Nai-Chang Yeh, Condensed Matter Experimentalist, Quantum Physicist, and Nanoscientist
There is a quip that circulates among connoisseurs of the history of physics, that if you could go back in time and ask Enrico Fermi if he was an experimentalist or a theorist, he would be puzzled by the question, because those distinctions would have meant very little to him. One of the great meta-narratives of physics since the end of World War II shows that the discipline has become so complex, that the experiments are so massive and the theories so precise and nuanced, that the binary of theorizing and experimenting have become sealed into career paths, whereby aspiring physicists must choose to become one or the other. There is no doubt that this bifurcation has served physics well over the past sixty years; it is difficult to consider, for example, how the creation of the Standard Model, the discovery of the Higgs boson, or the detection of gravitational waves would have been possible without the sustained and vigorous interplay of theorists and experimentalists. But does this historical trajectory leave room for a research purview that revives Fermi's approach?
In the lab of Nai-Chang Yeh, we have a resounding answer in the affirmative. Trained in condensed matter physics as an experimentalist, Yeh leads a research group that tackles a vast array of questions in high-temperature superconductivity, material studies of graphene, topological insulators, optoelectronics, and nanotechnologies, among others. It is an experimental environment that pursues physics in the vein of basic science and in application in the service of societal impact. But as Yeh explains in the discussions below, wherever possible, she serves as her own theorist, testing ideas against experiment, and vice-versa, simply because she is able, it is efficient, it benefits the research, and, one detects, because she enjoys it.
Yeh's dual talents in the physicality of the lab environment and in the ideas of theory trace to Taiwan, where she excelled in her primary education and in college at National Taiwan University. At MIT, she studied with the legendary Millie Dresselhaus, the so-called "Carbon Queen" who pioneered the blending of electrical engineering, materials science, and physics to make discoveries on a range of exotic materials, particularly graphene. As a leading woman scientist, Dresselhaus taught Yeh how to focus on the research and not get discouraged and distracted by the many structural and interpersonal challenges that can arise in a male-dominated world. Following her thesis focus on the novel properties of graphite intercalation compounds, Yeh joined the IBM Watson Research Center as a postdoctoral scholar, where she witnessed and contributed to the many exciting developments in high-temperature superconductivity in the late 1980s.
Reflecting on her decision to join the faculty at Caltech, where she was encouraged by the Institute's legacy of supporting junior faculty and a research culture that encouraged wide-ranging collaboration and a spirt of pushing research boundaries always in the pursuit of discovery, Yeh spent little time thinking about "firsts." But the firsts were significant, and doubly so: she was the first woman to achieve tenure in physics at Caltech and the first woman of Asian descent to achieve tenure across the Institute. Yeh's interest in these firsts centers exclusively around whatever encouragement it can confer to students coming from under-represented backgrounds, and she continues to draw on Dresselhaus's advice always to remain focused on the research.
In recent years, Yeh's research has increasingly incorporated applied science into her overall fundamental research purview. Her interests offer promising advances for nanotechnologies, microscopy, energy efficiency and storage, and in supplying materials in support of the profound engineering challenge to make a scalable and useful quantum computer. Looking to the future, Yeh is able to build out a research agenda a few years in advance, based on her students' interests and what is possible from the many funding agencies that support her work. But within and beyond this timeframe, Yeh is always prepared for surprises in science, and for the thrill of discovery that will take her lab in new and exciting directions.
Interview Transcript
DAVID ZIERLER: This is David Zierler, Director of the Caltech Heritage Project. It's Monday, June 27th, 2022. I'm very happy to be here with Professor Nai-Chang Yeh. Nai-Chang, it's great to be with you. Thank you so much for joining me today.
NAI-CHANG YEH: Thank you for having me. [laugh]
ZIERLER: Nai-Chang, to start, would you please tell me your title and affiliations here at Caltech?
YEH: I'm currently the Thomas W. Hogan Professor of Physics. I'm a condensed matter experimentalist, and I worked on things related to mostly quantum materials, nanoscience, and nanotechnology.
ZIERLER: Nai-Chang, who is or was Thomas W. Hogan, and is there any connection to your research?
YEH: I was just recently given this chair professorship, so I'm yet to know [laugh] Thomas Hogan a little better later. I think I'll be provided some information later. I don't know if it's the person or the family endowed this chair professorship to Caltech. I was nominated by my division chair, and this was granted jointly by the president and provost of Caltech.
The Matter of Quantum Information
ZIERLER: Nai-Chang, what about IQIM, the Institute for Quantum Information and Matter? Do you still retain an affiliation with them?
YEH: Yes, I'm part of it. I'm part of the IQIM.
ZIERLER: Tell me about some of the things happening in condensed matter experimentation that are really exciting and relevant for what's going on in quantum information, and the quest to build a quantum computer.
YEH: Institute for Quantum Information and Matter [laugh] is the real name, the full name of IQIM. This last word "matter" is where I come in because, as you know, when we build quantum computers or deal with quantum information, we cannot simply do it in a vacuum. We must have real materials to make the devices, build the circuits and put everything together. We need hardwares. Hardwares are anchored materials, and therefore we cannot do away with materials and build things in air. [laugh] That's where this relevance is. I studied quantum materials, and quantum materials are not just for quantum information and technology; or just for quantum computation. Quantum materials are already directly relevant to our everyday life in all the modern technologies, from computer chips [laugh] all the way to our cell phones, internet of things, to almost everything of modern convenience that we do. Many of the electronics, photonics and optoelectronics for sensors and detectors used in our everyday life are built on quantum mechanical principles and built on materials.
Quantum materials are particularly important to further advances of these modern technologies that are not just for quantum information and computation. Quantum materials consist of a very broad range of materials that exhibit very interesting high-level quantum phenomena that can further enhance modern science and technology, although it gets pretty technical if I want to elaborate further. On the other hand, at the Caltech Science Exchange website, I was interviewed for Caltech Science Exchange about quantum materials, and so you may look up the written documents and videotaped information for more details about quantum materials. In any case, quantum materials are crucially important if we want to further advance many fronts of technologies, including quantum computation. Currently, in the development of quantum computers, the most advanced and mature technologies are based on low-temperature superconductors. Low-temperature superconductors (such as aluminum and niobium) are used to make quantum bits, also known as qubits, and to build circuits to support the operation of qubits. These systems are not very scalable because they must be cooled to very low temperatures using dilution refrigerators, and the quality of the qubits and circuits is not perfectly reliable due to materials issues. We can build a few tens to a few hundreds of qubits, but that's far from millions of qubits that we would like to have for a fully advanced quantum computer. The bottleneck is really in the material that's being used. Further advances in materials, particularly in quantum materials, to achieve improved functionalities, reliabilities, and scalabilities, will be very important. [laugh] Anyway, that was a long answer to your short question. [laugh]
ZIERLER: That's great. Nai-Chang, many more questions on quantum materials. But just to stay on the topic of IQIM for a second, going back 20 or 25 years when quantum information was purely a theoretical concept—these were things that people like Alexei Kitaev and John Preskill were thinking about in a theoretical realm—by the time you got involved, what does that tell us about the maturity of the field of quantum information that we can now start to think about experimenting and matter and actually getting closer to a physical device, a scalable quantum computer?
YEH: Let me take it back a little bit. What Alexei Kitaev proposed at the time was based on a different kind of approaches of making qubits. Those are based on topological quantum computation concepts. They're thinking about using something called anyons, which are some complex quasiparticles in materials, meaning that they're not true particles. They are particles dressed in many-body interactions, so are very complicated entities in two-dimensional systems under extremely high magnetic fields and at very, very low temperatures. These quasiparticles in two dimensions can exhibit topological properties. Topological properties are usually less susceptible to environmental perturbations, and so could better retain their coherence and, ideally, better for quantum computation. However, that paradigm is never realized experimentally. That the particular paradigm of topological quantum computation based on anyons has not been realized is not entirely surprising because if a system is very insensitive to environmental perturbations, it would also mean that the system is very hard to be detected and manipulated. [laugh] It's a double-edged sword, in some sense. In fact, Microsoft had invested a substantial amount of funding in experimental studies of anyon physics to see if the beautiful idea of anyon-based topological quantum computation can be realized. But it's still not realized over more than a decade of research. To date, there has not been successful demonstration of topological qubits based on anyons in two dimensional systems.
On the other hand, if we have been able to make qubits, regardless of the mechanisms we choose to make the qubits, many important tasks for quantum information technology can be developed, like preserving quantum coherence, implementing quantum entanglement, propagating quantum information, and quantum error corrections. These tasks can be developed with proper engineering concepts as long as qubits can be made. People have tried many different mechanisms to make qubits, although the most mature technology to date is based on superconducting qubits using low-temperature superconducting materials that have to be cooled to very, very low temperatures using dilution refrigerators. Also, these materials are quite sensitive to the processing procedures so that uncontrollable impurities affecting the quantum coherence could happen. But, still, low-temperature superconducting qubits are the most advanced mature technology at this moment. There are many other types of qubits. For instance, people try to use laser-cooled atoms or laser-cooled ions, or other solid-state systems such as magnetic spin qubitsand quantum dots. There are many ways of making qubits but none of those other qubits are nearly as mature and scalable as the low-temperature superconducting qubits right now. But, as I said, low-temperature superconducting qubits have their own problems because it will be very difficult to connect and operate a large number of qubits that are housed in many bulky dilution refrigerators. On the other hand, superconducting qubits have improved and advanced quite substantially so that people have been able to make tens to hundreds of qubits to do some prototype demonstrations of quantum computation, even though it is still nowhere near a full-scope quantum computer that's desired. The need of large-scale dilution refrigeration and materials related issues are the big bottleneck for quantum computation based on low-temperature superconducting qubits, although this approach has made a lot of progress. There has also been a lot of progress made in other areas of quantum information technology. For instance, people are working on quantum memories, quantum networking, quantum information transfer, quantum cryptography, and quantum error corrections. These areas can be developed provided that you can achieve entangled signals from different qubits and then consider how to transmit the signals from point A to point B while preserving the fidelity of the information over long distance. Those prototype technologies have been demonstrated, which are all important experimental advances in addition to a lot of theoretical advances, although they're still far from how we truly want to achieve in the end.
ZIERLER: On that point, as you well know, there are many debates in the field, not only about timescales but utility, when it comes to quantum computation. From your unique perspective and your areas of expertise, what feelings do you have about when a scalable quantum computer will be achieved, and then, once it is, what will it be good for?
YEH: Now, I don't have a crystal ball to predict [laugh] how soon quantum computers can be truly realized, in the sense of making millions of qubits, and operate them together. But I'm seeing good progress, and I'm hoping that maybe within my lifetime [laugh] so that I can see it. But I cannot quite predict. As I mentioned, I think the biggest bottleneck right now is really the materials. The material that's being used to make qubits is not the easiest nor the most scalable, most reliable system. If there could be a breakthrough in the materials that can be used for qubits, then I think there will be a huge quantum leap of development. I cannot quite say exactly what will happen. On the other hand, you can do a brute-force approach, putting a huge amount of money to build lots and lots of incredible cryostats [laugh], connecting maybe hundreds of qubits and their circuits at a time in one cryostat, linking together many such cryostats each containing many hundreds of qubits and circuits [laugh], and then trying to control and operate all these components in this monstrous system that way. If you do a brute-force approach—it depends on how much money you can invest—it might be doable, but it won't be widely usable for everyday life. It might just be sitting at the Defense Department in Pentagon [laugh] or somewhere with extremely high security, etc. The reason why people are truly pushing for quantum computation is that you really will gain this incredible amount of computational power, far more than the classical computation approach, with the implementation of quantum computation. In addition to incredible computational power, we can have excellent ways of preserving high-security signals information. It is well recognized that any nation gaining an upper hand in quantum computation will have an incredible power [laugh] and incredible technological advantages over other nations without the capabilities of quantum computation.
That's why, although it's extremely difficult to do, the whole world is still—whoever has some money, trying to invest in it, knowing that it's potentially extremely important for the future.
We all know modern semiconducting technology is making nanoelectronic components in the chips smaller and smaller in order to pack in as many devices as possible in a tiny chip to achieve very fast computation and speed in performing all kinds of tasks. Computational power is a necessity these days in modern world, and that's why people are pushing for superior computational power. However, modern chips are based on classical, deterministic bits, and so there are limitations. Suppose that we can overcome all technical issues to pack nanoelectronic components in such high densities that are beyond Moore's law, there are still physical limitations of what classical computation can do. Also, if we pack too many devices in a tiny volume, the energy consumption will be enormous, which will lead to many, many technical issues.
The need for superior computational power is why there's a race towards advancing semiconducting technology in the world right now in addition to the race for quantum computation. The US is kind of lagging behind in the technology of manufacturing semiconducting chips, although Taiwan, an ally of US [laugh]—the Taiwan Semiconducting Manufacturing Company, the world's number one and well ahead of others in semiconducting technology, is cooperating with the US. It all boils down to the computational power [laugh] for everything. As an example, consider the ongoing war in Ukraine. All those high-tech weapons would be rendered absolutely useless without advanced chips. Now, imagine if we can implement quantum computation down to extremely tiny scales—although it's hard to picture the feasibility right now [laugh] because we're talking about huge cryogenic systems for storing these computational units, so we are far from the ideal situation of miniaturizing quantum computing capabilities. If the ideal situation can be realized someday, with the incredible computational power that quantum computers can provide, we will have many orders of magnitude better computational power than conventional digital computation. Then the impact will be immeasurable. You will need great imaginations [laugh] to think about what the new world will become. It will be incredible. That's why, even though it is hard, people are pushing for advancing quantum computation.
ZIERLER: Nai-Chang, as you emphasized, the real issue right now is building the necessary materials to make quantum computers. For you, beyond Caltech, who are some of your key partners or collaborators in academia, in government, in industry who are working to make these breakthroughs possible?
YEH: I know that in the US, multiple institutions are, of course, working on quantum information technology. Industrial companies like Microsoft, IBM, and Amazon are working on the technology. A lot of our competing institutes in the US are also working on it because people understand how important quantum computation, quantum information technology is. Now, in other countries, I would say that, of course, China [laugh] is making strong emphasis on quantum information technology. US has collaborations with Japan, Taiwan, probably also UK. Additionally, Germany and Canada also have strong emphasis on the research of quantum information technology. These are the countries that I know to be making significant efforts in developing quantum information technology. They're pursuing the research actively. On the other hand, current efforts worldwide are largely based on existing materials for qubits; there has not been sufficient emphasis on developing new materials for scalable and reliable qubits.
Nanoscience as Connecting Point
ZIERLER: Nai-Chang, I asked about your current titles and affiliations. A previous one I'd like to ask you about is when you served as co-director for the Fletcher Jones Foundation of the Kavli Nanoscience Institute. First, tell me about the Fletcher Jones Foundation, and the way they support nanoscience research.
YEH: The Fletcher Jones Foundation provided some endowments to the operation of the Kavli Nanoscience Institute, and also to this named chair professorship. That was the way they supported nanoscience because they believe that nanoscience is an important area for modern science and technology -- As you know, we talked about the importance of all the small-scale chips, etc, which are all based on nanotechnology. That was where the Fletcher Jones Foundation came in; they contributed to the endowment funds for the Kavli Nanoscience Institute. The Kavli Nanoscience Institute was primarily endowed by the Kavli Foundation, the Moore Foundation, and the Fletcher Jones Foundation, with additional private funds from other people like Dr. Chuck Wheatley. The endowment is important because it provides stable funding to sustain the advances of nanoscience and technology. The way how the Kavli Nanoscience Institute operates is that we primarily operate a suite of state-of-the-art instruments for nanoscience research on campus. The costs of these instruments add up to many tens of millions of dollars, which are not affordable by any single professor. With the endowment funds, we could purchase instruments and maintain them so that we don't have to always chase after money by writing many individual proposals to federal funding agencies for acquiring different instruments with a successful rate for each proposal below 10%. The endowed funds help us build a suite of cutting edge instruments quickly for research, which is essential and has been highly impactful for advancing the research of nanoscience and nanotechnology at Caltech.
In addition to purchasing and maintaining a suite of instruments, the Kavli Nanoscience Institute is also in charge of the operation of instruments and the training of the users. We charge campus and external users user fees to pay for the staff support because we have staff members in charge of maintaining the facilities, training students and users, and also to pay for the maintenance costs of the instruments. That's how the Kavli Nanoscience Institute operates. But, of course, we also have other programs funded by the endowment. In addition to supporting the instruments, we support talented young researchers, including undergraduate and graduate students, postdoctoral scholars, and junior faculty members by providing some funds for their research in nanoscience. The support for junior faculty members includes awards for research discretionary funds and seed funds for initial cleanroom usage fees. We also run seminars and social activities so that we bring together a coherent, synergetic nanoscience research community on campus. That's how we operate. Fletcher Jones Foundation is basically a part of this effort that contributes to the endowment that sustains the research and activities on campus on nanoscience.
ZIERLER: Nai-Chang, more broadly, how do you see nanoscience as part of your overall research agenda? Where does it fit in?
YEH: It fits in everywhere. For instance, a lot of the quantum materials that I work on are nanomaterials. It means that they have nanoscale dimensions like materials with only one atomic layer or a few atomic layers of thickness. That's definitely nano or below nano dimensions. Also, we make a lot of novel nanodevices based on these quantum materials, and so we need to use nanofabrication facilities to make these novel devices. We also study things down to atomic and nanoscale using characterization tools that are capable of probing such small dimensions, for instance, scanning tunneling microscopy, scanning electron microscopy, and atomic force microscopy. These are the tools, especially scanning tunneling microscopy, that go all the way down to atomic scale resolution. From materials to characterizations to devices for novel scientific and technological applications, it's nano everywhere. [laugh]
ZIERLER: Nai-Chang, I asked about affiliations and titles. I would now like to ask about nomenclature and terminology. Perhaps, at the most basic level, condensed matter experimentation, the phrase "condensed matter," first, a generational question. When you were a student, was it called condensed matter or was it still called solid state?
YEH: At the time, it was called solid state physics. Initially, the research field was called solid state physics. But then that was just not sufficiently descriptive of the entire field because when we dealt with materials that we studied, they were not only solid materials. For instance, some interesting liquids, such as quantum liquids, possess novel properties and are also condensed matter systems. A well-known example is liquid helium. Liquid helium, including helium-3 and helium-4, are very interesting quantum liquids with novel properties such as superfluidity at low temperatures, and many, quote, solid state physicists, unquote, [laugh] were studying those systems. Additionally, a lot of interesting phenomena in materials actually have the manifestations of—although they exist in solid state materials but the novel states that they manifest are effectively, sometimes, like liquids or gasses. I'm talking about the physical analogy of how the behavior of the collective interactions of the materials are like. For these reasons, people started thinking that just calling the research field "solid state physics" was not an accurate description of the field. That's why it's changed to "condensed matter physics" because then it can include solids, liquids, plasmas, or strongly interacting gasses, as long as we are dealing with dense and many-body interactions. Although I wasn't responsible for changing the name [laugh], I guess that the key reason, key driving force behind why the name got changed was to provide a more comprehensive description of the scope of the research field.
ZIERLER: If you might explain on a technical level so, for example, liquid helium, as a liquid, why would this fall under the category of condensed matter physics and not, say, soft matter or even rheology?
YEH: There's a big difference here because [laugh] you know the Planck constant?
ZIERLER: Yes.
YEH: H-bar? It's whether h-bar is relevant or not. [laugh]
ZIERLER: OK.
YEH: In liquid helium, there are quantum liquids below the superfluid transition temperatures. There, h-bar is highly relevant. If you have soft matters, h-bar is never showing up. [laugh] It's never going to show up.
ZIERLER: But why is that, Nai-Chang? If you could explain, why would it never show up?
YEH: Because you don't describe things in terms of quantum phenomena with quantized units of energy and momentum for classical behavior.
ZIERLER: I see.
YEH: You can describe a classical system with statistical physics or thermodynamics. The concept of quantum is irrelevant to those descriptions, whereas in liquid helium, you cannot escape [laugh] the concept of quantum phenomena, because the occurrence of superfluidity in liquid helium below the superfluid transition temperature is entirely the result of quantum interactions, even though it's a liquid. There are also electronic states in many solid state materials that behave like certain types of quote, liquid, unquote. I'll get to that in a second. That's why. But whether the system is quantum related or not makes a big difference.
For instance, how do we understand what a liquid is? We may think of it in terms of a more general description of the elastic properties of materials. For instance, let's first consider a solid, say, a crystalline material. We may shear it or compress it, and there are of course elastic moduli that resist the distortion to the material. But when we have a liquid, if we try to shear it, it just goes and doesn't resist the shear. That's one way of thinking about the difference between a solid and a liquid. There are collective phenomena in the electronic states of solid state systems that behave effectively like liquids, but accurate descriptions of these phenomena can get very technical. [laugh] I'm just making the analogy here to explain why we call these things "condensed matter" rather than solid state systems to include certain concepts and phenomena. You asked me why liquid helium is in the domain of condensed matter physics rather than soft matter. The reason is that the properties of liquid helium are dictated by quantum phenomena rather than classical behavior.
Crossing the Boundaries of Theory and Experiment
ZIERLER: Nai-Chang, as an experimentalist, what are some of the theories in physics that serve as an intellectual foundation for you that might provide guideposts to understand whether your experiments are working or not?
YEH: I'll be a little more cautious about claiming whether my experiments are working or not according to some theories, because physics is actually empirical science. As we progress in research, new experiments often will create new theory or will tell people which theories are wrong [laugh], and so it is not like we have some theories that would definitely dictate the outcome of the experiments and tell us whether the experiments were right or wrong. Having said that, theory and experiment actually go hand-in-hand. Any theory in physics must be proven empirically or observed empirically to be reality. Otherwise, it would just be a conjecture or, at best, math. For mathematics, you can make completely independent conjectures, and build on the conjectures [unrelated conversation] logical derivations of them, and then achieve some conclusions. That's perfectly good math. But whether the outcome of the conclusions has anything to do with what we observe in the real physical world is another story.
Therefore, the difference between math and physics is that physics must be built on empirical verifications. That's what I take as physics. I would say, to date, the physics that I have learned forms a good foundation for me to guide my intuitions, which are educated intuitions, of course, not random intuitions. The conceptual foundation for me include quantum field theory, relativity, condensed matter physics, and, of course, thermodynamics and statistical physics. These concepts form the physics foundation for me. A lot of these concepts are completely built on very well verified empirical phenomena, so I can trust the knowledge foundation that built on these physical concepts and principles. However, no theory is complete [laugh], and so very often, we are pursuing the frontiers by designing new experiments, making new materials, developing and observing new phenomenon that may not be explained by any existing theory. That's where we learn new things. That's where new theory gets created because of new understanding of new physical phenomena. Of course, the creation of new theory can feed back to developing new experiments, and so on. Throughout my research career, I have never stopped learning and studying new things. The new knowledge acquired then provides feedback for me to design new experiments and help me understand my experimental data. A critically important point to follow when considering experimental results is to ask: "Is the finding reproducible? Is it physically meaningful?" Even if we do not fully understand the underlying physics of the experimental observation, we must make sure that our experimental findings are correct because they're reproducible with many different independent experiments—not independent theory [laugh]. Independent experiments allow us to crosscheck the experimental findings by different approaches; if the same conclusion can be reached by independent experimental approaches, then even if there's no theory—and often that's where we feed in new information to theorists to figure out what happened— we can be sure that the new experimental findings are correct and imply new physics and/or new understanding. I would say that, yes, there is a theoretical foundation for me to judge whether my experimental findings are physically sensible, and so based on physical principles, we may conclude that certain observation could just be rubbish and that something must have gone wrong with the experiments.
But then there are situations when we keep seeing certain phenomena that cannot be understood nor expected, but they are perfectly reproducible and concluded by independent researchers using completely independent experimental techniques. Then there is likely new physics. We may go to theorists for help, or we figure out the theory on our own sometimes. That's what we do.
ZIERLER: Nai-Chang, as you alluded, in the history of physics, of course, there's always an interplay between theory and experiments. Sometimes, the theorists are ahead of the experimentalists and, sometimes, vice versa. Right now, in 2022, for all the fields that you're involved in, where do you see theory leading, and then where do you see experimentation leading?
YEH: In the area of quantum computation, I would say that theory is definitely leading because the experiments are so hard. But a beautiful example of something that is well known at Caltech is gravitational waves. The concept of gravitational waves was, of course, well led by the theorists a long time ago. When LIGO was first established, which I recalled was shortly after I arrived at Caltech although I don't remember exactly which year, I was very excited about the LIGO experimental effort, although I was not sure whether I would ever see the detection of gravitational waves in my lifetime. [laugh] But now the detection of gravitational waves has become reality, and it has become such popular experimental effort around the world that people in different places are building gravitational wave observatories. So, never say never. [laugh]
ZIERLER: [laugh]
YEH: It's an exciting example that theory initially led experiments in the case of gravitational waves, and then experimentalists worked hard to catch up, and to develop all these exciting new capabilities to detect the predicted phenomena. On the other hand, in terms of a lot of research activities in quantum materials, I would say that experiments are often leading theories in many aspects. A lot of things in the research of quantum materials are still not fully understood or easily predicted by theorists. Often new materials developments and new technologies go hand-in-hand to drive new breakthroughs, new foundations, and new physics that are not expected or predicted by theorists. But then, of course, new theory would encourage the developments of new technologies and new experiments to verify the theory as well. In science history, these occurrences go hand-in-hand. It's like the chicken and the egg. [laugh]
ZIERLER: Yes. Nai-Chang, if I could ask you to imagine or visualize all of your research as a pyramid, where the bottom was the most basic and general, and the top was the most specific, what would be at that base layer? I might guess that it would be strongly correlated electron systems, but you tell me.
YEH: You're asking the subject matter?
ZIERLER: Exactly, the most general, of all of the things that you do, what would be the category from which everything else fits into that, and then builds into evermore specific research areas?
YEH: I would say that it would be not just strongly correlated materials but also topological materials would be the foundation because some of the topological materials are not necessarily strongly correlated. But if you combine the two, strongly correlated topological materials will be extremely interesting also. I would say my foundation consists of correlated materials and topological materials. That combination would be the foundation.
ZIERLER: Topological materials would be big enough to contain correlated materials? In other words, correlated materials are a subset?
YEH: No. They're like two different sets with overlaps. That's why I cannot carve one out because both are important as the foundation of my research. If the foundation is the materials and the topics that I choose, then building on that, I'm interested in observing all kinds of quantum phenomena and different quantum degrees of freedom that come out of these novel materials, which would be like the middle part of the pyramid. I try to design experiments and investigate or even manipulate different quantum degrees of freedom. So why do I want to do that? It's fun. [laugh] For one thing, definitely, it's fun. But the ultimate outcome is that then I can create a completely new paradigm of quantum phenomena that are novel. A lot of things that I'm working on have not been explored before, and so they are exciting; the outcome is that I can sometimes explore extremely exciting new scientific frontiers. Whether they're useful or not, I don't even worry about. But then there are other outcomes from my research exploration that are also technologically relevant. So, I would say that the bottom of the pyramid is the materials that I investigate, the topics that I choose; and then the middle part is that the experiments and schemes that I design to realize, investigate and manipulate different quantum degrees of freedom. Ultimately, the pinnacle is the creation of novel quantum phenomena, basically, to engineer the physics that I want to see. [laugh]
ZIERLER: [laugh]
YEH: Then that can lead to both very interesting new science and also technologies.
Trend Toward Applications
ZIERLER: Nai-Chang, a question about your research motivations. What aspects of your research are geared purely toward basic science, understanding how nature works, and then what aspects of your research are motivated specifically, for example, like quantum computers, to applications or certain societal impacts?
YEH: I would say that maybe some years back, when I was much younger, I used to say that I was proud of being useless. [laugh]
ZIERLER: [laugh]
YEH: It was just my mentality that I got into physics simply because I love it. I love the purity of physics. I like to pursue the beauty and truth of the universe, and that's why I got into physics. That was my driving force. Things that I investigate must be fun in such a way that I feel like I can gain new understanding of the beauty of nature. Even though the new insight may be just a tiny component of nature, it would make me excited because suddenly, even for maybe just a microsecond, I felt that there was this resonance in me with the entire universe because I understood a tiny piece of it, or I figured something out, or I invented something that actually was proven true. This sense of resonance with the universe has always been the driving force for my research pursuit. I love to pursue those things that are novel, exciting, and can reveal the beauty of nature. This sentiment is still the driving force for my research.
But as I age [laugh], I begin to realize that we scientists also have societal obligations, to some degree. We get supported by the society, and a lot of things that we do actually can be extremely relevant to technological advances to make the world better and to make life easier for mankind. From this perspective, I have no objection anymore to doing things that could be useful. But I do not start doing research on certain topics because they are useful. I will always pick topics that excite me as good physics, good science, and exciting science. But then, because life is limited, we only have so much time. Therefore, if there are exciting scientific research topics that overlap with important technologies, and I happen to have the expertise and the funding in time to do the research, then I would do it. That's how it works. Sometimes, industry came to me for help, and I did have good collaborations with them, and have solved problems for them. But it wasn't because they came to me and then I had to accept it. I would not accept all the projects that industry came to me with. The projects must be extremely interesting scientifically for me to consider taking up the challenge. In fact, my students also love this approach, because they want to do science but they are also excited if their research outcome can have technological impact
ZIERLER: Nai-Chang, what would be an example of industry coming to you, and you agree to work with them simply because the science itself was so compelling?
YEH: There are various examples. But let me give you two examples. One example is that we, in our study of quantum materials, specifically two-dimensional quantum materials, one of the very famous quantum materials—graphene [laugh]—we were just trying to conduct scientific studies but then we happened to develop a very novel way of depositing graphene on all kinds of substrates without having to heat things up to high temperatures. There are many, many good reasons why this new graphene synthesis method is very useful for all kinds of scientific projects. This development caught industrial attention because graphene is such a good material that if we can deposit it on all kinds of important materials, we will be able to provide protection to the underlying material, provide excellent electrical, thermal and mechanical properties, and can functionalize materials in many ways. There are many promising applications that may be enabled by the synthesis of graphene on a variety of substrates. But other research groups could only synthesize graphene at very high temperatures, about 1000 degrees Celsius, which are incompatible with many industrial processes. Therefore, for graphene synthesized at very high temperatures to be useful for industrial applications, samples must be transferred subsequently to targeted substrates, which is not only costly and time consuming but also technically complicated and prone to contaminations and damages. In contrast, our approach can just grow graphene directly on all kinds of targeted substrates at much reduced temperatures. This new approach caught people's attention.
