, said, "It's impossible to make one. It's really hard." And they were all looking for it in the Atlantic Ocean. But I was reading all these drilling reports from the Pacific Ocean, and I discovered, "They're forming all the time all over the place. No one's paying any attention to this."
People would say subduction initiation was a really fundamental unsolved problem. "It's a really hard thing to do. How can you make one?" And I would say, "No, it's not. It's really easy." And I think they thought I was crazy. But it was because I was reading all these papers, and I had a different perspective. But I didn't piece it all together. Then, when I was at Michigan, Larry Ruff would ping me all the time and go, "What about the initiation of subduction?" And we were going to start working on that before I left, but we never did. Although, I went back and studied extensively the direction he pointed me in.
From Michigan to Caltech
ZIERLER: The seed was definitely planted in Michigan, but you didn't pursue this until you got to Caltech.
GURNIS: I did a little bit of work, but I knew that in order to understand how to make a new subduction zone, you needed a computational method. You could have convection and plates, but you actually had to have plasticity. You had to make a new fault out of nothing. That was what I thought. There was no tool that existed there. Then, I discovered that another group of individuals was making new faults out of nothing, but they were doing it for what's called a rifted margin. I hired a post-doc who was working on that problem to come to Caltech. Caltech was very fortunate, through its relationship with Gordon Moore. He gave this gift to Caltech, $600 million. $300 million stayed in the Foundation, and we had to compete for it, then $300 went to Caltech. Myself, Mark Simons, Kerry Sieh, and Brian Wernicke on the faculty created this thing that eventually we called the Tectonics Observatory.
They gave us a whole bunch of money, so I hired this post-doc [Luc Lavier]. He brought this code with him, and instead of looking at plates that got pulled apart, we also looked at plates that pushed together. Then, we came up with a new model for how a subduction zone could actually form. At the time, I was really thrilled. I thought this explained everything under the sun. But it didn't pan out. But what came out of that was a need to get more observations within the ocean basins, but historical observations. Here I am, a theoretical geophysicist, and I wanted geologists to tell me more about stratigraphy from an observational perspective. And no one was going to do it because, interestingly enough, this whole question of making a new subduction zone around the planet was dominated by individuals who looked at volcanic rocks. They thought that when a new subduction zone formed, it was this huge outpouring of a specific kind of volcanic rock.
I went along with the story for a while, but eventually, there were more observations. It's not just volcanic rocks, it's the stratigraphy, too. I started to read more papers from the old ocean drilling reports, and eventually got deeply involved. Over a period of four years, I went on a series of three different ocean drilling expeditions, two of which were meant specifically to test hypotheses for the initiation of subduction. The other one had to do with this dynamic topography question. From an administrative perspective, I remain engaged in that program, and I want it to succeed. I think it's really important for the Earth sciences. But I don't really see that being a huge component of my own personal research anymore.
ZIERLER: Just to get a sense, at the interpersonal level, you were happy at Michigan? You could've stayed there long term?
GURNIS: Oh, yeah, I was really happy. I really liked the people there, I was interacting with them. They were really disappointed. But what drew me back was the Seismo Lab. I had been here, and it was a different kind of environment. It was kind of interesting, I knew I would take a different direction. I knew that I probably would focus more on geophysics and less on geology. Initially, that was absolutely what it was like. When I came back to Caltech, it was all back to just geophysics. I also returned, through my collaborations with Don Helmberger, to looking at the Earth's deep interior, which I had left when I was at Michigan.
ZIERLER: Were you at Michigan long enough to get tenure?
GURNIS: Yes. I got tenure very quickly, within five years. Initially, they wanted to give it to me very, very early. I was never worried about tenure. But it was kind of surreal office politics.
ZIERLER: Were you getting interest from other departments while this was happening?
GURNIS: Yeah. Chicago wanted me to go there. Other schools would call up. I turned Chicago down because I suspected Caltech was going to hire me, then they did. Mostly, I would say, "I'm not interested." I was so happy there, very few schools could've pulled me away. It was the Seismo Lab that drew me back.
ZIERLER: Who was driving the recruitment from Caltech, as far as you know?
GURNIS: The recruitment was being driven primarily by Tom Ahrens. He was a very, very prominent mineral physics guy. I knew Tom.
ZIERLER: Had you worked with him before?
GURNIS: We never worked together, but in terms of academic family trees, my advisor was Geoff Davies, and he got his PhD in the Seismo Lab. First, he did a lot of work with Don Anderson. For some reason, at the time, I more thought of Davies as an Ahrens student, but if look at his thesis, it's a typical Seismo Lab kind of thing.
ZIERLER: What speaks to that?
GURNIS: A mixture of faculty, then going out on his own and mixing things in a somewhat different way. There's this very easy way of having multiple advisors. It was and remains extremely common. I knew Tom from when I was a post-doc and would interact with him a lot at meetings. But it turned out they tried to recruit Thorne Lay as well as myself. They tried to hire two people at the same time. I thought this was great. Thorne obviously didn't. I think he viewed that maybe he would still be under the shadow of these two greats, Hiroo Kanamori and Don Helmberger, and that maybe he wouldn't be viewed in the same way. I don't know why, I thought he would've been a terrific choice. I really wanted him to come and thought he would make a great director. But he decided to stay at Santa Cruz. And it's a very good school in our field. The students may not be as good, but the faculty is quite good, and it's a good environment. But it was Tom leading the effort on the recruitment side of things.
But the Seismo Lab was known to me, so I felt very comfortable. Not only at the time, but also what I started to learn even after I was here, people on the outside tended to view Caltech in a negative way. "Oh, it's very aggressive and competitive." I knew that was wrong because I was here. I said, "It's wonderful. Everyone was very supportive and nice to me. Everyone was focused and hard-working, but also very supportive." It didn't seem like there was much politics at all. But I subsequently could understand this because especially of the style of seminars. The seminars were so interactive. Not only were they interactive in the division, but they were especially interactive in the Seismo Lab, both when I was a post-doc and when I first came here. We would just ask questions continuously. It would be this dialog. I learned so much from this dialog. I'd learned as much almost from the questioning as from the answers. And a lot of people didn't like it. They felt it was hostile because they couldn't give the narrative as they wanted to, whatever story they wanted to tell.
They wanted to give their narrative unbroken like they were some master. And often, people would be able to go through much of their talk without any questions. Then, all of a sudden, especially in the Seismo Lab, you knew when things were going to crash and burn. Sometimes the questioning would go on forever, like an hour after the seminar. They would keep going on. Some of the questions, you knew, this speaker is in deep. Helmberger would ask, "Can you show me a seismogram?" People would do so many levels of processing, and there was this methodology that initially seemed to give only ambiguous results, seismic tomography. And they would never show a seismogram.
And if they did, after the fact, how well would the seismic data actually fit their model? Helmberger would say, "Can you show me a seismogram?" Often, the speaker would be up there shuffling away, and there would be no seismogram. I'd be like, "Oh my God, where's the data?" I talked about this before, the interest in the fundamentals of physics and chemistry at Caltech. Here, of course, the physics of seismic wave propagation and things like that. Yet, everything in terms of inference about the Earth is always rooted in real data you can see with your naked eye. That was one kiss of death, when he would ask to see a seismogram. Then, the second question you knew was coming during seminars was from Kanamori, who was very quiet during seminars. He would ask, "Can you explain that again?"
ZIERLER: And it's not like Hiroo didn't get it the first time.
GURNIS: Oh, he absolutely got it. Maybe from a polite Japanese way, he was giving them the benefit of the doubt, "Can you explain to all of us in the room why you're piecing the data together that you just told us, which none of us seem to get?" And he would never be hostile. The rest of us would be poking here or there. The seminars were so exciting. People sometimes view these things as being aggressive and things like that. I wonder if this old way of doing seminars is a thing of the past, at least for our lifetimes. Hopefully, scientists will come back to it because I don't think it's aggressive, it's to get to the truth because people are interested. I remember there was one guy in my field, and we were having a little party at my house for him with post-docs and other people after a seminar. He felt uncomfortable by his talk. We asked lots and lots of questions. Then, later, it came back to me through intermediaries that everyone was hostile and bad to this guy. It was like, "Holy smokes, are you kidding me? So we asked him a couple of tough questions because we're interested in the truth. That doesn't mean people didn't like his talk or visit or anything like that."
I don't know if you hear this from other folks, but I think these older seminars were great. There was another very famous person in the division, Gerry Wasserburg. I wanted to give a seminar, and they let me do it. And Wasserburg had just become chairman the week before. All of a sudden, the seminars were shifted by 30 minutes. I gave my seminar, it was going really well. I was introducing things, people were asking questions. Then, Wasserburg came in halfway through, hadn't heard any of the background material, any of the equations, the justification, or anything, and he immediately started to send artillery strikes at me. I fended them off, he was full of shit, but it was all about diffusion. He knew diffusion backwards and forwards. I knew it, too, but I also knew about advection, and he didn't. It was just funny, this super aggressiveness. But it didn't bother me.
ZIERLER: Just by virtue of coming back to the Seismo Lab, how did that influence the research? Did you change up what you were doing at that point?
GURNIS: One thing happened quite naturally. I then teamed up with this post-doc who was already here, Louis Moresi, who became very prominent in the field. He's now a professor at the Australian National University, and he had been developing a new method in finite element analysis, which was something I knew and had done as a post-doc. In fact, one of my claims to fame as a post-doc was to write the first paper in geophysics using a parallel computer. Yet, nothing scaled. You couldn't do super big problems. It was still pie-in-the-sky. Louis made a very important breakthrough as a post-doc, and with these [we] started to work together on a program that could scale on big parallel computers that could solve the physics of convection in a sphere. Another post-doc and former graduate student of mine, Shijie Zhong, now a prominent professor at the University of Colorado, developed this big spherical convection code, which was the first open-source software in the field on that particular topic. We could then do the physics of convection in a sphere, and we used this code to do different things. Then, through an external collaboration, I worked on the plate tectonic data model, and that was another big breakthrough, but it was the international collaboration.
ZIERLER: How did that get started? Were you there from the beginning, or you joined something ongoing?
GURNIS: I joined from day one, and it was two of us, myself and Dietmar Müller, a close colleague who spent his entire career at Sydney University in Australia. When I first left Michigan for Caltech, one of the big things to do was to try to explain the vertical motions on the surface of the Earth, not from a purely theoretical perspective, but to get it down in time and space. There were two ingredients there, which were the spherical convection model, which we'd started to work on in the Seismo Lab, and the other part was a data model representing the movement of the continents on the surface of the Earth. And I took an existing one and realized that in order to connect it to a convection model, it meant that you had to have the whole surface of the Earth where the digital representation didn't exist. Then, I also discovered that the time dependence and the motion of the plates was all inconsistent. It was never thought it needed to be internally consistent. I was talking about these kinds of things with a guy I had just met in 1994, Dietmar Müller. I wanted to go there so I could talk to this old geologist.
And I'd just won this thing called the Packard Fellowship, so I had all this money. I was writing letters to this old geologist in Australia who told me he was going to retire. I said, "Oh my God, I've got to pick this guy's brain." I got on a plane to Australia to go talk to this old geologist who had put together this very interesting story about how Australia moved. In the course of that, Müller had just gotten his PhD and arrived. He'd worked on the kinematics of plate tectonics. Through atlases, we could move pieces of paper around, and I could see how convection and the stratigraphy on continents would've been worked together. But there was no way to do this with a computer program. There was no software available. All the software which has existed to move the continents on the surface of a sphere over the previous 20 years had been taken over by small consulting companies who worked for the oil companies. They were all working at the behest of the oil companies with proprietary software, where they were assembling all this public data, then only making it available to the oil companies, or you had to pay.
Then, all the ways data would be represented was totally not modern. Dietmar and I said, "We have to start from ground zero." Two years before, I had tried to get one of these groups at the University of Texas doing this PLATES Project to open up. We assembled this group with support from the National Science Foundation, and in the end, we all walked away from Austin knowing that these people were never going to open things up. They were all working at the behest of the oil industry. Eventually, Dietmar moved to Sydney on a lark to be a lecturer. He had just arrived, and we started to look at maps and how this all could work together. Then, using the old infrastructure, which we basically pieced together in a truly ad hoc way. We connected this together in the finite element program, which was in a cartesian domain, not a spherical one yet, the one that Louis and I were working on.
It was called CITCOM, California Institute of Technology Convection of the Earth's Mantle. This then became the de facto standard in geophysics and eventually became a spherical code. But we were able to take a cartesian one, and with Dietmar, we put things together with the old codes, but in an ad hoc way. And we wrote this article in which we combined plate tectonics, mantle convection, stratigraphy, and geochemistry in this very quantitative space-time predicted way, but only for a region of the Earth. There were these two mysteries, one in geochemistry and marine geology, and it was really mysterious. They discovered that in the middle of ocean, there was this big convective downwelling. And that was globally unique. No one argued about it, it was absolutely the case. But no one knew why it was there. Then, on the continents, in stratigraphy, the old guy in Australia, John Veevers, who I wanted to go meet, had pieced together that Australia's stratigraphy had subsided way down and popped back up.
And we figured out through paper and maps that they were all connected. But instead of actually writing a paper in the old-fashioned way of geology, empiricism, we decided we were going to put it together by combining plate tectonics and mantle convection all together in one. I remember the editor for science magazine wanted to know if I had anything I could give her. I said, "I'm working on the most important thing I've ever worked on, but it's going to take nine months." She thought it must've been a baby. [Laugh] I don't know why I came up with that number. But I had literally pieced everything together on how to do it all. We had a nice article in Science where we pieced everything together.
I actually think it's the best paper I've ever written. It's definitely not the most highly cited paper. But we could bring convection, plate tectonics, stratigraphy, how geochemistry moved around in the mantle. Everything came together, but we still didn't have all the infrastructure we needed to do this professionally on a global scale. And that led to this big program called GPlates, which is now the de facto standard in plate tectonic modeling. Everyone around the world uses it. Once the cat was out of the bag, suddenly everyone could use this for all kinds of cool things. And they did, and we did.