There were two examples of industrial collaborations. For instance, I have been collaborating with people in a very famous research institute, the Industrial Technology Research Institute (ITRI) in Taiwan, which was actually where Taiwan Semiconductor Manufacturing Company (TSMC) originated from. [laugh] The collaboration started when ITRI researchers came to me with challenging materials-related issues that were of technological relevance: They were developing flexible and transparent electronics that required coating a very thin layer of copper on polymer materials. Polymer [laugh], typical polymer materials cannot be heated up to high temperatures. Meanwhile, the copper layer deposited on polymer materials must be very thin to make it flexible and transparent. But a big problem with a very thin layer of copper is that copper surfaces eventually oxidize in air, and so the conductivity for these very thin copper layers that are used in transparent and flexible electronics always degrade with time. So ITRI researchers came to me and asked whether we may be able to grow graphene directly on their thin and tiny copper wires on polymer to provide protection to copper used in flexible electronics. This idea was something quite challenging to us at the time because we had not directly deposited graphene on substrates that involved polymer. To figure out how to synthesize graphene directly on substrates involving thin copper wires on polymer using plasma enhanced chemical vapor deposition techniques without damaging the substrates was a highly challenging scientific project, because there were many parameters that must be systematically fine-tuned to achieve successful outcome. To make the long story short, it was actually a very interesting scientific project, and we were successful in growing just two atomic layers of graphene directly on the tiny structures of copper on polymer, and they really improved the properties in an incredible way. For instance, not only that we improved the electrical conductivity of these tiny copper wires for flexible electronics after the deposition of two atomic layers of graphene, but also that the copper surface remained completely, beautifully intact at least up to four months when we stopped doing measurements because we need to publish. [laugh] This finding was in sharp contrast to similar thin copper wires on polymer without graphene protection, which exhibited degradation in electrical conductivity due to surface oxidation within a week. Another incredible benefit that we found was that the mechanical strength of the material was also improved significantly. Specifically, we collaborated with ITRI researchers to examine the performance of the flexible copper circuits after folding them many times. For copper circuits without graphene protection, we found that after about 20,000 times of folding, the circuits started failing. In contrast, the copper circuits covered with two atomic layers of graphene were able to sustain at least up to 200,000 times of folding when we stopped at [laugh] 200,000 times [laugh] of folding, which was a lot already. This successful collaboration with ITRI is a beautiful example of some scientific research in the lab actually can lead to quick impact on technology, which is exciting. A joint paper based on the research outcome has been published in a high-impact journal ACS Applied Materials and Interfaces, and a joint patent was also filed. (Note added: the joint patent filed together with ITRI has been granted in May 2023.)
ZIERLER: Nai-Chang, as you mentioned, earlier in your career, you were proud to conduct useless research.
YEH: [laugh]
ZIERLER: I wonder at what point, perhaps with some maturity or perspective of a life in science, did you come to appreciate that even research that might not have any apparent use does, in the long term, have tremendous societal impact. I'm thinking, of course, of NMR but there are myriad examples. When did you come to that realization?
YEH: I actually knew that long ago, because of an eminent example: transistors.
ZIERLER: Of course.
YEH: Taking transistors for example. It was just like a toy for researchers in the lab. We would—I mean, not "we" but people at Bell Labs at the time, they were just playing with it. They were not really thinking about real applications. But now we all know that the invention of transistors has truly revolutionized modern life, essentially everything, in everything we do. You cannot go without transistors [laugh] in all the chips and all the sensors, detectors from such simple things as consumer electronics to all the high-tech space and defense technologies—everywhere. I knew that all along. It was just that I just didn't want to have to worry about applications when I conducted research because that wasn't what I got into physics for. [laugh] But I knew all along that many research subjects —laser is another example, that started as purely curiosity driven, could eventually become extremely impactful technologically. I never ruled out the application aspects of research. But when I was young, I just wanted to stay pure [laugh], and not think about applications. Let us come back to another example, energy research. The quest for green energy, especially in areas of photovoltaics, fuel cells, and carbon sequestration, will require advances in nanoscience. The scientific underpinning mechanisms needed to make them work efficiently often involve nanoscience and quantum science. So, this is a clear example of how fundamental science such as light-matter interactions or chemical bonding processes down to atomic and molecular scales can directly impact important technologies that are highly relevant to life, and even to the well-being of [laugh] Earth.
ZIERLER: Nai-Chang, with all of the fantastic advances in computation over your career, from data analysis to simulation, what are some of the things happening in your research now that might not have been possible 20 or 30 years ago?
YEH: Actually, you see, the computational power is really very helpful, for instance, it enables machine learning and artificial intelligence (AI), where enormous computational power is needed. Computation can provide the capability of performing simulations and make predictions of experimental outcomes based on different physical parameters, which can be extremely helpful for optimizing the planning of experiments, particularly for those experiments involving very complicated, time-consuming and costly implementation. Additionally, using first-principle calculations and artificial intelligence, people have been able to consider all kinds of elements and materials and make predictions of material properties, such as what kind of materials could be topological or not, and what type of topological material a specific system may be. This is because not all materials are topological, and not all topological materials are the same. [laugh] People are making such predictions that would not have been possible decades ago when computational power was not nearly so enormous.
In my own research, computation has also been very helpful. For instance, sometimes we need to design certain nanostructures or nano-devices for specific experimental studies before carrying out the complicated nanofabrication steps. Before we spent much time and money to implement the hard processes, we wanted to know how to optimize various parameters to achieve the best experimental configurations for our research purposes. I could do such simulations in advance, and then designed the best parameters for experimental implementation accordingly. But, of course, simulations are simulations. Often, we have to make some simplifications and/or approximations so that our simulation results may not always capture everything in reality. Therefore, we would do simulations, conduct experiments with the input from simulations to verify the validity of approximations made, and then use experimental findings as feedback to improve simulations, and so on, which has become very useful. The research activities in LIGO are also another example of the importance of modern computational power: Computation was essential in helping the analysis of critical LIGO data to realize the observation of gravitational waves. There are many other examples in modern scientific research that computational power is indispensable. I also believe that the rapid advances in computational power will not only help better design and understanding of scientific research but also enable further improvements in hardware development.
ZIERLER: Nai-Chang, as you well know, sustainability is a major effort at Caltech. What are some ways that you're contributing overall to ensuring that Caltech is a leader in sustainability research?
YEH: In the areas of sustainability, as you know, Caltech has the Resnick Sustainability Institute, which certainly plays a crucial role in promoting research in sustainability on campus. Sustainability is a subject that encompasses many different fronts, of course. Green energy generation is one of them. Energy storage is another, which is critically important because green energy often is not dependable: For instance, wind and solar energies [laugh] depend on the will of nature [laugh], whether Mother Nature will be amiable enough [laugh] to provide the energy that we desire. This type of energy generation goes on and off, and energy storage becomes critically important. Of course, working on means to reduce energy consumption in all kinds of modern technologies will also be helpful for sustainability. Additionally, sustainability also involves issues related to environmental protection, such as reduction of carbon emission and efficient carbon sequestration. An important quest in this area is to figure out how to convert carbon produced in different processes to useful things rather than directly emitting it into air to pollute the environment.
Also, of course, reusable and recyclable materials are important topics in sustainability because we human beings have committed such a big crime against nature [laugh] through the production of an incredible amount of junk that is not good for Planet Earth. All of these fronts are important areas to deal with. I think Caltech has very good efforts in research areas of energy conversion and energy storage. These are the areas that we can do even better, hopefully. You were asking me how we make sure that we lead in sustainability. I think that people just need to work diligently. Hopefully, with good collaborations of the great minds and have good strategies, Caltech researchers can work towards things that are impactful. Caltech has done a lot, but we can certainly do more, I think. Some of the nanomaterials that I am exploring are also useful materials for energy storage in lithium-ion batteries and supercapacitors. I have also worked partially in this area as a side project, because we can functionalize and optimize nanomaterials to enable their energy storage capabilities. This area of research is related to, again, fundamental science, and at the same time is relevance to technology. But I am not a key player in these research areas at Caltech. There are other faculty members who are actively working in these areas. The subject of power grid is also important when we talk about using green energy, because it is critically important to optimize the power grid to distribute energy efficiently. All these topics related to sustainability have involved researchers actively work on them at Caltech, and that's very important. But I think we need to expedite more effort [laugh] along those directions to be even more impactful.
State of the Art in Superconductivity
ZIERLER: Nai-Chang, some technical questions. First, what is exciting right now in the world of superconductivity, and what are you and your research group doing in order to further the field of superconductivity?
YEH: An exciting but not yet useful aspect that I am now working on is that people have found in certain types of superconductors, if you add enormous pressure to them—"enormous" means that the pressure will be as high as near the core of Earth. [laugh]
ZIERLER: [laugh]
YEH: Imagine that you can apply an enormous pressure to a tiny little bit of certain materials, and then they could become superconducting at room temperature. Unfortunately, these findings are still controversial because the measurements are very difficult and inconclusive: When one applies such high pressure to such tiny areas, it brings to question about how accurate those measurements actually are, whether superconductivity is truly superconductivity, and all that. But it's potentially very exciting that in certain extreme states of matter that room temperature superconductivity could be reached. On the other hand, when I said it's not useful yet, it is because if such high pressure is required to achieve superconductivity, even if such materials can be made in large quantity, it's never possible to apply the same extreme pressure as that in the center of the Earth [laugh] to sizable samples to achieve room temperature superconductivity. Then this type of superconductor will not be superconducting under normal conditions, and so that's not going to be useful. A holy grail of superconductivity is, of course, to achieve high quality superconductivity at room temperature.
ZIERLER: What does high quality mean? What is the threshold of high quality?
YEH: High quality means that the superconducting state is highly reliable so that when a small amount of electrical current is applied to the sample, it will have no dissipation, and the system can actually sustain superconductivity up to a critical value of electrical current or can sustain superconductivity equivalently up to a critical value of external magnetic field. When these criteria are satisfied, the sample would be considered as of high-quality superconductivity. Currently, the well-known high-temperature superconductors, such as the cuprate superconductors, exhibit very interesting and exotic properties from the perspectives of physicists. The research of cuprate superconductivity has, to some extent, led to modern materials advances. Certain types cuprate superconductors can be made into superconducting wires up to miles long, which can be wound into superconducting solenoids to achieve very high magnetic fields. Such high-field superconducting magnets can be useful for various applications. However, these cuprate superconducting wires are still very hard to make. When I said "miles" of wires, miles long of wires, it may sound very long, but in reality if you coil them into a superconducting solenoid, it will not be a very large superconducting [laugh] magnet.
Anyway, although high-temperature superconductors have very beautiful physical properties, they are not perfect materials. Also, they are not mechanically easily fabricated. When I say good superconducting properties, they should be able to sustain superconductivity under large applied currents, large external magnetic fields, and large external electromagnetic signals. High-temperature superconductors, because of their special physical properties, even though they can support superconductivity at relatively high temperatures, a lot of the properties that are characteristics of high-quality superconductivity are often compromised. For instance, they cannot be used for very large-scale applications because of the difficulties in mass producing these materials. Existing technology for fabricating cuprate superconductors can make good enough materials in smaller quantities, and then use them for specific applications, such as specific sensors, specific detectors, small magnets for imaging and scientific research, for instance. There are applications that a small quantity of these materials can realize to date, but the applications have not reached the level of high expectations that people had when high-temperature superconductivity was first discovered. For instance, if we want to use the very best properties of these superconducting materials, we still need to cool them down to relatively low temperatures; not just keeping them at liquid nitrogen temperature, which is not too much below their superconducting transition temperatures. These superconductors have some good properties that we could use for applications, but they cannot be manifested until the superconductors are cooled to temperatures much lower than their superconducting transition temperatures, which defies the original expectation of realizing applications of superconductivity without the need of excess cooling.
Most likely, if room temperature superconductivity can be achieved, a lot of the properties of these superconductors are going to be not good at all unless they are cooled down [laugh] to low enough temperatures. That is a problem because the expected benefit of room temperature superconductivity cannot be realized. On the other hand, from scientific perspectives, the cuprate superconductors and some of the ion-based superconductors, why they're superconducting at relatively high temperatures is still not a settled issue—not theoretically settled. But we have made contributions to, basically, narrowing down the correct theoretical domain. That's about what we have done. I still collaborate with other people every now and then on finding new superconductors. Although these new superconductors don't have very high transition temperatures, they have very interesting properties, which can contribute to further understanding of different physical mechanisms for superconductivity. These studies have led to very interesting and nice publications, but they are essentially academic publications for now; not very useful yet.
ZIERLER: Nai-Chang, what have been some of your contributions in the field of microscopy, and what are we able to see or analyze as a result of the kinds of expertise that you bring to this field?
YEH: Microscopy, OK. The primary thing we developed is the scanning tunneling microscope, but I wasn't the first person to develop it. I just built my own homemade system so that we could go down to atomic scale to see, to image the properties and inspect the properties down to atomic scale resolution. Then using this tool, we have been able to make important contributions to understanding the mechanism of high-temperature superconductivity. We have also carried out some very interesting scientific studies using the scanning tunneling microscope on topological materials. We use scanning tunneling microscopy as a tool to investigate the physical properties, particularly the spectroscopy, of interesting materials; so our emphasis is not on imaging the materials like a microscopist, although we can also carry out atomically resolved imaging of materials. We build our own instrument. We know how to design, construct, and assemble it. We know how to manipulate different parts of the instrument, and we can add new capabilities to the instrument. So, we can use our homemade instrument to investigate interesting new properties of various quantum materials. From this perspective, I would not say that I contribute to microscopy. Rather, I utilize advanced scanning probe microscopy to investigate the science of quantum materials. That would be my contribution. The new science that I derived by using our homemade tools to study quantum materials would be my contribution. On the other hand, I am trying to add new capabilities to the microscopy, which is not completely done yet so I cannot take credit for anything yet. What I am trying to do is to add light, that is, optical excitations, to quantum materials while I use the tools of atomically resolved spectroscopy and microscopy to study these materials.
ZIERLER: What does "excitations" mean in this context?
YEH: Light excitations. You're asking me what it means?
ZIERLER: Yes.
YEH: Think about it. Let me make an example of excitations that you can relate to. When light interacts with materials, for instance, they can interact with electrons in a material, such as silicon. Silicon is a semiconductor, so there is a valence band. The valence band's electrons are occupied, and then there is a conduction band where electrons are not occupied. For a semiconductor, there is an energy gap between the conduction band and the valence band. Now, you can either add a strong voltage to your material to force electrons to jump through the energy gap from the valence band to the conduction band, or you can just shine light on the material to excite the valence band electrons into the conduction band if the light has an energy that is bigger than the energy gap. In the case of silicon, the energy gap is about 1.1 electron volts. The energy of infrared light is above that energy, and visible light is even higher in energy and so will definitely fill the bill. So, when light with a sufficient energy is shine on silicon, the silicon can absorb the light and promote an electron from the occupied valence band to the unoccupied conduction band. This process leaves behind a hole in the valence band and create an electron in the originally empty conduction band, which gives rise to the formation of an electron-hole pair. But this electron-hole pair is an excited state induced by light, which is not the natural ground state.
The creation of this nonequilibrium state is because silicon absorbed a "photon" from the light. Here a photon is a quantum of the light that shines on the material. The creation of this electron and hole pair in the excited state is not stable. The electron and hole will recombine after a finite lifetime, which will emit a photon again after recombination. On the other hand, although the electron-hole pairs are unstable, if we can devise means to "grab" electrons out of the conduction band within the lifetime of the electron-hole pairs before the electrons recombine with the holes, then we can harvest photo-induced electrical current. This is the principle of how photovoltaic cells based on silicon panels work in the context of optical excitation, as an example. Is that clear? [laugh]
ZIERLER: It is, thank you.
YEH: OK.
ZIERLER: Nai-Chang, in preparing for our discussion, and in studying your publication list, I was impressed with myself. There were only two terms that I had not come across before, so I'll ask you about them. The first is valleytronics. What are valleytronics?
YEH: Valleytronics are kind of related to what I was just telling you about: conduction bands and valence bands. Silicon, for instance, has many different valleys. What do valleys mean? [laugh] This is getting very technical. They refer to the electronic structures in materials. Usually, we describe the energy bands and the electronic properties of solid-state crystals, materials, in terms of energy and momentum dispersion relations. Take silicon, for example. There will be regions that the conduction band—if you would consider energy versus momentum—then the conduction band at a certain momentum would reach a minimum. The valence band at a certain momentum will reach a maximum. Then where you have this minimum and maximum of the conduction band and valence band, near certain momentum value in the material, then that's called a valley. [laugh]
Silicon itself has multiple valleys, and these momentum values are where a lot of interesting things are happening because the minimum of the conduction band and the maximum of the valence band meet at those special momentum values. If you are doing excitations—now you know what excitations are. They can be electronic excitations or light-induced excitations; you can do whatever. The valleys are where a lot of interesting things can be happening. If you design electronic devices that are utilizing special properties at those valleys, then these devices are called valleytronics. But it is hard to describe them truly accurately without getting to very, very [laugh] technical descriptions. Anyway, those are special momentum values in certain materials, particularly semiconductors such as silicon, that interesting things happen. If you know how to control properties, utilizing the special properties at those valleys, you can achieve new devices that are different from traditional devices. Then one important thing is that valleytronics—valley-inspired or valley-based electronics—they can have very low dissipations. If you turn materials with interesting valleytronic properties into "valley Hall transistors" -- you can make transistors out of them, then when you apply electrical current and back-gate voltages to study the transistor properties, because of the special valley-related properties, you could have very low dissipations in the transistor that you design. That's my motivation. It's a low-dissipation transistor. But then valleytronics can also become topological if you can control different valleys. Supposedly, these valleys should be degenerate if you don't manipulate them. Here "degenerate" means that you may have multiple valleys in a system at the same energy, and then all these valleys will contribute equally, which won't be very special even though you may be able to make some interesting devices that exhibit low dissipation.
But if you can truly manipulate the valley degrees of freedom, you will also be able to create topological devices. When you create topological devices, an important outcome is that they will be less susceptible to external perturbations or disruptions. They can be useful for quantum information propagation as another possible example. To understand exactly how valleytronics work takes a lot of technical descriptions, and I cannot cover that all together. But at least you can appreciate the fact that valleytronics can be potentially made into low-dissipative transistors, and can also be useful for quantum information propagation and storage.
ZIERLER: Nai-Chang, the other term that I was not familiar with is perovskites. What are they?
YEH: Perovskites are a special type of crystals. They come in many different forms, but the basic form is called ABX3. What are they? "A" means a rare earth element. "B" means a transition metal element. "X" can be oxygen, sulfur, fluorine. Anyway, they belong to group 6 elements. You can combine ABX3 (with atomic ratios of A : B : X = 1 : 1 : 3) or variations of them. They form a cubic-like structure, and then variations of them can turn them into like rectangular-shaped. But they are all in the cubic or rectangular shapes of structures, and they have special atomic arrangements. "Perovskites" is just a name given to these types of crystalline materials, with ABX3 being the primary structure together with their variations. High-temperature superconductors are perovskite materials.
ZIERLER: Nai-Chang, for the last part of our talk today, for our remaining time, two last questions. First, all of our discussion has taken place within the context of history, all the things you've been doing in the past. What are you currently working on, and what are some of the exciting things happening in your research group where your postdocs and your graduate students might provide a window into the future of the field?
YEH: Actually, what I am working on is related to what you asked me earlier about my research phases in the middle and the tip of the pyramid. That's what we are pursuing. I still work on correlated materials and topological materials. But I am utilizing different tools. For instance, I can do nanoscale engineering of materials. I can then build in new properties in these already very interesting topological and quantum materials by doing nanoscale engineering of the materials, shape them into new structures and create new properties based on my theoretical understanding and simulations, and then verify the ideas by nanofabrication of these engineered nanostructures and experimentally study their properties. Using this approach, we can create brand new properties from these interesting materials. With these new properties, we are pursuing new devices and new physical phenomena. That's what we are working on. We make our own materials. I'm proud of that because a lot of people don't make their own materials. Some of them always get materials from other people. I am not against getting materials from others because I also get some of the materials from other people through collaborations. For some of the key materials that we are studying, like the so-called "van der Waals materials", which are two-dimensional quantum materials, including graphene, transition-metal dichalcogenides, and some of the topological insulators, for instance, we either make them (in the case of graphene and transition metal dichalcogenides) or get samples from collaborators (in the case of thin-film topological insulators). But then we design special structures using nanoscale engineering techniques. Based on our theoretical understanding and nanotechnology capability, we have been able to instigate new properties into the two-dimensional quantum materials based on our observation of very exciting new phenomena that never existed before. I'm going to see how far I can push with this methodology. I want these novel devices based on nanoscale engineering of two-dimensional quantum materials to be truly reliable, reproducible, and scalable. Then there are many other new things that I'm considering. I am combining nanotechnology with novel materials to create new phenomena, and I'm also adding light to create new states in materials and devices by causing light and matter interaction. With all these things combined, we are pursuing new states of matter, new properties, and new devices. That's the scientific part. But I also know that these developments can potentially be very useful for technology. But let me do the science first. [laugh]
Formative Advice to Achieve in Science
ZIERLER: [laugh] Nai-Chang, for our last question today, and that will work into our subsequent conversations where we go back and develop your personal narrative, you are the first in two very important regards. This is more of a social and a political question. As the first tenured woman professor in physics at Caltech, and also the first tenured Asian woman professor at Caltech, what has being the first in these two areas, what has that meant to you personally, and in what ways do you grab onto the obvious way that you might be a hero to others in the field?
YEH: Personally, in my professional research, I didn't really think about those issues that much because I had a wonderful mentor, Professor Millie Dresselhaus. She was my research advisor.
ZIERLER: The Carbon Queen.
YEH: Right, the Carbon Queen [laugh], yes. She used to tell me—which I fully agree, actually, even before she told me but I was glad that she told me the same thing I was thinking about. She said to me, "Nai-Chang, just concentrate on your research, and do it well. Don't think about other things yet. When you become accomplished, when you are accepted, then you can do more things for others after you establish yourself." I fully agree with that. While I was going through the tenure process, job interview process and whatever, I was not thinking about other issues besides research. I was just concentrating on doing well in research so that I'm one of the—hopefully—one of the top scientists, and so people would not think about gender or race issues when they interact with me. I think that's extremely important. In science, in the scientific community, when you reach a certain threshold of research accomplishments, people will just accept you as a good scientist and forget about other irrelevant things.
However, I do know there's a much higher threshold for people who don't look like a "typical" scientist, whatever your definition of "typical" may be. Yes, I didn't realize the hurdles until I [laugh] got into this business, and I actually had experienced excess hurdles. But then I tried not to think about the fairness (or unfairness) of such things, and just tried to do my best, because if I don't do my best, and if I don't work out, then I would have myself to blame. But if I manage to work out, then I would be more than happy to champion for others. That was my mentality while I was going through the tenure process. Of course, my experience made me realize that it's important to have role models. In this context, I must say that I was very lucky: My mother was a math professor. My advisor [laugh] was Millie Dresselhaus. Actually, I didn't look for her to be my advisor when I first went to MIT. She came to find me. [laugh] I was so lucky. I really didn't know anything then as a first-year graduate student at MIT. I just came from Taiwan to the US for the first time and all that. That's a long story. But, anyway, I was very lucky. Also, I grew up in Taiwan. The environment was such that if you were academically accomplished, you would be valued and respected. I was always like number one [laugh] in my class. Even though I was the only woman in my physics department at National Taiwan University, I was the best student at the time. So, I never had problems with any discrimination. I was always respected by my classmates and they were all my good friends. I was also the favorite of teachers and all that. I never experienced anything negative in my academic pursuit until I came to the US. [laugh] Then I realized that, oh, things were not so simple. And I also realized how important it was to have a role model because in retrospect, I found that having my mother and my advisor [laugh] there as my role models throughout my academic pursuit, I had always taken it for granted that women could be equals to men in science. I didn't realize that things could be any different for women until later. But my experiences also taught me to be supportive of young people. I feel strongly that the talents are independent of race and gender, and we should always look at people just based on who they are and what they do. All other things are irrelevant. But then the world is not perfect, and people are often uncomfortable, or suspicious even, with what they're not familiar with. That's something we still need to work on. Then I realized that I can be a role model [laugh]—
ZIERLER: [laugh]
YEH: —for others. I always try my best to do what I can to not only encourage young women but also encourage young men, because, being a woman mentor, when you do well, when your students look upon you or your junior colleagues look upon you as someone that they can respect and trust, and then they forget that you're a woman [laugh]—
ZIERLER: [laugh]
YEH: —they would just think of you as a colleague, as a mentor, that's the best. And so I do what I can, because the world is not perfect.
ZIERLER: That's right.
YEH: But [laugh] I try to make it better.
ZIERLER: Nai-Chang, on that note, this has been a wonderful introductory conversation about your research and your career. In our next conversation, I look forward to going back to Taiwan, and developing your personal narrative.
[End of Recording]
ZIERLER: This is David Zierler, Director of the Caltech Heritage Project. It's Thursday, July 14th, 2022. I'm delighted to be back once again with Professor Nai-Chang Yeh. Nai-Chang, it's wonderful to be with you again. Thank you for joining me.
YEH: Thank you. It's nice to talk to you.
ZIERLER: Nai-Chang, what we're going to do, following our initial discussion where we took a wonderful tour of your science and approach to research, I'd like to go all the way back to the beginning, and learn about your family background. Perhaps we can start first with your name, your given name, and your family name. What does Nai-Chang mean?
YEH: There are actually two characters in my name—usually, the first character for the first name often indicates a specific generation of a kin. Therefore, all my siblings share the same first character in our first names. The first name has two characters. The first character is, Nai, like, my name is Nai-Chang, and my elder brother is Nai-Chi. My younger brother is Nai-Sher. And my younger sister is Nai-Tzi. This first character indicates a generation, which usually doesn't represent very much. Elders of a kin would select a character for a generation. Sometimes, they would pick a character that looks pretty. But in most cases a neutral word would be selected. Not all families give names by following this tradition, although a lot of families still do. In this naming tradition, the second character of one's first name then becomes meaningful. My father picked a very beautiful word in Chinese characters for me. That word is usually not used in daily life. It usually appears in poems [laugh], in poetry. The word means "splendor". The character written in Chinese calligraphy is very pretty. As for my last name, the family name, it means "leaves", leaves on a tree. Anyway, that's what my name is. [laugh]
ZIERLER: Could you know from the family name where your family comes from, and what their ethnicity is?
YEH: Usually, from the family name, it's kind of yes and no. My family name is quite common, although it is not one of the most common last names. One of the most common last names will be like Chen or Chang. Yeh is not as common, although I do know in certain areas of Canton Province, for instance, there would be more people by the last name Yeh. Anyway, my ancestors came from China and went to Taiwan. China is such a vast country. There are different ethnic groups all over, so it's hard to say exactly what the ethnicity of my ancestors may be. But the fact that my parents came from the southern part of China, and that in the southern part of China, people with these family names were, in ancient times, from the central part of China. They migrated to the southern part because of the invasion of northern barbarians. [laugh] But then, in the end, there are a lot of mixed intermarriages of different ethnic groups. Otherwise, the dominant group in China is called the "Han" people, and I think my family primarily came from them, and they were the Han people. But, again, it's hard to say because it's so complicated.
ZIERLER: That gets me to my next question. Your parents, were they born in Mainland China, or were they born in Taiwan?
YEH: They were born in Taiwan. But their parents, my grandparents, actually lived in China for some time, and then they moved to Taiwan. My parents were born in Taiwan during the time when Taiwan was under the occupation of Japan. That was the time because Taiwan was a colony of Japan for 50 years. After the last dynasty of China, the Qing Dynasty, became very weak and corrupt, and they lost a major sea battle against Japan, the government gave Taiwan and a few other small islands away to Japan as a part of a shameful peace treaty, and also gave lots of money to Japan to compensate [laugh] for the war. Anyway, it's a long story.
ZIERLER: Nai-Chang, did you know any of your grandparents? Did you have a relationship with them?
YEH: My mother's father passed away before I was born, a few months before I was born, I think. But my mother's mother was a wonderful lady, and she was still around until I came to the US for graduate school. She passed away then. We were relatively close. I really liked her. She was wonderful, very, very kind, and generous. When I was very young, between the age of 1 and 2 years old, my older brother and I were staying with my mother's mother at the time, because my mother was teaching in a university in Tainan, a city in the southern part of Taiwan, while my father at the time had to work in Taipei. We stayed with the grandmother, and so she kind of looked after us. Anyway, she was my favorite [laugh], one of my favorite people. Then my father's father was also very nice—a very, very nice guy. He passed away when I was a baby, so I don't have a very clear memory of him, but I know he was a very nice person, and I have a few photos showing him holding me [laugh] when I was a little baby. But then, unfortunately, I didn't get to know him very much. I had a little vague memory of him but not very clearly. My father's mother lived with us for some time. Actually, we kind of grew up with my father's mother around for years. Then she also passed away when I came to the US for graduate school.
ZIERLER: Nai-Chang, what was your family's experience during World War II?
YEH: Absolutely awful, especially for my mom's family. At the time, because Taiwan was considered a territory occupied by Japan, Taiwan was heavily, heavily bombed by the US. There was nothing called precision bomb at the time, so when the airplanes dropped bombs, there would be a lot of civilian casualties. One time, my mother was still a little girl at home with her younger sister, and the US aircrafts came and bombed the area. They were trying to bomb some military targets but, of course, the bombs were not precise, and so the house was hit and was on fire. My mother had to drag her little sister out their house and tried to run for their lives. My father's side was kind of interesting because Japan had occupied Taiwan for some time, so they became Japanese citizens. My grandfather—my father's father—was sent to Canton as some kind of a bureaucrat to take care of things. It was a time towards the end of the war, so there was severe food shortage. Lots of people died of poverty, died of hunger.
My father recalled that every morning, if one went out there would always be a cart pushed out by people to pick up dead bodies. Because of the war, a lot of refugees escaped from where they used to live to Canton and then, of course, there was a severe food shortage. At the time, Canton was under Japanese occupation, and so they would ration food only to Japanese citizens first, and then other people. There was just not enough food around, so a lot of people died. My grandfather, because he was considered a Japanese bureaucrat, he had some food ration for the family. He was a very kind man, so he always tried to save some food for the hungry. Every day, he would give some food out to the refugees. He basically took it out of the family share and tried to help some people. In the end, after Japan surrendered and the war ended, my father's family were going to return to Taiwan from Canton. At the time, a lot of the Chinese people who were working for the Japanese were beaten very badly by the Chinese. But my father's father and his family were well protected by the locals because my grandfather was so kind to others during the war, and so they were spared from retributions. They left safely without being injured or beaten up, and so they went back to Taiwan.
ZIERLER: What level of education did your parents achieve?
YEH: Both of them have bachelor degrees, and they both went to the best, the top university in Taiwan, National Taiwan University. That was very rare, actually, for their time because only a small fraction of the population could enter universities through extremely tough entrance exams, and it was even tougher to enter the best university. Both my parents were able to pass the entrance exams to get into the top university, National Taiwan University, based on their own academic merits. [laugh]
ZIERLER: What were their degrees, and what were their professions?
YEH: My mother got her degree in mathematics. At the time, a bachelor degree was already a high-level education, and she was academically very good, so she was able to stay in the university to teach as a lecturer first. Then, throughout the years, she would publish research papers and passed evaluations for promotion through the professorial ranks. In the end, she was promoted to a full professorship, even though she did not have a PhD degree. At the time, it was economically very difficult for most people to afford education for a master or a PhD degree, and so, a bachelor degree from the best university in Taiwan was already considered very good qualification for entering a university faculty position. If one continued to have good publications in reputable research journals, one would get promoted through the professorial ranks. That was the situation for my mother. As for my father, he met my mother at the National Taiwan University because [laugh] they both went to the same school. My father studied economics. Then he passed the exams for civil servants after his graduation from National Taiwan University and two years of compulsory military service. Again, those were tough exams, and he went to work at a national bank, the Bank of Taiwan. He entered the bank through passing a test, again. [laugh] In Asian cultures, there are often lots of tests for entering schools and professional positions. But that's actually one way to make sure that the selection procedures are as fair as possible; not through back doors; not through relationships -- You have to demonstrate merits and qualifications to enter a certain level.
My father became a banker at the Bank of Taiwan. He climbed up to high positions from a lower-level management without being a clerk first -- I think he started at lower-level management because he had a high degree—at the time—from National Taiwan University. The best university bachelor degree was considered one of the top qualifications, and he also did well in the tests for civil servants, so he was able to start from a lower-level management position. He climbed all the way up to one of the top management positions throughout the years of his service. When he retired, he was one of the senior vice-presidents in Bank of Taiwan. He specialized in international trades and had lots of real-world experiences, and so several universities also invited him to be an adjunct professor to give lectures to their business school students. During the day, my father would work in the bank, and a few nights a week, by invitation, he would teach at some of the universities. His courses were extremely popular and were always heavily subscribed because he had all these real-world experiences in handling large-scale international trades and so students could benefit a lot from his lectures. Both my parents were kind of the academic type. [laugh]
ZIERLER: Nai-Chang, growing up, did you see your mother and her profession and her degree as pathbreaking for a woman in Taiwan at that point?
YEH: It was supposedly pathbreaking. [laugh] But, at the time, I was kind of not paying a whole lot of attention to her professional accomplishments. In retrospect, I realize that her role was very important. [laugh] She was a professor in a Catholic university (Fu Jen Catholic University). It was a Catholic university newly established in Taiwan then by a group of Germans. So, the university administration was actually more forward-looking and managed by some Germans. [laugh] Of course, they all spoke fluent Chinese, and they were generally more open-minded than most administrators in Taiwanese universities. As an example of the open-mind of the administration, which was an incident that I did not know until years later, my mother found out after she started teaching there that woman professors were paid less than men of the same ranks and duties, and so she protested to the administration, arguing that it should be equal work, equal pay. After her protest, the university administration agreed with her and changed their rules to implement equal pay for the same faculty rank regardless of the gender. It was in fact a breakthrough for the university system because at the time, Taiwan was still a society that men were favored. [laugh] It did not matter how good a woman could be, she would not be treated equally in most workplaces. It was like that then. But the administration at the Fu Jen Catholic University were much more open-minded, so when my mother argued for the case, they felt that it was the right thing to do. I believe that it was the first university [laugh] in Taiwan that really gave women and men equal work, equal pay. It would take more than a decade later for all other universities in Taiwan to achieve gender equality for their faculty pay.
ZIERLER: Nai-Chang, where did you grow up in Taiwan?
YEH: Before I turned aged 2 [laugh], supposedly—I don't remember a whole lot, I was living in a city called Tainan in the southern part of Taiwan. That was because, as I mentioned earlier, after my mother graduated from National Taiwan University in Taipei, she got a teaching job in the National Cheng Kung University in Tainan. My father, at the time, passed the civil servants' test, got a bank job in Taipei. Like many young couples, they just started their careers and had to struggle in the beginning. So my mother decided to stay in Tainan, living with her mother together with me and my older brother. The decision was made in part because my mother's father passed away not too long ago -- I mentioned earlier that my grandfather passed away a few months before I was born, and so my mother would like to keep my grandmother company, and to keep her busy so that [laugh] she had to handle two little kids. [laugh] My grandmother looked after me and my older brother when my mother was teaching, for a couple of years. Then my mother found a job in the Fu Jen Catholic University near Taipei, which was a newly founded university in Taiwan. My mother was able to move to Taipei then, so that the family could stay together. Then after age 2, I grew up in Taipei. Also, for my education, I was lucky to grow up in Taipei. A little background about the Taiwanese education system: In Taiwan, grade 1 to grade 6 is considered elementary school, and then grade 7 to 9 is junior high school. Grade 10 to 12 is senior high school, and then 4-year university education. It was a very strict education system when I grew up. At the time, if one wanted to get the best education in elementary school and junior high school, one would have to pass highly competitive entrance exams to enter one of the few very famous private schools, even though public school education was free for elementary school and junior high school whereas private schools were quite expensive.
Many parents still wished to send their children to these best private schools despite the relatively high costs and the requirements to pass very tough tests [laugh] to enter those schools. My parents didn't make a whole lot of money at the time. But we were all educated in private schools when we were growing up. For my elementary school and junior high school, I was able to pass tests to enter one of the best schools. Then after junior high school, at the senior high school level, there were the so-called joined entrance exams for different regions so that all senior high schools in the same region would hold the same tests on the same two days. At the university/college level, all schools in Taiwan would hold the same joint entrance exams on the two days, although there were four types of exams for people who chose different programs of studies, which included Natural Sciences and Engineering, Humanity and Social Sciences, Biological Sciences and Medicine, as well as Law and Business. What was interesting about the system was that starting from senior high school to colleges/universities, if you pass these joint exams—when all students were tested together; not tested by individual schools/universities, they were all ranked together, the whole island. [laugh] Whether one could enter a specific school/department depended on the test scores of the student as well as the ranking of each school/department. Therefore, only a small fraction of the students could get into top schools.
Interestingly, starting from the senior high school level, the best schools were always public schools. Of course, then, the tuition and fees for public schools would be much cheaper, because they were heavily subsidized by the Taiwanese government. But it was very hard to enter those schools. I happened to pass all of the tough tests and entered as the number one student [laugh] in the school/department. Anyway, I went through these tests. What I was trying to convey is that the very best schools were all in Taipei, and so I could just stay at home while attending the very best schools throughout my education in Taiwan. I went to the best girls' high school. Then I went to the best university. They were all in Taipei and were not far from home. I only had to take a bus to go [laugh] to school every day. Anyway, I grew up in Taipei for that reason.
ZIERLER: Nai-Chang, besides testing so well, were you interested and were you particularly good at science and math, growing up?
YEH: I was actually good at almost everything, except for painting. [laugh]
ZIERLER: [laugh]
YEH: My older brother is superb at painting. [laugh] We joked about the fact that he took the painting genes [laugh], and then I took a lot of the other good genes. [laugh] Actually, when I was growing up, originally, when I was small, I really loved music. I also loved literature and history. I love those things very much. But I was also simultaneously very good at math and science courses. I was good at learning and taking tests. Those things were easy for me. [laugh] It was just fine. I enjoyed studying, and always studied well beyond what the school curricula offered. My mother always had to spend a lot of extra money to buy books for me. [laugh] We were not that well off at the time. But because I loved reading, my mother would keep buying books to keep pace with my reading. Whenever she had extra cash [laugh], she would buy books for me. Also, although my mother was a mathematician—she loves math, yes, but she loved music and literature even more. [laugh] I think I was strongly influenced by her, and so I love those things.
Actually, I didn't think I would become a scientist when I was in elementary school because, like a lot of young girls growing up at the time, I kind of thought, oh, sciences are things for boys—although I was good at them. They were no big deal because I could handle them well. But I thought that they were topics more suitable for boys to pursue until I went to junior high school. In elementary school, we only learned science as one topic, as well as math and other topics. But in junior high school, we started learning separate topics of science, including physics, chemistry, and biology. In other words, the science subject was divided into three major areas. When I started learning physics, I found that I really loved it. I was so amazed by the fact that one could use relatively simple physical laws to describe so many things in nature. It was like there was truth in the universe, and one could find the thread of it through physics and discover the beauty in it. I was so amazed, knowing that, wow, I could understand things from elementary particles all the way to the whole universe through the understanding of physics. I found that absolutely fascinating. I felt physics was as beautiful as music, as literature, as poems, except that it is a different higher-level language. I kind of fell in love with physics. But, of course, I was learning quite elementary topics at the time, and so I started reading a lot, hoping that I could understand physics even more, better, deeper. Then, while I was doing that—I was 12 at the time [laugh], age 12 at the time—reading lots of books, I realized that there were two important concepts I didn't know. One was quantum mechanics. The other was relativity. [laugh] These concepts were everywhere in all those books I tried to read. I was like, "oh, my god, I am so ignorant. I have to learn a lot, lot more."
That was when I said to myself that I would become a physicist so that I could learn the language in order to truly appreciate the beauty of the universe, and to pursue the truth and beauty of the universe through the language of physics. I felt that I could always enjoy music, and I could always enjoy poems. But physics is something that unless you know the language well, otherwise, you don't get to truly appreciate it. That was the reason why I decided to become a physicist. But I was also good at other subjects. All my teachers of different subjects wished that I would take the profession of their subject, [laugh] including my history teacher, literature teacher, and, of course, math teacher [laugh]. In Taiwan, the lucky thing was that if one was an academically good student, at least when I was growing up, then one would generally be well liked by teachers and fellow classmates. As long as an academically top student was not a very bad person [laugh], he/she would be the favorite of teachers and highly respected by classmates and peers. I was lucky to grow up in this type of environment so that I never felt that there was anything women could not accomplish because I was always treated well in an academic environment, and my classmates and friends were all very nice to me. Additionally, it was common for academically accomplished students to be elected by their peers to leadership positions. So, I had to learn to lead. [laugh] Also, I was good at learning and taking tests, and so my classmates often asked me for help, and I would teach them how to improve in their studies by explaining to them how to truly understand various subjects through the methods that I developed to understand the material.
ZIERLER: Nai-Chang, did I hear you correctly, that you went to an all-girls high school?
YEH: That's the senior high school, yeah. At the time, the best senior high schools were divided into all boys schools and all girls schools. These were public schools. But then, of course, at the university level, there were no longer such divisions so that all universities were coeducational.
ZIERLER: What were the family decisions that led to you going to the all-girls school, and what were some of the either challenges or benefits of that for you, looking back?
YEH: The all-girls school, that wasn't the family's decision or anything. At the time, the best senior high schools were divided up that way.
ZIERLER: I see.
YEH: We just took the joint entrance exams, and people were extremely proud if they could get into these best schools because they were so hard to get in. Only a tiny percentage of the students taking the tests could get into these best schools. The system at the time was all-girls schools, all-boys schools for the senior high schools. I suspect it was a conservative idea at the time, thinking that, oh, around the senior high school age, probably boys and girls would try to start getting to know each other, [laugh] and so if they were in the same school, then maybe they would not study hard [laugh] because they would get distracted. [laugh] I think that might be a reason. But, of course, it did stop anybody [laugh] because you can go to school during the day, and then after school, you can still get together with people or join different activities. Anyway, it was a conservative society at the time, so that was the system.
Early Interests in Physics
ZIERLER: Nai-Chang, in Taiwan, is it like in Britain where you declare a major right away, or is it more like the American system where you take a general field of study before declaring a concentration or a major?
YEH: For entering universities, you had to declare a major first during my time in Taiwan. But my understanding is that the system is more flexible now. But at the time, when I was taking the tests, exams, we had to choose the general areas. There were like four major different areas of topics. I was in the area of natural sciences and engineering. Then there would be another area for literature and social sciences. There would be a third area for biomedical, and a fourth area for certain types of social sciences and laws and businesses and trades and accounting. That's the fourth area. Before we took the tests, we have to choose one area. The exams were tailored into four different groups.
There were some common things among the four groups. English tests and literature, Chinese literature tests would be the same. But it depends on which topic area that you are in, then there will be different tests. I didn't have to take tests in history and geography. But I had to take tests in physics and chemistry. For people who were doing bioengineering, no, sorry, biomedical, they have to take chemistry and biology tests. But they didn't have to do physics. I didn't have to do biology in the tests, and biomedical people didn't have to take physics. But other things were similar. For us, for natural science and engineering, our math test would be the hardest, would be made extremely hard for the math. But then for the other two groups not related to science or engineering, then their math test would be much easier. We had to declare an area first to take the test, and then we had to rank all these different universities, different departments. We ranked them according to our wish from top down, all the way, for all of the schools and departments within the area. But then, of course, different universities, different departments, they set their own standards, so if your scores did not meet the minimum entry standard, then you could not get into that department. There was a matching issue. There were school and departmental standards, and you had to match that standard in order to get in. I was one of the top 10 students in the entrance exams for natural sciences and engineering, and I picked physics as my top choice. But, at the time, electrical engineering at National Taiwan University had the highest entry base point, and I was well, well over that. I could have gotten into electrical engineering. Had I wanted to do biomedical, I would have gotten into the medical school also with a flying color because [laugh] that was the highest entry point, also. Anyway, I already chose physics, and so I entered the physics department.
ZIERLER: Were you thinking back to earlier in your childhood about quantum mechanics and relativity? Was that part of your calculation, to further explore those topics?
YEH: Yeah, of course, because [laugh] those are basic things I must know, otherwise, I will not understand physics at all. But the funny thing was that, because my mother was teaching also, she was primarily teaching at the private school Fu Ren Catholic University. But she was also lecturing at National Taiwan University's math department. They had the best math department with all these genius math professors, and I knew them since I was a little girl because they were my mother's colleagues. They knew I was very good at math, so they were very upset when I didn't choose math to [laugh] be my top choice. I entered the physics department. Also, that year, I scored a perfect score in math. I was the only one in the whole Taiwan island that scored a perfect score in math, because the test was very hard. Those math professors were extremely upset [laugh] that I didn't go to study math. But, as a physicist, I had to study a lot of math anyway. That was my argument. [laugh] I still study a lot of math. I need to know a lot of math as a physicist. But I like physics even more. I love math too. Don't take me wrong.
ZIERLER: Tell me about the curriculum in physics in college. What was the divide between your exposure to theory and experimental physics?
YEH: That was a different time at the time. Taiwan was still not very prosperous at the time, and so it was much harder to do top quality experimental science. A lot of professors, if they were in experimental research, they didn't have enough money to buy fancy equipment. Often, they had to build things on their own, and so experimental physics was much harder to do at the time. For that reason, there were a lot of restrictions—no, I shouldn't say restrictions—limitations. For that reason, a lot of the best students then would rather do theory because theory is not limited by the availability of best equipment. But in the university, we went through a rigorous curriculum. We also had some experimental courses. But, of course, we didn't have very good facilities, and so it was not as inspiring. Then the theoretical [laugh] courses were more inspiring and challenging because there was no limitation. You could just do as much as you were capable of. You could go beyond standard curricula. At the time, actually, I had exposure to both experiments and theory but I was actually leaning towards theory as most [laugh] good students did at the time just because of the environment. It didn't have enough good facilities for you to do very interesting and advanced experimental research. Things are totally different now. [laugh] Things are totally, totally different. But while I was in college, that was the situation.
ZIERLER: Now, did you live at home throughout your undergraduate years?
YEH: Yes, to save money. [laugh]
ZIERLER: What did you do during the summers? Did you work? Did you study more?
YEH: Typically, many parents asked me if I could be their children's tutor. But my parents didn't want me to work. They wanted me to just keep reading, studying, whatever I wanted to do. [laugh] During the summer, I just studied more. I would go out, buy more books [laugh] and do more readings. We did things in a modest way because my parents, they did their best to support us. I actually also took piano lessons. All of my siblings learned different things. But my parents wanted us to study instead of trying to make money. We were very shielded. I just spent most of my time being a bookworm [laugh], reading lots.
From Taipei to Boston
ZIERLER: Nai-Chang, as you were getting close to graduating from college, and it was time to think about next steps, were there professors or your parents, who gave you the idea to apply for graduate school beyond Taiwan?
YEH: It was very typical. At the time, all the best students left Taiwan for graduate schools in top universities outside, and primarily in the US—some in Europe. But it was common. It was very, very common. All of the best students [laugh] left because the research environment at the time in Taiwan wasn't that good. If you wanted to pursue advanced knowledge, you left Taiwan for graduate school. At the time, it was like the entire class, maybe the top 60–70% or 80% all left for graduate school in the US or in Europe sometimes, but mostly in the US.
ZIERLER: How did you determine what programs to apply to? Did you already have a field of interest that you wanted to pursue, or how did you narrow that down?
YEH: No, at the time, I didn't have a clear idea of the area of pursuit, a specific area in physics. My strategy at the time, like most of my predecessors who came to the US, they just tried to apply to the very best schools so the research programs were generally good in all areas. That was the strategy so that we could go to one of the top schools, and then decide which area we wanted to pursue.
ZIERLER: Was it only the United States or did you apply to schools in other countries also?
YEH: I only applied to the US.
ZIERLER: How was your English at that point?
YEH: My reading English was just fine but I never practiced conversational English [laugh] so it was like a cultural shock when I went to MIT in Boston because I was having trouble with the Bostonian accent. [laugh]
ZIERLER: [laugh] You're not alone. Americans do as well. [laugh]
YEH: [laugh] It caused a lot of confusion because, for one thing, English wasn't my first language—and it still is not [laugh] but I'm much better at it now. But then with a strong accent, I was having [laugh] great difficulties.
ZIERLER: [laugh]
YEH: But, anyway, it was OK. I was kind of confused for a month. Then I got used to it. [laugh]
ZIERLER: Besides MIT, where else did you apply?
YEH: I also applied to other schools, I think, Harvard, Caltech, University of Illinois Urbana-Champaign, UCLA. I don't quite remember [laugh] some of these schools.
ZIERLER: Why did you choose MIT?
YEH: MIT admitted me. [laugh] I didn't get into Harvard, and I didn't get into Caltech. I was told I was waitlisted at Caltech. [laugh] But then, good, I came to Caltech to be a professor. [laugh]
ZIERLER: [laugh]
YEH: I think, it's hard to say. At the time, I didn't have much research experiences, unlike students in the US. That was one thing. I was just a student with good grades. I guess there were many factors. Who knows? MIT was one of my top choices, and so I was thrilled when I got admitted there. Caltech was also one of my top choices but I didn't get into Caltech. My top choices would have been Caltech or MIT, and I got into MIT so that's it. [laugh] I went to MIT.
ZIERLER: Nai-Chang, what were your first impressions when you arrived on campus? What sticks out in your memory?
YEH: Actually, I liked the campus. MIT was right beside the Charles River, and so along the river, it was nice, and there were some historical buildings that were fine. But I think at the time, I just felt a lot of uncertainty because [laugh] that was a different time. Again, nowadays, all these universities try to be extremely welcoming and nice to international students, and gave them a lot of good orientations, and look after housing and all that. At the time, MIT had this very weird system. Their dormitories were by lottery, and the higher seniority you have, the better chance you get. If you're an incoming student, it's almost impossible. Unless you're extremely lucky, you couldn't get into the dormitory. Of course, coming from Taiwan, Taiwan was still under martial law at the time. I had never left the island. When I came to the US, I couldn't find housing. I simply couldn't find housing, and that was before the internet. You couldn't do a search. You had to physically be there to look for housing. But I didn't want to spend too much of my parents' money because before school started, you didn't have any scholarships. I didn't want to spend a lot of money renting places first, so I kind of pushed until a week before school started to come to the US, and then that was too late to find housing, cheap housing outside of campus because all the cheap housing was already taken. I really had no place. I was actually staying in the Chinese Student Association building, sleeping on the floor for a week, with cockroaches moving around me. [laugh] Anyway, I couldn't find housing. But, luckily, later on, I was trying to think about which professor's groups I would join. One of the professors whom I talked to found out about the problem that I encountered about housing, and he was so nice—Professor David Pritchard at MIT. He was a professor in areas of atomic and molecular physics. That was one area I was thinking about pursuing, in addition to condensed matter physics with Millie Dresselhaus, and also high-energy physics with Professor Ting. Professor David Pritchard found out that I didn't have a place to stay so, he contacted two friends, a couple, who lived near Harvard University; still a distance from Harvard but closer to Harvard, far closer to Harvard than to MIT. The couple had a two-story house therewith a guest bedroom. It was like a separate unit. They said that I could stay there if I would just babysit their little boy whenever they had to go out. Through that arrangement, I was able to have a place to stay for the first six months.
ZIERLER: I hope your parents weren't upset that you were working. [laugh]
YEH: [laugh] I think they were just relieved I could find housing.
ZIERLER: Right. [laugh]
YEH: That was a beautiful area. It was in an upper-class area near Harvard University, and the couple were working at the Smithsonian. They both were astronomers, so kind of physics-related also. A very nice couple, a very cute little boy, and so I was lucky that way. Then, luckily, the second semester after the first six months, I was able to get the lottery, and got into the MIT dormitory, so I didn't have to spend a lot of time traveling, commuting every day any longer. Especially when I started doing experiments, it was hard to have to go back to babysit. [laugh]
Learning From the Carbon Queen
ZIERLER: Nai-Chang, what were the initial interactions with Millie Dresselhaus that led to her being your advisor?
YEH: I was darn lucky again. [laugh] That was the time when we (international students) didn't know very much about faculty members in different universities. When we tried to find university information, each university would send us this huge, thick telephone book-like booklet of their university, with all the departments, professors, etc., a gigantic book. Those were shipped. They were sent, really, by ship, literally [laugh], because it would be too expensive to get those things by airmail. I had to go through those jumbo booklets to get a sense of the universities. Of course, I knew which universities were top universities. But what I was trying to say is that I didn't know very much about these professors. [laugh] You couldn't have a website to take a look and figure out what they did and all that, so I didn't know very much. I didn't know who Professor Millie Dresselhaus was. But the physics department told me, "Oh, Professor Dresselhaus wanted to see you," so I was told. I think Millie was very supportive of women, and there weren't that many women students. I guess that was the one reason why she asked me to go to see her, and then asked one of the senior graduate students to show me around to find out if I may be interested in the research. I was very surprised, and so I said, "Oh, Millie Dresselhaus, who was she?" [laugh] I didn't know. I remember the day when I was told by the physics department. I was already staying with the couple at Harvard, who were working in the Harvard-Smithsonian Institute. I went back, and the lady asked me, "How was your day?" I said, "It was fine. I was told that Professor Millie Dresselhaus asked to see me, and so I am arranging an appointment to meet with her." The lady was like, "Millie Dresselhaus?" I said, "Yes." She said, "Oh, you are so lucky." [laugh]
ZIERLER: Yeah. Wow.
YEH: "She is so famous and all that." I was like, "Oh." [laugh]
ZIERLER: [laugh]
YEH: I didn't know anything. [laugh] I didn't know anything. It was my first trip going abroad. It was my first airplane ride [laugh] to go to MIT. I didn't know anything. It was lucky. I was trying to choose among three groups: Professor David Pritchard's group in atomic molecular physics, Professor Millie Dresselhaus' group in condensed matter physics, and Professor Sam Ting, a Nobel Laureate, his group in high-energy physics. Professor Sam Ting, I knew him because he was originally from Taiwan. He also asked me to consider his group, and he had showed me around to talk with his students. The reason why I made my final decision to work with Millie wasn't because how famous Millie was; all these three professors were extremely famous. Sam Ting was a Nobel Laureate. Professor David Pritchard was one of the top people in atomic molecular physics. My decision was based on bits of different reasons. For high-energy physics, I loved the physics but when I visited the research groups, I realized that each person—because it was a huge, gigantic team to do high-energy physics, particle physics. Each person was only working in a tiny area. That was the key reason why I felt I would not be very satisfied; I would not feel very satisfied with working on a small area of a big project. I'd like to understand everything and to take a bird's-eye view of what I work on. I did not want to be a little screw in a big machine, so that was the reason against particle physics and high-energy physics. I would consider doing experimental research, I should say, in part because MIT had fascinating experimental facilities, and it was nothing like what I could have ever had in Taiwan. With atomic molecular physics, at the time, that field was kind of a pretty old field in the sense that people were just trying to push for further, better experimental resolution of this and that in verifying theory and getting a little better resolution for better confirmation of certain theory. That was before the time when laser cooling was possible. After laser cooling became possible, there were so many exciting new developments, but those had not happened at that time when I entered MIT. For this reason, I felt that the research in atomic and molecular physics was not too exciting. Then with condensed matter physics, I realized that I could do things from making the materials, characterizing the materials, to designing experiments, studying the materials, and then, if I wanted, I could work on theory to explain my data. It's like I could do everything from one end to the other end, so that was the primary reason why I chose to join Millie's group. I felt that the research style would be more consistent with my personality. I liked to understand the big picture. I didn't want to just do a small part of a big project. I just didn't want to specialize in one little thing. I wanted a big picture. That was the key reason for my decision.
But there was another secondary reason. In Professor Millie Dresselhaus's group, she had a very international group, so I felt much more comfortable in her group. She had students from all over the world, and she ran a big group so she also had visitors from Germany, Mexico, Brazil, Japan [laugh], everywhere, including collaborators and postdocs. I felt more comfortable in that environment. Professor David Pritchard's group at the time, had I joined his group, I would be the only non-white student. [laugh] As you know, I was still trying to learn the Bostonian accent at the time. [laugh] Diversity played a secondary role in my decision. But primarily, I made my decision based on the topic, what agreed better with what I was interested in doing.
ZIERLER: Nai-Chang, when you first got to meet Millie, what was she working on at that point?
YEH: She was working on things called graphite intercalation compounds. Again, graphite, that's a form of carbon. She was the Queen of Carbon. She was working on graphite intercalation compounds, and also on carbon fibers. She was working in those two areas.
ZIERLER: What aspects of Millie's research were fundamental science, and what did she have her mind on societal applications, translating the discovery?
YEH: She didn't really pay attention to societal applications. She was just interested in the science. She would do things just curiosity-driven. But, of course, later on, carbon fibers, you know how useful that material is. After she got involved in the research of carbon fibers, she was able to make contributions to the applications of carbon fibers. But, otherwise, her research had always been just curiosity-driven, whatever science she enjoyed doing, I think. [laugh]
ZIERLER: How big was her group, and where did you slot in in terms of your interests and your areas of expertise?
YEH: I didn't have [laugh] a whole lot of expertise [laugh] at the time. I was just good at learning, I guess, and then I often had a little bit crazy ideas here and there. But I was just busy learning at the time. Because I didn't have a whole lot of experimental research experience, I had to learn a lot of things from senior graduate students, postdocs, visitors and visiting scientists. She had a huge group though—I don't remember—maybe like always 15 to 20-something people, and that was a huge group for a typical physics professor. But she was doing well, and she was famous already at the time, so she had lots of visitors who would come to collaborate with her. I was working closely with a senior graduate student who taught me a lot. But then, later on, when she had a Japanese visitor who came to stay for a couple of years, I also learned an enormous amount from that Japanese visitor. He went back to Japan and became a big professor at Tokyo Institute of Technology. When he was around, he was so kind and patient. I was just this young kid who did not have a whole lot of experience in the lab, and he would teach me a lot of things. I learned a lot from people, and so I learned many, many different experimental techniques, and I also knew how to make my own samples. Later on, I also collaborated with a Japanese theorist who was a research scientist at MIT in Millie's group. I collaborated with him to work out all the theories for the new data I took because I discovered a lot of interesting things. They were small discoveries, some interesting phenomena for some interesting materials that I synthesized. The physics was not fully understood so I collaborated with the Japanese theorist on doing the theory. My thesis turned out to be 400 and something pages long—
ZIERLER: Wow.
YEH: —because I did all kinds of experiments, and then I also had theory included. [laugh]
ZIERLER: Tell me about the laboratory environment in Millie's group. What was it like?
YEH: It was a little complicated. I can tell you but, at the time, I didn't quite like the lab environment. Millie was very busy. Millie was very nice, but she was super busy. Right after I joined her group, she was elected to be the president of the American Physical Society. She had all kinds of responsibilities. She didn't go to the labs. She didn't do experiments herself. Things were delegated to all these postdocs and senior graduate students. I did run into a few nice people. They were very helpful. I mentioned this Japanese visitor, and also, at one point, a senior graduate student. I learned a lot from them. But the lab was a little complicated because people had to work things out on their own. I was glad I was able to graduate fast enough. I was on the fast track. I finished my thesis. I was just 25, turning 26. I didn't have a master's degree. I just went straight. [laugh]
ZIERLER: Nai-Chang, finishing the thesis, what was beneficial from doing it so quickly, and what was difficult, given that you felt compelled to do it quickly because of the pressures you were feeling in the lab?
YEH: In the end, life always works out in a funny way that, sometimes, bad things can turn out to be positive things. I was motivated to work hard, and would do things fast. But, I must say one thing very nice about Millie Dresselhaus. She was always very supportive of her students. She would always send her students to at least two conferences per year so that her students could have good exposure, good practice about how to present their work. For each conference, before we went, she would rehearse us. She would give us rehearsals, and we would practice our talks, so we learned how to give presentations. That was one very nice thing about Millie. Also, we had regular group meetings when we had to take turns to give presentations. She was very good at educating us on how to present our ideas and sent us to conferences. Also, although she didn't work with me on experiments, when I had draft papers written, she would really go through the paper many times. [laugh] I felt like, at the time, she would use a red pen to write [laugh] many things down—
ZIERLER: [laugh]
YEH: —and drove me nuts. But that was how I learned how to write. I learned how to write. I learned how to give talks. I benefited enormously from the training. She was so supportive. Also, she even sent me to Italy once to a conference and, of course, she paid for the whole thing. She was extremely supportive. Coming back, I was trying to say about conferences. One of the last American Physical Society conferences I went—I think the last conferences I went before my graduation —that was 1987. It was right around the discovery of high-temperature superconductivity.
ZIERLER: Woodstock, the Woodstock conference.
YEH: That was the Woodstock conference, yes, in New York. I was there. I was there past midnight, listening to talks.
ZIERLER: Oh, wow.
YEH: It was electrifying. We were all thrilled. I was finishing my thesis at the time. That was my last year. It was in March, and I graduated that year in December. But that March, of course, I was getting close to the end of my PhD. But then I was giving talks on graphite intercalation compounds but not on [laugh] high Tc, and high Tc was so exciting. I went back, and Millie talked with me about my experience at APS. She asked me how I felt, and I was excited talking to her about high-temperature superconductivity. Then it was so interesting. She kind of looked at me, and she said, "Yeah, Nai-Chang, I think you will do well if you work on high-temperature superconductivity." Later on, she contacted IBM and Bell Labs for me to go and do postdoc interviews for positions on the subject of high-temperature superconductivity.
ZIERLER: Wow.
YEH: It was amazing. I often found that she seemed to know me better than how I knew myself at the time. [laugh]
ZIERLER: That's the sign of a great advisor.
YEH: Right. She was wonderful, really wonderful.
The Wonders of Graphene
ZIERLER: Nai-Chang, what would you say were the principal conclusions or contributions in your thesis research?
YEH: My thesis research, I actually worked on several different kinds of graphite intercalation compounds, and they were relatively novel. Let me explain what graphite intercalation compounds are. Let's think about these graphene layers in graphite -- graphite consists of many, many layers of graphene. Now, if you were able to intercalate certain elements in between the graphene sheets, then you could transform the properties of graphene because these elements can have charge transferred to the graphene sheets or have unique magnetic properties or other properties, and that can modify the graphite properties. You will make new compounds that have properties that are hybrid properties of graphite and the material, and sometimes completely new properties. Like, you could intercalate materials that are not superconducting into graphite, and then turn the graphite intercalation compound into a superconductor. I had also done that. I had intercalated potassium mercury into graphite. I had also intercalated cobalt and chlorine [laugh] into graphite. These intercalations created new electronic materials with new properties.