ZIERLER: Last question for today, just an institutional question. When you got to the Seismo Lab, was the USGS, the proximity and historical connections, relevant to you at all?
GURNIS: Not for me. I knew it existed, I knew all the folks, I went to their talks. I found it very fascinating, and I could see all kinds of things emerging. I got to Pasadena, and within the first couple weeks was the most important earthquake I faced in my whole career here, which was Northridge, January 17, 1994.
ZIERLER: What was it like for you that day?
GURNIS: Oh my God. Like for everyone, it was shocking. It was scary. The sun wasn't up yet, it was totally dark out. And I lived in a new apartment several blocks from here to the east. It was unbelievable. It just shook the building like hell. It didn't damage anything. It was a new building. But boy, oh boy, I got to the Seismo Lab very quickly. Since I had nothing to do with earthquakes at the time, it was the most extraordinary flurry of activity I had ever experienced in my life. Subsequently, major discoveries were made about the earthquake, major advances in observational seismology were made, public policy, university-government relations. It was just extraordinary.
ZIERLER: What did you witness in terms of any partnership between the Survey and the Seismo Lab on that day and in the aftermath?
GURNIS: It was extraordinary, the level at which everyone worked together in this harmonious way. It wasn't Caltech, it wasn't the government. There was no hostility. Everyone was sharing information. Tom Heaton wasn't yet back at Caltech, but he was there all the time. And there were several other individuals, Ken Hudnut, Lucy Jones, who we saw every day, and they were USGS people. Then, there were Caltech people. Hiroo and all the students were looking at the seismogram from this brand-new network they had just put in, which wasn't being used except for basic research. It was called TERRAscope. It was amazing to see science in support of this extraordinarily important and devastating thing that had just happened.
ZIERLER: On that note, we'll pick up next time as your career develops in the late 1990s, going from there.
[End of Recording]
ZIERLER: This is David Zierler, Director of the Caltech Heritage Project. It's Friday, September 9, 2022. It's great to be back with Professor Michael Gurnis. Mike, once again, it's great to be with you. Thanks so much.
GURNIS: You're very welcome, David.
Building a New Era in Computational Geophysics
ZIERLER: What I want to do today is pick up the story in the late 1990s. I've already heard from Jeroen Tromp in his discussions with people like Steve Koonin about the need to really increase the computational capabilities at Caltech. In the four or five years prior to that in the late 1990s, from your perspective, where was the Seismo Lab computationally? Where was it doing things, where were your interests in terms of those computational needs that were already met, and where did you see serious need for growth?
GURNIS: The late 1990s was a period in which, I would say, the computational needs of most of the faculty except me were met with fairly large servers we had in the Seismo Lab itself. We were already fairly far into these Unix workstations, more than a decade, I suppose. Most of the work was being done on those workstations themselves. But for me, they just weren't enough. From the mid to late 1990s, we had actually moved into a time when we had our codes, the stuff I did in geodynamics using the finite element method, we had things that scaled on parallel computers. Just before I arrived, Caltech had what was called the Intel Delta on campus. After parallel computing was invented at Caltech in 1984 or '85, people could do quite a bit. And because of Caltech's lead in this particular area, Intel donated the Intel Delta, which I actually never used. That was the early 90s. And that was the fastest computer in the world, I think, at least at a university campus, and Caltech had it. Then, there was the Intel Paragon. These used the Hypercube architecture. Intel Corporation wanted to get involved with this because it was their chips running it. They figured a big computer needed a lot of Intel chips. And they built several of them.
They were basically very large versions of the computers built at Caltech or in several companies, which eventually folded. I was able to use those machines. Then, somehow, in that period of time, I think they were supported by NSF, but I forget the actual details. We had some very large HP supercomputers on campus. And interestingly enough, these were national facilities. Caltech, for a period of time, competed in this arena of supplying or hosting what was called Big Iron. I was already used to using these sorts of facilities, first when I was a post-doc, when the San Diego Supercomputer Center was one of these facilities, then when I was at Michigan, I continued to use these facilities. I continued to do this at Caltech. But we basically had sort of tapped out. I actually even used some of these workstations the other faculty were using. There was one paper in particular in the mid-90s I wrote with Shijie Zhong. These actually didn't scale on big parallel computers.
This was when we first introduced faults into the codes. I remember there was an article in Science magazine, I think in 1995. There were, like, five runs in this paper, and each one took five weeks to compute. We had one big really nice workstation with, at the time, a lot of memory. We actually, for that one paper, just ran solid for nine months, I believe. This other code then became available, CITCOM, which was originally written by Louis Moresi. Before MPI became available, there was a distributed way of distributing this on a set of workstations. It wasn't very efficient, it was overwhelmed by communication costs. Then, Shijie took the code, and he introduced this thing called MPI, which suddenly became the de facto standard in parallel computing, and largely is to this day. It was basically a rewrite at Argonne of the Cubix, which was the original message-passing language written at Caltech for parallel computers.
We were able to start using this software, but we were also able to use one of these in-house machines with a different piece of software that used shared memory. It was a different model for parallel computing. We were all set with CITCOM; we basically needed a bigger computer. The number of parallel computers that were out there wasn't enough. They brought in this guy, Paul Messina, from Argonne National Laboratory to run CACR. And one of the people they brought in as a staff scientist was Tom Sterling. And he basically came up with this concept that you could take all of these workstations, put them together with an ethernet, use message-passing, and build your own homegrown supercomputer. They called it Beowulf. Jeroen and I wanted to do this. I had already gone through all of my startup money, but Jeroen and I pooled our resources in the Seismo Lab with some other faculty members for an equipment grant.
Then, the computing paradigm had changed so much between when we wrote the proposal and when we got the money that we decided we were going to build our own Beowulf. I forget how many processors we actually had, but Jeroen and I set up an assembly line in the computer room of South Mudd. Interestingly enough, before I arrived, from the 1970s on, on the second floor of South Mudd, we had a giant machine room. We still sort of had it, but people kept carving it back. As workstations and supercomputers got smaller and more powerful, the room got smaller. They took space away from it. But it had a raised computer floor, you could put wires under it, lots of air conditioning. Because the Seismic Network operations and staff kept growing, competing with TriNet, TERRAscope, all that stuff, it got squeezed. Then, Jeroen and I wanted to build a big computer again because the paradigm had changed.
We basically bought all the parts, and we bought really good parts. We made sure we spent more money on the fans. And these basically were going to be desk-side computers. We built this thing and had it operating there in about two weeks, and our graduate students and post-docs would serve time on the assembly line. We built maybe 200 workstations. Probably more. Then, we went to Home Depot or something like that and bought these warehouse shelving units and assembled it. I even got a pinched nerve because I was working in there, assembling all this stuff. There were two rows, and on the two outer faces, on each side, there'd be, like, 100 or so, which meant 200 processors because we had dual processors in each box. On the back side, the covered part, we needed to have all the wires, so we bought all these routers on the inside, and all the ethernets were connected and stuff like that. There was originally a window there, too. We covered that up for air conditioning systematics.
And this was great. On one side, we'd run CITCOM, and on the other side, we'd run SPECFEM. And we'd switch depending on what the students really needed. We got some really big calculations done with SPECFEM and CITCOM. Two things emerged from this collaboration. One, the Seismo Lab needed its own big supercomputer. We had gutted our room because we needed more room. The other thing was, we were running this high-performance computing with a lot of people. We needed to develop software in a more modern way. Let me talk to you about that first.
ZIERLER: If we can go back just for a second, in the 1980s, why was parallel computing relevant to you? How was it good for what you were after?
GURNIS: Initially, it wasn't. When we wrote the first geophysics paper in parallel computing, we took a domain, and we broke that domain up. It's just a natural way to imagine how to solve a spatially continuous domain which you have naturally put into subdomains with something like a mesh, elements, something like that. Even when you think about what happened, if you go back to when John von Neumann built the first von Neumann machine at Princeton, they had this finite difference methodology, where you broke your domain up into a bunch of subdomains. If I wanted to make things faster, I wanted to break my domain up into smaller domains and compute all of those simultaneously. This is interesting because different equations, the way that they're formulated in a computational way, are solved in different ways. Some things are inherently naturally parallel. There's no connection between anything spatially. You can take 1,000 processors, subdomain it up, and make it 1,000 times faster if you use 1,000 little computers that all work simultaneously.
Then, you combine your results at the end. I'm going to use seismic wave propagation and contrast it with mantle convection because the equations are quite different. In seismic wave propagation, all the interactions are local. Even the way the equations are solved, all the interactions are local. You have a wave here, and it suddenly interacts with the surrounding domain and how the wave affects the displacement in pressure around you. That's really quite different from the problem I solve. It's called Stokes flow, in which the whole problem is always globally interconnected. If you move it over here, it instantaneously has an effect over there. Some things could naturally move over to a parallel computer, like seismic wave propagation, whereas this Stokes flow remained quite difficult. We created a global matrix that represents the solution of the whole problem. You have to invert the matrix at every moment in physical time.
The big breakthrough in that happened in the Seismo Lab with Louis Moresi, who was my post-doc, when he invented what's called an iterative method. You take this big matrix, and in some sense, different parts of the matrix are put onto different computers, and they're solved simultaneously. The thing about this and parallel computing is that it's always a balance between how much computing you do and how much communication you do, both local and global. But Louis seemed to have cracked the nut in the early 1990s. Even though we were still sort of setting up the global problem, we'd broken up our mesh into subdomains, but we were doing a lot of computing locally and a lot of communications. That's why this term, HPCC, high-performance computing and communications, is important, because you have to communicate, and you have to compute. Some things worked well, some things didn't. Mantle convection is one of the most difficult problems to solve, but we cracked it. It took a lot of work. Because we could crack that nut, we wanted a big computer so we could actually do it.
ZIERLER: What would be an example of a research problem where you know you need the big computer to get the answers you're looking for?
GURNIS: Both seismic wave propagation and mantle convection are great examples of that. They're classical problems in science and geophysics that both require a gigantic computer. We just knew it was a big problem from day one. We knew even the day I got involved with this, "This is a toy problem. We've eliminated all the interesting physics in this particular problem so we could squeeze it onto this computer." Eventually, we reached a point where we would do bigger and bigger problems on a von Neumann machine. Most computers, even the ones we're using, are still von Neumann machines. The big breakthrough happened, of course, in 1983 at Caltech when they invented the concurrent computer, the Hypercube. There are different models of how that's done these days.
ZIERLER: What was the computing paradigm shift you referred to in the 90s?
GURNIS: We knew that we could now compute it. We did the math and computer code, but we didn't have the hardware. And because an individual PC was so cheap–that was the whole thing, it was the Beowulf paradigm that allowed us to jump forward on this. It was only a small window of time where the individuals knew they could race ahead. Computers had become very cheap because they became a commodity. Before this, big supercomputers were extremely expensive. It was dominated by several companies initially, but by the mid-90s, one company was left standing, Cray Computer. And each of these computers was so expensive, and there were so few of them, they were mostly used by the weapons laboratories at Los Alamos and Lawrence Livermore, the oil companies to process seismic data, and weather prediction. Those were the three big things.
Then, we had the PC, which changed the world. But then, this idea of the Beowulf came up. That was invented at Caltech. That meant that because computers were individually so cheap, you could go to the computer store and buy this stuff, then put them all together with an ethernet. Suddenly, you could make a computer for $100,000 that was just as powerful as a Cray Supercomputer was for $5 million. If you had a bunch of cheap labor, e.g. graduate students, and you had a soldering iron and a couple of wrenches, you could build some of these things, so Jeroen and I built it. That's the paradigm shift that happened. Then, they said, "Oh my gosh, we want to build a bigger computer." Steve Koonin was still at Caltech. He was a very good provost, in my opinion. He only stayed five years in that job, but the reason he was good in this position was that he understood and recognized this.
He knew the power of computational science. He was deeply involved with the weapons laboratories, so he knew how important these big supercomputers were, and he knew what we did at Caltech. We needed to build a bigger computer. He was in mechanics and other areas of physics, and he sort of got the general idea of what we did in geophysics. He bought into this idea. The division chairman at the time, Ed Stolper, convinced the provost or whoever to renovate the sub-basement of South Mudd. That floor was in the control of the Seismo Lab. The whole floor–it was giant real estate down there–was in the control of the Seismo Lab. But it turned out a vast area was occupied by microfilm. It was one of three or four laboratories in the country in which we stored all these seismic records from WWSSN, the World-Wide Standardized Seismic Network, which was put in place by I guess the US Air Force to monitor nuclear explosions from the Soviets.
That's what paid for the Seismo Lab before I came around. We agreed to give up this space. Later, outside of Caltech, we got a bad reputation. They were foaming at the mouth. I'm sure you've interviewed people who want to put my neck in a noose. But it wasn't my fault, it was Helmberger and Kanamori, who are observational seismologists. But we had this idea because we wanted to move forward. Jeroen and I said, "We built one with 200 processors. This time, we'll build one with 1,000 processors." What Caltech needed to do was to throw the resources in to build a big computer room again. We were asking them to build what a university would usually build, a huge computer center. But we wanted one in the Seismo Lab. We built the infrastructure, the air conditioning, a massive amount of electrical power, huge room. Clean, beautiful floors, moving air. But it was empty. Private enterprise was not going to let this Beowulf paradigm get in the way of making a lot of money.
Dell Computer, Compaq, even Intel tried this out with the Paragon, IBM, they all were going to get involved with this. They were going to take this and build these computers. Are we going to build it ourselves? Are we going to find a startup company to build it? Some people were making the transition that they weren't going to build it but buy it from a startup. There were startups around the country building these compute clusters. This was before the big data farms and Google took the universe over, but it's very similar to all of that. I remember we got involved with Dell. I have no idea how Dell Computer initially got involved, but there was this guy named George who worked for Dell, and we brought him to Caltech. Jeroen and I brought him down to the computer room, and he saw this thing. He knew we were at the bleeding edge of software, then there was this gigantic empty computer room. One of the big problems Dell was having was, it was trying to sell these computers with giant footprints. They were, like, 50 feet long. They'd take up half the size of a house.