Now, you can also imagine when you intercalate certain elements into graphene sheets, you can have the elements form a layer. Basically, these elements that are intercalated in between graphene sheets and form their own layers, and they're separated from the adjacent layers of these intercalants through layers of graphene. You can have one layer of graphene separating the intercalants, or two layers of graphene separating them, or three layers or many layers. The properties, you can imagine, will be different because you're modifying things in a systematic way. You can have something that starts as a quasi-two-dimensional system to a three-dimensional system. Basically, I was exploring intercalating different materials to create new electronic materials, including superconductors, magnetic materials, and then study their optical, electrical, magnetic, and structural properties. That was my contribution. I worked on, primarily, three major different classes of intercalation compounds, and I worked out their detailed properties. Then, when we found very interesting electronic properties modified by these intercalants, I also had theory worked out to fully explain what I observed. My thesis title was The Electronic and Magnetic Properties of Graphite Intercalation Compounds. That was what I did.
ZIERLER: Nai-Chang, what aspects of the research did you see as refining or building on what Millie had already done in this field?
YEH: No, I was not refining anything because I made those samples. All of the measurements I did were all original. They were all original. Nobody else had done those before. She gave me a general direction to investigate certain types of graphite intercalation compounds, and so I made these compounds, and then studied them thoroughly from structural to optical to magnetic to electronic properties. Those were all original data, original contributions. These research experiences were very nice PhD training because, imagine, I started from making my own samples, and I had to figure out if they were good or not. I had to characterize them. I had to study their structural properties. Then I had to do optical experiments to study their optical properties. I had to conduct cryogenic experiments to study the magnetic and electronic properties. I even worked at National High Field Magnet Lab to run experiments going to very high magnetic fields to study the quantum oscillations of these materials. It was a very full, well-rounded training from structural to all these characterizations, and then to theory. It was very, very good training.
Also, by the way, Millie was also a very good teacher and lecturer; very good at lecturing. I learned group theory from her, and that was also very important for understanding the physics that I did better. I would say that I had very solid training, and I contributed original experimental data to the understanding of several types of graphite intercalation compounds because that was the whole point of a PhD thesis. If you don't get original research results [laugh], you are not supposed to graduate with a PhD degree. But I didn't build any specific instruments at the time when I was a graduate student. I would decide on the experiments I wanted to do, and then I would either use available equipment or buy new equipment if needed. My research in graduate school did not involve highly elaborate experiments, [unrelated conversation] and so I didn't really have to build instruments, although I did design my experiments and decided what experiments I wanted to do. That was how I did my graduate school experiments. But then I had to build lots of instruments after I came to Caltech. [laugh] What was important was that I had very solid training, and so I was ready to take on new challenges. I will put it that way. I wouldn't say that my PhD work was the most earth-shaking anyway, but it was very useful for me. I would say that most of my graduate students' PhD theses -- I feel as if I had gotten many PhD degrees [laugh] because, for every student who did a PhD thesis with me, I had to work so hard with him/her -- were more exciting than my own thesis because there research directions were chosen by me and all the experiments were based on my own design and then carried out by my students. [laugh] Anyway, I wasn't sure if I answered your question.
ZIERLER: Nai-Chang, in the graduate lab, what instruments were most important for your research?
YEH: What instruments? Of course, we had to do a lot of cryogenic experiments because we study interesting properties and materials that often only happen at low temperatures and, therefore, our cryogenics set up and vacuum systems would be critical. Vacuum systems are extremely important because if you have air-sensitive materials or if you want to go to a low temperature, you have to start with a good vacuum first. I would say that the cryogenics and vacuum technology would be the most experimental instruments at the time for me. But later on when I came to Caltech, I kept exploring and expanding my research into totally different new directions so that new technologies and instruments for nanofabrication and nanoscale characterization become needed. Mostly, a lot of the nano and other surface science techniques.
ZIERLER: What theories were relevant for your graduate research? In other words, were there any theories that provided guideposts to give you a sense if you were on the right track?
YEH: As physicists, we operate in a way that there are certainly physical principles that cannot be violated, and certain things could just go with what people had done in the past and then you could figure out whether there might be a trend. But, generally, what's the guidepost? The guidepost would be that if you would come up with a theory, this theory would have to be able to consistently explain all aspects of experimental findings. Otherwise, the theory should be thrown out of the window. We shouldn't use theory to dictate experiments. Theory only provides kind of a guideline that gives us an idea of which direction we may want to explore. But then when you observe certain experimental phenomena and want to come up with some explanation, you would have to formulate your explanation into rigorous expressions. Then you must verify whether what you have conjectured can self-consistently explain many different aspects of the experimental findings. I would say, often, theory gives us some ideas of the research direction to design experiments. But then experiments are the ultimate tests for the validity of any theory, any good theory. That's how I view it. Physics is empirical science. If a theory does not agree with physical phenomena, then the theory is, at best, math or philosophy. It's not physics.
ZIERLER: [laugh]
YEH: Also, I remember a very famous chemist, also a friend of mine. He's a professor at Princeton University, Professor Bob Cava. He told me one thing that left strong impression on me. When I was an assistant professor, he came to Caltech for a visit. He was already a very famous scientist. Then he asked me one question. He said, "Nai-Chang, when you design experiments, you have some theoretical ideas. Would you be very happy if the experiments came out in agreement with your theoretical prediction?" I said, "No, I would be very upset because I would have learned nothing new."
ZIERLER: That's right.
YEH: "It would be all obvious. I could not have possibly done anything that's exciting." [laugh] He fully agreed with me. I was so happy that he asked me that question. We were in perfect agreement. [laugh]
ZIERLER: It's good to be wrong in science.
YEH: Yeah, and it's so important to be able to keep observing new things, and then pushing the limit, and creating new knowledge. It's always the case when we have new findings, I learn new things from my experiments.
ZIERLER: Nai-Chang, last question for today, besides Millie, who else was on your thesis committee, and what was the defense experience like for you?
YEH: Let's see. There was another professor on my committee. There were two other members on the committee. Actually, I don't fully remember whether there were two or three more. [laugh] But I remember two more of them. One was Professor Patrick Lee. He is a theorist. He is visiting Caltech these days half a year per year. He was already extremely famous at the time. He was a very, very famous theorist. Of course, he continues to be very famous. [laugh] He is a guru in theory. Actually, I have started collaborating with him recently. There are some interesting phenomena that we observed from our experiments, and there are some interesting theory behind it. We found some exciting phenomena, and so he and a postdoc were working on the theoretical aspects. He was on the committee. There was another professor. I think he was actually a research scientist at the National High Field Magnet Lab at MIT at the time, Dr. Agarwal, who was an experimentalist specializing in optics. No, my thesis defense was—I honestly didn't remember a whole lot anymore because it was just that everything was done, and I just gave a presentation, and took some questions. That was pretty much it because I already did so much work [laugh] at that time. I think it was not very eventful for me, although I was happy when it was done. But there was nothing very exciting [laugh] for that exam.
ZIERLER: Nai-Chang, on that point, next time we'll pick up the opportunities that you pursued after completing your graduate research at MIT.
[End of Recording]
ZIERLER: This is David Zierler, Director of the Caltech Heritage Project. It's Tuesday, August 23rd, 2022. I'm delighted to be back with Professor Nai-Chang Yeh. Nai-Chang, it's so nice to be with you again. Thank you for joining me.
YEH: Thank you for having me.
Superconductivity at the Woodstock Conference and IBM
ZIERLER: Nai-Chang, today, we're going to pick up right at that moment when you're finishing up at MIT, and you're considering next opportunities. First, did you get any advice about whether you should do a postdoc or should you go right into considering faculty appointments?
YEH: Actually, in physics, it's almost 99.999% [laugh] that you must do a postdoc before you can be considered for a faculty position. Naturally, I was looking for a postdoc position at the time. Then I had my conversation with Professor Dresselhaus, so I asked her where I should do my postdoc. At the time, the best two industrial research labs were Bell Labs and IBM Watson Research Center research labs. I went for interviews in both places. But, for some interesting reasons, I didn't know exactly what went behind. My advisor at the time told me that IBM would be the best place for me, IBM Watson Research Center. She said I would fit in very well. I went to IBM Watson Research Center. I went for a job interview for a position to do research on the topic of high-temperature superconductivity, although my thesis had nothing to do with that. But it was a hot area at the time. I think I went for an interview in the winter of 1987, and I graduated in January 1988, so I went straight to IBM Watson Research Center after graduation. When I did the job interview, I got an offer right away. It was a very, very exciting time for high-temperature superconductivity, so I was excited about the opportunity of doing something that was very different from my thesis, but it was a very exciting area. The fun thing—I don't know if I mentioned to you last time—was that when I went to this "Woodstock conference of [laugh] physics" at an APS meeting in 1987, in March, and I went back and I was talking to Professor Dresselhaus about how exciting high-temperature superconductivity was, and then she looked me, she said, "Nai-Chang, I think you will do great in that research area." I think what she did subsequently was that she contacted one of the people she knew at IBM Watson Research Center—I think. That was my guess. She introduced me to them, and they contacted me for a job interview. I went there. On the same day, I got the job [laugh], and I came back to finish my thesis. In retrospect, I think Millie did a lot of good things behind my back [laugh] without telling me. She wanted me to work hard towards what I would like to get. But I'm grateful. Anyway, I went to IBM Watson Research Center in January 1988, right after I finished my thesis defense. I stayed there from January 1988 to August 1989, before I came to Caltech, right before I came to Caltech.
ZIERLER: Nai-Chang, if I may ask, what aspects of your thesis research were relevant in this shift to high-temperature superconductivity, and what was really brand new that you had to learn from the beginning?
YEH: High-temperature superconductors, they were totally different animals. [laugh] Those materials were so exotic that people didn't quite understand what the heck was going on for many, many of the properties. However, the experimental techniques I had learned as a graduate student, all of the techniques, were applicable to investigating high-temperature superconductors. The subject and the physics may be quite different from what I did for my thesis because superconductors are very, very different from other materials, especially high-temperature superconductors are even fundamentally different in many ways from conventional superconductors, although there are some similarities. There are common principles, but some other properties are totally different. I had to learn fast. Also, the field was developing so incredibly fast. Every day, you would see lots and lots of new papers, and you had to filter the new papers because a lot of them could be junk [laugh] because people were rushing for publications. They may not be sufficiently rigorous, or they may not understand what they were really doing because the system was so new. That was the challenging part, that I had to learn the materials, learn about the system. It's such an exotic system and, actually, nobody truly understood [laugh] the system at the time. But I had to learn and then to figure out how to do creative experiments because it was a worldwide frenzy in conducting the research. Many countries and major labs were actually investing a huge amount of resources into doing the research at the time. If you wanted to stay competitive, you would have to be very creative and different from others. You would have to think original ideas, and do unique experiments, or figuring out unique properties of the system. It was an exciting and challenging time, but I love doing difficult things, so it was not bad. [laugh]
ZIERLER: Nai-Chang, the focus on industrial research labs, and Millie's advice to consider Bell Labs and IBM, is that to say that academic institutions were not yet doing cutting-edge research on high Tc?
YEH: Yes in some sense, at the time, that was the early days of high-temperature superconductivity. As you may know, for decades in the US, some of the very best research was done in industrial research labs. As you know, Bell Labs created lots of Nobel Laureates, and so did IBM. At the time, industrial companies were willing to invest money into cutting-edge fundamental research that may not have anything to do with immediate applications. That was the time. In industrial labs, they had more resources than most universities, at the time. But there were also national labs, and national labs had a lot more resources than universities, so they were also doing good research. But, really, for decades, the very best cutting-edge research, at least in my field, in condensed matter physics, was often done at industrial labs at the time. But when I graduated, I would call it the end of the golden period of industrial research, because industrial companies started feeling that they were facing fierce commercial competitions worldwide. The management started realizing that they didn't want to invest so much money into fundamental research. They felt that maybe those things should be transferred to universities, and they should be doing more profit-driven research.
Then, of course, you know that the monopoly of Bell Telephone as the telephone company ended around that time. Bell Telephone Company, basically—I forget what it's called [laugh]—they basically got disassembled into several small companies, smaller phone companies. Those smaller companies could not support Bell Labs anymore. IBM used to be the dominant company servicing all the major computer facilities for major corporations. But then when PC started coming along [laugh], the advantage that IBM had in computers diminished, and so IBM also tried to make PCs. Anyway, IBM was no longer this big giant in the computer business. Those circumstances were the consequences of technology evolution, and so these companies could no longer have this huge profit to spare a little money [laugh] for fundamental research. Shortly after I finished my PhD and came to Caltech—I came to Caltech in 1989—shortly after that, I think, in the 1990s, Bell Labs basically let go most of their research scientists. IBM, also around the same time, stopped hiring new research scientists—not entirely. But they used to hire a lot of young research staff members. At the time when I started there, I could sense that the tide was changing rapidly worldwide for industrial research companies. At the time, there was still like GM and General Electronics, GE, GM, Exxon. Those companies also had wonderful research labs. Now, they're all gone. You don't have any of those. IBM still has a good research lab, but it's much smaller in scope. Of course, we don't have Bell Labs anymore.
ZIERLER: Nai-Chang, as you alluded to, this was really the end of this period of industrial research in the United States. In choosing IBM over Bell Labs, did you appreciate at the time that between the two, IBM was still in a stronger position or that the coming breakup of Bell Labs was already on the horizon?
YEH: It was not so clear at the time, and the reason why I chose [laugh] IBM over Bell Labs was actually something Millie already foresaw. [laugh] She said, "Oh, you will fit in at IBM much better." I think the reason was that IBM was a much smaller lab, and it was kind of more friendly. People were more friendly. Whereas at Bell Labs, it was really cutthroat. People were extremely competitive. I think it might be from that perspective that Millie already said, "Oh, Nai-Chang, you'll fit in [laugh] at IBM much better." When I got a job, she said, "Oh, yeah, you don't have to go to Bell Labs. Just take the offer." [laugh] I took her advice.
ZIERLER: Nai-Chang, what were your early impressions of IBM? What was the research culture like when you first joined?
YEH: When I first joined, it was very nice, in the sense that it was not a very big place, and then there were the people—I joined a department called physical sciences department. It was nice in the sense that people interacted well, including theorists and experimentalists. The director of the physical sciences department knew all the young postdocs in his department, and would talk with us casually to find out how our research was going. There was no hierarchical structure. It was almost like all people interacted just as peer scientists. That was the aspect that I really liked. I worked with two managers, and both of them were very good scientists, and they were incredibly supportive. They were very nice, and then very accommodating because I wasn't a typical young person who would just take orders [laugh] or something. I always had my own ideas, and sometimes if I had different scientific opinions from my managers, I would argue with them very fiercely. [laugh] They were like looking at this young kid very patiently. They were really some of the nicest people. They were completely understanding, and they didn't take anything personal. They knew I was just passionate about what I did, and if I agreed with them, I would love the idea and go with it, and if I did not agree [laugh], I would argue. [laugh] It did not matter. We also had these Nobel Laureates coming through the labs, and I would argue with them too. [laugh] I would discuss and argue with them too. Science is science, right? There's no authority other than truth itself. That was my attitude. Therefore, I would go with whatever was right or whatever I believed was right, but I could be convinced too. That was a very healthy environment because that was how you would feel comfortable to bounce ideas around, and you would not be bound to any authority just because of the position or age or seniority. You're just free to pursue the truth. That was a good time. I do appreciate the time and the support I got at IBM.
ZIERLER: Nai-Chang, as you explained, in the late 1980s, with all of the excitement around high Tc, was IBM's approach purely in the basic science mold, or were they thinking at that early juncture about possible applications and even turning a profit for IBM?
YEH: They were looking at both. Fundamental research at the time, actually, was the emphasis—because the material was so new and there were so many exotic and unanswered things, the beginning effort was mostly towards understanding what the system was like, and how to control them, because you need to understand things so that you can truly harness the properties and turn things into applications. But there were also considerations for applications, like making devices, etc. People were working on different things. At the time what happened worldwide was like anybody who would do any kind of scientific research would just jump into high-temperature superconductivity. They would take the material, and just studied it with whatever methods that they used to be good at, and then observed their experimental findings to see if there could be something new. [laugh] As you can imagine then, IBM, of course, at the time, they also made devices like superconducting Josephson junctions. In fact, before high-temperature superconductivity came along, IBM wanted to develop new Josephson-junction computers based on low-temperature superconductors in 1970's, but it was just too difficult technically and too expensive. In the end, IBM terminated the project, but they did work on superconducting computers for some time. So, IBM had strong foundations for doing superconducting devices and materials.
Then, of course, the 1987 Nobel Prize in Physics was awarded to two IBM scientists [laugh] in Zurich because high-temperature superconductivity was their discovery. IBM had a history of doing superconductivity, both in terms of fundamental pursuit and applications. Actually, there was a fun episode during the time because superconductivity research was a worldwide fierce competition, and so people were paying close attention to developments in other countries to make sure they wouldn't miss any big news elsewhere. Then one day, one morning, I came into my office, and a research staff member at IBM brought me a piece of news published in some newspaper they'd printed out elsewhere. It was completely in Japanese. [laugh] He and others at IBM could tell there were some chemical formulas, and they knew something important happened that morning, but none of them could read Japanese. I could read some Japanese although I couldn't speak Japanese, and so I just took a look at the news article, helped translate the article [laugh] into English on the same day, and then distributed it around the IBM Watson Research Center because it was important. It was an important new material that some Japanese scientists successfully synthesized, but they purposely made the news only in Japanese so that it would not be [laugh] immediately captured by everybody, by all the competitors around the world. It was a funny time [laugh], anyway.
ZIERLER: Nai-Chang, as a woman in science, did you experience any difficulties at IBM at a time when perhaps not many women were working at your level?
YEH: I had one unpleasant experience. I don't think it was because I was a woman. It was just because I was young and naïve. It was long in the past, but I can mention a little bit of it. I won't mention the names of people. At the time, there was a very, very bright scientist, a theorist, at IBM. He was a young theorist. He was already a permanent research staff member. He came up with an idea. It was called "vortex glass". The concept was developed to describe the high magnetic field properties of high-temperature superconductors, and it was not like the behavior of conventional low-temperature type superconductors. High-temperature superconductors are Type-II superconductors. I won't elaborate. However, the behavior of high-temperature superconductors don't seem to behave like conventional Type-II superconductors in a magnetic field.
This young theorist came up with an idea to explain the unconventional behavior, but he needed to get an experimentalist to figure out experimental approaches to verify his ideas. What was funny was that I was just a young postdoc, and then the director of physical sciences department called me to his office one day and told me about this idea of this young theorist. He said, "Nai-Chang, you're the only one who can think about the right experiments to do to prove the idea." [laugh] I was like, "OK." I went back, and I was thinking hard about it for a day or two. Then I figured out a series of experimental approaches and data analyses that I thought could rigorously verify the idea. I went to this young theorist's office. I was so excited. I told him about my ideas, and he was so happy that he repeated everything back to me to be sure that he did not miss anything that I proposed. Then, naturally, because the experimental signatures that I figured out were promising to verify the concept of vortex glass, I wanted to do the experiments myself. I was excited. I started conducting experiments using my own ideas on some of the high-temperature superconductors. Meanwhile, my ideas were spread around the Watson Research Center by the young theorist, which I didn't mind so much at the time because I always got excited about telling people my ideas. The ideas were spread around, and there was another research group led by a senior member who decided to do the same experiments that I proposed on different systems, different materials. Then both groups, both me and the other group, had all these experimental results collected, which was considered a big deal at the time -- I'm trying to recall what happened chronologically. Then the director of the physical sciences department suggested that maybe the two groups should discuss and maybe publish the findings jointly, and the director basically said, "Nai-Chang should be the first author." That senior researcher was absolutely against it. He wanted to be the first author, even though all of the ideas came from me. He did his experiments, I did my own experiments, and we investigated two different systems and found similar results. He was absolutely against having me be the first author. He just would not budge.
In the end, we decided to publish separately so that my group published our own work and the other group published their own. But then while I was writing up the paper, I started noticing experimentally there were things that I had concerns. I started having concerns of the full rigor of my conjectures, of my approach to verify the ideas. I realized that it was like, if you have those conditions, you should have these results. But if you have these results, it does not necessarily mean that those conditions exist, which is basically an issue of sufficient or necessary conditions. Then I realized there were some issues that I was concerned about, and so I decided to do more work. I had to figure out even more rigorous approaches to verify the vortex glass idea, because I thought that with the experimental signatures that I had developed then, I could extrapolate some universal numbers associated with the vortex glass system. But after I did more experiments, I realized the numbers I extracted were not universal at all. That raised concerns in my mind, and I said, "OK, something else, some other approaches must be taken in order to extrapolate a truly universal component, which was not what I have extrapolated." I kind of told people about it and decided not to rush publication. But that other senior scientist, he grabbed on my ideas, ran with them despite my subsequent warning about potential problems, and rushed to publish a paper. It was a big splash in the research community of high-temperature superconductivity. What happened was that he made a huge fame out of it, and my original contributions were nowhere mentioned. I was not even in the acknowledgement. But that wasn't the worst. The worst thing was that I realized my own ideas were not quite accurate, and then the whole world started doing experiments using exactly the recipes I put out [laugh] there. I was like, oh, my god. [laugh] Oh, my god, see what I have done. My immature ideas—I only thought about things for two days [laugh], and then I went to present it. But I would not have known the subtleties until I actually did very rigorous experiments and many experiments. Then I realized there was the issue of reproducibility with the incomplete experimental approaches that I proposed. Meanwhile, this guy got all of the fame, and then I got multiple job offers, so I left IBM and came to Caltech.
When I started at Caltech, I started much more rigorous experiments to basically do research on verifying the idea of vortex glass all over again. Then, in the end, I was able to, but it was much harder. It took much harder experiments, all kinds of different approaches, to verify the universality of what we were pursuing. [laugh] The ironic thing was that the whole world was misled by my own bad ideas [laugh] earlier but published by somebody else. Initially, when I tried to publish my new and correct results, I was running into obstacles all the time. [laugh] In the end, it took some years for things turned around and, fine, OK. That person already passed away, so I don't want to mention [laugh] any names or anything. But that was one unpleasant experience. I had a lot of supportive senior members at IBM. But then I also ran into someone who just wanted to run over me [laugh], and just took my stuff, and turned me into a pancake. [laugh] But I don't think it has anything to do with the fact that I was a woman. It had everything to do with the fact that I was a young scientist, and I was too naïve. I just told people everything that I knew. I thought that's how you do science. You exchange ideas, and bounce things back and forth. But I learned to be a little bit more cautious because not everybody is noble. [laugh]
ZIERLER: Nai-Chang, what was the overall research goal with regard to high Tc at IBM during your postdoc years?
YEH: High Tc, there were many fronts that you could consider. There were just the fundamental research aspects. There were these things about interesting phenomena. But then there were these potential applications. Then there were these areas about understanding exactly how high Tc becomes high Tc, and that's still not solved till this day, although we have much better ideas now. We have general ideas, but it's not rigorously solved by theory right now. At the time, IBM was exploring all those fronts. Of course, they were also trying to make superconducting quantum interference devices, and those could be useful for many things. For instance, those are called SQUIDs and Josephson junctions, and those things can be used for qubits. But, right now, high Tc qubits are not good. If you want to make good qubits for quantum computation, you have to still go to low Tc qubits, and there are real physics reasons why. Just generically, high Tc qubits are much noisier, just because they have special properties that are different from low Tc, low-temperature superconducting materials. At the time, IBM was basically exploring all areas, because it was an open field, so people were trying to see what could come out of the broad range of research. Ideally, then, you narrow things down on the most promising things.
ZIERLER: Nai-Chang, was anyone talking about quantum information at IBM during those years?
YEH: No.
ZIERLER: Too early?
YEH: Yeah. People were not thinking about quantum computation, qubits, and all that. As you know, a lot of that came from Caltech. [laugh] But they were looking into using superconductivity for computation but more in the conventional sense and for communications, for technology, but mostly in the conventional ICT, information communication technology, that kind of approach. They were not thinking about making qubits or quantum entanglement and those things, no, not at the time.
ZIERLER: What would you say was your most significant research at IBM?
YEH: I did two important things. But one was more materials related. These high-temperature superconductors are oxides. It turns out that together with my managers, we figured out the best way of controlling the oxygen content in these materials so that we could optimize the superconducting properties of these materials. That was the material aspects. But the other important thing was really related to the vortex dynamics. Those are associated with the fact that superconductors have interesting properties in the presence of magnetic fields. When you apply a magnetic field to Type-II superconductors, magnetic fields at low fields will be completely expelled from this material, so that's known as the Meissner effect, and that's there in high-temperature superconductors also. But then when you keep raising the magnetic field, at some point, magnetic fields will start penetrating into the material, but they will nucleate into flux quanta, and so there will be these quanta of flux all over inside the material. You can allow some field to get into the superconductor. The magnetic field in the superconductor is completely inhomogeneous. They show these periodic patterns of flux distribution. These quanta of flux will not move at low temperature. But when you raise the temperature, then they will start moving. They will behave almost like atoms, and so it's like you can raise the temperature, the atoms would start oscillating, and eventually start moving, then you lose your solid crystal. Similarly, you have this vortex lattice structure, and then when you raise the temperature, they would start moving, and turn into like a liquid phase equivalent. In high-temperature superconductors, there are these impurity sites that can pin these vortices so they don't easily move around. You want to optimize the pinning in order to raise the superconducting critical current of the material. You want the vortex lattice, vortex glass not to melt because, once they melt, quote, unquote [laugh], you actually have resistivity. But before they melt, it's a truly zero-resistance state, and so you can use the material for zero dissipation. Anyway, I was studying how different impurities, defects, and the intrinsic properties of high-temperature superconductors interact with this magnetic flux. I studied the phase transitions of this vortex phase from the vortex solid and glass phase to a vortex liquid phase. That was my major contribution at the time.
ZIERLER: Nai-Chang, if you had wanted to, when your postdoc was wrapping up, could you have joined the permanent staff at IBM? Was that available to you?
YEH: I thought so. I didn't really pursue because when I went around for job offers, I got seven. [laugh] IBM were very happy for me. Actually, oh, yeah, let me think. My manager at the time did ask me if they should start the process of getting a permanent position for me. It was a funny thing because I knew all along, I wanted to become a professor in a university. But my original career plan was like, back to a few decades ago, my original career plan was that, oh, maybe I should be a research scientist in an industrial lab, one of the top industrial labs first so I don't have to worry about raising money and all that, and I will not have to worry about teaching. Then I could establish myself. Then I will move to a university to become a tenured professor so I will not have to go through [laugh] the tenure process. That was my ideal dream at the time. But the thing was that it just so happened that I was applying for jobs for fun. I was just practicing, because I was only a postdoc for one year. Typically, for the physics field, people have to do minimum two years, and typically three to five years of postdoc before they will go for a faculty position.
After about one year, I saw all these job announcements, and I said, "OK, let me try to practice job applications," so I sent out my CV. I didn't expect that I would even be invited because as far as I knew, all those places would be receiving like maybe up to hundreds of applications. In the end, they probably would just invite five or six people for an interview. When I got a bunch [laugh] of interviews, I was like, "Ah [laugh], what happened?" [laugh] I went for job interviews, and I was young and naïve; didn't know anything of anything. But then I got seven job offers, and it was like, OK. [laugh] Anyway, I was originally thinking about staying in the industry, but when these job offers came, they were from top places: Caltech, Harvard, Columbia, and some other schools. I was like, "If I stay at IBM, later on, would I have the same opportunities to go to these schools?" I was asking myself about that. Then in the end, I said, "OK, let me just give it a try." [laugh] I was thinking these are my dream places, and if I give up the opportunity now, I may not get the opportunity again, because I cannot predict how well I would do as a research scientist [laugh] at IBM at the time, so how would I know? I said, "OK, I will just pick one of the schools [laugh] and go to a university."
ZIERLER: Nai-Chang, just a personal question. At this point in your life, did you consider moving back to Taiwan? Were there any opportunities there that you would've considered?
YEH: No, not at the time because Taiwan was still not so advanced in science and technology. It was only much later. At the time, had I wanted to go back, I could have. But it didn't even occur to me because the research environment was just too different, and I wouldn't want to give up the opportunity of doing cutting-edge research in the US, and interacting with people who are at the very forefront of research. It didn't even occur to me. But I knew, had I wanted to go back, I could have.
ZIERLER: Of course, I would have to ask the individuals and committees who were recruiting you and giving you these job offers. But, from your perspective, why was the field that you were working in at that point just exploding with all of these job openings, and what was it about your research in particular that you think resonated so well that all of these faculties wanted you to join them?
YEH: [laugh] It was the field of high-temperature superconductivity. They wanted to hire someone who was doing research in high-temperature superconductivity. That was one key reason. But then many people were doing high-temperature superconductivity. Then I went for job interviews. I was completely young. I was just absolutely 100% engaged in scientific interactions with people. That was just the way I was, so I was not even thinking about other issues. I was just so engaged in discussions. I think that maybe one reason why people really liked me was because they saw my passion, and they also knew I was fearless. [laugh] I was too naïve. [laugh] I think that was probably the reason.
Recognizing Condensed Matter as Real Physics at Caltech
ZIERLER: Tell me about your initial visit at Caltech. What was it like?
YEH: Actually, Caltech was my second or third job interview. My interview at Harvard was even more interesting. At Caltech, it was fine. I came and, at the time, Caltech physics did not have anyone doing full-time condensed matter physics research because Caltech for a long, long time—it was partially because of some of the senior members, the Nobel Laureate—what's his name?
ZIERLER: Murray Gell-Mann.
YEH: Yeah, Murray Gell-Mann. He had this very wrong impression of condensed matter physics. He thought condensed matter physics was nothing but just perturbation theory.
ZIERLER: He called it "squalid state."
YEH: Yeah, it was totally false because, in the end until this day, a lot of major theoretical breakthroughs in high-energy physics, or something, actually they borrow ideas from condensed matter physics. Condensed matter physics is actually much harder even than high-energy physics in many ways because we're dealing with this many-body interaction of this huge number of particles. Sometimes, with strong interactions, you have to completely change the physics paradigm to describe the ideas. We use quantum field theory all the time, we use topological field theory all the time but not at the time when Murray Gell-Mann was around. He didn't see that, but it was his ignorance. [laugh] He was always against—he just didn't think condensed matter physics was anything important. At the time, the physics department at Caltech emphasized particle high-energy physics and astrophysics. There was only Professor David Goodstein who did a little bit of condensed matter physics, but he was the vice provost at the time so he was only doing half-time research. Then high Tc came along, and Caltech was like, "Uh-oh." [laugh] At the time, there were condensed matter physics-related activities but they were all in the engineering division. They were all in applied physics or electrical engineering at Caltech, not in physics. When high Tc came along, people started thinking differently at Caltech. They first hired a famous theorist Michael Cross from Bell Labs to Caltech. He was doing soft condensed matter physics and statistical physics type of theoretical work. But then they still had no full-time experimentalist doing any condensed matter physics research in the physics, so they started searching for someone to come to Caltech.
ZIERLER: Nai-Chang, do you have a sense, on the faculty, among the physics faculty at Caltech, who was driving this effort to modernize the department, to get more experimentalists, to get more condensed matter researchers to join?
YEH: I think there was consensus at the time. But then the chairman of the committee at the time was Mike Cross, Michael Cross, because he was the first theorist full-time hire into it. But, actually, if you think about it, Feynman, Richard Feynman, did a lot of important things in condensed matter physics.
ZIERLER: Yes.
YEH: But he already passed away. Had he been around, it would be truly, truly fun, I think. For what I did, I could have wonderful interactions. But, anyway, I think there was consensus at the time, but I would think that Mike Cross, of course, played an important role. I also believe David Goodstein and another professor, Tom Tombrello, I think they were the people who were really actively driving this push for condensed matter physics. That's what I think. My experience was that I came, and I gave a talk, and I had lots of discussions with everyone, just like my other interviews. I was always very engaged [laugh], and so would argue and discuss and then whatever. I remember I was impressed with the beautiful Caltech campus. It's very tranquil. Then I interacted with people. I went back to IBM. People asked me, "Nai-Chang, what's your impression of Caltech?" I said, "Actually, people are very nice, although they are a little weird." [laugh]
ZIERLER: [laugh]
YEH: They were different from the Harvard people. [laugh] But it's California. I actually had a very good impression of Caltech at the time.
ZIERLER: What did you talk about in your job talk at Caltech or anywhere else that you were considering? What were the big themes that you emphasized?
YEH: At the time, I was talking about the vortex states of high-temperature superconductors, what I just mentioned earlier. It was associated with this idea I created. But then I realized it wasn't rigorous, so I basically explained my ideas and then said how it should be done more rigorously. Then, of course, some of the things I really completed what I said [laugh] that I was going to do when I came to Caltech a couple of years after I set up my lab. Then in a few years, I was actively pursuing what I considered as the rigorous experimental approaches to do things right. I was talking mostly about vortex physics of high-temperature superconductors at the time.
The Culture of Support for Junior Faculty
ZIERLER: Nai-Chang, because your recruitment was part of a broader effort to build up and diversify the program in terms of the kinds of things physics professors did, were you hired on the basis that there would be others like you, that you would have others in the department to collaborate with, or were you understanding that this was going to be a project that you would take on mostly on your own?
YEH: I knew they were hoping to hire more people, but I couldn't count on that. Anyway, a tenure process is such that I have to prove myself, so I was always under the understanding that I had to do it mostly on my own. Actually, interestingly, one of the key reasons why I chose Caltech over Harvard at the time, although I was also very impressed by Harvard -- As you know, at the time, Harvard already had many major theorists and experimentalists all in condensed matter physics, unlike Caltech. When I came, I knew I would be doing everything on my own mostly. But at Harvard, there was this long tradition—and also, at the time, there was this very, very famous professor, Michael Tinkham, who was a guru in superconductivity. I went there. I really loved the intellectual environment. But then I was thinking about one thing. I was saying to myself, "OK, if I go to Harvard, then whatever I do, people may end up crediting the senior professor, wondering how much original work may have come from Nai-Chang or whatever." I said, "OK, if I go to Caltech, then, one thing, it's California. [laugh] The other thing was that if I am not good enough, it would be my own fault not getting tenure. But if I do anything, it would be 100% to my own credit." [laugh] I was young and naïve and [laugh] completely fearless, and stupid maybe. [laugh] Anyway, that was one key reason I convinced myself to come to Caltech.
ZIERLER: Nai-Chang, what about, to the extent that you appreciated it when you were making this decision, Caltech's reputation for supporting its junior faculty, and that tenure was something that everybody wanted to see happen, as opposed to other institutions where that may not have been the case?
YEH: That was one thing I was told. But then the department chair at Harvard, who is a very, very famous theorist whom I absolutely admire, he was trying to tell me that Harvard has changed. [laugh] He told me, he said, "You are very scholarly, and I believe that you will have a very, very good chance of getting tenure. We're no longer known as a place kind of hiring junior faculty members as effective senior postdocs [laugh] or something to do teaching." He felt strongly that I could make it at Harvard for the way I was conducting research. I knew he was sincere. He meant it. It wasn't like he was trying to recruit me and, therefore, he lied to me or something. That was a person I absolutely admire. It was very hard for me to turn him down in the end. I actually visited both Caltech and Harvard twice before I made my decision. I told you I had seven job offers, and then I narrowed things down to two, so I visited both places twice before I made my decisions. It was very hard for me to turn Harvard down because I really appreciated the intellectual environment. But I was a little rebellious. I wanted to be doing things on my own. [laugh]
ZIERLER: [laugh] Nai-Chang, we talked about this previously, and you mentioned some excellent advice you got from Millie. When you joined the faculty at Caltech, were you aware of the potentially historic nature of your appointment, both possibly to become the first tenured woman professor in physics, and also possibly becoming the first tenured Asian woman professor for all of Caltech?
YEH: I didn't even think about those things. To me, it was just research and the environment and, of course, students. Those were the important factors, so I did not even think about those other things. It never occurred to me until I came. [laugh]
ZIERLER: It probably helps explain why you achieved those things, because you did not think about them so much.
YEH: That's right. That's right, actually. Also, interestingly, I was always like that. But Millie also emphasized that to me after I came to Caltech. Millie became a Caltech trustee. She was on the board of trustees, and so she would visit Caltech every now and then. I would meet up with her to talk about research or something. Then, I remember, she was also telling me—and I was doing exactly what she said already before she told me. But it was interesting to see she thought the same way. She said, "Nai-Chang, you should just concentrate on your research, and try to do the best, and not to get involved in a lot of other things." What she meant was like too many other activities to advocate for women or something. She said, "Those are the jobs of we senior people, and you, as a junior person, just concentrate on research. Let your research prove yourself." That was her advice, and I was that way because, really, you only have 24 hours a day [laugh], and the bandwidth is limited. When I dived into research and worked hard, I just didn't have the time and energy to do these other things. But, of course, much later when I—she also said, "When you become established, then that's when you can help people."
ZIERLER: Tell me about setting up your research when you first got to Caltech. What was that like?
YEH: It was extremely hard because I had nothing. I came, and then I was given just a totally empty lab: four bare walls. Nothing. Also, I was just too young and naïve, and didn't have any real advice from senior people at the time. I was given a start-up package, a start-up fund. The start-up package was for buying some equipment, initial equipment, and then supporting students for the beginning. Of course, then, I had to write grant proposals to get money. Then, of course, for all the schools I applied, I was given a start-up package that was for equipment and student support, and then there would be some separate funds set aside for renovating the lab because there were different lab requirements for different instruments. But, at the time, there was miscommunication for my startup at Caltech. Of course, I was too young. At the time I came, I was given a start-up package so that I had that account number, so I started buying equipment and hiring students. But that was a limited amount of money, so it would be used up in a couple of years. I knew that I had to start writing proposals. But then I also had to renovate the lab. The lab didn't have all like low-noise power, or water, a chilled water supply, etc. I needed to renovate my lab. But, at the time, I didn't know. I thought there was something set aside, so I called up the Physical Plant to say that I needed these things done. Then I said I'm a new assistant professor in physics, so I thought they had an account number for me. But apparently, the Physical Plant was not given an account number for my lab renovation. The division administrator had an account number but I didn't know, and he never told me. I called the Physical Plant, and then they said, "What account number?" I only had one account number, and I was like, "Oh, my god, what's this? [laugh] I need to have this money for equipment and students, and now I have to use that for plumbing?" I was like, oh, my god, that was awful. I started cutting corners and trying to figure out how to do things. Luckily, at the time, the division also gave me a staff engineer for two years. They supported the staff engineer for two years to work with me, and then after two years, I would have to support him. He was absolutely skillful, and so in the initial two years he was able to provide critical help for me to set up my lab. His name was Nils Asplund. We actually just had a memorial for him this past Saturday. He passed away recently in his 80s.
But, at that time, he was just absolutely skillful, and he was so helpful and supportive of me. He helped me do a lot of the plumbing [laugh] so I could save some money. Then, also, I didn't have enough money to buy very expensive instruments, so I built a lot of experimental components on my own. I designed things with him, and he was just absolutely amazingly skillful. He built all kinds of things for me, so I was able to get by with this engineer's help. But, of course, the engineer was supported the first two years by Caltech and then, subsequently, I got grant money, so I supported him. We worked together for like almost 15 years until then he went to work for the Kavli Nanoscience Institute afterwards. But the initial part of setting up my lab was hard. It was hard. Then only after I became a full professor [laugh] and I found out that, actually, there was money set aside for me for renovation of the lab, but nobody really told me. I didn't know. At the time, I was the first woman in physics doing experiments and, unlike my male colleagues, when I thought I was stupid, not getting this thing written up in my offer letter, I automatically assumed that I had to swallow everything and solve my own problem instead of jumping up and down. Whereas all of my male colleagues, they always jumped up and down, demanding things, so that was why the division administrator never told me things. He thought I would go to him to jump up and down, and bang on the table or something if I didn't have anything or if I needed something. Subsequently, I found out other professors who were hired in condensed matter physics experiments after I was who would demand more than what the money was allocated for them, then, of course, there was this pot of money for me that was never used, and so it got used up by other professors. As a junior faculty, I didn't get much in the beginning, and that was tough. That was tough. But I was young. In retrospect, I don't know if I could have [laugh], well, I could have been much smarter. I would have demanded things. [laugh] But, in retrospect, I didn't know how I managed to pull through. I think I just fully concentrated on getting things done and tried not to worry about things I thought was out of my control. It was not easy.
ZIERLER: Nai-Chang, despite these difficulties, did you take on graduate students right away?
YEH: Yeah, because condensed matter physics was a hot field already. That was another reason I think Caltech was anxious to hire faculty members into condensed matter physics because students wanted to do condensed matter physics. I actually had far more students who wanted to work for me than I could support at the time. It was funny though because I was in my 20s, and so I was not much older than the students. Then, also, actually later on, I had students who were older than I [laugh] and wanted to join my group. At the time, when I first started, I was thinking, "Oh, my god, am I ever going to be able to attract students to my group?" [laugh] But it turned out not to be a problem, actually. I immediately took on students.
ZIERLER: Tell me about the major research questions that were propelling your lab. Once it got up and running after the difficulties, what did you want to focus on? What were those questions?
YEH: Those initial questions were related to what I told you earlier. Again, I was continuing the vortex physics research. In earlier days, I realized my original experimental signatures could not really extrapolate the truly universal properties of the vortex system. Therefore, I developed completely new experiments to rigorously investigate the vortex dynamics and phase transitions in high-temperature superconductors. That was my initial focus of research. That was the beginning part, and I did a lot of that as an assistant professor. But, at the time, there were also other interesting materials that popped up on the horizon. Those were very interesting magnetic materials, so I also started working on those exotic magnetic materials. They exhibited very interesting physical properties, and they were similar to high-temperature superconductors in one sense. They all belong to the class of materials that are called strongly correlated electronic systems. I was also very intrigued by those things.
Generally, whatever interesting popped up, and if I felt that I could do something different, I would not hesitate to jump in. Basically, I had two major themes that became key two pillars of my research when I won my tenure. I worked on high-temperature superconductivity mostly on the vortex physics. Then on these novel magnetic materials, I demonstrated interesting, brand new physical properties. I discovered some important phenomena, and then developed the understanding for the underlying physics that led to those interesting properties. Those were the two major things that I did. But then, of course, after I got my tenure, my research topics went completely loose because I felt that I had no constraints anymore, and then I could explore something much more risky. That was when I started doing microwave experiments for precision measurements, and started doing scanning tunneling spectroscopy down to atomic scale. I started launching brand new experiments that I had never even touched ever when I was a graduate student or a postdoc.
Vortex Dynamics and Novel Materials
ZIERLER: Nai-Chang, those early experiments pre-tenure, if you could help me visualize, what did it look like? What does a vortex dynamics experiment look like?
YEH: What do they look like? [laugh] We did different types of measurements. At the time, I developed several important experimental signatures. I basically said that if there's a universal type of vortex phase transition, then near the phase transition temperature as a function of temperature in magnetic field, for a given transition temperature near the transition, there will be some universal behavior. It doesn't matter what kind of experiments you do, there will be some universal phenomena that can be manifested. To manifest that, you could either say you could do current voltage characteristics, and do these measurements, measure at different constant temperatures, constant magnetic field. Then you can do some scaling manipulation. Then each curve at a different temperature and different field, of course, will be different. But when you do proper scaling, then it turns out that these different curves, these different isotherms of current versus voltage characteristics would collapse onto a universal curve. That collapsing process gives you some universal exponents, and the shape of the collapsed curve also tells you important things about the vortex state.
That was one way you do current voltage characteristics in the high field, low temperature, and different high field, different temperatures, or you can do AC conductivity as a function of frequency. You can fix the current that you apply through the material, but then you can start ramping the frequency of the current that you apply. Then over a wide range of frequencies, then you look at the resistance versus frequency, and then you can, again, do the scaling operation, and then they will collapse into a universal curve for all these different temperatures and magnetic field curves. Then you can also do microwaving impedance measurements, and then you have these microwave properties as a function of the microwave frequency. Then you can also collapse the frequency dependent microwave impedance data into universal curves. Then it doesn't matter which experiments you choose, in the end, some universal exponents you obtained for all these different experiments ought to be the same for the same type of phase transition. That was how I was able to prove by doing these different experiments. Then I could demonstrate different universality classes of materials. Also, it depends on the kind of defects (such as point defects or columnar defects) you introduce to your superconductor; the universality of the vortex phase transition will change fundamentally.
For instance, in a superconductor, you can imagine you put in point defects, and then the vortices will interact with them and get trapped at low temperatures. You can also put in columns of defects -- We used heavy ion irradiation to irradiate columns of tiny defects into the superconductor. Then if you apply a magnetic field parallel to the columns, they can pin the vortices very, very efficiently. If the magnetic field tilts away from the columns at a fine angle, a small angle, the behavior will still be just like when you have the field parallel to the columns. But then this phase transition will be fundamentally different from the phase transitions associated with point defects. Those were the things I demonstrated, from zero-dimensional defects to one-dimensional, and even to canted columns, I can create all these different university classes of vortex phase transitions. That was a major thing I did at the time.
ZIERLER: Now, the materials, the novel materials, the properties that you had discovered, what were those properties, and how did you discover them?
YEH: The properties? They were the vortex state properties. That's what I was talking about. You have different ways of measuring them. You measure the dissipation or measure the resistance because superconductors are supposed to be not resistive if you're in the zero frequency, zero current limit, or even for a small current. But then we would drive the superconductor near a phase transition, so we had a very, very low level of dissipation. But then they showed very interesting dependences on different parameters that we controlled. Those were basically resistive measurements, whether in zero frequency or finite frequencies. For finite frequencies, then the measured quantities are called impedances. Those were the properties that we were exploring as a function of current or frequency and magnetic field and temperature. Those were the properties we were exploring. I'm talking about the research before my tenure.
ZIERLER: Nai-Chang, some of the excitement in superconductivity from Woodstock, BCS theory, some of the major goals remain outstanding today, of course. What do you feel in your early years were some of your key contributions in ultimately getting us to some of the promises of superconductivity?
YEH: I did two important things beyond the vortex physics. After I got my tenure, IBM, at the time, they had a very old homemade scanning tunneling microscope, and my previous managers just gave it to me. It wasn't easy to use. It was just a very old system. But I set it up so we were able to start doing atomic scale tunneling into these materials. At the time, we were the first to address a very important issue. High-temperature superconductors are fundamentally different from conventional superconductors due to two major things. One is that the pairing symmetry is not s-wave. Rather, they're d-wave. But d-wave was extremely controversial. People were proposing all kinds of different pairing symmetries at the time. We were the first—"we" meaning me and a postdoc in my group at the time—we were the first to use scanning tunneling spectroscopy to unambiguously demonstrate that we have d-wave superconductivity. There were other experiments to demonstrate d-wave superconductivity but we were the first using scanning tunneling spectroscopy to unambiguously demonstrate that these high-temperature superconductors are d-wave superconductors. Subsequently, I also demonstrated—that was after my postdoc left, and I did it with my graduate students—that when you dope the high-temperature superconductors, whether they're underdoped or overdoped, the pairing symmetry would change. The pairing symmetry in the underdoped regime will be still d-wave, and the optimal doping is d-wave. But when you over-dope the material, then it becomes d+s. S-wave started setting in, and we were also the first to unambiguously demonstrate it from scanning tunneling spectroscopy. Pairing symmetry is one thing that I made major contributions to. The other thing I made major contributions to was to demonstrate that high-temperature superconductors in their ground state are not only having superconducting components. There are competing orders, meaning that there are other phases that coexist with superconductivity.
That was actually a key reason why people were very confused for almost two decades about the phenomena of high-temperature superconductors because they were seeing things that seemed to indicate superconductivity, but then there were all these other features that seemed to indicate other phases. But then when you change from materials to materials, then these other features would look different. People didn't understand that the way to look at these simply puzzling phenomena was to separate things out, considering that there was not only a superconducting phase but also a competing phase. This other phase may or may not be there, depending on the crystalline structures associated with different families of high-temperature superconductors.
[unrelated conversation]
ZIERLER: Nai-Chang, I asked at IBM, with the advances, if there was any potential for applications. By the time you had arrived at Caltech, by the time you were making these contributions, were those possibilities more obvious that there were potential applications for superconductivity research?
YEH: Actually, at the time, it would appear that a lot of applications would be difficult, would require a heroic effort in dealing with the materials because these are ceramic materials. They're not like metals, easily turning to wire so that you can make long, long wires and turn them into magnets or low dissipation transmission lines. Anyway, what I was trying to say was that when you move from one class of materials to another class of high-temperature superconductors, and there are these different competing orders that are causing trouble because they're competing with superconductivity, and you want superconductivity, but these other phases are there. They don't go away. They're complicating things. All of these issues demonstrate that these materials are hard to deal with, even though the superconducting transition temperature is very high.
Can you surmount the difficulties? Yes, in principle, but it's very hard. It took an even longer time for people to realize how to handle these materials. Originally, people were naïve, thinking that you have this beautiful high-temperature superconductivity that should have many applications. But it turns out that there are many other complications associated with these features. You have to do a lot more elaborate work to suppress what you don't want, and to elevate what you want. [laugh] It's not easy. Also, I was going to say, d-wave superconductivity is beautiful by itself but it's bad news if you want low noise systems for applications because d-wave means that in the momentum space—one nice thing about superconductors is that you have an energy gap. In an s-wave superconductor, the energy gap is isotropic, regardless of the direction. But in a d-wave superconductor, along certain directions, the energy gap is zero. Then in some other directions, the energy gap is very large. When you consider these d-wave superconductors in momentum space, you have some zero energy gap region, that's bad news because it means any excitations can cause noises. It's only when you have fully gapped superconductivity, if the excitation in energy is smaller than the superconducting energy gap, it would be suppressed so that you get a clean superconducting system with low noises. But now [laugh] you have generically zero gap behavior in the superconductor, so any excitations, thermal, radio frequency, whatever [laugh], will cause noises. That's one reason why high Tc materials are not good for making qubits.
ZIERLER: Nai-Chang, I asked about potential collaborations within physics at Caltech. What about in electrical engineering? Were there any opportunities to work with your colleagues in EE?
YEH: When I was an assistant professor, I collaborated with one of the professors, Tom Tombrello, because he was the person who knew how to do irradiations on samples. We actually used accelerators to irradiate the samples to create controlled defects, and then to look at vortex physics. Subsequently, I've had collaborations. I also had collaborations with JPL people, JPL scientists for some time, to work on microgravity-related projects, precision measurements. Then much later, I do have collaborations with people in applied physics—not EE—applied physics. Harry Atwater, I actually collaborated with him. We had a few papers together, but that was much more recent. We are studying two-dimensional van der Waals materials, and they have interesting optical properties, optical and plasmonic properties, and so we collaborated on those. But the earlier days, actually, I did most things on my own or collaborating with JPL people, and then occasionally with Professor Tom Tombrello.
ZIERLER: Nai-Chang, from those early difficulties in getting your lab up and running, did you have a specific memory of when things really started to click; when, despite those difficulties, it was all working really well for you?
YEH: Actually, with experimental physics, experimental physics research, there are always challenges. You try to solve one challenge [laugh] at a time but, one after another, things will keep coming up. If you're trying to do new experiments, then there are always new challenges. Initially, nothing was going on so I was doing a little bit of theory, and I published a couple of theoretical papers myself [laugh] before my lab was ready. After that, I started running experiments, so then things were going but I don't know what would be like "going well". It's never good enough. [laugh] There are always challenges, and you always want things done yesterday. I don't know. I had steady publications after a while. But, to me, I was always more anxious to get more things done, and I'm never complacent about anything. [laugh] It was like I solve one problem, then that's in the past, I don't look back, and then next. [laugh] I don't know. I'm always dealing with new things and challenges.
ZIERLER: Nai-Chang, when it was time for you to come up for tenure, were you concerned at all? Was it obvious that this was going to happen for you? What was that like?
YEH: I was not concerned at all. [laugh] I was not concerned at all. I thought I already did a number of original things in a fierce competition environment for high Tc around the world. I did some things that came out of my head. [laugh] They came out of my brain. I did truly original things, so I thought I had a good case, and if they would not want to tenure me, I would have other job offers. [laugh] I was not even concerned at all, actually.
Demonstrating Universality
ZIERLER: Was there a tenure talk or was there an opportunity to give a summation of what you had done up until that point?
YEH: In physics, we always have this practice. If an assistant professor is up for tenure, then that assistant professor will have to give a physics colloquium. I remember I gave the colloquium, and I was very at ease because I got so much to show, and so I was not even worried.
ZIERLER: What did you talk about? What were some of the themes you emphasized in your discoveries?
YEH: At the time, I emphasized the way or how I demonstrated different types of universality classes of vortex phase transitions; how I designed these experiments; how I actually rigorously proved that it did matter what samples I looked at, it did matter what experiments I did, it didn't matter who did the experiment, I always got this universal behavior coming out for specific classes of materials. I remembered my talk. I was demonstrating how all these different things led to true universality behavior.
ZIERLER: Which tells you what, Nai-Chang? What's the takeaway there when you see this true universality?
YEH: It tells you different classes of phase transitions that to a physicist to prove a specific class of phase transition is something like one of the holy grails that you want to do. Then they would tell you the type of interactions that you have. When you find different universality classes, then it basically is telling you there are different types of interactions in your system with certain types of defects. That was what I was trying to demonstrate. It was one of the things that when you try to think about phase transitions, it's something that people care a lot about. Are they useful? They're useful in the sense that then you know what kind of systems will give you better behavior for superconductivity, what kind of interactions, so from that perspective, yes. It was a nice demonstration. Physicists were always in pursuit of universal rules, and so that's why it's something that, to us, it's something that we really love. If we can demonstrate that, regardless of what you do, you demonstrate this universality, and then you understand the fundamentals of the interaction, then that's great. That was great, but I would say my subsequent work would be much more important than this.
ZIERLER: Nai-Chang, last question for today. You've said to me steadily over the course of our conversations, you didn't give much thought to being the first in this or that. When you did achieve tenure as the first woman to achieve tenure in physics at Caltech, were you even aware of this? Did somebody have to tell you? What was your response once you realized it?
YEH: Actually, I already knew. I remember my first faculty meeting after I got my tenure. I walked in, and my colleague Tom Tombrello shouted, "Nai-Chang, welcome to the old boys' club." [laugh]
ZIERLER: [laugh]
YEH: As I look around, yes, they were all old boys. [laugh]
ZIERLER: [laugh]
YEH: But when I realized it, actually, I felt it was important for me to be supportive of people, of young people who come after me. Also, I know that I became like a role model in many ways, and so, actually, I realized I could give students, regardless of their background, confidence in pursuing what they love, regardless of their background. Even for white male students, my presence actually made them feel that it's absolutely normal to interact with a scientist that may look totally different or may have a totally different background. As long as this person is a good scholar, a good scientist, then other things are irrelevant. I realized that I do have a positive impact from that perspective, and I try my best to interact with students positively. Actually, for myself, when I look at students, and I don't even care what gender, cultural background [laugh], whatever, absolutely. Those things are completely irrelevant to me. I only think of them as very bright students, super bright students [laugh], extremely hard-working students or whatever. [laugh] People can get along much better that way. People respect each other for who they are or what they do; not for how different they are. [laugh]
ZIERLER: Nai-Chang, on that note, for our next talk, we'll pick up on the science that you pursued, that you were able to pursue after you achieved tenure. We'll take the story from there.
[End of Recording]
ZIERLER: This is David Zierler, Director of the Caltech Heritage Project. It's Thursday, September 15th, 2022. I am delighted to be back once again with Professor Nai-Chang Yeh. Nai-Chang, wonderful to see you again. Thanks so much for joining me.
YEH: It's my pleasure.
ZIERLER: Nai-Chang, we're going to pick up in the mid-1990s. We're going to focus on the research. But I did want to ask another question about the significance of you achieving tenure at Caltech at this point. Did you see that as an opportunity for more women at Caltech—physics or across the institute—to see this as a possibility? Did you talk to people about these kinds of things?
YEH: Not really. [laugh] Not really. But I just thought that it came with a—it's obvious, without saying it. Having a woman tenured from junior faculty, not inviting some established people from outside, I think it does send a very important message, I think.
ZIERLER: Do you have a sense of—I mean, at this point, obviously, you do—but the tenure process, who makes those decisions, the committees and the individuals?
YEH: The tenure decision, actually, in a place like Caltech, it's actually quite rigorous and complicated. What typically happens is that the division chair would appoint a tenure committee, and that constitutes a bunch of tenured professors. There will have some experts in the area, and also people not so much experts in the candidate's area, because it's important to make sure that a professor who is being tenured at Caltech has an international reputation with accomplishments that well-qualified people in physics would appreciate. It doesn't have to be in a very narrow area. People have to appreciate the general impact of the research, and so you need to have a committee that consists of a range of different subfields of physics to be on the committee. Then, there will be a committee chair, and then these professors would come up with a list of external reviewers whom they would like to seek advice from. It's extremely important to have a rigorous list of international advisors, international recommendation letters or any reference letters, whether they're recommendations or not [laugh], because you want to make sure that the person has established international reputation. That was a critical element that letters would be sent out to leaders in the field of the candidate, and then to get their evaluations. Of course, Caltech professors themselves can come up with their own evaluations or so. But, overall, then it's a collective evaluation of what this person has achieved in the research field as well as in teaching and also in doing service. But teaching and service are important but not so important. [laugh]
ZIERLER: [laugh]
YEH: The most important part is really the accomplishment of research.
ZIERLER: Of course.
YEH: But you also want a person that's also reasonably good as a colleague, as a mentor to students, so those still also considered. But if those two aspects are excellent but the research is not, then the person still would not get tenure.
ZIERLER: Of course. Nai-Chang, we'll talk about the specifics of your research. But at a general level, did achieving tenure free up the kinds of things that you could do. In other words, could you be more adventurous in your research—
YEH: Yes.
ZIERLER: —as a result of getting tenure?
YEH: Precisely. When I was trying to get tenure, I tried to be a little bit more focused, although my personality is such that I like all kinds of different things. I just love to explore. But I knew that I had to be a little bit more focused so that I wasn't all over the map. [laugh] But then, after I got tenure, I was more daring in venturing out towards riskier projects. I typically would arrange my research in a way that I could explore difficult things. For some difficult things I felt that I could still be very productive. But some may take some time to become productive or, sometimes, it might run at the end. [laugh] You never know if you're doing something nobody else has done before. I had to do a little bit of a risk assessment, and then plan things accordingly, because there are an infinite number of fun things out there—at least to me. I have to be selective about what I want to do that would produce good results, good for training students, so that I can also secure funding. But, at the same time, I also have this rebellious aspect of my personality that if something is darn hard, I want to try. But I cannot only do that. If I only do that, I could get myself and my students into trouble.
ZIERLER: [laugh]
YEH: [laugh] You want to make sure your students also graduate within a reasonable amount of time and get good jobs. I had to factor those things into consideration. But, indeed, receiving tenure did give me more freedom to explore some risky things.
ZIERLER: Let's focus perhaps first on superconductivity. What was the outer edge, some of the riskier areas in superconductors that you might have been able to pursue after achieving tenure.
YEH: For instance, a very important aspect is on why high-temperature superconductors superconduct. What are the differences between high-temperature superconductors and conventional superconductors? That's the holy grail of superconductivity. If we understand how to make materials superconducting at much higher temperatures, and hopefully with better properties, then you can talk about a lot of wonderful applications, and then that can have a big impact on society. But then, of course, to purists, we also want to know why, because that's what's driving all the physicists. We are so curious, we want to understand the underlying mechanism. But that area of research was very cutthroat and very nasty, to say why these high-temperature superconductors superconduct, because the materials are so complicated, and there are so many different camps of different thought, and people sometimes were at each other's throats. [laugh] Everybody felt that whoever could solve the problem would get the next Nobel Prize in superconductivity. That was one thing. But the other thing was the materials, the systems were so complicated, so if you're not doing things carefully enough, you could fall into traps that you may say, "This is my scenario of explaining why these materials are superconducting at higher temperatures." You may be able to explain yourself around certain systems, certain types of experiments, but then fail in other systems and other experiments related to similar materials. To me then, if it's not universally applicable to be correct, then that theory, that scenario cannot possibly be correct, or at least will be missing critical components. It was so nasty because then people, when their scenario couldn't explain everything, they would accuse others of—must have done wrong experiments or whatever, instead of saying that "Maybe my scenario [laugh] is not quite accurate." It was very nasty. I tried to not immediately step into it before I got my tenure. Then, after I got my tenure, I jumped into it. Of course, it was very nasty. I had to fight. [laugh]
ZIERLER: Now, the various camps that you refer to, what camp did you represent? Where did you slot into these debates?
YEH: I didn't try to align myself with any camps. I was just going after the facts, looking at all published results, designing experiments objectively, and then finding out what the data would tell me. I think now my view has become a consensus but, at the time, when I was talking about it, some people really were trying to beat me down, etc. But I felt that my data would live, would survive with time, and now the view that my data supported has becomes a consensus in the field. The "camp" that I referred to is that these high-temperature superconductors, their ground state is not superconductivity only. The ground state can consist of other phases that can coexist with superconductivity.
ZIERLER: Nai-Chang, if you can explain, what does "ground state" mean in this context?
YEH: "Ground state" is a very common word for physicists. "Ground state" means the lowest energy state, the lowest energy possible states for a physical system at low temperatures. In conventional superconductors, in general, if you go to the lowest energy state, superconductivity wins. If you raise your temperature above the superconducting transition, then that state will prefer to be, say, a metallic state, or some other "normal state". But superconductivity cannot survive above certain temperatures. In the case of high-temperature superconductors, it turns out, if you cool down below the superconducting transition temperature, superconductivity is not the only winner. It's like you have a family of siblings. They fight. [laugh] They're competing. They compete for the electrons that are present there, so you could have superconductivity when superconductivity forms. Then, let's say, if it's an electron-type superconductor, electrons and electrons like to pair up to form bosons, and go to the lowest energy state. But in high-temperature superconductors, that's one phase that can exist. But there will be other phases that can also compete for electrons, and they turn themselves into other phases, for instance, charge density waves.