No one had any space for these things. Universities had all these big computer centers around the country, but no one had any room. He came here, and he said, "Holy smokes, we can put a Dell Computer in here." [Laugh] I have no idea what happened, but Caltech bought a gigantic Dell Computer of the same size that the national supercomputer centers were using. And the Seismo Lab had its own. It was, like, a 1,000 processor Dell supercomputer, state of the art. It ended up on the list of one of the fastest computers in the country for a couple months. They put it in there, and we just went to town on this thing. We were running CITCOM, Jeroen was running SPECFEM. It was incredible. But of course, the rest of the country caught on, and within a year, we needed to upgrade the system.
And we eventually got an upgrade. But they wanted millions and millions of dollars. And we did get another NSF proposal funded, but when we went back to the trough, we would just get slammed. We got so annihilated a couple years later. The jealousy was just dripping from the reviews. "Why does Caltech get the thing the whole country needs to use for themselves? Bad proposal." Even though we had one great science question after another and were the only ones in the country with the software. Everyone knew this was the future, so why should one department have it all? That was the end of that. [Laugh]
ZIERLER: Had you worked with Jeroen before he got to Caltech?
GURNIS: Yes. I'm a couple years older than he is, but I met Jeroen because he and I were Packard Fellows at the same time. I actually met Jeroen even before I came to Caltech. I went to Harvard to give a symposium, and he and I met. We talked there. Initially, we didn't hit it off because I was already totally infatuated by high-performance computing, but Jeroen's claim to fame before he got to Caltech was refining normal mode theory to go directly from seismic data to large-scale Earth structure. He was a long-period seismologist, sort of like Hiroo, and a master mathematician in the use of mathematical methods. He was not a computational guy. It took a couple of years to recruit him to Caltech.
ZIERLER: Were you involved in that recruitment?
GURNIS: Yeah, eventually. Initially, it didn't really work. We wrote our report, and the faculty approved it, we made him an offer, and it was a little bit difficult. Then, Ed basically sat on it for a while. He was conniving. Ed was really good at convincing people to come to Caltech. It was very unusual within the division. The committee did its work like we normally did, then the division voted on it and eventually turned us down. It just sort of sat in limbo, but at some point, Ed used his own discretion and figured out he could hire him, and he did. [Laugh] And that was great. And immediately, we started to hit it off. One thing Jeroen and I did was the hardware stuff, and we saw the connection. Because we had invented this new software at Caltech, I submitted a regular geophysics proposal to NSF, and it was a use of the big finite element codes. Because of that, we could actually do very fine-scale things at the same time we could do coarse things. It went to NSF, and presumably it did very well in geophysics.
But the program manager called me up on the telephone and said, "Something very interesting happened." Her name was Robin Reichlin. She was really important for both Jeroen and myself, to other people in the Seismo Lab, and to geophysics overall. Apparently, when Al Gore was vice president, he came up with this KDI program, Knowledge and Distributed Intelligence. It was just an NSF program, but it was totally run by the computer scientists. Apparently, a program manager went to Robin and said, "Let's look at your proposals. Let's see if there are any in there that computer science can fund," which was very unusual. They went through her proposals, and he went, "Wow, look at this one." They picked my proposal out of the pile. She said, "Mike, we're going to fund your proposal right away, and I'm not even going to pay for it in geophysics." That was really cool, but two years later, NSF decided to create another program. They were going to call it ITR, Information Technology Research.
And these needed to be interdisciplinary proposals. I was already involved with CACR. I think myself and Jeroen by ourselves put something in, and we got trashed. Then, we went over, and I talked to Paul Messina, the director of CACR. I said, "We want to play in this game. Can you help us out?" There was another guy named Michael Aivazis, a former physicist who was brought in to CACR to help with computing. He was doing stuff in Python, and then there he was doing something called Frameworks. The other thing Michael was big on was software engineering. We teamed up with Michael and Jim Poole, deputy director at CACR, and we wrote a totally different proposal. We were going to do two things. We were going to bring software engineering into geophysics. We were going to train them to use repositories and best practices. No one ever talked about this in geophysics at the time.
Two, we were going to then put our codes, SPECFEM, written in Fortran, and CITCOM, written in C, within Frameworks underneath this object-oriented system within Python so they could share information with one another. And we would do this individually within SPECFEM and CITCOM. Jeroen and I did this. We got a huge amount of money at the time. We got a post-doc, paid Michael's salary, graduate students. Our software just skyrocketed. No one in geophysics was using Python, and no one was doing Frameworks or anything. We just ran ahead for several years and did this. We learned so much. In the early aughts, Python took science over and really exploded. Another thing was happening simultaneously within geophysics. The community writ large, mostly in my field of geodynamics, but sort of geophysics, with the encouragement of the NSF, wanted to create a national center for geodynamics in the US.
They thought it'd reached the point it needed to move forward. Everyone liked the idea to move up to the next level of bigger science, maybe more funding, and this idea had floated around. Starting in '86, we spent part of our summers at Los Alamos, and this was the way we were going to solve the access to computers issue. Simultaneously within that, NSF generated the supercomputer centers. Nevertheless, it was such a cool program that people from around the country would gather at Los Alamos, nominally for two weeks, but a lot of students would come for the whole summer. At the time, within computing, Los Alamos still had this auger sense. They just took off with computing. Of course, it was all behind a fence.
I would go there in the 80s and early 90s, and it developed an extraordinary sense of community and sharing. And geodynamics and mantle convection were way ahead of the whole community in benchmarking, validating our codes, doing inter-code comparisons, things like that. That started in the 80s. I bring this up because of the sense of community. A whole group of people around the country in my field, about half a dozen of them, professors at top universities including myself, Brad Hager at MIT, Rick O'Connell at Harvard, Peter Olson at Johns Hopkins were all behind this idea to build a center in geodynamics. But no one wanted it because we were still arguing about the equations, how to formulate a problem, and all these breakthroughs were being made, in some sense, at the graduate student, faculty, level.
We didn't want anyone to guide our research. I came out really strongly against this, especially in the 1980s. "We don't need a center for geodynamics. This is going to undermine creativity." Nevertheless, we knew where supercomputing was going. We knew everything was getting better, and we had to, too, even though we had to do this competitive way of doing highly creative science. They all wanted this science center, but no one wanted to give up their science. We were going to have a one-day powwow at Johns Hopkins. All these people came in, and we had this meeting about how we were going to do it, who would be the PI, and stuff like that. This was Jeroen's idea, and it actually created some bad blood within the community that I spent the next six months working to fix. Jeroen was not in geodynamics, but Jeroen and I ourselves, but the community overall, had made this big leap forward. What we needed was not a center for science, we needed a center for software engineering.
ZIERLER: To keep up with the hardware because the hardware was already ahead?
GURNIS: To keep up with everything. To move the software forward, so we could have bigger, more robust software and take advantage of all the hardware changes, do more benchmarking and validation, things like that. We knew the future was not one of a science center, but one in which it would be an infrastructure center for software. But no one was talking about this. Jeroen, myself, and Michael Aivazis showed up together at this meeting. Everyone had already put a proposal in. We came to the table and said, "What the community needs is software engineering." Then, Jeroen and I brought Michael Aivazis, who was incredibly articulate on this topic…
ZIERLER: Where was Aivazis at this point?
GURNIS: Caltech at the Center for Advanced Computer Research. He could articulate that what we really needed to make this big breakthrough was engineering. And guess who had already gotten this giant NSF grant and had all this experience in-house in the Seismo Lab? Us. Instantly, everyone knew this was the solution for the problem. The reason we had the powwow is because everyone was an equal player, and everyone wanted it, but no one wanted to give up anything. But no one had any experience in software, then we showed up with a person who could articulate this and a whole team of individuals who knew exactly what they were doing. Then, we voted around the table, and everyone voted. Caltech only got one vote. And of course, it was unanimous. They felt we were disingenuous. "I could've brought someone from my university, too." They weren't working with these people. Then, it was decided, "Gurnis, you're in charge now. You have the next year to orchestrate the whole community, make a center, then give it to the NSF."
ZIERLER: What did you think of the prospects before you got started?
GURNIS: I didn't necessarily really want to do it. I was confident. Jeroen was totally confident. He said, "You're going to go there and blow them out of the water, and you'll be in charge." I didn't want to be in charge. These were all my friends. NSF loved it. And I went on a peace mission to Cambridge a couple months later. But it would be a center at Caltech, NSF would support it, and it would be done with staff members, but it would have all these committees the community would have complete oversight on. Even though I would be director, I'd have these committees, an executive committee, a steering committee. This was worked out in the proposal process. We put the proposal together and sent it to the NSF. They went out for 12 reviews and got 12 "excellent" reviews back.
ZIERLER: What were the key points in the proposal?
GURNIS: Software engineering, Frameworks, community-building, benchmarking. It was all the things Jeroen and I had all this experience in, except for community-building. And we knew all the words to say thanks to Michael Aivazis, who became our chief software architect. Here's another interesting aspect that happened at this time. I had previously been associate director of the Seismo Lab. The Seismo Lab at the time had the Kresge Laboratory, our own campus above the Rose Bowl. I knew the space, the building, everything like that. We put together an internal proposal at Caltech, Jeroen and I. I worked with the National Science Foundation on this particular aspect, because we'd be part of the Seismo Lab, that this thing which emerged–and immediately, I knew what the future was, so I instantly got the domain geodynamics.org, even though we wouldn't use it until a year later.
We were going to build it in the Seismo Lab facilities. I already went through the space crunch here. We'd just built this giant supercomputer facility in the basement with the Dell supercomputer, and the Seismic Network had expanded beyond our wildest dreams, so we had no space. But the director controlled the old Seismo Lab. We went back and said, "We want to renovate the facilities." We'd do several different things. We'd house what eventually became the Computational Infrastructure for Geodynamics, CIG. It was designed on purpose to take the science out of it. We had this facility, and we said, "We want to renovate the Kresge Lab." In its cellar was this long thing for one of the Benioff strainmeters. The mansion had already been sold, but this was built from a grant specifically on the part of the Seismo Lab. We proposed that we'd renovate this whole facility.
Not only would we house this NSF center up there, but we'd make it like a conference facility. It's in a beautiful neighborhood. Unfortunately, it ended up being three McMansions on a little road once Caltech sold it. But the administration didn't really like the idea, and a person on the division's oversight committee, Sean Solomon, who eventually became the director of the Lamont-Doherty Earth Observatory, didn't like the idea. He didn't think it was good to take scientists and cloister them away off campus because one of the key things is for people to interact, especially mathematicians and computer scientists.
ZIERLER: Isn't this the whole reason the mansion was relocated to South Mudd?
GURNIS: That was one of the intellectual arguments, but I think another was that they didn't like the Seismo Lab being so independent, so they wanted to put it under their arms. A control thing by the division chairman, I think. Those are the two reasons, intellectual as well as control. Because the Seismo Lab was more powerful than the division, and that's always been a problem. Although, the division's gotten so big at this point, I don't think it's an issue anymore. But when I first came here, it was an issue even having a director of the lab. In earlier times, they viewed he'd be more powerful than the chairman, and they didn't like that. One of the problems that happened was, the provost, I think Koonin, realized that the Seismo Lab had this really incredible piece of real estate off campus.
It was part of our facilities, so I used to go up there. Koonin said, "Let's sell it." Somehow, the division chairman, provost, and the director at the time, which was Jeroen, had this idea they were going to sell Kresge. This was before real estate went so out of control, it was beyond the pale. They sold it and divided up the money. I think the provost took half, then they gave the other half to the Seismo Lab as an endowment fund. It's been a pretty good endowment fund for me, one of the few resources I have. But I'm bitter that they didn't give me all of it. It was ours, not theirs.
ZIERLER: Was Hiroo still director at this point?
GURNIS: No, Jeroen was director. He and I were scheming all the time about the future of geophysics in the United States. But here's the laughable thing. They put us here on campus, but we still had no space. But in the same year, Caltech suddenly bought the St. Luke Hospital in north Pasadena. It's still there, owned by a real estate developer who can't develop it. Caltech bought it for $12 million. Steve had the bright idea they were going to create this thing called CCIT, the Caltech Center for Integrated Technology. It was going to be a technology hub, we would have a north campus. My desire to open an NSF center on campus, which the director of the Seismo Lab at the time, Jeroen, had no space for–and they took the space we wanted to use away from us.
In one case, "Oh, no, you can't be off campus because you'll be isolated." Then, "Oh, let's put you off campus because now we've got this gigantic campus." They had two tenants eventually up there. They had what was going to become the Thirty-Meter Telescope and myself relocated up there. The Thirty-Meter Telescope was put in the religious compound, and I was put in the medical building. We took part of the floor out. It was basically like being in commercial real estate. I had my office and did my research program here, but this center I ran for NSF for more than five years was a facility off campus, and it was a stunning success. I got the thing going from 0 to 60, the community absolutely loved it.
ZIERLER: What were the research projects there?
GURNIS: We did everything. We had a whole team of half a dozen software engineers up there. And we basically took over the software development for mantle convection, seismology. It was a collaboration. We had the engineers there, and we worked with people all around the country. We divided ourselves into these different working groups. We had mantle convection, we had what was called short-term tectonics, which was earthquakes and things like that, long-term tectonics, which was mountain building and things like that, then seismology, and we also tried to develop [into the] Earth geomagnetic field, and I was nurturing that. We built all these things, and we had working groups. We basically did exactly what the NSF wanted to, we brought software engineering to the community in this really successful center.
It's incredibly successful today and just grows. But several things happened. In its last year at Caltech, it actually moved to CACR, which was much smaller, and there was some space over there. Then, Caltech decided to sell it. No one on campus wanted to go up. How did the Thirty-Meter Telescope and geophysics know it wasn't going to be a success? This thing was happening all over the country and had been going on since the 1960s. People had been building research campuses, building these arms. In the end, David Tirrell was the czar of it, but in the end, it was the czar of nothing. The interesting thing is, because I was actually on campus, Caltech charged the full overhead rate on this. They pulled in a lot of overhead on that grant. They renovated this area, and it was very successful, but then they wanted to get rid of it because no one wanted to get up there. Caltech sold it, when real estate was really high. Some numbskull bought the property for more than $30 million. Caltech made a $20-million profit on it.
Becoming Director of the Seismo Lab
ZIERLER: This was before 2008, 2009, I assume, when the market crashed.
GURNIS: I was director up to 2010, but we were on campus at that time, so a couple years before that. Then, Jeroen left Caltech unfortunately. They didn't know who they were going to make director of the Seismo Lab, so they had an acting director for quite some time. I was really, really busy. Then, the chairman basically came to me and said, "Mike, I need you to become the director of the Seismo Lab." This was Ken Farley. They needed someone prominent in the field and with a lot of management. I decided if I gave up CIG, even though I made it with Jeroen–he didn't understand geodynamics. He totally bought in with me on software engineering and software, but in terms of the culture of my community, I had those two aspects of it, the negative and the positive. And Jeroen only saw the positive. The negative being that my community did not want a science center, but it wanted something.
But I also saw the benefit of software engineering. Jeroen and I had this series of students and other folks we trained. We totally bought in. Then, Jeroen left, and there was a long period there without a regular director. I eventually accepted Ken's offer, but I said, "I'm going to have to pull back. I'll help the community move forward." We were actually up by then, and I would have to help write the proposal. I was on the writing committee. Even though I was director, it's all done by committee. We even appointed a chairman of the writing committee, even though at the time, I was going to be PI. When it was all being written, I was going to be the PI of this. It was a big group of people. We were writing this proposal, then near the end, they said, "We're going to have to find a new director." The community appointed a person, now deceased, named Louise Kellogg, who was in this field. Some activities would stay on campus, but it would move up to the University of California at Davis.
And it's still going like gangbusters. The proposal came back and reviewed with "excellent" reviews across the board yet again, and I take credit for those with the community. But I have to admit, sometimes people don't really understand why suddenly I was not the director after a while, because things were going so well. And it succeeded after that. But I became director of the Seismo Lab because I was passionate about it and knew it needed a director, even though I was not a seismologist. But I was very close to Don Helmberger, and he and I would talk all the time about different things, but almost always about science. I said, "Don, what do you think I should do? I'm not a seismologist." In the typical way of Helmberger, he stared out the window for a long time, like he always did. Then, he looked at me, and he just burst out laughing. He went, "Mike, Don Anderson wasn't a seismologist either." [Laugh] I can't believe he said that because he was really one of the giants of seismology.
ZIERLER: What do you think he meant by that?
GURNIS: He meant that for the last 20 years, Don wasn't a seismologist. In fact, he was more like me. We were putting things together, synthesizing how the Earth worked, leading the community. We weren't just observational seismologists. And at the time, Don hadn't been, since probably the late 70s. He was a big ideas person within the community, extremely influential. But Don Helmberger said that being a seismologist was not a prerequisite to being director of the Seismo Lab. That's basically what our core observational seismologist said.
ZIERLER: But isn't one of the bigger stories of the move to South Mudd…
GURNIS: Geophysics.
ZIERLER: Yeah, that's it. That it's more than seismology, it's now sort of geophysics.
GURNIS: Right, but it already made that transition because Tom Ahrens had been hired. I forget what year he came in. I know he was up at the Kresge Lab. There was a big explosion one time, and they blew out one of the labs up there. I don't remember the story, and there's no one around anymore who would remember that.
ZIERLER: Tom Ahrens got to Caltech in '67.
GURNIS: There you go. South Mudd hadn't even been designed yet. But I think Tom had already built some stuff up there in one of the labs. But because he was up there, they were going to design a major component of the South Mudd building for a next bigger light gas gun. Interestingly enough, I remember Tom telling me a story, and it must've been already when was at Caltech. He would become quite prominent in the field of mineral physics. If I've got this right, maybe part of the reason the building I'm in was built the way it was a reaction to the Green Building at MIT. There's an iconic picture of MIT from the Charles River on a beautiful, sunny day, which never exists there, with all these sailboats.
You get this building right in the middle with these radar domes on the top. It's this little, teeny skyscraper. That's the Green Building, which is an absolute abomination for collaborative science because there could only be a couple of professors on each floor, and it just totally segregated things. All of a sudden, Caltech was going to build its new geophysics building, and Clarence Allen took the lead. In reaction to the Green Building, they were going to build the South Mudd building here on our campus, and it was going to be just the opposite. Totally level, with the ability for everyone to interact with one another.
The staircase in particular was designed to be completely open, so people could see each other from one floor to the next, could start talking, could have discussions there, and the hallways were wide, so people could have discussions in the hallways. Of course, our seminar and coffee rooms were built for everyone to talk with one another. And it was in response to the Green Building. I think Ahrens told me that. He told me a story one time that MIT was trying to hire him away from Caltech, and he was having a hard time thinking about how they were going to mount this gun in the Green Building. Somehow, it was going to be shot up vertically. Crazy. Tom was an interesting scientist.
ZIERLER: I want to go back to the 200 computers you built with Jeroen. Let's start with the name. Why Beowulf?
GURNIS: Beowulf is from Nordic mythology, a small, scrappy guy. He fights Grendel. It's one of these David and Goliath stories. It's a Caltech story much more than a Seismo Lab story. Wikipedia attributes the name to NASA. "The name Beowulf originally referred to a specific computer built in 1994 by Tom Sterling and Donald Becker at NASA." Presumably, NASA/JPL. There's a lot of stuff about MIT in there, but he was at Caltech for a long time. The name Beowulf comes from the Old English epic poem of the same name. But it filters into this idea that we just rolled up our sleeves, spent $100,000, and built the equivalent of a multimillion-dollar computer. That's what made it an underdog. And I think we built the biggest one at Caltech.
ZIERLER: How long did it take, soup to nuts, to get it up and running?
GURNIS: Several months, at least. Jeroen delegated the research to someone else, but he was very careful to make sure he spent a lot of money on the fans so air moved. We didn't want it breaking all the time. I'm sure there are pictures of the computer and us assembling it. It was fun.
ZIERLER: Did you see this technology as sort of the updated version of parallel computing?
GURNIS: It was specifically for parallel computing, period. We built a high-speed interconnect. We bought a system from an internet provider, Cisco Systems or something, to make it go very fast. This was definitely in our calculations. And that was one of the most expensive parts of the computer. It wasn't the ethernet cables, it was this box from Cisco Systems that could transfer the message-passing from one computer to the next very quickly.
ZIERLER: What was an example of a particular research project or question that became answerable either more efficiently or period due to it?
GURNIS: I wanted to take CITCOM and run it with the detailed material properties of the mantle. And it took a lot of computer power. That was one thing we were doing. The other thing we were doing, and this was in line with the connection between SPECFEM and CITCOM, we wanted to do a lot of seismic calculations simultaneously. One of the things we had pioneered at the time, which was very powerful, was to take our models of the mantle and do the kind of stuff Don Helmberger did with the synthetic seismogram, except do it for millions of seismograms. There were several papers in there in which we combined seismology and geodynamic models in this way. I had a student, Magali Billen, who became a prominent professor at UC Davis, and she used one of the versions of CITCOM on these parallel computers. Again, she put in all these intense variable-viscosity and material properties. That's what makes plate tectonics different from fluid-dynamical convection. There are all these intense material properties. We wanted to scale it up into 3D. A lot of Magali's work was done on these big parallel computers we built in-house.
ZIERLER: Did this system convert some of the faculty and students in the Seismo Lab? Did they see what was possible as a result of having this?
GURNIS: A lot of people left and built miniature versions of what we had here, that's for sure. But the software, both SPECFEM and CITCOM in particular, required computers like this. We may have been first, but it was quickly forgotten that Caltech led in parallel computing. Simultaneously with the work Jeroen and I had done, there was a student in the Seismo Lab as a post-doc named Brad Aagaard, a student of Tom Heaton. He became an expert in finite element analysis. He wasn't that close to Jeroen and I at the time, but he was also using parallel computers, probably the Intel machines and other variants. Eventually, I guess he did probably use the Beowulf here. They actually ran the first 3D dynamic simulations of earthquakes. The area that Nadia Lapusta, current faculty member, has come to dominate. But maybe when she was a graduate student or whatever, Brad was doing these things at Caltech with Tom Heaton. That's another big example of parallel computing.
All these different areas, dynamic rupture, mantle convection, tectono physics, regular seismic wave propagation–all or a large fraction of our students realized that large-scale computing was required. For a lot of people, but not for everyone. Interestingly enough, some of the work Don Helmberger's students would do would not be that computationally intensive, but we would take it and make it computationally intensive. With students, they made it with lateral variations in seismic properties, then we would want to do millions of calculations simultaneously with Don's codes, with other students, and things like that. Computing and geophysics have gone hand-in-hand ever since the 1960s. Even before we got our hands on supercomputers, one of the big uses within the country, before the NSF supercomputer centers, was the oil industry for seismic processing. The big three things in the country in the 60s and 70s were making atomic weapons, predicting the weather, and finding oil with seismology.
ZIERLER: The holy trinity. [Laugh]
GURNIS: There you go.
ZIERLER: In our next talk, I'd like to pick up on when you first got the sense that Jeroen Tromp was going to leave and what that would mean for you.
[End of Recording]
ZIERLER: This is David Zierler, Director of the Caltech Heritage Project. It's Tuesday, September 27, 2022. It's great to be back with Professor Mike Gurnis. Mike, once again, great to be with you. Thanks so much for joining me again.
GURNIS: You're very welcome, David. It's great to be here this afternoon.
ZIERLER: What I want to do today is pick up in the area of 2008, 2009 when it became obviously that Jeroen Tromp was going to move over to Princeton. First, on the research side, what did this mean for you? Were you still intensively working with him at that point, or had the computer system more or less been set up, and you were both onto different things at that point?
GURNIS: Once Jeroen had set all of this up, with the development of his new software platform and his ability to do these computations of synthetic seismograms, for all intents and purposes, and our simultaneous ability to do convection, it was kind of a hard slog in Jeroen's research. And I think we all recognized that, even though he had made this big jump in methodology. It turned out the next step for his code was called full-waveform tomography. And we wanted him to do it for the deeper Earth, to do it for regional problems, and we had different ideas about all of this. But in reality, it actually could only be done in a practical way for limited regions. He had a student named Carl Tape. I think he finished up after Jeroen had left, but I think it was Carl's research, which demonstrated to all of us both how powerful the methodology was, yet on the other hand, how intensely difficult it was to move forward, and progress was going to be slow because in order to do the full inverse to the 3D structure with this method would be very slow.
I think it was disappointing to see Jeroen leave because he was a great colleague, and it was great to talk with him. He also strongly believed in computational methodologies, which initially not everyone was fully behind, but generally most people were. It was mostly just sad. That was just the course of things. For personal reasons, Jeroen thought it was best that he move to the East Coast. It obviously was a disappointment for us. There were a lot of other people at the time, so it didn't feel as harsh as it probably should've felt. I think we also had Pablo Ampuero on the faculty, and I'm trying to remember if we had yet hired Victor Tsai yet. It was a disappointment to see Jeroen leave. We had a lot of things planned, and he liked to think big, which was great.
ZIERLER: I know there's some variation for the length of term for Seismo Lab directors, but I assume for Jeroen, it was pretty short in the grand scheme of things.
GURNIS: It was kind of short. I think he would've remained a very nice director. I think Don Helmberger was director for five years, and Jeroen's tenure may have been just slightly less than that. Caltech has a standard term these days for most administrative appointments. The default appointment is five years, and they don't really like to continue to appoint people after two terms, but they're continuing to appoint me after 10 years. We had this intermediate period, Hiroo became director with TriNet and TERRAscope, which was really great. Then, Don took over, and that was a little bit difficult. Scientifically, it was wonderful within the lab. We discovered a lot of things and wrote a lot of papers, but I think it was quite difficult for him because we were making this transition where the Seismo Lab was a little bit on the forefront of our digitization and computerization of our operations, which kept continuing.
Don had to sort out this transition between having people who normally read the records and having the computers do a lot of the work, and then people checking on it. Then, the funding was a little bit low for a while pre-earthquake early warning, and then, of course, earthquake early warning took off. But that was during my term. Don only kept it for five years, and then when he finished it up, Jeroen seemed like a natural pick to be director. For reasons entirely unrelated to Caltech, Jeroen thought it was best for him to move to the East Coast. His time was a little bit on the short side. It would've been really nice if it was longer.
ZIERLER: As he put it to me, as a Dutchman, he just needed more rain. [Laugh]
GURNIS: Yeah, he left for reasons unrelated to science. But he's a great guy.
ZIERLER: What did it mean for you? Did you recognize at the time that you would get tapped next?
GURNIS: Initially, I was not tapped next. I was so busy with other activities that Ken Farley did not appoint a regular director for a while, maybe a year or something. Then, he asked me if I would be willing to do it. And I wasn't really happy because I was helping the NSF out in the geophysics community move forward in software engineering at the national level, and we were going like gangbusters. There were a lot of people to manage, a lot of money to manage, a lot of politicking because it was a national thing. But because I had all this experience, more than anyone else around, I think Ken eventually felt he had no alternative than to ask me.
At that point, I didn't know how things would work out because I had this role–the community was okay, we put in enough checks and balances within community oversight that we could figure out how to do it. It was also at kind of a difficult time. I had gotten the thing going, and it was really going well. We were already writing the proposal for the next phase. The committees had appointed a committee to write the proposal, even though I would be the PI, then there was an executive committee, and we liaison-ed with them, and they set up a procedure by which the community would find a new director in, if not a democratic way, a way which was transparent.
ZIERLER: From your perspective, what did the year without a director for the Seismo Lab mean? What was lacking that Ken clearly needed somebody to run things at the Seismo Lab?
GURNIS: Eventually, a director is absolutely needed, especially these days. There are budgets, people to manage, a lot of giant projects. Now, we have two research professors, but we need people to be their supervisors, and there's just a large staff. Rob Clayton was the acting director, and the division eventually decided that it needed a permanent director. I forget whether I announced to the community that I would pull back from the community efforts in geophysics or if I first agreed to do this for Caltech. But then, for a while, I had to do both. It was fine. Now, I know I could've done both, which is a terrible thing to say. But it wasn't good for me to do.