I don't want to get into very, very detailed [laugh] technical terms, say, charge density waves, or, pair density waves. There will be some density waves that are not superconducting, but they can coexist with superconductivity. What's causing all the confusion in the high-temperature superconductivity field in the beginning was that when you have all these different families of high-temperature superconductors—they consist of different elements, etc.—the only common thing they have is copper and oxygen. There are many, many different elements you could put in to the superconductors, and you can have somewhat variations of structures also. What's confusing people was that when you cool down to low temperatures, if your ground state is not only superconductivity, you can have other phases coexisting. Then, the experimental data you're going to see are going to vary from different families of materials to different families of materials, because you will be seeing things that are signatures of superconductivity, but you will be also seeing signatures of other phases coexisting. Then, people were just not thinking the right way. I wasn't the only person talking about competing orders in the ground state of high-temperature superconductivity. But, in the beginning, we were the minority. But I felt that all the data I trusted from other research groups and all of my own data were pointing towards the fact that it was absolutely clear that there were competing phases; that superconductivity wasn't the only child of that ground state. [laugh] In that family, there are siblings competing.
For materials, if you had different competing orders, different competing phases, and then they would give rise to phenomena that would be combining with superconductivity, and causing people a lot of confusion because when you looked at one system, you would see certain phenomena; and then you looked at other systems, you saw other phenomena. But then I designed experiments to sort things out. Basically, I was finding that I always would see signatures of superconductivity in the ground state for different materials, but then I would also see different competing phases. It depended on how I modified the superconducting material. That was one thing. I then tried to advocate that the presence of competing orders in the ground state of high-temperature superconductivity was an important fact. But I have solved the question regarding why these superconductors superconduct at such high temperatures, although I do have some ideas based on this observation. But the research field was pretty vicious for a while, because people didn't want to concede that they may be wrong. [laugh] The field was very vicious, and so I'm glad that I only jumped into it only after I got my tenure. [laugh]
ZIERLER: Why this field? There are arguments in science all the time. What is it about high Tc that made this so personal for people where they acted in this way?
YEH: I do not know, actually. Maybe it's just the sheer number of people who jumped into it for some time. Now, it's not like that anymore because after high Tc didn't show lots of immediate applications for some time, people spent money on it, and then people—"people" meaning funding agencies—started backing away from funding it, and other fashionable things showed up, and then people started going towards other fashionable things. Within the high Tc community, it's no longer like that. But it took decades for people to start working on much more careful experiments, and becoming much more rigorous. The same is true for theory, and people would think much deeper on all the complicated issues. Now, it's much better and it's back to a scholarly environment, but the number of people is also much smaller, I think. [laugh] I think it's the sheer number of people but, also, the topic attracted a lot of big personalities into the field, and so people started taking it so personally. It was an interesting thing. Maybe in the future, if I write [laugh] some memoir of things—I won't name the names if people are still around [laugh]—but I can talk about some very interesting personalities. [laugh]
The Impact of Tunneling Spectroscopy
ZIERLER: Oh, my goodness. Nai-Chang, to go back to the data, what the data was telling you, simply taking an objective approach, you mentioned that now there's the consensus about your analysis. That really begs the question, what was the data telling you, and what were you conveying to the community, whether or not they wanted to hear it?
YEH: My view is becoming a consensus, not only because my data supported that view; there is much more new data supporting that kind of view, although they're from different experiments. I never believe in using one experiment to prove everything. You need to have all kinds of different experiments that all lead to the same scenario. When the scenario is correct, you can explain all of the experimental observation. This scenario that I have about competing orders in the ground state of high-temperature superconductivity, that scenario now can explain lots of different experiments, including my own experiments. But I first designed my own experiments to demonstrate that idea, and then there were other different types of measurements, experimental techniques that also led to this kind of direction, which is a happy situation. I have some experiments; not just one kind of experiments. But the primary experiment that I did was called "scanning tunneling spectroscopy". We have a scanning tunneling microscope (STM) that can really go down to atomic scale resolution to do imaging and spectroscopy. In high-temperature superconductors, because the materials are very complicated, it could easily have disorder and variations. It's quite important to use a highly spatially resolve probe to study the spectroscopy, and so that was what I did. I don't know how technical you want this to get into.
ZIERLER: No, please, as technical as you like. Thank you.
YEH: Really? OK.
ZIERLER: Sure.
YEH: [laugh] What happened was that then we did this tunneling spectroscopy on high-temperature superconductors, different types of high-temperature superconductors, or high-temperature superconductors with different electronic doping levels. But they're superconducting at different temperatures, so I wanted to see if I could resolve information that were related to different phases. What I have done is that I went down to low temperature, well below the superconducting transition temperature of all of these different types of high-temperature superconductors, and then I could always see when I did the tunneling experiments that not only we could demonstrate—we were one of the first research groups, actually, to use scanning tunneling microscopy to demonstrate that high-temperature superconductors are actually d-wave superconductors. We demonstrated experimental signatures of that. That was not controversial because our experimental data were just clean cut, so that was not controversial. But we were the first to use STS—that's scanning tunneling spectroscopy—to demonstrate this pairing symmetry, which is very important, pairing symmetry. Then, to demonstrate that there are competing orders, we also did a lot of detailed studies of the tunneling spectroscopy over a substantial area of each superconductor with atomic resolution. But then we measured it over a large area, so it took a long, long time to collect the data. But when we studied these things over a large area, then we could analyze the tunneling spectrum as a function of energy, and then we found that there are these—it gets more and more technical.
ZIERLER: Please.
YEH: When you do these spectroscopy measurements, for every pixel at every spatial position, you can do measurements of the tunneling conductance as a function of energy. You have a range of energy, which is the electron tunneling energy between the STM tip and the sample, and, for the corresponding conductance, of course, you have a range of tunneling conductance. Then, you go from spot to spot to collect the atomically resolved spectroscopic data. You go over a sufficiently large area, and then you can do Fourier transformation of millions of tunneling conductance versus energy spectra taken in the spatial space into the momentum space. Then you would end up getting very useful spectroscopic information that's a function of energy and momentum, and so, from that, the electronic energy and momentum relation of the superconductor at low temperatures. From that we can get something very important called the dispersion relation of the electronic states. The dispersion relation is the energy versus momentum relationship. If you're in a truly superconducting state, then the energy versus momentum—OK. If you're in a superconducting state—let me back up a little bit—then the tunneling conductance should be zero because that's a gapped state. You have no tunneling conductance and, over the range of superconducting gap, you have pretty much zero conductance. But then when you move away from the zero conductance, or go away from the gap, then you will get some tunneling conductance. There are special signatures for the tunneling conductance versus energy behavior if you have pure superconductivity. That tunneling conductance as a function of energy has some special signatures, and it's very distinct if you have d-wave superconductivity. But if you have competing orders then, often, those energy states, the dispersion relation, would be completely different. What we have found was that we could always analyze each tunneling spectrum in terms of two different components. One showed the expected behavior of superconductivity, d-wave superconductivity. The other showed something else totally different from what superconductivity should be, and were very much consistent with signatures of density waves that were later on confirmed by people using other experimental techniques, and we were already finding those types of dispersion relations for density waves from our STS studies long before others.
At the time, I always observed two contributions everywhere I did my measurements. It didn't matter what samples I was looking at, different families of high-temperature superconductors with different superconducting transition temperatures, there was always this d-wave component that showed superconductivity. But there was this other component that changed from samples to samples if you studied a different type of superconductor. That was how I found out. Then, we had other confirmations, say, from optical experiments and then, later on, people who would do x-ray measurements using synchrotron radiation, and then they were also seeing these types of things. Now, I think nobody would disagree with the presence of competing orders, at least among the mainstream; most people will agree that these superconducting ground states are very complex. There's not just one phase. But I should be a little bit more rigorous, actually. It's not only one phase for most of the different types of superconductors and doping levels. But sometimes if you so-call overdope your material with carriers, then it eventually becomes more like a conventional superconductor. It becomes more and more like a conventional superconductor with excess doping of carriers. In this case, the superconductor would only have one ground state in the over-doped limit. But when you have the highest superconducting transition temperature for a given type of superconductor family, which is called the optimally doped regime, and also the underdoped regime of a superconducting family, the competing order is definitely there. It's always there. I think that are already overwhelming experimental evidences now for the presence of competing orders in the ground state of high-temperature superconductors, at least the experiments that we can trust are all pointing to this picture.
ZIERLER: Nai-Chang, what circa the mid-1990s was considered the holy grail of superconductivity research? What was all of this working towards?
YEH: People were hoping if they understand how to achieve high Tc, and then maybe they could synthesize even better materials, and then they were hoping for a lot of applications. It was really for application purposes that funding agencies invested very much into high-temperature superconductivity —well, OK, the physics was very exciting too. The physics was completely unconventional. We had to learn and figure out new physics to understand the system. But people were hoping that if we could achieve a superconducting state at relatively higher temperatures to reduce the cost for cooling, then that would be no energy dissipation in the superconductor, and so we would be able to make all kinds of devices, or we could have energy transmission without power dissipation. In other words, we could have electricity transmitted without losing any energy, and that would be wonderful for a lot of applications.
But there are also many important devices that could be made from superconductors. For instance, some of the best metrology and precision measurements are done by conventional superconductors because of the unique properties of superconductivity. People were hoping that high-temperature superconductivity can do the same thing for all these wonderful precision or wonderful metrology measurements or technology. Then, if that's the case, and you don't have to cool the devices down to such low temperatures, then it will be ideal. But the problem turns out that high-temperature superconductivity doesn't fulfil this expectation for the fundamental fact that they are d-wave superconductors. They're not s-wave superconductors. D-wave superconductors mean that in momentum space, you always have certain momentum direction that the energy gap is zero. When the energy gap is zero, that means you can have arbitrarily low-energy excitations. You cannot gap excitations out. When you have low-energy excitations, you start creating losses and noises. [laugh] In conventional superconductors where they are s-wave superconductors, that means you have a very robust energy gap over a certain temperature range. When you limit your excitations, say, temperature or frequency or energy, below a certain threshold, you can have true zero dissipation and also filter out noises below the threshold, and that's critically important for a lot of applications.
But in high-temperature superconductors, there's always certain momentum direction that the energy gap is zero. [laugh] You always have low-energy excitations. It's a matter of how much you can reduce that. If you still have to go to a very low temperature to reduce the low-energy excitations, then it's not helping the situation, even though the average energy gap is much bigger and the superconducting transition temperature is much higher in these d-wave superconductors. It's for true physics reasons that the applications are somewhat limited if they depend on the energy gap of the superconductor. But if you just want to use the conductivity part, then it's now being used for zero dissipation wires. You can turn zero dissipation wires into superconducting magnets by winding them into solenoids, and then you can create extremely large magnetic fields without losing superconductivity, without causing dissipation, because the superconducting transition temperature is indeed very high, and so you can still run some large currents, and create large magnetic fields without losing superconductivity, without causing dissipation. Let me put it that way. It depends on the application. Now, there are some applications that are achieved now. But, let's say, it would be hard to use high-temperature superconductors for qubits. Qubits really have to go to low-temperature superconductors because you really, really, really want to get rid of the low-energy excitations. It depends on the applications. The physics, the interesting physics properties are actually causing trouble for applications. [laugh]
ZIERLER: Nai-Chang, I wonder, as you mention, funding agencies were increasingly excited about potential applications, did that in any way make your research more applied than it otherwise might have been?
YEH: I always choose my research topics based on if it's fun to me. If it's no fun to me, I won't do it. I just simply will not do it, because what's the point of doing it out of all these research topics? I will do things that I consider as very interesting; very, very rich in the physics. But, these days, there are so many interesting topics that can be both of fundamental importance and also with potential for applications, and so then you can sort of design things that will be good for both worlds. Right now, I'm doing a lot of two-dimensional materials, van der Waals materials, like graphene, transition-metal dichalcogenides, and all these. These are materials that are extremely interesting in their physics but also of great potential for applications, and for some of them we have already demonstrated applications. But I would not jump into anything for the applications alone. I will only do things when it's fun, and then often it's when I found out all these interesting things that I'm exploring can also have applications, then that's perfect.
Sometimes, industry came to me to ask for help and to collaborate, and that's great. But I will only collaborate if those are interesting scientific projects and at the same time, there's that technology direction. Also, I would say, these days, when things get smaller and smaller, as we're pushing towards the quantum limit, when you are making smaller and smaller devices, chips, everything goes into the quantum regime. When you go to the quantum regime, there's no boundary between frontier science and frontier technology any longer. They go hand-in-hand. You have to solve technological issues to address the scientific issues, and you have to solve the scientific problems to advance the technology. It's perfectly fine. It's just by design. You can choose topics that are both exciting for fundamental research and with potential for applications, and some of the things can be even having applications in the relatively near future.
ZIERLER: Nai-Chang, in superconductivity, where has there been value for you to interact with theorists or to pay attention to some of the theories underlying these questions?
YEH: Mostly, when I work on high-temperature superconductivity, I actually dive into educating myself very deep into quantum field theory because I found a lot of the fundamental concepts, when I wanted to describe the physics that I observed, I really needed a very, very deep theoretical background, a very strong and deep theoretical background in quantum field theory and sometimes in topological field theory. Because of high-temperature superconductivity, I actually kind of force myself to become theoretically much stronger. I sort of learn a lot of things on my own, and then I could teach these topics in advanced courses to students, and my students could do theoretical analysis without having to really ask theorists for help, because we can manage it. But then, at the same time, we could have very good conversations with theorists on all aspects of things, and we could understand state-of-the-art theory much better, so that was how I did it. Most of the theoretical analysis…actually, not most. All of the theoretical analysis I did for my experiments on high-temperature superconductivity were all done by me and my students.
ZIERLER: In some ways, you've served as your own theorist?
YEH: Yeah, for some of these things. But I was driven to go deeper in theory because the physics is so rich and interesting.
ZIERLER: Of course, this is very unique in the modern age. There is such a strong bifurcation in physics between experimentation and theory. What was it for you that you wanted to cross these lines the way that Fermi would have, perhaps?
YEH: To me, it's like I love to design the experiments to verify my own ideas. But then when I come up with very interesting data, I want to be the first person to, hopefully, figure things out without having to go to other people, because I work so hard for the experiments, and I want to have the joy of figuring things out, have this aha moment [laugh], like the utopia moment. I want to have that. It's just me. [laugh] I'm not a very typical experimentalist. I enjoy all these things. But it's also like I'm not a very typical physicist. Actually, a lot of scientists, a lot of physicists are also very versed in other things. I love literature. I love music. I love fine arts. I love history, philosophy, and all these things. But life is too short. I can only pick one profession. [laugh] Then, within this profession, theory is so exciting. Experiments are so wonderful. I want to dab into both if I could, just for whatever energy and time and money I have. [laugh]
ZIERLER: Nai-Chang, looking back 20–25 years, what was your sense of timescale in terms of getting to these breakthrough discoveries that everyone was so excited about? Did it seem around the corner? What's been achieved since?
YEH: You mean in the field or me?
Physics in Pursuit of Truth and Beauty
ZIERLER: Thinking about the field of high Tc, and the breakthroughs that you were working toward, did you expect these things to be happening in the year timescale, five years, a decade? Could this consume the rest of your career and perhaps even beyond? What were you thinking circa the 1990s?
YEH: At the time, I was not really worrying about the number of years; just however it takes to understand something. To me, I never really said, "I must know this within this year or next year or something." It's just that I try my best to look at the issues, and design the experiments. Then, once I had the experimental data, sometimes I didn't understand them, then I learned new physics from the data. Then I had to design new experiments to do additional investigations to reach a better understanding—so, whatever it takes.
My attitude is that physics is to pursue the beauty and truth of the universe, and so whatever it takes, and that's the goal, and just we do our best to explore. I never really worry about or thinking about the timescale, because I also know research is not predictable. Actually, if you're a good researcher, then whatever new things you do, your original plan often has to be modified because if it's exactly as you planned, you must not have done anything interesting. Then it's all predictable. If it's all predictable, what's exciting about it? I already learn when I do research. Actually, when I was doing earlier-stage research, things were indeed much more predictable. [laugh] Then, as I started doing more and more exciting things, things were getting less and less predictable. But that's exciting because, then, I consider topics that I want to explore, and design something that guided me along an interesting direction. Then I get interesting results that are beyond my expectation. Then I learn something new, because if everything is within my expectation, I learn nothing.
ZIERLER: That's right.
YEH: Then it's boring. [laugh] Then I will be disappointed. I'm always excited when I learn something new from these experiments I design, at least it guided things towards the right direction on the whole, but I don't know exactly what to predict.
ZIERLER: Nai-Chang, tell me about winning the Outstanding Young Research Award from the International Organization of Chinese Physicists and Astronomers.
YEH: Actually, I was surprised I won that [laugh] because I thought, at the time, the review committees consisted of multiple Nobel Laureates and the gurus in the field I really, really respect. I was like, "Wow [laugh], they actually gave the prize to me." [laugh] Also, I was the only person getting the prize without sharing the prize in the year. Later on, I became the President of that organization, and that prize often was split among two or three people because it's always very competitive. I was pleasantly surprised when I won that award. It was nice. It was a nice recognition of my work.
ZIERLER: Does the organization draw a distinction between Mainland China and Taiwan?
YEH: No, it was for physicists all over the world—for Chinese-heritage physicists all over the world.
ZIERLER: Where is it located, the organization?
YEH: The organization is officially established in the US, but the members consist of people in the US, Mainland China, Taiwan, Singapore, Hong Kong, Europe—anywhere. You can be anywhere, as long as you have Chinese heritage.
ZIERLER: Nai-Chang, let's move now to magnetic materials, and the way that you pursued these materials in adventurous ways, perhaps as a result of getting tenure.
YEH: Magnetic materials, that's also very interesting, so I was always interested in systems that exhibit these very novel phase transitions as a function of temperature or other variables. In these systems, we can go from one phase to the other by tuning some parameters, such as the temperature, magnetic field, or doping level. Often, when you go from one phase to the other, you're changing the symmetry of the system, and so there's symmetry breaking involved. These days, it doesn't have to be symmetry. You can change the topology and achieve a new phase also. Generally, I enjoy looking at physical systems with phase transitions and trying to understand what drives the phase transition, and then study the properties of the physical system near the phase transition, and also well below and well above. I generally find that quite interesting. I got interested in some magnetic materials with structures similar to those of high-temperature superconductors, known as the perovskite structures. This class of magnetic materials that I started exploring were also perovskite magnetic materials. They just have different elements from those in high-temperature superconductors, like you replace copper by other elements such as manganese, cobalt, or nickel, and then the properties change dramatically, even though you might have similar structures.
In part, at the time, people also studied these materials because they were also hoping some of these materials could become superconductors—but they didn't. But then they also showed very interesting magnetic properties. For instance, on some materials, when you go below a certain temperature, and they become, let's say, they become ferromagnetic materials, you go from paramagnetic materials to ferromagnetic materials. Then, when you go to the ferromagnetic phase, you can have this property that's known as the anomalous Hall effect. Hall effect, any metals, if you apply a magnetic field perpendicular to the current moving direction, then you can always deflect the currents a little bit. If you apply a current along the x-direction, then you could get a voltage along the x-direction. Now if you add a magnetic field along the z-direction, you could have a voltage appearing along the y-direction due to the deflection of moving carriers by the external magnetic field, not just a voltage across the x-direction. A magnetic field will do that. But if you have a ferromagnetic material, then you don't have to apply any magnetic field. If the sample is cooled below the ferromagnetic phase transition temperature, then you observe this Hall effect, and it's called the anomalous Hall effect. It's just because you have intrinsic magnetism that's established inside the sample. This anomalous Hall effect can be useful for various things. We actually discovered a class of these magnetic perovskite materials that has this incredibly, I mean, a record-high anomalous Hall effect at temperatures near the ferromagnetic phase transition temperature. To me, it was fun [laugh], and there are reasons why this anomalous Hall effect signal for that material is so strong. It's to do with a phenomenon called the Berry phase and magnetic fluctuation effects. It's the fluctuation effect of the spin degrees of freedom that gives rise to excess Berry curvatures in the system -- It gets really technical. [laugh]
ZIERLER: In what ways is the anomalous Hall effect different from the quantum Hall effect?
YEH: Quantum Hall effect and anomalous Hall effect are totally different phenomena. Anomalous Hall effect can appear when you don't apply any magnetic field. When you have ferromagnetic materials, then you can have a finite Hall voltage due to finite magnetization inside the sample. But for detecting quantum Hall effect, you will have to apply a magnetic field. You have to apply a very strong magnetic field perpendicular to the two-dimensional electron gas. Then, as you go to a very high magnetic field and very low temperatures, electrons will move in "cyclotron orbitals" so that their trajectories will form these little circles called cyclotron orbitals. So the electrons move in circles and go nowhere. They cannot move from one side of the sample to the other. The sample becomes in an insulating state, except near the edge of the sample. Near the edge of the sample, the electrons cannot form a complete orbit. They will skip the orbit, and so they will actually link up, and form a conduction channel near the edge. That's when you get quantized Hall resistance. It's a quantized resistance with just one quantum channel. That's the quantum Hall effect, or you can have different quantum Hall phenomena. You can have a different number of edge states, and these edge states are completely topological. You have to apply a very strong field, and you have to go to low temperature to see the so-called edge states that got linked together. But the bulk of the material is insulating, and you're only forming a conduction channel along the edge. The quantum Hall effect is associated with a special quantum Hall liquid that's a topological phase, and then it's mostly insulating except the edge. The anomalous Hall effect also has some topological nature in it, but it's something that you just have a finite magnetization in your sample as if you are effectively having an internal magnetic field. I'm simplifying it. [laugh] Then, you're measuring a Hall voltage, and that's not an edge effect; it's the global effect, so that's different. They're different.
ZIERLER: Nai-Chang, you wrote—
YEH: But you can also have quantum anomalous Hall effect.
ZIERLER: You wrote a very interesting article for the Chinese Journal of Physics in 1997: The Science and Technology of Condensed Matter Physics from Atomic Imaging to Space Research. I wonder if one of your motivations was to demonstrate just how rich and exciting condensed matter physics was, and how far away we were from people like Murray Gell-Mann referring to squalid state.
YEH: That was my motivation because, at the time, I was doing different topics. I realized that when I had conversations with young people, especially young students about what they would like to get into for the future, and then I would tell them, "Look closely into condensed matter physics because there are all these different examples," and so people then asked me to write an article [laugh] to give this introductory idea to students.
Lattice Distortion and Return to Graphene
ZIERLER: Tell me about lattice distortion. You were so fascinated by lattice distortion. What were you looking at?
YEH: Lattice distortion can have many consequences. For instance, if you have certain types of lattice distortion, you are changing the symmetry of your original crystalline structure. When you change the symmetry, you also change the physical properties, and so that's one consequence. You can also induce lattice distortion by engineering the strain on the sample. When you engineer the strain on a sample, you induce lattice distortion by changing the bond length, bond angle, and the symmetry. With these structural changes, the electronic bandstructures would also change. For instance, consider taking a piece of graphene that's a honeycomb flat sheet. If we create these periodic nanostructures on a substrate and then we put a graphene sheet over the nanostructures, we will obtain a graphene sample with periodic strain superlattices and completely change the electronic band-structures of graphene. The physical properties are fundamentally changed. Like, a piece of graphene, if you just measure it, a flat sheet of graphene, there are lots of interesting properties but it's just a piece of graphene with special properties.
But when we engineer strain lattices, we can create gigantic "pseudomagnetic fields". "Pseudomagnetic fields" means that the local magnetic field can be positive or negative, but the magnitude is so large that I could achieve hundreds of teslas of local magnetic fields without needing an external source to generate any external magnetic field. I can just strain a monolayer graphene, which leads to changes in the bond angle and bond length of graphene in addition to of some special original properties of graphene. These strain-induced effects result in gigantic pseudomagnetic fields that also give rise to fundamental changes in the electronic properties globally. I could do that. Lattice distortion, it can be induced by strain. More generally, lattice distortion could be due to impurities or artificially designed strain, or you can just change the temperature. Thermal effect can also cause lattice distortion, but it would be kind of a random situation. There are many ways you can introduce lattice distortion, or you can substitute certain atoms in certain crystalline structure. If you substitute certain atoms, let's say, by smaller atoms in the position where the original larger atoms are, you're immediately causing the local distortion of the structure already because you put a smaller atom in an originally supposedly to be a large atom, so the crystalline structures are modified. There are many ways you can do lattice distortion. Then, they do lead to very interesting properties if you know how to engineer things.
ZIERLER: You clearly do. What kind of interesting properties did you find?
YEH: What I just said, for instance, this is in graphene. I can create. I can take a substrate, and use nanofabrications to create lots of nanostructures, periodic nanostructures and, let's say, periodic nanocones, some sharp cones. Then, I can transfer a piece of graphene over it. Then, these sharp cones would, of course, distort graphene. Graphene is just one atomic layer, so it's like a membrane. Imagine you have some sharp features on a substrate, and you put a sheet of very thin material membrane over it, and if the membrane is very elastic, then it will conform to the distortion. But then the carbon atoms on that sheet started changing the bond angle and bond length. Then, if you distort it properly—there's a lot of physics going behind how you design it properly. But when you design it properly, then you create these gigantic local magnetic fields. The local magnetic fields would go from extremely large positive fields to extremely large negative and, in between, it would go to zero.
If you integrate over all of the magnetic fields over the area, you include both positive and negative fields, then the total magnetic flux will be zero. That's why they're called pseudomagnetic fields. But they're not zero locally. When you send electrons through the structure, electrons are extremely tortured [laugh] because they're seeing these enormous up and down, up and down, enormous, large fields. You can design it in such a way that you can create a superlattice pattern so that that it creates new band-structures and completely new physics. For example, graphene itself, flat graphene, the electronic mass near the so-called "Dirac point" of the material is zero. Electrons are effectively massless in graphene. The low-energy excitation in graphene is as if electron excitations are almost behaving like photons because electrons have no effective mass near those special Dirac points. But when I create giant pseudomagnetic fields by distorting graphene, and turn them into a very strange superlattice structure, I create "flat bands". That means the energy versus momentum is independent of momentum, and that means I have an infinite mass for the electrons. I go from zero mass for electrons to infinite mass for my electrons. That's a fundamental change of the electronic properties.
ZIERLER: Definitely. [laugh]
YEH: It's fun. It's fun that I can kind of play God [laugh]—
ZIERLER: [laugh]
YEH: —by designing things the way I wanted, and then seeing a lot of new phenomena.
Metrology and NASA
ZIERLER: You mentioned metrology. Tell me about your work thinking about superconductivity, and creating ever more precise clocks.
YEH: I was doing that when NASA was still supporting the microgravity project. We were hoping to do all these precision measurements because when you are doing really, really precise measurements, gravity could cause some issues, and so it would be good to do these experiments in the International Space Station for all these precision measurements. I was hoping to do precise measurements of the dielectric constant of helium, liquid helium, in a microgravity environment because liquid helium itself, its dielectric properties would actually be affected as a function of temperature by gravity. There would be some gravity-induced rounding effects to the dielectric responses of liquid helium as well as its superfluid phase transition temperature. NASA was funding these projects that we could first do a lot of testing, and make things as precise as possible on Earth, and then maybe some of these experiments could be selected to be conducted on the International Space Station. That was the original plan, and I was being funded for doing precision measurements related to liquid helium, and the superfluid phase transitions in liquid helium. Liquid helium will go from a normal fluid to a superfluid at sufficiently low temperatures. A normal fluid means a fluid that when it flows, it has dissipation. Liquid helium would go from normal fluid to superfluid at a temperature called the Lambda transition. When liquid helium enters the superfluid phase, it's similar to the situation when a normal metal becomes a superconductor and electron motion will not cause resistance. Similarly, in the superfluid phase, liquid helium will be flowing without causing dissipation. Superfluid, in many ways, is similar to superconductivity, although not identical. But a lot of the physics concepts are kind of similar.
I was trying to do precise measurements, dielectric constant measurements of liquid helium, and the design was to construct a superconducting microwave cavity with a very high quality-factor (Q). We would fill the high-Q superconducting microwave cavity with liquid helium, and when we changed the temperature of the system through the Lambda transition, the response of the microwave cavity, including its resonant frequency and the linewidth of the resonant peak will be changing very sensitively with the temperature near the normal fluid to superfluid phase transition. That was the idea. But if you fill the microwave cavity with liquid helium, then the density of liquid helium from the top to bottom will be different because of gravity, because it's a liquid, so the bottom will have higher density just because of a little bit of gravity. That difference is very small, but that difference could cause the phase transition temperature to be smeared a little bit. We would like to determine the superfluid phase transition temperature to very high precision, which would require the use of high-resolution thermometry and a high-quality-factor superconducting cavity. We were working on those experiments. We developed high-quality-factor superconducting microwave cavities using niobium. Niobium is a low-temperature superconductor. We treated the cavity at an extremely high temperature, well over 1,000 degrees, under an extremely high vacuum, and that wasn't easy to do. Then we were able to achieve a high-quality-factor superconducting cavity. Then we were also developing high-resolution thermometry. We were able to reach a world record temperature resolution near the Lamba transition using the high-resolution thermometry with a superconductivity-enabled detection mechanism, known as the SQUIDs (Superconducting QUantum Interference Devices). But, in the end, we developed it, but we didn't use it because NASA canceled all microgravity projects later on. We developed high-resolution thermometry with a temperature resolution of better than one trillionth of a degree (about one part in 1012) near the Lambda transition temperature. We developed it using a superconducting technology also. [laugh] That's the metrology that we were talking about. It was really pushing to the precision limit and pushing to the high-quality limit.
ZIERLER: Nai-Chang, what happened with the NASA funding? Was it applied in different ways than were originally expected?
YEH: I had fun doing some of those experiments, and I knew that we could push technology to some extreme. But then later on, no, we couldn't quite use what we developed because those were very specific for a microgravity environment. Supposedly, we could use some of the instruments but, no, we were not able to in the end, because it was too expensive to run those setups anyway. Then I jumped onto other things. [laugh] When the money was used up, OK, too bad. [laugh]
ZIERLER: Time to move on?
YEH: I got a PhD thesis student out of that. I was having fun doing precision measurements, but it was a pity that we couldn't—we were going to use that also for clocks. You were asking me about clocks. They could be used for clocks, but there was another reason at the time. Using superconducting cavities, you will have to develop clocks that will be operating at microwave frequencies. But, later on, optical techniques that operate measurements at optical, visible light frequencies can achieve much better quality-factor than systems operating at microwave frequencies using superconducting cavities. That was another reason why we could not use high-Q superconducting microwave cavities for state-of-the-art precision clocks. Originally, we were trying to measure high-precision measurements of the Lambda transition, and we were also going to use that for precision clocks. But another technology becomes more advantageous and easier to operate, and that's the optical techniques with optical resonators. The superconducting resonators are no longer the prime choices for precision clocks, so people then just went on with optical clocks.