And it was very interesting, it turns out there was kind of a freedom that I also felt on the community side of things because within my own area of geodynamics, I wanted to steer things in a different direction from my research of going into inverse models, but the rest of the community did not want to do this. And I had started these collaborations with these mathematicians and computer scientists, and our goal was eventually to do inverse models, but we had to do this big step in terms of methodologies for doing the computations on parallel computers. And there was massive skepticism amongst my colleagues about doing this, so it was kind of good that I freed myself from the community oversight of geodynamics because the community didn't want to do what I wanted to do. It freed me up a bit, I think.
ZIERLER: What was the big ask at this point when you were thinking about these things at a national level?
GURNIS: We had already set this stuff up at the national level. We'd been going for five years by this time. We convinced the National Science Foundation that what geophysics needed was infrastructure for software and software engineering, and with community oversight and best practices. And Jeroen and I figured it all out from our own research more than five years prior at that point, and we figured out the way to move forward. And I'd spent the last five years implementing it for geophysics. Through the grapevine, we found out that the NSF eventually created what were called software institutes and patterned them after our idea in geophysics. They don't publicly say this, but you can hear program officers occasionally mention this. But things get lost, there are a lot of egos involved. But I'd heard that, so it kind of tickles us pink in geophysics that we played this role for the broader scientific community, even though it may not be widely appreciated outside. And there's a lot of turnover for program officers at NSF.
ZIERLER: When you became director, what perspective did you come to appreciate about the Seismo Lab that you couldn't get as a civilian, so to speak?
GURNIS: Two things. I had my own experience in administration, which was now quite extensive by that point, but then I mostly grew up scientifically within the Seismo Lab, so I knew why it was special, that interdisciplinary interaction between people. And for me, that's always been the most important thing, to keep the Seismo Lab small and keep people interacting with one another. And that's it. The science is going to keep moving forward, and I personally can't move the science forward myself, it's got to come from the brilliant young scientists with new vision in terms of technologies, methodologies, and ideas. It's always been that I leave things in the hands of young people, but the other senior faculty and I who believe in this strongly try to keep this going, and that's sort of it. I don't want to make the Seismo Lab this gigantic soft money outfit. That's a typical way that a lot of things progress. Years ago, I'm sure the Seismo Lab, if it wanted to, could've broken apart in the sense of doing stuff on campus, then having these gigantic centers. Sometimes those centers become larger than the parent institution, as large as a department. We see them in the neighborhood here.
But on campus, the things that really make it work are the things that are small and intimate, things that harken back to what makes Caltech good, which is people interacting with one another, whereas the overall trend in science, including many things Caltech spins off, and very successfully, is to make things gigantic under the government's largesse. Oceanographic institutions are a great example of this. They became gigantic and grew and grew, until they reached the point they couldn't grow anymore, and then there was all this pain. My ambitions have never really been to make this thing big. When I did the geophysics things for the community, I wanted it to be of a certain size, and it got to that certain size. I didn't want it to be my own or anything like that. I just sent it off on its own way.
ZIERLER: On a personnel level, what opportunities did you have for hiring at that point, while keeping it small and interactive? Were there new, young faculty you were in a position to recruit?
GURNIS: The folks who'd left, like Victor Tsai and Pablo Ampuero, were recruited with Jeroen. The geophysics faculty wanted to hire these two folks after we'd identified them, which were Zhongwen and Zach. I think that's the greatest thing that's happened since I've been here, the hiring of those people. They're the ones with the brilliant new ideas and moving the scientific community forward. But they're moving it forward because they're at Caltech, which is a great, interactive, and supportive environment. That's my role, in my opinion, to do that. It's about the core faculty. It can't be too big. And we really want to hire some more young assistant professors. The faculty have various ideas, but when it really comes down to it, it's almost always going to be that we're going to hire the best people we can find. We're going to look around, and when we see another great person, we will hire them. And we're looking around.
ZIERLER: What did the 2009 financial crisis mean for the Seismo Lab? Was there any belt-tightening that needed to happen?
GURNIS: No. We got money in a number of different directions. Once the idea of earthquake early warning came out, there turned out to be another very interesting direction we could take. FEMA was sending money to different disaster districts. That's not the right word, but there are districts around the country centered around large metropolitan areas where FEMA was sending lots of money, and they were sending money mostly to buy a lot of fire equipment. It also was the time when police departments got highly militarized with a lot of heavy-duty funding from the federal government. The city of Los Angeles actually had quite a bit of money in their pot, and they couldn't really spend it anymore. But the mayor of Los Angeles and his team got really convinced about earthquake early warning, and they funneled a huge amount of money back to Caltech. It was from FEMA, to LA, to one of these districts, and it somehow got back to Caltech. And we bought all these seismometers with this.
Simultaneously with this going on in the 90s, Ken had a predecessor named Hall Daily, and he worked basically for the Caltech president as our government relations officer. Hall worked very closely with the Seismo Lab, and he also works very closely with our lobbyist in Washington DC. With the lobbyist and Hall, we set up this group of Caltech and several other universities in the western United States. But it was all organized by Caltech. We got everyone together to move forward on earthquake early warning, and it was a really, really strong effort to engage with our congressional members, the senators in our respective states. Eventually, through this really honest lobbying on the part of the scientific community, in a very collaborative way, we convinced the congressional delegations in these states to move forward on earthquake early warning. There were even people in the USGS who weren't fully behind earthquake early warning, but it eventually got into the president's budget, and it was huge.
I forget what year all of this started, but we had earlier funding through the FEMA program, this funding, and then funding from the state of California just five years ago called CEEWS, California Earthquake Early Warning System. But of course, Caltech was way ahead of the curve in terms of seismometers. You look at maps in the Western United States, where we needed to monitor so we could really do earthquake early warning in a very practical way, all the dots were in Southern California. It was just crazy. First, after Northridge in '94, the Seismo Lab had convinced FEMA to support all these seismometers, and they all went to Southern California. We kept building up, and then around 2010, we got this money funneled through FEMA through these disaster areas, and we got more seismometers. At that point, earthquake early warning was going to hit the fan several years ago, then we brought in the state of California in an even bigger way. We're still way ahead of the curve at this point.
Another interesting thing happened at that time, and maybe this was closer to 2000, when Jeroen was director. But the scientific community had started on this other thing called US Array as part of this program called Earthscope. They were going to cover the whole United States with seismometers, and it would be this moving thing. The stations would sit for 18 months and then move. It was going to be called Bigfoot. They started right here in our operating area. For the first year, Earthscope was, like, a year ahead of schedule. According to their statistics and the numbers that the NSF was quoting, Earthscope was one year ahead of schedule because Caltech, to all these other ways, had gotten all this support from the federal government to put seismometers here, and now they were re-designated. It was an administrative thing to get this NSF program a year ahead of schedule. Of course, all those stations stayed put because they were ours, and the rest of Earthscope just moved out from here. But that was great.
Building on a Tradition of Earthquake Early Warning
ZIERLER: What was the promise of earthquake early warning at that point?
GURNIS: It's always remained the same. Tom Heaton, who really is the father of earthquake early warning in the United States, wrote this very famous paper in Science magazine, even though the overall concept was not invented here. But the implementation within the United States was Tom's idea. He was the driving force behind it. The difference with our earthquake early warning system, to contrast with the Japanese, is that we have our network here, and the earthquake's going to happen right here in the network, whereas in the case of Japan, I won't say it's easier or harder, but it's different in the sense that you have a remote earthquake, and you're now detecting that it's coming towards you. We're basically sitting on the time bomb, and it just goes off, so part of it's going to go off without any warning whatsoever. The promise of early warning has always been that we're going to get some warning of earthquakes to help us mostly with the infrastructure, and to give people some confidence that they can drop, cover, and hold either seconds or up to one minute before the intense shaking will actually happen.
And to be honest, we will have devastating earthquakes here in the urban area of greater Los Angeles and surrounding counties. And they're not even going to be the big one. They're going to feel like it, but they won't be it. We have a couple of big-one scenarios, and it will happen in the southern part of the Salton Sea potentially, rupture upwards, and then go on the back side of the San Gabriel Mountains, or it could potentially happen up near Fort Tejon and maybe migrate downwards. We'll get a good minute of warning, especially if it happens out in the Salton Sea. For the large majority of the infrastructure within Southern California and for the largest population centers, we're going to get a big one, and it's going to be extremely important for the utility providers, in my opinion. And it's worth the money.
ZIERLER: That was my question, if it's really a good investment from their perspective.
GURNIS: Yeah, it is. Villaraigosa, the previous mayor of Los Angeles, took this on, and the current mayor and governor are extremely supportive of our efforts for the broader scientific community. But it's well worth it. In the end, it's not a gigantic investment compared to all the other investments we make in public safety and preparedness. But it's a good one because it's very well-understood, and all the politicians now can claim credit. And rightly so. The main politicians, at least in Southern California and at the state level, can claim a lot of credit for what they've done. They've known this will be a really big problem that will hit the fan at some point. But yes, it's a good investment, if only for the peace of mind. When this thing goes off for me, even if it's a little bit of shaking, it gives you some peace of mind when the alarm goes off and you know it's coming.
ZIERLER: To clarify, was this investment from Los Angeles all about buying more seismometers, or was there opportunity to invest in basic research?
GURNIS: No, the Seismo Lab faculty hasn't thrown themselves universally behind this because there's not much research the Seismo Lab faculty can do on it. There are important scientific unknowns. The biggest issue we have in Southern California for earthquake early warning is when the earthquake can happen. Let's say it's going to happen near Bombay Beach or something like that, the southern part of the Salton Sea. It's going to start going, and it's going to be a 5 and grow into a 6, then into a 7. But we don't really know because the earthquake just gets bigger and bigger. This is the interesting thing. The Caltech faculty have been on one side of the fence, and a lot of people in the community have joined us, then there are these other people–we just can't tell if an earthquake is going to be big or not. An earthquake starts, and it grows, and at some point, it stops. Little earthquakes, right at the beginning, look just like the big one's going to look. Sometimes they just get bigger and bigger, whereas at some point, the earthquakes get slower.
Some people in earthquake physics, which is not my area, ask why an earthquake stops growing. That's one of the big questions in earthquake physics that people working at a very fundamental level, like Nadia Lapusta, work on. But we just don't know. For earthquake early warning, one of the issues is that we tend to initially underestimate things. If we have an earthquake right now, even a small one of 5, and it starts in the Salton Sea, my computer will start buzzing. But it's going to say it's a 3, and then it's going to grow bigger as time goes on. As the rupture starts propagating up the San Andreas Fault, it's just going to get bigger and bigger. And with our seismic instruments, initially, we can't tell, so we tend to underestimate the size of the earthquake. But by the time it unfolds with GPS, you can. In some scenarios, we will already be shaking here in Los Angeles, and the big one will still be growing. It's still a big fundamental unknown, partly scientific, having to do with the physics of the earthquake, and other parts are sort of practical.
Earthquakes don't get big until they're big. I know that sounds silly, but it's the truth. But some people thought there was some magic where you could see, at the first part of the P-wave, that it was different. Hiroo was the greatest naysayer of this. He'd just show the two seismograms and look at what the displacement looks like as a function of time. He'd lay them right on top of each other, and there would be zero difference. Hiroo was the biggest person who poo-pooed the idea that you could tell something special about that little one because there wasn't anything. It was sort of a pipe dream. I think everyone in the Seismo Lab agrees with that perspective. But people keep pursuing it, thinking there's going to be something special they'll be able to see right at the beginning where they'll be able to say, "Oh my gosh, it's the big one. Sound the alarm right now in LA. It's a magnitude-8.3. Everything needs to be turned off, the power company needs to redirect the power, everyone needs to duck, cover, and hold." Then, it comes in as a 5.
ZIERLER: Let's turn back to your research. Once you figured out how to balance administrative responsibilities directing the Seismo Lab, what research were you focused on circa 2009, 2010?
GURNIS: The most important thing at that time I was involved in was this external collaboration that got freed up for me. It was all the people I had met through this CIG project. My contention has been that what makes plate tectonics plate tectonics is that we have these extraordinary mechanical discontinuities in the system. If we were going to simulate mantle convection and flow, we really needed to get these very fine-scale features. But we wanted to do it on a global scale. Since the late 70s, early 80s, individuals had been simulating, with mathematical tricks but incorrect physics, how you could have global plate motions but still viscous flow with faults. People still hadn't been able to do that, and it remained a gigantic computational challenge. But I was working with this group of individuals, and there was this mathematician.
Because of CIG, I struck up a collaboration with Omar Ghattas, a professor at UT Austin. I convinced him that what we wanted to do was see if we could simulate the Earth at a kilometer scale. People at that time weren't even doing tiny models of this. I brought the physics to them. I guess Omar had just moved to Texas. He had these three post-docs who were all brilliant in computation and applied mathematics. Those three post-docs, Omar, and myself all worked closely together on this topic. And I actually can't claim credit for it, but I was motivating it, and they made a huge breakthrough in computer science. It was to do a methodology that had been around since the 1960s called adaptive mesh refinement, AMR. One of the great things about computational methods is that they can have a mesh, and if you have a lot of details, you can have a very fine mesh. The word adaptive is critical. It adapts, so the mesh itself adapts to the dynamics. Again, this had been around since the 1960s. This would be hard to do, but I'll use it as an example anyways.
Take something like turbulence. Let's imagine you have a turbulent eddy that went off. "Oh, boy, that's got all this fine detail," so you want to zoom in on it, have that as a fine mesh, and let that mesh follow that thing. But then, everything behind it is sort of just laminar flow, and you don't need the mesh. People figured out that you could do this, but on a parallel computer, you needed to hold all this information in memory, this very complicated mesh. People could make AMR, but they could only scale it up to about a dozen processors. That just wouldn't work. Then, this group I was working with said, "We can use a tree structure," which is a logical way to represent data that's very compact and can be represented by this very simple data structure with a bunch of integers, yet it can represent in incredibly complicated spatial detail. But we were going to do the Earth, and the Earth, as a sphere, has naturally periodic boundary conditions.
It comes back on itself. They came up with this idea that you could have a forest–first of all, you could represent a mesh with this tree structure, and then you could have a forest of them, and they could have a periodic body condition, so they could talk to each other, no matter which direction you went in the forest with these different trees. We'd take equations I knew very well and that we'd been solving for a number of years, the Stokes equation, solve it with AMR, and do the whole world and at a kilometer scale. I may have still been the director of the CIG, but we had a small workshop on this, just people who were very, very good in this field. We brought all the people in geodynamics together with the people in applied math and computation who do this method. We were going to do Stokes equation on a globe at a kilometer resolution. We were laughed out of the room. They said, "What you're saying is impossible. You're fantasizing." 18 months later, the front page of Science magazine was our visualization with our article inside.
We showed we could solve the problem at a kilometer scale. We got all the physics of plate tectonics in. We worked really, really hard on that. And that was a breakthrough attributable to the broader community, not just Caltech. That was one thing I had done at that time. But in parallel, I was working with my students here on using the stratigraphic record and the record from the continents, how continents went up and down, as a constraint on what the stresses and forces were on the mantle. But it constrained it as a function of time over long periods of geological time. I was working with two students, and this was done on the big parallel computers we were building, which is why it was so important to have those computers. We basically built an adjoint of the mantle convection problem. I was not the first person to write a paper about this.
Now, we're talking about the time-dependent flow of convection in the mantle in a sphere. We were going to take it back in geological time. We'd start with our present-day images of the Earth, then we'd use plate tectonics–because I was also working on this GPlates project, then I would take my stratigraphy, then there would be the constraints as a function of time–and try to solve the four-dimensional problem backwards in time with an adjoint. This is a mathematical trick we use that's widely used in weather forecasting. When we have this weather forecasting for storms, they use an adjoint model, and they're continuously assimilating present-day information, and they project it backwards and forwards to try to get it right. We were going to use some of these ideas for mantle convection. My students had to develop all the adjoint equations for the convection equation, then integrate it backwards in time. And we also had an article in Science magazine on this with my students. It was just a Seismo Lab thing. That was very important because it was an inverse model.
Those were two of the things we were working on. The big thing I did with Omar Ghattas, we couldn't use our own computer here internally because it wasn't big enough. And we continued that work over several years, and several years later, we won the Gordon Bell Prize, which is the top prize in computing. That was when we took our equations and were able to run them on a million processors. Because they're implicit equations, not explicit. Jeroen's equations are solved explicitly. It's just natural to solve them on a parallel computer. When you do things implicitly, it's a nightmare to solve them on a parallel computer, but we worked with this group to figure out a way to get it on the largest computer that Lawrence Livermore Laboratory had. It actually was behind the fence. We actually went up to two million processors, and it was staggering.
We basically had linear scaling all the way up to this. And it was the same problem we'd been laughed out of the room about. We eventually showed we could go without any bounds whatsoever if the computer was big enough. I glossed over a bunch of things in there, but computing was one of the major themes in all of thanks. I met a lot of people in high-performance computing through the CIG program. A lot of it was done in the Seismo Lab with Caltech students. The paper on the first adjoint was done by Lijun Liu, who's now a professor at University of Illinois. He's become very prominent in the field and has had a number of breakthroughs since leaving here. He's very creative.
Unresolved Questions in Plate Tectonics
ZIERLER: What were some of the big debates with mantle convection at this point?
GURNIS: At this point, for most of us who really understood the problem, the upper mantle and whole mantle convection thing was totally solved. Basically, it was a hybrid mode of convection. We knew that the big slabs had gotten deep into the mantle. And that was actually kind of critical for us to reverse convection all the way back over the Cretaceous and solve a major sea-level problem with that with the adjoint models. But basically, the slabs go down into the deeper mantle, but the big issue that started to emerge was that besides the return of plate tectonics to the deep interior, we had these other gigantic structures in the mantle, not the downwellings, but the upwellings.
There were these very large regions in the lower mantle that, when seismologists first discovered them, we called super plumes. Don Anderson didn't like plumes, so in a tongue-in-cheek way, I really took to calling them super plumes. Eventually, the geophysics community did not like that word and moved on to unfortunate names that were too technically complicated. And there were two big debates the community participated in. Are they thermochemical? And are they stationary or mobile? These had been known since the 1980s. One of my former students, Shijie Zhong, and as post-doc took Louis's code and made it spherical while he was a post-doc, and he called it CitcomS, and we've used it to do a lot of things. But one of the things Shijie did was, with one of his post-docs, he could take the history of subduction and reproduce where these guys were located.
He could basically get the large-scale geography of the lower mantle. To me, it was obvious, so I wasn't surprised at all by this, but it was an important breakthrough, and we've worked on it quite extensively ever since. But we have thermal convection, and cold material goes down, hot material comes up. Then, you have all this very dense material, and because it's dense, it's going to mostly want to sit in the deep mantle. It's stationary, but it gets moved around. This first emerged in a calculation I did as a graduate student, the first time you could see these large thermochemical structures in the lower mantle that would move around with plate tectonics. They were kind of opposite to this idea I'd developed for continents, which was the computation of an idea Don Anderson had, that you'd have these light rafts on the surface of the Earth that would move around, go into supercontinents, and then break apart. But they'd stay up there, sit over the cold areas, accumulate into a supercontinent, then break up and move apart. We showed this when I was a post-doc at Caltech.
But the opposite of these things are these thermochemical structures in the lower mantle. We worked a lot on those things, a number of students did lots of terrific work on them in terms of their stability and mobility and what the nature of them could be. That was the big debate, these big mobile thermochemical structures. Most of the scientific community believes they're mobile. There's a group with senior members of the geology community that thinks they're stagnant and stationary. But I don't think they've convinced most of the geophysics community. There's been a big debate involving geodynamics community, including people like myself and Shijie Zhong, and the University of Colorado has done a lot of work on this, about the mobility of these structures in the deep mantle. That was generally what we worked on into the 2010s decade. And we're still doing a little bit of work on that, but not a lot.
ZIERLER: Where is plate tectonics in all of this? Where does it fit into the research questions you're asking on mantle convection?
GURNIS: It's such a complicated problem that it's not fully solved. Because of that, I've tried to take the problem apart. Last time, I discussed the initiation of subduction and trying to understand that problem because there's not wide acceptance on it since it doesn't follow directly from the kinematic record that you can clearly read with plate tectonics. Subduction sort of eats its own geological record, so you can't directly infer the beginning of subduction from the plate tectonic record. You can piece it all together partly by the plate tectonic record, then with the record of other observations. I worked a lot, myself, with post-docs and then with graduate students on the mechanics of this process because it's still sort of the holy grail.
But because our models had gotten ahead of the observations, I did go off on a secondary career in marine geophysics in terms of both ocean drilling as well as regular geophysics out at sea to try to collect the observations at key areas in the ocean, in the western Pacific, in particular, to help us constrain the models. That's one way, the mechanics of how it works. But there's another whole issue. Don Anderson coined the terms bottom-up or top-down convection. Is what we see at the Earth's surface the guiding principle? It basically determined everything you see in the deep interior. The physics of plate tectonics and how it works would be top-down. Then, in bottom-up, you have a bunch of plumes, maybe in the mantle, and they cause gigantic upwellings. This causes and influences the tempo of the Earth. I believe it's probably a balance between the two. Because it's such a complicated process, I don't believe it's fully solvable.
What we've learned, in large part thanks to Shijie's work demonstrating that the large-scale geography of the deep mantle can be predicted by evolutionary plate-tectonic models, is that you can take this sort of top-down approach observationally. We've done a lot of work, as have others in the community, on trying to use more and more sophisticated models of mantle convection with some of the physics, but not all of the physics, of plate tectonics, plus the observed geological record of plate tectonics–another thing I've worked very hard on synthesizing with collaborators–and using that approach to "predict" the things we see in the Earth's deep interior. But I don't think it's a fully solved problem. Don Anderson's catchphrase of top-down or bottom-up–I think people in the community like to pick one direction or the other. But I don't think we can fully answer it at this point in time.
ZIERLER: Last question for today. As high-powered computing was becoming more and more recognized in geophysics, where was the software playing catchup with the hardware and vice versa?
GURNIS: The hardware generally just gets better, and you have to figure out better algorithms to take advantage of it. Generally, bigger speed-ups have occurred because of software compared to hardware. But new hardware triggers new software development. I think Jeroen Tromp's research with SPECFEM and the spectral element being applied is a great example of that. I think the collaborative work I was involved in with Omar Ghattas was a great illustration of catching up on the geodynamic side of things with the hardware. But in general, it's sort of like, "We have this hardware. How do we exploit it?" Right now, the largest machine NSF is supporting for scientific research is called Frontera. It's sort of a take-off on Vannevar Bush's thing, The Endless Frontier. But it's also Texan, so Frontera. In any event, this is a big computer, and we're on it. We competed to get onto it. One of my students uses it. But we're actually in the next phase of this right now, trying to think of what will be beyond Frontera.
NSF is going to come up with two different supercomputer centers, one sort of the routine supercomputer centers they're currently funding, which we use a lot of, and the other one is going to be a follow-on, which will be more like a national center based in Austin. NSF is now trying to figure out what the architecture of this computer is going to be. NSF has selected 30 projects called CSAs, Characteristic Science Applications, and we have one, a great earthquake project. There are four projects, I believe in Earth science. One of them is for the atmosphere, and three are for seismic. Three out of 30, that's 10%. The other two are basically speed-ups of existing methodologies. They're already characteristic science applications. We've actually proposed one where we have no idea what the heck we're doing. We're trying to make a quantum jump. Nadia Lapusta is on the team. It's a national team, but the biggest group is at Caltech.
I guess I'm the project leader. It's Great Earthquakes and Plate Tectonics. Our idea is to try to do the great earthquake cycle at the same time we do the motion of tectonic plates. One of the big areas of geodynamics right now is trying to cross time scales, between the short-time-scale physics of earthquakes unfolding on one hand and slow tectonic motions on the other. Essentially, in all cases, the way this is being looked at–Nadia did a classical example of this for her research on the San Andreas Fault. The San Andreas Fault moves with the motion of the Pacific Plate with respect to the North America Plate. She takes that slow motion and says that in the far field, she knows the two plates need to move at that rate. Then, she unfolds, based upon the physics of the earthquake process, how the earthquake unfolds.
And people are also doing this with method subduction zones, and a number of very interesting papers have been written. What Nadia and I are attempting to do is to not impose plate tectonics, but have plate tectonic motions emerge from the earthquake model itself. They actually would go back and forth. But this is such a transformative calculation beyond anything we've been trying to do. It basically would be the same model we're trying to use for instantaneous plate motions, the code that won the Gordon Bell Prize, with the physics of both elasticity and viscous flow in there with the frictional physics of an earthquake. Ours probably won't get selected because we're competing against codes that already go against it. We want to do something truly transformative and take earthquake science to the next step. My own feeling is, this is it for plate tectonics. This would be the next big thing.
Can I get the Pacific Plate and any super cycles in the occurrence of earthquakes simultaneously with the great earthquakes themselves as opposed to assuming one or the other? In geodynamics, it's always been, "I put a fault in there. The fault exists, and now the plates just slowly creep along with the negative buoyancy that exists in the system," which we can measure with various ways. Or, "Can I just say plates go at five centimeters per year, grind past each other, and then all the frictional physics unfolds?" I want to do the true coupling on a global scale. People are going to probably take me to the mental hospital for trying to imagine that one can actually do this on a computer. But we've been laughed out of the room before, and I'm prepared to be laughed out of the room again. I might be able to do this before I finish up, and I want to finish up being director quickly so I can spend more time on this problem with Nadia. And again, there's a small national team of investigators working on this. That's what I think the holy grail of geophysics is.
ZIERLER: We'll pick up next time and see where these things are headed.
[End of Recording]
ZIERLER: This is David Zierler, Director of the Caltech Heritage Project. It's Wednesday, October 19, 2022. It's great to be back once again with Professor Michael Gurnis. Mike, as always, great to be with you. Thanks for joining me.
GURNIS: You're very welcome, David. It's great to be here.
Emulating Greatness in Geophysics
ZIERLER: Today, I want to pick up something we talked about in our very first conversation, but now we can talk about it in the historical narrative. When you were named or decided to become Seismo Lab director, with your appreciation of history and the rich heritage of the Seismo Lab, were there any particular directors in the Seismo Lab's past that you wanted to emulate or who had particular leadership styles that spoke to you?
GURNIS: That's a very interesting question. Most of the directors I knew–I guess, in some sense, I knew four directors extremely well, Don Anderson, Don Helmberger, Hiroo Kanamori, and Jeroen Tromp. And Jeroen and I are more contemporaries. I think maybe Don Anderson. I've been quite hands-off, and I think he was, too, in the sense that I didn't want to steer the direction of the Lab in my personal direction. My own feeling is to sort of get the best people and then just symbiotically, the group evolves forward on its own. I sort of have a very different view of the Lab than I have of my own research program, especially when I was younger and mid-career, where it was kind of like, "Absolutely, I want to go in this direction. We're going to go in this direction and throw all the power in there."
There are two views of the Seismological Laboratory. I'm sure there are more than two views. But there's the naive view, the probably external but nonscientific view, that we're sort of a seismic monitoring group, we study earthquakes in Southern California, monitor them, and report on them. It's a very narrow view. Logistically, it's a huge undertaking, and we do it with great skill and success. But that's one view. The other view is that we are a small group of scientists that communally works together. To me, that's the most important thing about the Seismo Lab, in the sense that it's an open environment and one with an enormous amount of collaboration. Interestingly enough, I need to point out that the GPS division overall has historically been viewed as a place by the national community of one in which people will work together and in a way that both transcends traditional boundaries, and that transcending often leads to something very important.