ZIERLER: I wonder if you can explain the connection between tunneling spectroscopy and the study of quasiparticles. In other words, are there other methods that you can use to understand quasiparticles, or is it really only tunneling spectroscopy?
YEH: There are other things you can understand quasiparticles because quasiparticles then, generally, you will already have some dissipation associated with quasiparticles, although they kind of still "remember" superconductivity and, therefore, the losses won't be that high. There are other experiments that you can look at quasiparticles, like angle-resolved photoemission spectroscopy. That usually is done either using laser or using synchrotron facilities. You can do optical excitations also to observe quasiparticles, because quasiparticles are the excited state of the superconducting state, and there are different ways you can excite them. You can excite them with photons, with different photons of different energies. The superconducting system can absorb the energy and create quasiparticles. There are different quasiparticle spectra that you can study. But then, usually, it's a function of frequency. Then with STM, you're doing it in the DC limit, so there's that difference. We can also add optical excitation to it too.
ZIERLER: If you can explain, it's just a nomenclature question, what is doping dependence mean as it relates to cuprate superconductivity?
YEH: "Doping" means the following. High-temperature superconductors, if you don't dope the system with carriers, it's an insulator. Then, you can change the chemical composition of the system, let's say, change the oxygen content or add some elements that will contribute carriers to this insulator. Then, the material becomes more conducting. It was an insulator. It was not even conducting at all. Then, you can add carriers, and there are different ways you can add carriers, and that's called doping. You can dope it either with electrons or with holes. You can dope this insulator into a conducting material either with holes or with electrons. You can do it that way. That's called doping.
Now, when you dope the system, it's so that you're increasing the density of carriers. When the density of carriers reaches certain thresholds, then the system becomes conducting, and then it can also become superconducting. When you keep doping, initially, you start having superconductivity, then we call it the "underdoped" region because the superconducting transition temperature is not very high. You keep doping to a higher and higher carrier concentration. Then your superconducting transition temperature reaches a maximum, and the doping level corresponding to the maximum superconducting transition temperature is called the optimal doping. If you keep doping, you get too greedy, you keep doping then the system becomes more like a metal. But then the superconducting transition temperature drops down, and then that's called the "over-doped" limit. Then, when you really dope it too much, it becomes a metal. It won't become superconductors anymore. That's what we call doping because this material started as an insulator, actually.
ZIERLER: Nai-Chang, in our very first conversation, we talked about your affiliation with the IQIM. In that 1990s, were you aware of the development of information theory, quantum information theory, things that people like John Preskill were talking about, or did you only become aware of this later on?
YEH: I was fully aware of it. I was fully aware of it because it was kind of fun to watch. In the beginning, it was all at the theoretical level, and then some experiments were done, mostly in cold atoms. It was only much later, the 1990s, that theory was far ahead of experiments for some time. Then there were these different proposals, and then people started trying to do experiments. People started trying to do experiments, follow the theoretical ideas, and see if they can design qubits, and demonstrate the quantum information computation part.
ZIERLER: Did you recognize early on that condensed matter experimentalists would have a hand in pushing through some of these advances in what was originally just a theoretical pursuit?
YEH: Actually, experimentally, I could see already a lot of potential, because there were different systems like quantum dots, etc., and then, of course, superconducting qubits. Also, people were thinking about spin qubits. There are different solid-state systems because the qubit ideas, basically, you just need to create a two-level system with their energy levels far away from other energy levels. In a solid-state system, there's so many things you can do to create two-level systems. It's just that qubits are not just about two-level systems. Then you have to reach coherence. You need to have phase coherence, and you have to minimize losses, etc. It's not so trivial. But, to me, I could see that solid-state systems have a lot of potential. It's just that I had no idea how good it could be. It was not a surprise.
ZIERLER: I asked earlier about your sense of timescales for breakthrough in superconductivity. I was thinking about the review article you wrote in 2002: Recent Advances in High-Temperature Superconductivity. What were those advances, and how did they relate to the things that you were thinking about over the past five or seven years?
YEH: The advances at the time, I already mentioned, I think, at the time, that I was already thinking about competing orders, etc. Then those, I think, became much better established. But in terms of applications, there are some advances in materials development, and so people were able to use high-temperature superconductors to make record-high superconducting magnets, DC superconducting magnets in Tallahassee National High Field Magnet Lab. I'm an external advisor for the lab, so I watched their development. It was very impressive, how they overcome the materials' difficulties to achieve that. Those all happened later, after I wrote that article. Now, I don't quite remember what I considered as the frontier [laugh] at the time then. It was quite a while back when I wrote that article. I probably should take a look. But, at the time, anyway, a lot of things are becoming possible or better established. But, still, the mechanism, the theory for the mechanism is still not there.
ZIERLER: What do you think it will take to get there? What's the breakthrough that we're waiting for?
YEH: I don't know.
ZIERLER: Of course, if you knew, we'd be there. [laugh]
YEH: Right. I know the general direction, but to work out the theory, because it's such a complex system, it's very nontrivial.
ZIERLER: Are there computational limitations that we currently face, given the complexity?
YEH: Theorists are trying to have better theory now through computational power. They're trying to do that, because the system is so complex, any analytical approximation just is not adequate, generally. It's nothing like conventional superconductors. Conventional superconductors, things were relatively simple so some approximations took were justifiable, and still gave the overall global good picture, a consistent picture. But in high-temperature superconductors, it's just not so easy. Theorists are working on using numerics, numerical simulations, computations, doing better and better computations to lead to a better and better understanding. Maybe at some point, we can reach that level. But I think it may take computational power to truly understand exactly what's happening. [laugh]
ZIERLER: Is it possible that it will take quantum computational power to get there?
YEH: Maybe we don't need that. [laugh]
ZIERLER: [laugh]
YEH: Hopefully not. [laugh] I want to see the answer when I'm still around. [laugh]
Scientific Advances in Taiwan
ZIERLER: That's right. We want a shorter timescale than that. Nai-Chang, just a fun question. You were awarded the Distinguished Alumni Award from National Taiwan University in 2003. Is that similar to the same exact wording, the Distinguished Alumni Award that Caltech confers, where it's basically—
YEH: Yes.
ZIERLER: —the equivalent of an honorary doctorate?
YEH: It's not an honorary doctorate degree. It's just an honor given to physics alumni. But I was very honored. I was kind of flattered that I was receiving that award because there were just so many distinguished alumni from the physics department of National Taiwan University. I think the fact that I got tenure at Caltech played a factor, I guess [laugh], because they consider that pretty much an accomplishment also, I guess. [laugh] I don't know.
ZIERLER: It must have been so nice to go back. In what way had the department of physics changed since you left?
YEH: It changed a lot. [unrelated conversation] It has changed a lot. When I was a student, Taiwan was still under the martial law, and Taiwan was not very prosperous. That was the time when Taiwan was just starting to develop a semiconducting industry. It was before the semiconducting industry became the dominant enterprise in Taiwan. Taiwan wasn't very prosperous at the time, and we were still under martial law. Normal citizens could not go abroad unless you tried to go abroad studying, or you had business. Travel was not automatic if you wanted to go on an international trip, because it was under martial law. It wasn't very prosperous, so our department at the time didn't have very good experimental facilities, and most professors were theorists, and there were few experimentalists. Then the experimentalists had to build their own equipment most of the time. They couldn't buy very expensive things. At the time, basically, the best students always thought they would become theorists because theorists didn't need a whole lot of money [laugh] to do experiments. But, now, they're extremely well-funded.
They have a lot of good people that got their PhDs from abroad and went back. Some universities have their own Taiwanese PhDs who became professors also. Then National Taiwan University has become very, very well-funded, so they have actually more research money than a lot of professors in the US. The US funding situation is generally very bad. In Asia, it's a lot better. The governments give much better support to these top universities. Here in the US, we have to find every penny we spend, and then we have to pay for everything. Then we have to pay a huge overhead to Caltech: 68.4% overhead. In Taiwan, I think, their overhead is below 20%, and in most places, the government will give funding to universities to begin with. Then universities divide the money up and give it to different departments so they have basic operation funding. Then, if you want to do more, you write proposals. But the proposal success rate is much, much higher. Here in the US, it's usually below 10%. In Taiwan, I think, if you're coming from a good university, you'll probably have a success rate between 30% to 50%. In China, if you're from a top university, after you get all of the basic operation funding, your proposal success rate is 90-something per cent. The top university professors in Asia usually will be swimming in money, but in the US, we are professional beggars. [laugh] We really work so hard just to get a little bit of money.
ZIERLER: Nai-Chang, as you explained in an earlier discussion, you really had no choice but to leave Taiwan in order to pursue physics at the level that you wanted to. In light of the comments that you're making now, is that necessarily still the case. In other words, if you were an undergraduate at National Taiwan University today, do you think you'd still feel compelled to leave, or has the situation really changed?
YEH: Now, they have a lot of good professors so, indeed, a lot of students will stay in Taiwan. But if you want to do the very best, you should still come to the US. That's what the top students still do. That's the same in China.
ZIERLER: Despite all these problems that you mentioned, it's still the best here?
YEH: Right, because it's just I think the US has built this tradition of incredible creativity and thinking out-of-the-box kind of approach, and the environment is so nurturing for great ideas. That's a wonderful thing about it. I can tell you a little story. In 2007, I was invited back to Taiwan, to National Taiwan University, to teach an intense summer course. I taught this course, quantum field theory for condensed matter physics. Me, being an experimentalist, I went back to teach a purely theoretical course. It was incredibly popular, so I would be teaching in a seminar room, and it was jam-packed, and it wasn't just all the students from National Taiwan University. There were even students coming from the southern part of Taiwan who came to my course, learning that course during that summer, because nobody had offered a course like that before.
Quantum field theory is usually taught for high-energy physics, particle physics. Never ever have people taught it for condensed matter physics like I did. But, coming from an experimental background, I taught it in a much more practical way because I knew what experiments I wanted to do, and then how I would apply this theory to analyze data. I had a practical perspective, totally different from theorists. That's why that course was so popular. I taught that course. I taught it for 40-something days—I forgot—two hours a day. It was just lots of lectures, and very intense over I forgot how long; maybe six weeks or something. Students really, really liked it. I taught two hours a day, and then they would ask questions for another hour. [laugh] Students would just surround me, and ask questions, and they love to learn. Then I taught that course. It was a subset of the course, a subset of what I taught at Caltech for over a year. But this was an intense course I taught every day, so I jam-packed it into over a month, and so I only used a subset of it. I remember these students in Taiwan. That was the time most Taiwanese students decided that they would not go overseas because they felt that they could get a very good research environment already, and they would just stay in Taiwan. Taiwan was so comfortable for most young kids. They didn't want to speak English every day [laugh] and then work damn hard in a lab or something in a different environment. But after I taught that course, students asked me, "Is this what Caltech students get?" I said, "You're only getting a subset of it." That year after I taught that course, that was a period of time already when top students would not leave Taiwan. But after I taught that course, continuing for several years, the very best students all left Taiwan, and came to the US, came to Caltech, MIT, Harvard, and other schools, because they felt that they still have to go overseas to broaden their horizons. I remember students told me they thought they were so good already, and then they realized, "No, not yet." [laugh]
ZIERLER: [laugh] Nai-Chang, another nomenclature question, the phrase "competing orders and quantum criticality," first, what is quantum criticality?
YEH: Quantum criticality refers to a special point at zero temperature when a phase transition can take place. Let's say you can plot some kind of phase diagrams as a function of temperature and, let's say, doping level. As a function of doping level, I was saying that if you dope high-temperature superconductors, and one phase transition temperature you will look for is that you cool down in temperature, and below a certain temperature for certain doping, you will see a superconducting transition temperature. You draw a point on that temperature versus doping level phase diagram. Then you go to a different doping, and you get a different transition temperature, so you draw another point. Then you have some points connected. You'd look at temperature versus doping level, and below a certain curve that I said that are drawn by putting all those dots on that two-dimensional plot, below that phase, below that temperature, below a certain temperature and doping level, then you will get superconductivity. But I did mention, for instance, if I keep changing the doping beyond a certain point, superconductivity either vanished in the over-doping limit, or vanished in some under-doping limit. Those points then, basically, the superconducting transition temperature is zero temperature. Then you can imagine now you make a point, you make a diagram of temperature versus the doping level, and then you will have two points that would be associated. One is on the over-doping side. The other is on the under-doping side. But those will be the two points at zero temperature, two special points that correspond to two special doping levels. Each one of those is like a quantum critical point, for instance, because that's when you go beyond that point, you go from one phase to the other at zero temperature, and so that would be a quantum critical point. But the variable doesn't necessarily have to be a doping level. A lot of materials you can have, let's say again superconductors, you can plot temperature versus pressure, and then some superconductors, they're not superconducting until you go apply a pressure. Again, you can plot on the temperature versus pressure diagram. You can have some materials. You have some superconducting transition. Then you have some temperature versus pressure. Then you'll make some plots.
Some of these materials may not be superconducting at all at any zero pressure. But then you can start applying pressure, and then, suddenly, superconductivity will show up. You can then start thinking that you could also achieve some critical pressure above which you can create superconductivity in zero temperature. Then that point would be a quantum critical point also. A quantum critical point basically refers to a special point in the phase diagram that above or below that point of some quantity, either pressure or doping or something else, that you change the phase from one phase to the other. But that special point occurs at zero temperature, so it's called a quantum critical point.
ZIERLER: Nai-Chang, what was some of your work relating to thin films around this time?
YEH: I also worked on thin films. I made thin-film superconducting materials. I also made thin films of the perovskite magnetic materials. I did make thin films using pulsed laser deposition and created different thin films on different substrates. I did do a lot of those things at that time.
ZIERLER: How does one characterize a thin film? What are the parameters?
YEH: It depends on what properties you are referring to. Are you talking about the thickness, or you're talking about the superconducting properties, or magnetic properties, or transport measurements? There are different things you measure. If you're just talking about the thickness then, of course, you can easily make the thickness measurements using a profilometer, for instance, or you can use an atomic force microscope or something to scan across from where the thin film is to where the thin film is not, and then you can measure that step. If it's sufficiently thin, then you can use an atomic force microscope. If it's thick enough, you can use something called ellipsometry. It's an optical technique to measure what the thickness is, judging from the reflection, because when you have the thin film on a substrate versus when there's no film on the substrate, your optical reflection will be different. You can use ellipsometry also. If it's thick enough, then optical techniques would work. If it's very thin, then you can use atomic force microscope or profilometry. You can measure the thickness, but you can also measure many other properties. Like, you can make electrical contact to the material, then you can measure superconducting properties and electrical transport properties such as resistance and the Hall effect.
ZIERLER: Nai-Chang, last question for today, and this will serve as a segue for our next discussion. In the 2000s, the early 2000s, what were some of the advances either technologically or simply things that you were interested in that got you more involved in the field of nanotechnology?
YEH: At the time, I was talking about scanning tunneling microscopy and spectroscopy. Actually, I stumbled into that because I was a postdoc at IBM. Then IBM, I told you, they started letting go of a lot of researchers. Then they had a homemade STM, so my manager when I was a postdoc there, one of the two managers owned this STM. Then he didn't have use for it anymore because the people who were working on it were gone. He said, "I can donate this STM to Caltech, to you." [laugh] That was a homemade version, and it was very old, so I had to revive it and did things on it. But that was when I started learning STM.
When you do STM then, naturally, you go down to atomic scale, nanoscale, atomic scale. I started paying attention to things that were really atomically resolved and also at the nanoscale. That was the time when scanning probe microscopy was rapidly developing, so STM was one. Atomic force microscope was another. Then a lot of scanning probe microscopies, including scanning electron microscopy, that can do nanoscale studies started becoming quite popular. There were these frontiers that just to characterize things using these probes was interesting. But, of course, that was also the time when nano started becoming more and more important. Nanomaterials, to me, were interesting in that when you go down to that kind of scale, quantum mechanics, quantum physics is naturally there. Generally, you can easily find interesting things to explore at nanoscales. It was the technology that was developing at the time but also, because of a lot of physics that I was studying, I started paying attention to that area.
ZIERLER: Wonderful, Nai-Chang. On that note, we'll pick up in the 2010s, and we'll bring the story right up to the present. Thank you so much.
[End of recording]
ZIERLER: This is David Zierler, Director of the Caltech Heritage Project. It is Tuesday, November 22nd, 2022. I am delighted to be back once again with Professor Nai-Chang Yeh. Nai-Chang, as always, it's wonderful to be with you. Thank you so much.
YEH: Pleasure to be with you.
Quantum Computers Need Nanotechnology Breakthroughs
ZIERLER: Nai-Chang, we're going to pick up today in the 2010s from our last conversation. I want to return to a topic we've previously covered, but we'll do it chronologically now. When the Institute for Quantum Information added the "M," the matter, and it became the NSF Center, IQIM, Institute for Quantum Information and Matter, I'm curious if there was anything you were doing at the time, either at a theoretical level or an experimental level, that contributed to this development where condensed matter and experimentation now had a greater role to play in the quest to create a scalable quantum computer.
YEH: More generally about my research at the time, I had always been working on superconductors. As you know, superconductors are one of the most important ingredients for making qubits. But then also I was working on low-dimensional materials like graphene at the time, and those are also promising for a lot of scalable nanoscale electronics. Naturally, I worked in areas of strongly correlated materials. Those are quantum materials. Low-dimensional systems are also quantum materials. Naturally, when they expanded—"they" meaning the original Institute for Quantum Information—expanded into materials areas, quantum materials, then my research actually fit into that general direction. Of course, superconductors and a lot of quantum materials are still at the frontier of making a difference for not just quantum computation, qubits, but also for quantum sensors, quantum networking, quantum communications.
ZIERLER: Nai-Chang, I wonder if you could explain what the overall connection is between advances in nanotechnology and advances in quantum computation.
YEH: If you want to use solid-state systems to do quantum computation, then naturally you need to make qubits, you need to make the circuits, you need to make connections, and you need to do quantum entanglement, and you need to enhance signals and filter signals. All of those require nanofabrication. You take whatever materials you use, and in this case maybe superconductors and other materials, thin-film materials, and then you try to make quantum circuits, and then you have to do nanofabrication. You have to turn them into things on the chip so that they can be cooled down in dilution refrigerators, and you want to build many chips and all the circuits. All of those things require nanofabrication. There's no escape.
ZIERLER: When quantum information became advanced enough that it was not purely a theoretical pursuit as it had been starting in the late 1990s, I wonder, from your perspective, if that made the achievement of producing a quantum computer in a scalable fashion, if it made it seem like a more realistic scenario now that we're actually thinking about engineering and materials.
YEH: Yes and no. To make a true quantum computer, my personal view is that you have to do it by means of solid-state devices. You cannot use cold atoms or chilled ions. You cannot use those systems to make a truly scalable quantum computer. Now, if you want to make truly scalable systems, you'll have to do it with solid-state systems, as I said. But the current solid-state systems, the only mature technology is really superconducting qubits. There are other competing systems. People are thinking about using spintronic systems or quantum dots, or diamond NV centers, things like that. But those are not yet mature and scalable. Currently, the only scalable and mature system is superconducting qubits. Unfortunately, though, the current superconducting qubits are based on low-temperature superconductors, and then they have to be cooled down to very, very low temperatures using dilution refrigerators. For that reason, there are only a limited number of qubits you can cool down in one dilution refrigerator. You can try to build a jumbo dilution refrigerator, but there are only that many qubits you can cool because, for each qubit, you need to have all of those assisting circuits and design electronics. It's not truly scalable. You cannot cool millions of them in one dilution refrigerator.
If you want to build many, many dilution refrigerators, then you have to think about when you cool down all those qubits in one system how you actually communicate that information with other dilution refrigerators. Those are not that scalable yet with the more mature technology. Yes, people can realize a certain number, tens of qubits, and then make them do certain things. There are a lot of demonstrations of concepts, etc. Those are all developing. But I think, in the end, for true scalable quantum computers, I think we still need materials breakthrough at some point, that is, if somehow we can find the right kind of materials that we can make superconducting qubits, and they don't require such low temperatures, or even the cooling requirement is not so strict, or maybe not even cooling is needed, then that's when true quantum computation can be fully realized. Right now, there are a lot of developments, promising developments, progress. But I think we are hung on the material, and so [laugh] for quantum information to move forward, materials development will be very important too. That's my view.
ZIERLER: Nai-Chang, in light of your comments and the various ways that certain companies are pursuing quantum computation, doing it in very different ways, I wonder if you see, for example, Microsoft's approach versus Amazon's approach or other companies, if you see a particular approach as the most feasible.
YEH: I think currently, for instance, the Amazon approach is still based on superconducting qubits. All of the advantages and disadvantages I just mentioned are still there. They're still based on these very low-temperature superconducting materials, and so the limitations are still there. You can push them as far as the cryogenic cooling capabilities, the money [laugh] there is, and then maybe some clever design in the electronics, some proper engineering. All of those things can help. But I don't see in the near future we will have millions of qubits working together. It's still not there because we are hung on the materials. Microsoft pretty much, again, those are all similar approaches. They're all based on superconducting qubits and superconducting circuits, so the same constraints are there. I think it's a heroic effort, and I think they're making good progress globally, also, and a lot of good people are jumping into it, trying to make a difference. But my own assessment is that, yes, we can advance to some extent. But, in the end, the material is still the bottleneck, the limitation of the material.
ZIERLER: Nai-Chang, more generally, I'm so fascinated by quantum information because it provides reflection on the various ways that academia, industry, and government can come together for these shared goals. What do you see as the key strengths in each of these areas as we work toward the development of quantum computation? What is the best way that academic scientists, government scientists, and industrial scientists can play on their strengths in this regard?
YEH: Academic researchers, of course, they can concentrate on the research aspects looking to scientific issues. Whereas industrial people, they have to look at things from the perspective that, in the long run, things have to be scalable, profitable. They have a different angle of looking at things in a more practical and scalable way. I think government, because these things can be very expensive, at least for the material right now we are dealing with, they're not the most ideal material, and it costs so much to cool them down to make the devices. Government, of course, can come in with a more coherent effort and funding support. All three areas of the players are important to push this thing forward because it's highly nontrivial. It takes a lot of talents and effort. You need to have funding. You need to have organization. You need to have practical views because if you just leave things to academic people, they will just dive into whatever is fun and interesting. They won't even think about what's scalable, profit… commercially realizable.
But you have industrial people who come in, then they would take a more practical view. But that also takes some ingenuity to understand how to optimize things, to make things usable, and make things that could be integrated into real world. That's the important aspect when you have industrial people coming in. All those aspects are important. Also, government is also important in that the implication of quantum information and technology can be also highly relevant to national security. [laugh] You also want to have government coming in and looking at it from that perspective so that the research is being supported, and then we need to have some guardrails to make sure that US interest is also protected. At the same time, you want to have scientific openness but, at the same time, you want to address other issues too.
Delegating Scientific Applications
ZIERLER: Nai-Chang, for you personally, have you ever seen opportunity to get involved in entrepreneurial pursuits where you can apply some of the technological innovations in a startup context?
YEH: Oh, many. Actually, a lot of things I do have the potential, and so I have lots of patents already. I have been asked, actually, by people to see if I would be interested in running a start-up company. But that's not what I am interested in. I'm very interested in seeing science turn into technology, but I don't want to run a company. To me, it's just not something I want to spend my time on. It's too complicated. You have to raise the money. You have to work with the people. You have to deal with all of the legal issues. It's just not my cup of tea, so to speak. But I have students who are excited about that possibility, so now I have a few students who are thinking about getting into—a couple of them have graduated, and then there might be a couple who are interested in that possibility. They can do a start-up. Also, I have collaborations with big industry also. I have collaborations with industry. To me, the industry can take what I do [laugh], and turn it into products, and let them do the management and the manufacturing and all that. But that's just not what I particularly enjoy. I don't mind interacting, collaborating with people from industry, but I don't want to run a company myself. It's just not my type of thing.
ZIERLER: What are some of the technologies that show the most promise in the marketplace? What are some of the translational opportunities you might be looking forward to?
YEH: You mean currently with what I do?
ZIERLER: Yes.
YEH: Plenty, like the area of graphene that I'm working on. Graphene can be used in flexible electronics, and also even biodevices. Actually, there was a recent special interview of me and my research group on the Caltech website. It was related to my collaborations with Industrial Technology Research Institute (ITRI) in Taiwan. That's the organization where TSMC originally came from. [laugh] I collaborated with them, and we demonstrated that we could use graphene on nanoelectronics. The incorporation of graphene can benefit the durability, mechanical strengths, the conductivity, and the possibilities for better flexible electronics. I'm also planning new collaborations with ITRI on the next generation, 5G or 6G communication capabilities, by incorporating graphene. But graphene itself, also we have found—and this is something related to a student of mine who graduated, who just got some seed funding for a start-up—we had found extremely interesting optoelectronic properties related to graphene, so they could be used for nanolasers and for nanophotonics, potentially. That would be for information and communication technologies. They can also be used for other things, for passivating materials, for superlubricity, for energy, for lithium-ion batteries, for supercapacitors, energy storage, and even for photovoltaics, nanolasers. There are all kinds of possibilities in those areas, so I can see why my students want to do start-ups [laugh] and turn things into technology, and I'm fully supportive of them. I'm happy to give them my contacts. But, again, I don't want to run a company. [laugh] It's just not my thing. [laugh]
ZIERLER: Nai-Chang, there's so much excitement over the possibilities in these technologies. For you personally, not just beyond the potential for business success, what are you most excited about in terms of benefiting society? What are some of the technologies where you see real potential that it will simply help people?
YEH: Actually, what I was just mentioning, information communication technology. If we can make things better and a lower cost in energy, low-loss communication information technology will be very important for the future because more and more advanced electronics and photonics are costing more and more energy. You want to reduce the energy consumption. That, for one thing, you can reduce the energy use if you use better and more advanced technologies. Then, of course, for energy generation, energy storage, all of those can be useful for sustainability. Actually, some of the materials I'm using can also be applied to medical use, and also for desalination, filtering, detoxification. There are a lot of things that can be useful, and also for energy generation, the energy storage, energy generation, more advanced information, communication, technology, many things. There are many, many things that can be beneficial to the society. If you want to ask me what's beneficial for the society, then I talk about the application side, the technological side. But, to me, there are also a lot of very interesting fundamental things that we are exploring. Those are pushing the frontiers of knowledge that people have not explored before. To me, that's also exciting. What's the best for the humankind? It depends on your view. I like to look at it from intellectual perspectives, but I also understand that we can talk about technologies that can certainly benefit people.
ZIERLER: Nai-Chang, I wonder if you could tell me a little bit about some of your more recent work with topological insulators.
YEH: Topological insulators. This is more fundamental [laugh] so far. We have done different things on topological insulators. We have investigated topological insulators, which are insulators in the bulk, but they have some surface states that are almost like perfectly conducting. The surface states are reflecting the topological nature of the bulk, but they exhibit very different electronic properties and magnetic properties from the bulk in terms of its conductivity. We investigated different types of topological insulators, and then we also found that if we introduce magnetic species into topological insulators, then we can turn the surface state from originally un-gapped to a gapped state, and a lot of other interesting properties. That's what we do with the topological insulators, just studying their special characteristics in the presence, how the surface state evolves from a gapless to a gapped situation when you introduce magnetic impurities, and then a lot of detailed properties. But they can also be turned into interesting devices but not necessarily very useful. That's the topological insulators. But, more generally, if I talk about topological materials I try to study, I can actually build, I can actually create artificial topological materials. Using the combination of low-dimensional materials and nanofabrication technology, I can actually create new topological states. I can also use light interacting with novel materials to create topological states. Those are the more fun things. You asked me about topological insulators. That's a given material. They have fixed properties. But then if I take other materials, and I start playing games, and then turn them into topological materials or topological photonics, I find that even more exciting. I can create topological electronic properties, topological photonic properties out of nanofabrication and quantum materials. This is called playing God. [laugh]
ZIERLER: [laugh]
YEH: I create new systems that I want, and that's even more fun.
ZIERLER: I'm intrigued by your use of the phrase, "playing games." What would it mean to win? What does the game look like as an end goal?
YEH: The end goal is that I create the properties that I want. [laugh] I want certain properties, and then I actually can design things and create them. But the even more exciting thing is this. For us who have done research for a long time, if you make some predictions, and you design things, and if you only see what you get that's agreeing with what you predicted, then that's no fun. It's OK. [laugh] But then you learn nothing new. What's even more exciting is that, generally, often I will be designing something -- I have some ideas in mind, and I kind of predict what I will see. But most of the times, because I'm heading towards the right direction, I will be seeing above and beyond what I expected, and then I learn a bunch of new things.
ZIERLER: Which is always the goal?
YEH: Right. You say, "What's the game?" The game is that I want to learn more. I want to create things that I want, but then I want to learn from what I created, more about frontier knowledge that I had not even been exposed to before, and that's even more exciting.
ZIERLER: Which probably is a prompt for future research that you couldn't even imagine until you got to that level?
YEH: Yes. We have seen a lot of exciting new things recently. It was because I designed things in special ways, but then I'm seeing things far beyond what I even imagined. I saw things I wanted, but then I saw a lot more. This is always a humbling experience when you do research because it's an endless, never-ending pursuit. It's a never-ending quest to pursue new knowledge, to pursue the new frontier, and being surprised and humbled every time when you do new things. That's the fun part. [laugh] That's really the fun part.
ZIERLER: Nai-Chang, just the technical question. Your work on pseudomagnetic fields, what does that mean? What makes a magnetic field a pseudomagnetic field?
YEH: You know how typically a magnetic field is generated? Either you have permanent magnets, and you generate a magnetic field, or you have to use a lot of electricity, use electrical current to create a magnetic field. When a magnetic field is created, you break the so-called time reversal symmetry. You have a magnetic field on in one direction. Then if you reverse the time axis, the symmetry is already broken. If you have magnetic field applied along one direction, you already break the global time reversal symmetry. A pseudomagnetic field is that you don't have to create them out of using all these external means. You can effectively create local magnetic fields, but the local magnetic fields would keep changing sign. You can go from positive to negative. If you integrate the magnetic flux out of a given area, then total magnetic flux is zero because you have alternative positive and negative magnetic fields in different places and you also have different strengths. The total magnetic field is zero. Total magnetic flux is zero. You don't break global time reversal symmetry. However, if you go to local regions, then local regions, you can have positive or negative or zero, whatever fields. Locally, you can break local time reversal symmetry. That's what's called a pseudomagnetic field. What we have been able to do is that we use nanofabrication technology to distort graphene at the nanoscale, and then we could create these enormous local magnetic fields to hundreds to even up to near 1,000 tesla locally. But they are inhomogeneous, but they have spatially varying patterns that we design. Then if you integrate over all of the magnetic flux again, you get zero. You don't break global time reversal symmetry. But locally, you have such enormous effective magnetic fields, either positive or negative. Therefore, you change the electronic properties of the material in a fundamental way. Then new physics, new properties show up in this situation.