We often see this–and I can speak to this in generic terms as a senior faculty member here–when we get commentaries back from other academics who write to us, either when evaluating someone we're going to hire, or maybe a person has come up for promotion, they'll see that there's been this change in the person's career. They were only a modeler, and they became very interested in the real world. This has actually happened repeatedly within geophysics. Don Helmberger is perhaps the greatest example in all of seismology. And I think the Seismo Lab is an embodiment of that, but an embodiment of that perhaps more focused on geophysics and clearly, way more communal in that regard. And in some sense, they say it's supposed to be a hallmark of Caltech. I think the Seismo Lab really stands up for these things.
As I've mentioned before in previous discussions, some people want to make things bigger and bigger, and certainly that's not the view of Caltech and the Seismo Lab. Clearly, there are big groups now around campus that become giganticized. But I view the Seismo Lab as something more on a human scale, in which people work with one another. I think that's the most important thing. I think as director, to me, that's key. And I hear repeatedly individuals who try to create some sort of communal environment. For example, over the decades, we'd have very prominent geophysicists–I won't mention any names–coming from very nice universities within the United States to go down for coffee. And sometimes they'd say, "How do you do this? I tried to have tea at our place, and it just fizzled and never worked." My feeling has been, that communality and sharing are important. To me, those are and have always been the most important things.
ZIERLER: One of the narratives I've come to appreciate about the Seismo Lab within Caltech and GPS is the push and pull factor regarding how much the Seismo Lab should be integrated more generally within GPS and where it should be–not an island, that's not the right word, but where it should retain some of its discreteness or cohesiveness that existed physically and metaphorically when it was up in the San Rafael Hills.
GURNIS: It's a tension. And I felt that as just a faculty member here, probably even as director. It used to be stronger.
ZIERLER: When you became director and felt this tension yourself, how have you navigated that? What has been the right balance where the Seismo Lab is its own organization, but seismology, in its modern form, of course, must be intellectually and academically integrated more broadly in geophysics and planetary science?
GURNIS: Right now, the geophysics faculty is just as integrated in the rest of the division as any other faculty members, but we have this extra communal aspect to it. I think the walls are not as sharp as they were when I first came here in the mid-1980s with Don Anderson. At some level, you sort of felt that there could be tension between Don and the rest of the division in the sense of, "This is geophysics, and we're going to do everything ourselves over here." But in some sense, part of it is that people feel like there are walls and things like that, but there really aren't. Here's a very interesting exchange I had. There was some external review of the division, and they were questioning me about geophysics and the Seismo Lab. One of the external reviewers said, "We were meeting with students, and they feel really excluded." It was almost like, "What's your problem? You're excluding people." To me, it was like, "No, we don't actually exclude anyone. It turns out we have all these things we do together, and it just happens we do them all together."
The fact is that you may be outside of the Seismo Lab, but it's not like we put a wall up so that you can't get in. Coffee used to always be open to anyone who would want to come from the division. But that doesn't mean people would come in a sustained way from the division because the topic of discussion would maybe not be what they were interested in. But the point is, if you're creating all of these things that work well, you have coffee, you're sharing students, you have seminars, maybe we do trips together, that doesn't mean that it's there to create a wall. It's to create what they tell us to do anyway, which is to create a dynamic environment where people work together. And that's what we've created. People work together. You can view it as there being a wall, but it wasn't us intentionally creating a wall. It's interesting, people from the division will walk over here and get free coffee. I've done that for 20 years.
When I first came here, you had to be part of what was called Coffee Club, but I decided that was kind of a waste of everyone's activity and just bought a coffee machine and a contract with a contractor. The coffee machine's there, and anyone can come over here. And this sounds very trivial, but it was really important for people, more so than I had thought, and it's temporarily on hold right now, but someone would get donuts once a week and put them in the coffee room. Very few people would bring those into the coffee room, where we would have our discussions, but they were there, and anyone could walk over here. You'd see people from the division walking over and getting their free donuts. The point was, we're just doing things, be they social activities, refreshments, things like that. The division does this, too, but none of the other groups do it. They may do it at a division level, offer things like that, or attempt to try to do things like that, but they often will fizzle out after a while.
Over the years, I've collaborated a lot with Paul Asimow, and he's not part of the Seismo Lab, he's in geochemistry, geology, and things like that. But we share projects and students. Jennifer Jackson, same thing. We have something here that's very helpful, but it doesn't cut us off from the rest of the world, and I think that's really good. It's just comfortable here. All those interactions people have throughout the division are much more–maybe you have a meeting every couple weeks or so, or you have a seminar. The thing is, everything is here. All the faculty and students are right up in this small hallway together, and we go downstairs for coffee. I had heard this many years ago, a story that probably goes back to when Don Anderson was the director, or maybe even before that. One of the complaints that people would have was that the administration never put forward a major campaign for endowment funds.
The argument that was told to me was that the provost had decided that because they didn't want to make the director more powerful than the division chairman. Since that time, of course, the division chairmen have become extremely powerful and have lots of resources. But by the same token, now, all center directors have endowment funds, including the Seismo Lab. Now that a model has been established throughout the Institute by which this happens–and now, the Seismo Lab is, in some sense, not unique. It may be the oldest and the one with the most cohesion to it, but there are other centers now within the division that are probably more or less permanent, like the Linde Center. And not to be contrasted with what the Seismo Lab had created back near the turn of the century at the Tectonics Observatory, which many of us had viewed as a project, even though we called it an observatory. It was a successful project that came and went. When the funding went–and two-thirds of that was the Seismo Lab anyway–it just vanished.
ZIERLER: I asked about the Seismo Lab within GPS. What about more broadly across the Institute, just how computation is a connecting point that brings together all kinds of different disciplines? Did you see opportunity, particularly in your interest and expertise in high-powered computing, to bring in, for example, partnerships with CMS?
GURNIS: The interesting thing about that is that it has evolved dramatically, so let's contrast two different things, the way things were in the 1990s and the things there are in this half decade right now. Back then, in the 90s, there were several groups on campus, several of us within geophysics, developing our own software, and we needed our own hardware, even though it wasn't determined where it was going to be. There was a clear desire to continue to develop our own actual hardware within the Seismo Lab or more broadly, within the Institute. I was part of this group in the 90s called CACR, the Center for Advanced Computer Research. Eventually, the Hypercube project, which was the evolution of the parallel computing project–after the parallel computer was invented, we created CACR a couple years before I joined the faculty, then I was part of the group after I joined.
One of the big desires of that group, of course, was to get a big computer. And as I mentioned in past discussions, there was a real desire to get this big iron, as we called them at the time, which is a word that hasn't been used for decades. At the time, a single university could get such a large computer. Caltech had several of them, as I spoke about. There was a clear desire, especially among Jeroen Tromp and myself, to do that. In the end, we did it within the Seismo Lab. We talked in great detail about that. And then, there were other groups on campus that wanted to do that as well. But the way it evolved at a national level, it was no longer an interest of Caltech to do that because it became more and more of a service activity for those supercomputer centers, and it wasn't really clear the extent to which there would be a dramatic advantage to the host university. I do think there are advantages to it, and several universities went that route.
The University of Texas at Austin, up until a couple years ago, the University of Illinois at Urbana-Champaign. They hosted this big iron, and it did give their faculties extra access to this. But now, it's less and less the case. Although, some universities continue to compete in that. Now, for me, that's all commodity in the sense that myself and other people within the area of high-performance computing compete within that arena. In some sense, the peak of that had happened probably in the 90s, and then the way technology and the funding of science evolved, that became less of a focal point around campus, I would say. Okay, then things evolved. Suddenly, 2014 or 2015 to the present day came around, and the research done in CMS and some of the faculty members there, and suddenly, especially for the work of Zach Ross, it became clear that the software and the algorithms were absolutely key in machine learning. And he was at the cutting edge of that.
That's very, very important. I'm not actually participating in that at all, and I don't think, outside of Zach, others are actually involved with that kind of interdisciplinary research. Now, as you probably see all over the Institute, one of the things we see is the application of machine learning to anyone who has big data. But what I see widely deployed are the same tools that have been broadly developed now reapplied to a new area of science. Graduate students and undergraduates with training at Caltech or other nice universities who have a nice training in this at the undergraduate if they're getting a computer science degree, can take these algorithms and rapidly apply them to any area of big science.
It's interesting, I think there are these synergies that exist amongst a few faculty in CMS and around the Institute, and Zach is one of those focal points within the Seismo Lab. But it's become so widely applied. Are there going to be other areas of seismology and the Seismo Lab that will actually take advantage of that? Almost certainly. The work of Zhongwen Zhan will almost certainly require algorithms and ability to deal with big data beyond anything we can currently work with now because of all the work he does with fiber optics. The rate-limiting factor there is probably going to be algorithms going forward. I suppose that a lot of the Seismo Lab students are probably going to need to pick up on this if that continues to evolve in the way it's evolving.
ZIERLER: As you emphasized, when you became director, you did not want to sort of impute your own research interests on what the Seismo Lab should be doing.
GURNIS: Yeah, but there's a reason for that. That is because my own personal research, either being in the computational science or the stuff that really sets me apart from my colleagues, which is the interface between geology and computational science, are so different than what most people do within the Seismo Lab that I wasn't going to bring the Seismo Lab in that direction. I'm not that arrogant to believe that that's its purpose. There are bigger purposes of scientific organization than promoting one's own work. It's come at a huge expense, I think, to my own research, unfortunately. But that's okay.
ZIERLER: Of course, that's exactly the right leadership approach, to not impose anything based on your own research interests. But to flip that question around, what about going the other way? When you became director of the Seismo Lab, and in the years since, did that give you a broader-angle vantage point, where you were seeing more things that were happening, and those things might've influenced your own research?
GURNIS: I was already seeing things broadly, so no. Maybe if I had been younger, it would have, but not at my age, it didn't. And I could've done things differently. One thing I consciously did at the time, and to be honest with you, I've thought maybe this was not the right way to do things, I was leading this national effort in my area of geodynamics initially called CIG, Computational Infrastructure for Geodynamics. And it became a model for software institutes within the NSF. But what it evolved into was really a software center for high-performance computing for geophysics for US university researchers. That was what I evolved it into. Even though it was named for geodynamics, it actually was bigger than that. And I had the option to fold it all into the Seismo Lab. Probably, most people would've done that. But I viewed that as counterproductive. I thought the national center was really for the national community. Why should I dominate that?
To me, it seemed arrogant and not the right direction. And actually, there was another reason. I saw that my own community was going in one direction, and I wanted to be free of that and go in a different direction. And we already spoke about that. I wanted to take my own area of geodynamics into the direction of inverse problems, whereas the community was still steadfastly into the forward physics problems, and it remains that way, even though we've done all this work and cleaved it off. But that's for another younger scientist to achieve. Maybe we were ahead of our time. But I could've done this, and that would've been the normal thing. And there would've been several advantages to that. There was lots of NSF funding, we clearly would've been a focal point of computational geophysics within the United States.
But there was also another non-Caltech element to it, which was the service part. I think the service part is very, very important. But then I thought, "Will it bog us down?" I decided to give it up and let the community take it from there. Then, of course, I eventually took on the same role, but more from an administrative perspective than an intellectual perspective, of trying to develop a way to broadly develop software across all areas of science over Caltech, and that's the Software Academy. It has a lot of overlap with what we'd done two decades earlier for geophysics. That became sort of necessary for science more broadly. Again, it has a very different flavor to it.
ZIERLER: With only so many hours in the day and all of the new administrative responsibilities you took on over the past 12 years, did that force a certain narrowing or prioritization in your research agenda to figure out, "What's most important to me, given that I only have so much time to work on the things I can do in science"?
GURNIS: It came at a huge expense, and unfortunately, sometimes things don't unfold in the most optimal way because several other things unfolded concurrently that were very successful for my own research, yet caused even more dilution of activities. It's something that goes through my mind all the time now, but didn't so much a decade ago. It was more that I'd just take on things and keep charging forward, and some things naturally fell by the wayside. Obviously, with students, I tended to focus more on some things than others. For me–and this ended up being a great, great success, but it more affected myself as an individual scientist and not as a leader of my own group–there was this divergence of activities off into marine work, going on oceanographic expeditions, all the work I had done with the Ocean Drilling Program.
That came about because there was a natural kind of evolution that I was pushing my science to very slowly over the previous years, going all the way back to the early part of the century, where within several different veins, we had brought the science of geodynamics to the point where specifically trying to understand the underpinnings of plate tectonics, there were some big questions I wanted to address and that no one else was addressing from an observational perspective. I'd taken the models to the point where they needed to be tested, and I didn't see other people doing that. I needed to step forward within that whole community of initially ocean drilling to test my ideas. The big thing I got off on in terms of the research direction was the whole question of how to make a new subduction zone.
That sounds like a particular thing, but in terms of the dynamics of the Earth, this was so important because this process of subduction creates most natural hazards, creates most geology on the planet, and it also is the key place, in terms of the whole energetics of the planet as a dynamic system, on the planet where you have the tectonic plates returning to the Earth's interior. From the physics and energetics perspectives, all the activity happens, in some sense, in one spot in terms of the forces which drive the system and the dissipation in the system. Then, in that process, there was always this outstanding question, "How did it start?" People were very interested in how this started over in Earth history because it didn't exist several billion years ago, and that's a focus of enormous discussion within the scientific community. "When did subduction first begin?"
Yet, no one knew the answer, even as the Earth continues to evolve, to, "Why do new subduction zones form today?" If you go back to when I first started to get interested in this question off and on at the turn of the century, when we wrote our first paper on this, no one else was working on it. But we quickly realized there weren't the necessary observations to test the models. It required going out not in geophysics, but in terms of geology, to collect the data that was required. With other people in the community, and sometimes by myself, I had to go compete with trying to articulate the need for these particular drilling expeditions.
And they were in the pot for a while. Then, all of a sudden, stuff hit the fan within a short period of time in 2010 or so, and a lot of things got approved all at the same time. I don't know if you've found this or other people have told you this, but sometimes I can, within certain activities, have a perfect sequence of events, but sometimes I can't connect it to what the date actually was. I want to give you some specific dates here. Stuff really hit the fan in 2014. In the Western Pacific, there are these two gigantic subduction zones. One is south of Japan. Most people think about it as the Mariana Subduction Zone. There's this place in the western Pacific called the Challenger Deep, which is the deepest point on the surface of the Earth, and that's connected to this subduction zone. Then, there's one north of New Zealand called the Tonga-Kermadec Subduction Zone. They both formed 50 million years ago.
We sort of generally knew that, but we didn't know how they formed. There was so much missing geology we needed to do. And it's very expensive to mount one of these expeditions. I got involved with a big project called Project IBM, tongue in cheek. Izu-Bonin-Mariana Subduction Zone. It's the whole plate margin that goes from just south of Tokyo all the way down to Guam. It formed geologically 50 million years ago. But I became part of this bigger project probably 2005, 2008, I don't know exactly when. It was called Project IBM, and we were going to have four drilling expeditions to try to understand this. And it didn't go anywhere for a while. Then, all of a sudden, I took one of those expeditions and was able to specifically turn it around to test an idea I had on subduction initiation.
As soon as I did that, that proposal flew through the system. And it dragged everything else along behind it. That was my view of it. But I was on the panels, and I could see that things had broken forward. And I had never done any of this kind of stuff. I had pieced together stories from the Ocean Drilling Program ever since I was post-doc here in the 80s, when I would get all these books and get all this information about the geological history of the Pacific. Now, all of a sudden, I was one of the proponents, not the chief scientist or anything like that. But then, almost as this thing got approved, I was working with another very close colleague to study the one south of New Zealand, and that got approved. Then, I started to go out to sea. I spent six months at sea over a period of two and a half years on this drill ship, drilling south of Japan. For some of my other ideas, we drilled on the northwest shelf of Australia, then we drilled north of New Zealand. It turned out that all of these expeditions were extraordinarily successful, and it helped.
And now, it's like everyone and their mother's uncle is working on this subduction initiation business. I've got to get out of it and do something else. But in any event, I only brought this up to talk about having a limited amount of time. As you get older and have more responsibilities, you just can't do as much. You've got to figure out, if you're going to do anything before you leave, how you're going to do that. All of the research went off in this direction. And the crazy thing is, when I was proposing all of this around 2006 to 2008–the first drilling was in 2014, but the interesting thing is, several years before that–and it also developed this other collaboration, we'd talked about this in high-performance computing, having to do with these super high-resolution forward models to understand the dynamics of plate tectonics.
That research also unfolded. And during the course of this, things just happened. As an individual scientist, my research through collaborations has gotten more and more dirty in the sense of closer to the geology than I'd ever gotten before on the one hand. Then, on the other hand, with other collaborators in a different universe, this brain versus that brain, I had gone off in this other direction on trying to work with mathematicians developing new algorithms. Before, we would just invent our own algorithms or read something in a book that an applied mathematician had written years before and apply it to our codes. Now, we were collaborating directly, especially with my close colleague, Georg Stadler, who's at the Courant Institute. The research got down and dirty in geology for part of the problem, and it got really mathematical on the other side. Which is probably the stupidest thing I've ever done in my life, to go off in these different directions.
But there is method to the madness. For all of those things, they still come down to how plate tectonics works and trying to figure out how to either create the observations or how to create the computational underpinning to sort of solve the problem. It's still all to try to solve the same problem, but from entirely different directions. And of course, these are all with lots of people. Still, a number of students over the years, the student and I would write a paper, and there were many papers like that. But there were these broader, bigger teams. There was a time in there with the NSF where you had all these conflicts of interest. They wanted to know who you collaborated on a project with or wrote a paper with. There was a time in there that I had people on there from–the number of scientists on those drilling expeditions is 30. Then, over three years, it's 90. Now, you supply this as an Excel spreadsheet to NSF. But it got so out of control for a while, with all the people out there I was interacting with. But at the same time, we had a big team trying to go forward on the computational science side of things.
ZIERLER: In previous conversations, you've conveyed your enthusiasm for what Zach and Zhongwen are doing as some of the most impressive work happening in seismology anywhere.
GURNIS: Totally disconnected to me, but it's just wonderful work.
ZIERLER: And you're so proud of the fact that they're at the Seismo Lab. My question there is, how much of that pride and excitement is about how scholars like Zach and Zhongwen could be at a Harvard or at an MIT, but they're here? And how much of that is because they're just so representative of what the Seismo Lab has always been, which is at the cutting edge? I wonder if you can disentangle that a little bit for me.
GURNIS: That's a very good question. We spend a lot of time trying to figure out who to hire, and sometimes you just see that there's a great fit within individuals. And it's a several-stage process. This is how it's worked the whole time for me, although maybe it'll evolve in a different direction in the future. The group will identify several good options. There are several aspects to that. One is that they're a very good scientist, very creative, they have the ability to develop the program. Then, there's another aspect that's really played a very important role, I think probably in the division, but especially in the Seismo Lab. "Is that person going to be a good fit?" You don't explicitly ask that question, but you sort of feel it, and it drives you forward. "That's the person I want." You just feel that they'd be a good person to have here.
The other reason they've been so successful in the Seismo Lab tradition and what makes it work so well is, they sort of…Zhongwen grew up here. He got his PhD here, spent two years away, then came back as an assistant professor. Scripps didn't destroy him. Scripps is a wonderful place, and I have great respect for all my colleagues there. I know the ones in geophysics especially and have great respect for them. That was a tongue-in-cheek joke. But the point is, he wasn't away enough to know that he just wants to build his own research group and create an empire. He wants to have a research program and lots of students, but he's quite willing to work within the soup. Zach was here for two or three years as so a post-doc before we hired him, but he did a mountain of stuff before he was appointed to the regular faculty. But it was such a natural fit. Zach was doing totally different things than I was, but I'd be talking to him all the time about what he was working on when he was a post-doc.
I just sort of felt he was the kind of person we wanted here, and I think the other faculty did as well. But that's an argument that won't work very well without the whole division or Caltech. They're going to look at the papers, they're going to read the letters the international community will write about these people, even when they're young. But it's just identifying the right people. Jennifer Jackson has fit into the Seismo Lab and been one of the most wonderful colleagues I've had in my whole career. And she's in an area of mineral physics. We've wanted to hire a person in mineral physics for ages. And we weren't even really searching anymore by that point, but we knew it was somewhere–and we'd bring in all these brilliant people, but no one could communicate with them at some level, so they didn't seem like good fits. Then, one day, Jennifer Jackson walked through the door, and she gave a seminar. One of the things we had already identified was that she was at the absolute cutting edge of using these advanced photon sources to do this very high-resolution Mössbauer spectroscopy to understand the vibrational state of the atoms under high pressures and temperatures.
She was absolutely doing the thing. Yet, when she walked in, she just immediately was excited by the fact that everyone was talking to each other and were very interested in, "What is the meaning of your stuff for what we're doing?" That was it. We hired her immediately. She had already signed up to go to some other university, and she wasn't even coming here for an interview. But we figured it out, and we got her here. That's the way it is. We take a long time to find people, and when they're a good fit, you sort of know it, and it works out really well. If you go back 50 years, the Seismo Lab probably had higher turnover than other parts of the division. They would bring a lot of people in who would leave. I don't know why they left. A lot of these people were before me. Although, people have left while I've been here, and I understand the reasons. But generally, they had nothing to do with Caltech or the intellectual environment we offer people. Essentially, everything had to do with other things because we're all human beings and have lives.
The Centrality of the Sedimentary Record
ZIERLER: For the last part of our talk, I want to ask two broadly conceived questions, one retrospective, one looking to the future. If you survey your career, both on the science side and the administrative side, where do you think you've moved the needle the most? Where do you think you've had the greatest impact both on geophysics and the community of seismology in supporting your colleagues to always improve the field?
GURNIS: I think I have two answers to that because I think those are two different questions, what I did personally as a scientist. I think the most important stuff I did in terms of changing science was this whole bringing together of the dynamics of the planet with the sedimentary record. I think ultimately, the whole field changed the course of the connection between sea level, and sediments, and the kinds of things we can see and perceive that the continents and other plates have done in terms of vertical motions. Before plate tectonics, you go all the way back maybe from the early 1960s all the way back to the mid-19th century, geology was about vertical tectonics. Then, 1966 to 1969, plate tectonics was discovered, and it all became about horizontal. Everyone worked on horizontal tectonics.
But what geodynamics was able to do, and I think my work was quite pioneering in this direction, was to bring these two views of the Earth together and resolve the big questions of long-term sea-level change and how the dynamics of the interior of the plate relate to the geological record. I think now, it's a whole gigantic field. A lot of people have done duplicative things out the wazoo in the decades since. But I think that's the biggest change I contributed scientifically. Probably by the time I was an associate professor or early years as a full professor, that was mostly done. I think it mostly has to be cleaned up at this point. That's why I don't work on it particularly anymore. In terms of changing the course of geophysics and seismology, I think what I tried to do, along with Don Helmberger, was to bring a physics-orientated view of processes for the Earth together with the seismic wave field.
I think we didn't really bring all of geophysics with us. I don't think that really happened. People continued to do what they always did. But we tried to do it, and I think that was the right thing to do. And I'm trying to do several other things now. For my own research, scientifically, the big thing I want to do is to bring the whole cycle of great earthquakes together with the actual motion of the plates. It's a big area and a hot topic now, but it's all viewed in one direction. It's all about the big earthquakes. It says, "Plates move like this. It's going to drive the great earthquakes like that."
I actually view that these are way more coupled together in terms of the physics and doing the physics properly, and that's what I'm trying to do with Nadia Lapusta. We have students here, and we're assembling a team to do this big thing. On the computational side of things, it's beyond anything we've ever done before, so it'll be a big team Nadia and I will be involved in, in terms of computational scientists and applied mathematicians. That's one of the big things. If I can do that before I can't do anything anymore scientifically, that's what I want to do. But I have to get rid of a lot of things. No more director of this or that. I'll have to carve off a lot of other things to be able to focus on that.
ZIERLER: Something to look forward to. In the previous conversation, you made this very interesting point that the work you're doing with Nadia right now, you might get laughed out of the room, but you don't care. I wonder if you can contextualize that with the Seismo Lab's long history of forward-looking science that the rest of the field had to catch up to, so that you were no longer getting laughed out of the room. I wonder how you might see that in a historical perspective.
GURNIS: I don't know where things are going there. Maybe nothing will come of it. That's a very dangerous direction to think about. You never know if anything's transformative until after the fact. NSF asks us to propose transformative research. Everyone thinks their research is transformative. That's why they do it. But you never really know that until after the fact, when you can clearly see, "Ah, that was transformative." But it's all in hindsight, never in prediction. I think that's too dangerous, as a scientist, to think about. I have what I'm trying to do right now, and I'm hoping to go forward with that in the future.
But scientists, in this case, geophysicists, working together with data, models, and theory, I think that's a big mashup. I think it remains the core of the future of geophysics, this blend of theory, observation, computation, and having people work with one another, whatever the problem is. If it's for the dynamics of the whole planet, how an individual earthquake works, or how other geophysical processes work on the Earth or other planets, I think that's the direction.
ZIERLER: Mike, this has been a terrific series of conversations. I'm so glad we were able to do this. Thank you so much.
GURNIS: You're very welcome.
[END]
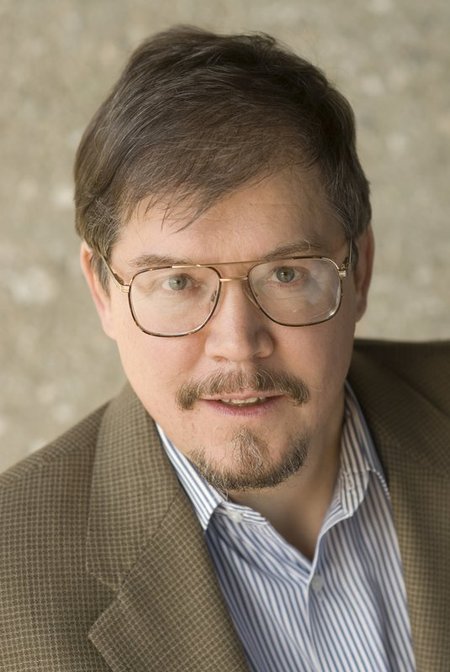
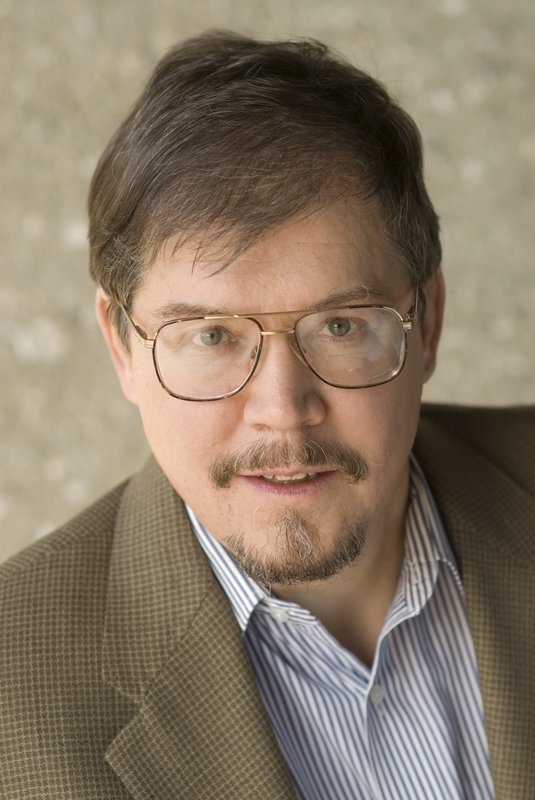
Interview Highlights
- Where Geophysics and Software Engineering Meet
- From Mansion to Campus
- The Universal Benefit of Data Sharing
- Straddling Observation and Theory
- Developing Interests in Seismology
- Debates Over the Interior of the Earth
- The Impact of Parallel Computing
- Old and New Questions on the Origins of Rocks
- From Michigan to Caltech
- Building a New Era in Computational Geophysics
- Becoming Director of the Seismo Lab
- Building on a Tradition of Earthquake Early Warning
- Unresolved Questions in Plate Tectonics
- Emulating Greatness in Geophysics
- The Centrality of the Sedimentary Record