ZIERLER: Nai-Chang, I'm curious. Photonics, of course, is a mature field. It's been with us for quite a while. In what ways has a nanotechnological approach made photonics feel like a new field where there's very exciting things happening?
YEH: Photonics, when you use nanotechnology, think about photons. You talk about photons. Usually, if you are talking about infrared photons, like what you use in telecom, they're about 1.5 micrometers in wavelengths. You're limited by the wavelengths. That's not a very small scale. If you talk about visible light, it's between 400 nanometers to 700 nanometers. All of these things, the wavelengths, if you talk about photonics, these far-field photonics, they're by no means nano at all. However, you could play with materials that have a negative refraction index like metals, like certain metal surfaces, and you make them small. You make them small. You can actually confine light, change light down to nanoscale dimensions. Think about it, all these wonderful things we have been using photonics for. Now, if you can build photonics into a chip, nanolasers, nanotransducers, nano whatever, optical fiber, you confine things down to nanoscales, and you can confine things onto a chip. That's very useful, you can already imagine, very useful for many things. Also, another very interesting recent applications of nanophotonics I found was that people are trying to think about—you want to study the signals in the brain of neurons. Neurons would have basically electrical signals and photonic signals. But then if you want to study the brain, you'd better make things very, very small into the brain if you want to detect and analyze neuron signals, how they communicate under different stimuli. You just make photonic things that are usually in the microns, hundreds of nanometer range, down to a chip, a small sized system, and then you can do all kinds of photonic properties and manipulations on a small chip. The potential applications are boundless.
ZIERLER: I'm curious the ways in which you've seen Raman spectroscopy offer new possibilities for your research.
YEH: Raman spectroscopy is always very useful for many things because if you study materials, different materials, they will have different phonon signatures, and different phonon signatures will be manifested in Raman spectroscopy. Raman spectroscopy is always useful if you want to look at the quality of your materials. It's a very convenient way of looking at the quality of your materials in a non-destructive way because you just shine light on it, and you look at light scattering. Then the materials will have certain signatures, Raman modes, and so the scattered Raman signals will be indicative of the quality and the characteristics of your materials. That's something very, very commonly used. But Raman has another thing that, even if you don't have a whole lot of material, you can also still—for nanomaterials, you don't have a whole lot of thickness and quantity. You can still do Raman spectroscopy to figure out the quality and the characteristics of the materials, so it's very useful in nano systems and also in low-dimensional materials.
ZIERLER: Nai-Chang, I know using cryogenic scanning probe microscopes has been very important for your research. Have you been involved in actually developing the microscopes themselves, or are you simply an end user?
YEH: I build them from scratch.
ZIERLER: You do?
YEH: Yeah. [laugh] I build atomically resolved scanning probe microscopy from scratch.
ZIERLER: Is that because they're simply not available, or because you have specifications that you want to control?
YEH: At the time when I started research using scanning tunneling microscopy, they were not commercially available, so we just built them based on the physics we understood, and we collaborated. We got information from people who had experiences. Then when we became good at it, we could design things to our specification. But, of course, it takes a long time to build instruments from scratch: You have to build these things that are atomically resolved, and you need an extremely clean environment, electronically, vibrationally, or otherwise. We are talking about atomic scale resolution. If anybody sneezes, and if you don't have proper vibration damping, and then forget it, everything will be all ruined. But building things, at a time when I started, there were no commercial systems. But then later on when commercial systems became available, they were outrageously expensive. They cost millions of dollars. I was already an established professor. Nobody was going to give me start-up funds of $1 million to buy brand new equipment, although my younger colleagues were able to make such requests and got commercial [laugh] systems completely made for them. That's a different time, different story. But, on the other hand, the fact that we were able to build things from scratch of course implied that we have the capability of adding new components to our homemade instruments.
Currently, I'm adding light to my system. Typically, if you want to purchase those things commercially, probably it would cost about $2 million. We have our homemade system, and so we can add light. We just need a few tens of thousands of dollars. Then we are able to add new components, new capability. The other good thing about building our own instruments is that because my students have to learn how to build these things, they know everything inside out. They know how to optimize the operation of these advanced tools. That's much better than people who are given a commercial system. They may not know everything. Sometimes, there is a danger with not understanding your tools because these are very sophisticated tools. If people don't understand their tools, they could be seeing artificial signals without knowing that they're seeing artifacts. But it costs us time, a lot of time to build things. In an ideal world, you want somewhere in between. [laugh] You want to have some convenience of buying things and, yet, still have some understanding and hands-on experiences knowing your tools.
ZIERLER: That's the perfect balance, you're saying?
YEH: Right. [laugh]
ZIERLER: Nai-Chang, you mentioned sustainability previously, and some exciting possibilities in nanotechnology. Do you see advances in graphene as contributing specifically to developments for solar energy?
YEH: Yeah. Actually, there is that possibility. It depends on how you use it. We are actually seeing very interesting potential of using graphene-based materials for harvesting energy. But we haven't commercialized it or something. We just see hints of such possibilities.
ZIERLER: Why is that, Nai-Chang? What can graphene, at least theoretically, what can it do better than other materials?
YEH: Typically, when you want to harvest photons, you use semiconductors. Semiconductors have an energy gap, and so if your photon energies are larger than the energy gap, then you create an electron and a hole in your system. You excite an electron from the valence band to the conduction band. Before electron and hole, before they are recombined, if you can capture the electron or the hole into currents, then you have photovoltaic behavior, and so you can harvest the current, the electricity. Graphene has no energy gap. When you use semiconductors, and find the energy gap, you can harvest the energy of photons that are bigger than the energy gap. But anything smaller than the energy gap is wasted. You cannot harvest energy within the gap. Graphene has the advantage that any energy can be excited and then supposedly harvested. But the problem is without an energy gap, usually the process of recombination of electrons and holes and then the reemission of photons again is extremely fast, so you have no chance of capturing the electricity. However, if you use nanomaterials of graphene, then that's a different story. Nanomaterials of graphene, it turns out, you can still absorb all of the light, and you can actually trap the photons inside the nanomaterials. That's what we found. If you can trap the photons, then you can figure out a clever way of capturing, of turning these photons into electricity. Then there is a chance that you can have a very highly efficient system to convert energy because all energy scales can be captured if you do it right. But it's easier said than done. That's the good part about graphene. But the negative part about it is that the recombination process usually is very fast, and that's why you have to do something like nanomaterials so that you can trap photons and enhance the material and light interactions to keep the lifetime of these excited electrons or holes longer. Then you have a chance of extracting that electricity out before they recombine and turn themselves back to photons.
ZIERLER: Nai-Chang, the process of forming graphene, something that you've thought a lot about, is the field still new enough where there are different schools of thought, different methodologies about the best way to produce graphene, and you represent one of those schools, or is it more unified at this point?
YEH: There's nothing unified about it. It really depends on the kind of applications you want to do. For instance, we do PECVD, plasma-enhanced chemical vapor deposition method, and this is the method that you turn greenhouse gas methane into useful material, high-quality graphene. This is a very, very good way of getting the highest quality graphene, and also it's a scalable approach, but it's not cheap. That's one thing. This method, I would say, is the most flexible method because we can grow graphene on all kinds of different substrates, and even on nanostructures, very successfully. It's very good for a lot of applications, but it's not a cheap approach. It's a scalable method, so if you have a large PECVD cell, and that's a technique that has been used in industry, you can do this on a large scale. But the cost is higher, but the quality is the best, and it has the highest flexibility. But it all depends on the application. For instance, if you just want to create a lot of graphene flakes, and use them so they don't have to be the purest they can be, you want to blend them into different materials like polymer or other things, then our PECVD method may not be the most effective way of growing graphene. People use different ways. They take graphite, and then they go through a very horrible chemical process to exfoliate graphene into tiny flakes. In that case, it's cheaper; it's fast. You can produce a large quantity of not very high-quality material. But if that's good enough, that's OK. It depends on your application. But then if you want to grow very large sheets, then people are using chemical vapor deposition. But that's a high-temperature process, and it takes a long time. It's also not cheap. Then, of course, there are these people who just use Scotch Tape techniques, but that's absolutely not for application. That's only for publication [laugh], because you cannot exfoliate billions of devices. In your cell phone, you have hundreds of billions of transistors. Just forget it. You have to hire not only all humankind on Earth but also a lot of aliens to do the exfoliation. [laugh]
ZIERLER: [laugh]
YEH: It just won't work. [laugh] There's just no way. But that's a way of doing scientific studies, tiny devices, prototypes. That's fine. But that's not for applications. For applications, there are different approaches. It depends on the applications. But I would say what we do, PECVD is probably the most flexible approach to get a high-quality material.
ZIERLER: Nai-Chang, another technical question. Your work on Dirac spectra, what are Dirac spectra, and I wonder if you see a particular connection to what Paul Dirac did?
YEH: Dirac spectra, it means that the particle that you're interested in, electrons or whatever, the energy versus momentum dispersion relation is linear. That's called a Dirac spectrum. For most materials, this energy-versus-momentum relationship usually is more quadratic, so higher power than linear. Having a linear spectrum, there's one thing that's well-known to be a linear dispersion relation. That's photons, light. [laugh] Your energy and momentum are exactly proportional to each other with a coefficient of speed of light. That's a Dirac spectrum. When materials are having Dirac spectrum, usually, that's due to many-body effects, the special band structure effect in materials. When you have a Dirac spectrum, what that means is that your electron has no mass. Effective mass is zero. But that's an effective mass. You take the electron out of the material, it will become massive. [laugh] But inside the material, it's a many-body interaction that could give you an effective zero mass. That's what's special about the Dirac spectrum. Like a topological insulator, the surface states shows a Dirac spectrum. Graphene, before you do other things to it, the electronic low-energy excitations show the Dirac spectrum. That's what it means, a linear dispersion relation. If you write down the Hamiltonian in the quantum field theory language, then you have the Dirac equation. When you write down a Hamiltonian, that's how the Dirac equation comes in. [laugh]
ZIERLER: We've talked about graphene from a nanoscale perspective. Are you involved at all in graphene as a building material for things like bicycles, for example, or as an engineering material?
YEH: Graphene, because itself is so strong, it is mechanically like 200 times stronger than steel, so you can blend graphene flakes or graphene nanomaterials into, say, polymer, etc. Then that can really strengthen the material. I don't personally do that. But I have industrial people coming to me, interested in using graphene to strengthen polymer so that when you make plastic bottles, you can reduce the amount of plastics by blending in a little bit of the graphene nanomaterial. They're trying to do that, so they come to me. I'm helping them to look into that perspective because one aspect of sustainability is to reduce the use of plastics. The world's largest plastic bottle-making company would like to see if they can reduce the amount of plastic material that they use by incorporating graphene nanomaterials into it. That's one thing. But what I'm trying to say is the concept, because there are proven cases that when you incorporate graphene or carbon nanomaterials into bicycles or mechanical things, manufacturing things, they do strengthen materials.
ZIERLER: Nai-Chang, are there any difficulties or challenges toward the production, the large-scale production of graphene, either in terms of extracting the raw materials or in the production process itself, that might limit simply the availability of graphene for these applications?
YEH: If you want to just make graphene nanomaterials, then you can probably make a large quantity of it, and you can try to purify and all that. I see the biggest challenge right now is that if you want to make high-quality, large panels of graphene sheets, that can be extremely useful for many, many things, but that's hard because you have to probably think about doing roll-to-roll growth. Also, once you grow the graphene, you need to transfer the material to wherever the target substrate that you want, and that transferring process is also highly nontrivial. I would say that the biggest challenge is in the production of large sheets of graphene. That's the biggest challenge. If that part, the manufacturing, theoretically, I know how to do it. [laugh] To implement it is actually by no means trivial. If that can be done, many, many things can be used. You can really use graphene sheets to do desalination, actually, and you can use it to protect all kinds of material surfaces, if you have air-sensitive material surfaces. It can be used for many things, but the large sheets are hard to make. On the other hand, if people are primarily interested in nanoscale materials, then you blend graphene into other materials to form composites, and they can provide very strong mechanical support. But the biggest challenge, I think, is in the mass production of large sheets.
The Legacy of Millie Dresselhaus
ZIERLER: Nai-Chang, on the interpersonal side, when we lost Millie Dresselhaus in 2017, I know you had an opportunity to reflect on her legacy. What were some of the things that you wanted to emphasize, to tell people about what she accomplished, and specifically what you learned from her?
YEH: She was a person of great merit. [laugh] She was just such a generous person towards others. She was kind, always encouraging. She was kind. She was a tough lady, tough in that she held onto her principles, and she would not back down if she knew that she was doing the right thing. She would stand up for her students, her colleagues. She was always very supportive, very generous with her time. She actually took great care of students in many ways. But what was special about her was that she seemed to understand her students better than her students understand themselves. [laugh]
ZIERLER: [laugh]
YEH: I myself was an example. She seemed to know me long before I realized what I was like. [laugh] She would look at students, and she would have different approaches of mentoring students. She knew what kind of person I was before I fully understood [laugh] what I was like. I was always slightly rebellious, and I liked to do things on my own, in my own way. When I was her student, she gave me all kinds of freedom. She was just supportive. She gave me a general direction. Then I liked to figure things out, to find my own direction, detailed directions. I liked to design my own experiments, worked out my own theory, and then I just liked to do that. I didn't like to be told what to do. She would give me some guidance, general guidance, then I would figure things out. But some students needed more help, then she would really spend more time with those students. She looked at me, and she found me my first job as a postdoc because she said to me that I would work well—I think I mentioned this before—in superconductivity. Then she found me a job to do high-temperature superconductivity [laugh], although I never did anything on that for my PhD thesis. But she seemed to know what kind of person, what I was good at, and then she would nurture me along those directions, and help me out. She was incredibly wise [laugh], in that sense. She understood her students, and she really took care of her students in different ways. But she was just a great mentor and a very kind person, a very fine musician too. She played the violin and viola. She actually got into physics because of music.
ZIERLER: Really?
YEH: Yeah, because she was born into a pretty poor area in New York City. But because she was talented in music, she got a music scholarship, and she got a music scholarship so that she could get into, I think, City University in New York. She had a music scholarship so that she could get a good science education [laugh], and that was when she started working in physics. Her whole life, music was a critical part of her life. I like the fact that she was quite versatile, and so I always think that a pinnacle of scholarship should include virtuosity, versatility, and virtue. The three Vs, I call it, and she has all three. [laugh] That's how I would consider her as a very special person.
ZIERLER: Nai-Chang, in the way that you shared with me some formative advice that Millie gave you as a woman scientist, for your own women graduate students, just generationally, do you feel like things have improved? Do you need to pass on the same kinds of wisdom that Millie shared with you, or have we moved beyond that, to some degree?
YEH: I think things have improved a lot. I think women are treated much better now. But I think the advice Millie gave to me is still applicable to young people. It's very important you work on yourself, you establish yourself, you make sure that you have all of the strengths and merits that you can stand any scrutiny, high standard. You build the strengths in yourself, and that was what Millie emphasized. Once you have the strengths, then you can go and help others. I think that kind of advice is still universally applicable, not just to women, to young people.
ZIERLER: Yeah. Nai-Chang, back to the science, we talked about graphene and sustainability as it related to solar energy. What about some of the possibilities with lithium batteries, given their importance?
YEH: They can be used in lithium-ion batteries. People are using graphene materials as the anodes in lithium-ion batteries. There are other things we are considering to improve lithium-ion batteries by incorporating graphene. This is something I will be working on. It's not done yet. But graphene can be used as the anodes or the materials that can further improve the lithium-ion battery performance. For instance, let me just mention what I'm thinking about working on. One problem, although lithium-ion batteries are very powerful batteries for energy storage, they do have a very severe shortcoming, that is, lithium-ion batteries often would just ignite. [laugh] They would burn. That's why airlines have all these restrictions related to lithium-ion batteries. The reason is that in lithium-ion batteries, when you use them for some time, when you cycle them for some time, there are these dendrites that would form inside the lithium-ion batteries, and these dendrites then will short off electrodes, and then it could catch fire. There are many reasons why these dendrites could form. These are nanoscale dendrites. We are contemplating various causes for the formation of dendrites. For instance, on electrodes, if we use graphene to pacify the surface to make things smoother, then the roughness, there are some roughness spots on the electrodes that could be where these dendrites will form, will nucleate and develop. If we can mostly eliminate those—and then we are thinking about using graphene to achieve it—then we can reduce the risk of dendrite formation, for instance. People are already using graphene material for anodes in lithium-ion batteries already. It can be used for different purposes. But the point is that they can be incorporated into lithium-ion batteries.
ZIERLER: Nai-Chang, a technique you've worked on a lot in recent years is chemical vapor deposition. I wonder if you can explain what that is.
YEH: We use chemical vapor deposition and also plasma-enhanced chemical vapor deposition. Those are actually two different techniques. Chemical vapor deposition typically is that you heat up your chemicals, and then they will turn into vapor, and so they can decompose into different components in the vapor. Then you have a substrate on a different side of the furnace, and then you can control the temperature gradient or different distances between your chemical source and your substrate, and then you can eventually deposit materials with desirable chemical compositions on the substrate. You can have different chemical sources, and then, eventually, they can all condense onto the substrate, and grow into the material that you want. If you start with the right combinations of the chemicals, and you heat them up, they turn into chemical vapor. Then you deposit them onto a substrate, turn into the material that you want. That's chemical vapor deposition. It usually involves high temperatures. You heat things up, and turn them into chemical vapor. Then you'll play with different chemical compositions, temperature gradient, and all that, temperature of the substrate, different types of substrates, and you can grow the material. Plasma-enhanced chemical vapor deposition is different in that you have plasma. You create a plasma environment, and inside the plasma, you could have different gases that consist of the chemical components that you eventually want to grow onto your substrate. But the plasma environment is different from chemical vapor. It has a lot of radicals and energetic particles. The plasma-enhanced chemical vapor deposition is that you have this environment of lots of radicals, including all the components that you want, and then you have a substrate, and then these radicals can bombard the substrate, form nucleation sites, and develop into the materials that you want. But then you'll have to play with the plasma energy, the radical energy, the flow rate and pressure of gases. All those things will affect what you get. These are depositions of materials, but then it depends on your environment. It's just typical chemical vapor or energetic particles, including plasma and ion species.
ZIERLER: Nai-Chang, moving closer to the present, when the pandemic hit in the spring of 2020, and for a time you might not have had access to your laboratory, how did your science change during those difficult months and years?
YEH: It was very difficult because, as an experimentalist, when you cannot do experiments, that's just like a death sentence. We did have some data we already accumulated, and we had some papers we were working on, so there were things we could work on. But it was very difficult because, also, my students' graduation was all delayed. I actually graduated five PhDs during the pandemic time, but for each one of them, the delay in graduation was between three to six months. During that time, I still had to pay them, and they couldn't do much, and I didn't get any supplementary funding from funding agencies. I just had to swallow the whole thing. It was very difficult. [laugh] I can tell you this. Money-wise and research-wise, it was just very, very hard. But we tried our best to arrange it. There were three months, we couldn't do anything. We were just not allowed to do anything. We could only go to the lab to check for safety issues. Nothing could be done. But then after three months, we started opening up like 25% capacity, then after some time, 50% capacity. We tried to do our best to schedule students around the clock. At the time, we were not allowed to have two people together in a certain sized lab. It has to be social distancing requirements and all that. Also, a lot of shared facilities could be accessed. Kavli Nanoscience Institute was basically shut down for three months, and then partially open in phases for the following months. It was very, very, very hard because it had a huge impact on our funding because I had to pay people when they couldn't do things, and their graduation was delayed. I didn't get any extra money [laugh], so I just had to work things out. I pumped in my own salary into paying for the differences. That's [laugh] how it works.
ZIERLER: Nai-Chang, do you see opportunity, heaven forbid if this happens again, or the next pandemic, for more computer-based experimentation where you can actually run aspects of the laboratory remotely?
YEH: There are things we could run remotely to some degree, but many things you still have to get in and get things worked out. Then you can run them remotely. But a lot of very delicate things, I just don't see how we can just somehow do them remotely. Also, we have vacuum pumps and all these hardware. They have to be maintained, unless in the future we have all robots built, so that they go in and maintain things. But, otherwise, a lot of hardware, when we work on real hardware experiments, there are things software cannot do. You must get in, intervene, and work on them physically. That's the problem. Certain things, certainly, certain data, once we set up all of the hardware, then we can have computers taking data automatically. But, for instance, I'm doing a cryogenic experiment. I had to come in and transfer liquid helium, or transfer liquid nitrogen. Maybe in the future, you can have a robot or something to do it, but it would be very expensive for a university lab to build all those things and automate all of those things. It's just that we are not funded at that level. We are not in industry, and so for those tasks, you still need people to come in. When you don't do it, nothing happens. [laugh] Unfortunately, it's not so easy. In principle, a lot of things can be automated, but you need the funding to do it. We are not in industry to be given funding to automate things, physical things. It's a problem. I'm concerned.
ZIERLER: Nai-Chang, for you personally, because you enjoy, you're comfortable working in theory, not being able to go into the lab, were you able to do more theoretical work at all during the pandemic?
YEH: I can do a lot of thinking, etc. But a lot of things, you want to verify them experimentally. Otherwise, it's just a conjecture. We could do some simulations. We did do some simulations, etc., that will help us design our experiments better. But, in the end, I'm still an experimentalist, so things have to be verified empirically. Otherwise, it's just meaningless. I was doing a lot of reading, thinking, and simulation with students. Actually, also, when you try to concentrate on theoretical work, you need to be able to concentrate. When I was dealing with an infinite amount of awful things, like approval of arranging the lab arrangements so that people could get in at a certain time, we were under a lot of strict guidelines, just the sheer amount of paperwork, and all of the activities turned online. An infinite number of meetings and things like that is very distracting. I did think about some interesting new ideas, but I didn't really do theory during that time because I felt my time was chopped up into pieces, and I was dealing with a lot of distractions that were not productive [laugh], unfortunately.
Equal Opportunity in Science as National Policy
ZIERLER: Nai-Chang, on the policy side, tell me a little bit about your work with NSF on the Committee on Equal Opportunities in Science and Engineering.
YEH: Actually, the two-hour meeting I had before our meeting [laugh] was that.
ZIERLER: Was that?
YEH: Yeah.
ZIERLER: Oh, so your term hasn't ended yet?
YEH: No. I was extended for one more year just because we are in the middle of writing. Every two years, we have to write a biannual report, and so we are in the middle of writing the report, and so they wanted me to finish the report. [laugh] This is the only congressionally mandated committee at the National Science Foundation. I think this is the third report—maybe the third or the fourth report [laugh] I wrote. I think I was at the end. I joined in towards the end of a report that was finishing up. Then every two years, I participated in writing a report. I have served for six years, so I think this is my fourth report and [laugh] final report. I think it's a very interesting experience because that gives me a bird's-eye view of a lot of issues, a lot of issues facing the country, a lot of issues that still have not been improved sufficiently over many decades. But then the positive side is that when we saw things, we could make recommendations that actually impacted NSF funding and policy. That's something I was happy about. But before I served on the committee, of course, I was always pro-equality, etc., but I didn't realize that for a lot of these underprivileged groups the kind of severe environment and problems they had to deal with. I didn't understand the degree of problems. I was like, yeah, I'm always for equality and inclusiveness. I feel that I'm a freethinker and I'm very open-minded and I'm pro those things. But when I actually served on the committee, and had better understanding of all of the issues that these groups had to face throughout history, it was an eye-opening experience, and it made me feel that I'm even more pro-inclusiveness, diversity, and really helping people to give them opportunities so that they can fully develop their strengths, talents, to contribute to society, to the country. I think, really, people need to be given equal footing.
We are not talking about giving people an unnecessary advantage over others. We are talking about bringing people to an equal footing. A lot of people, they never had that opportunity for the environment they were born into. I think it's very important to do that. Also, there are talents in all people, all kinds of different people. Right now, we are looking at the US global competitiveness, so why not also grow a lot of talents from our own? We have all these different people. Also, diversity often brings in creativity too. I would say that it's an interesting, eye-opening experience for me. I actually have a deeper understanding of different things I was never aware of. We are not just talking about minorities. We're talking about very general things. There are people who are socioeconomically disadvantaged, and there are different groups. We are not just talking about minorities. We are talking about underprivileged people in general, and you want to empower them. You want to give them opportunities through education, through research, and just bring people out of poverty, and realize their full strengths. I think that's just the right thing to do as a human being.
ZIERLER: Nai-Chang, now that we've worked right up to the present with your current work, a Zoom that you were on just today, for the last part of our talk, I'd like to ask, if I may, a few broadly retrospective questions about your career, and then we'll end looking to the future. The theme I'd like to develop for this last part of our discussion is the fact that your research agenda is so broad, that you work on so many different things. If I can ask you to reflect on your career and all the things you've been involved in, in what areas do you feel the most advances have been made, and in what areas do things feel almost as fundamental as even perhaps when you were in graduate school?
YEH: You mean what I have accomplished, or you're saying what has been progressed the most?
ZIERLER: Both fields where you've seen the most progress, and fields where you've specifically contributed to that progress versus fields where, despite efforts that you've been involved in and others, there hasn't been as much progress compared to other areas.
YEH: Let me put it this way. For instance, in the area of strongly correlated materials, including high-temperature superconductivity, I think there have been a lot of advances, and I know that I have made some important contributions there. In terms of these van der Waals materials, low-dimensional materials, I also think there are a lot of wonderful progress made in both fundamental side and technology. I think I also contribute to different aspects of that kind of research area. I have also worked on something in the past that was based on using superconducting technology for precision clocks at the time. That area, I think we probably touched upon that a little bit. That area, unfortunately, it was basically taken over by optical resonators.
Optical resonators have all kinds of advantages over superconducting resonators for precision clocks. If you want to use superconducting resonators for certain other types of application, you can still use them. But as precision clocks, then the superconducting system, once optical system advanced, they were basically thrown out. I worked in that area, and it got thrown out [laugh] there. That's just because of the nature of a different frequency range, and optical resonators have far more advantages in that area. I would say that superconducting microwave resonators would be the area. That's just because, it turns out, it's just not the right system for that purpose when another better system comes through. But, otherwise, I think a lot of things that I'm working on—we also talk about topological insulators, etc. Topological systems, that's another area that has a lot of advances in terms of both theoretically and experimentally, and in terms of the materials. But what I am trying to do right now—so this is like an ongoing thing, ongoing exciting thing I'm trying to do as what I told you. I try to design my own topological systems beyond what you can find in nature, and basically artificially made topological systems in the electronics and also in photonics, because they're very, very interesting scientifically, but they also have great potential for applications. If I think about what I have done over the decades, I would say that most areas I worked in, I have seen very good progress over the years.
ZIERLER: Nai-Chang, because of your unique approach, you're equally comfortable in theory as you are in experimentation where, generally in physics, the trend has been scientists identify as one or the other. What do you see as the greatest advantage? What have you been involved in where, in serving as your own theory in thinking through the theoretical implications yourself, where has that proved most useful in pushing the science forward?
YEH: It's tremendously useful because I don't have to be told by anybody what to do. I figured it out. I figured out what I want to do. Then when I actually do things, and I get data, I feel much happier to have my first take at the data, and to understand, try to figure out what my data are telling me, instead of bringing my data to theorists, and saying, "Sir, could I ask for help? What am I seeing? I have no idea." I really enjoy the fact of looking at my own data and figuring things out. That part is why I work so hard on the experiments. [laugh] If I have wonderful experimental data, but I have no idea what they mean, and always have to ask other people for help, then that's not very satisfying. To me, just figuring out things from my own data, and learning from my own data, is the most fun part of the research. Then, of course, sometimes things get really hard, and then interacting with people, of course, is great, talking to top theorists, and then get different perspectives, and then I get a better and better understanding. That's also fine. But I do want to have my first stab at the data to see what my data are telling me. [laugh] I want to design my own experiments, and I want to understand my own data. That's why I do research. [laugh]
The Moment of Understanding
ZIERLER: Nai-Chang, of all the discoveries that you've made, all of the advances that you've contributed to in basic research, what has been most satisfying to you, either because of the magnitude of the discovery, or simply the enjoyment of seeing all of this hard work really yield an important result?
YEH: The most enjoyable part is really this aha moment of understanding something. To me, the moment when you figure something out, that moment when you truly understand something, to me, that's the key thing that drives me to do research, because life is short, and we are just nothing in the universe. But, with that moment, when you truly understand something, to me, it's like I feel that I resonate with the universe and that kind of like eternity [laugh], and I feel that sense. That's the most satisfying part. Just whenever I truly understand something, and especially if it's something that I discovered, I worked hard on, that's the most wonderful part of why I do research. [laugh]
ZIERLER: Nai-Chang, in thinking through your motivations, and working so hard to increase diversity and inclusivity, what aspects are motivated by the idea that science owes it to underrepresented voices, that science should be a way of increasing opportunity? In what ways is it because science needs different perspectives, different backgrounds, different outlooks, because it's only that multiplicity of perspectives that allows science to move forward?
YEH: It's both. The beauty and the benefits of science really should be shared by all people. Then you can use science to inspire talents, and then they can do even better science or work out technology to benefit the world. It's a mutual thing. Also, as you know, throughout history, if you look at the best civilization, the most brilliant civilization, those all involved a period of time of diversity. I can say for Chinese history, the best dynasties during their prime times when they were most inclusive, most open. That was when the culture, that civilization blossomed during that period. We learn that from history. We know diversity, inclusiveness can bring about prosperity also. But then, at the same time, I just think as a human being, we should work together to make the world better, and so we should not have groups that are just so underprivileged, so deprived of opportunities. It's just not right. To me, it's just not right. I feel strongly about this importance of having diversity, inclusiveness, but also, at the same time, I also feel that as human beings, we should work together to make as many people as possible realize their full potential, and to make the world better. Did I answer your question? [laugh]
ZIERLER: Absolutely. It's both. You're emphasizing that it goes both ways.
YEH: Yes.
ZIERLER: Nai-Chang, a troublesome current events question, of course, is the specter of a Chinese attack on Taiwan. I'm curious what your personal concerns are about this, and what role you might see for scientists to encourage peace and understanding.
YEH: I do have grave concerns. The problem is that we are dealing with a regime that's not rational. We are dealing with a system that does not take reasons, does not take logic, analysis. When you have that kind of system, you cannot reason with them. It's all about power and control; about big ego. It's nothing rational. [laugh] As a scientist, actually, we talk about logic, evidences, analysis, etc. None of those things apply, unfortunately. But, coming back, how can we hopefully avoid war? Of course, Taiwan is being recognized as science and technologically so important for the world. It's really the technology that's making Taiwan visible right now. Otherwise, I bet most countries will not give a darn about [laugh] potential problems with Taiwan. But it's because of the semiconducting industry that's making Taiwan in the frontline of the world attention. Taiwan should keep that edge, technological edge. But, of course, we will try our best not to provoke China. We just have to be as wise as possible, diversifying the high-tech manufacturing locations [laugh], so that if anything happened, it won't be like, OK, China is not happy, and say, OK, let's just bomb Taiwan, and so the whole world goes down together. If they don't get it, the rest of the world should not get it. Then Taiwan will be just like Ukraine, being sacrificed. We certainly hope that would not happen, but Taiwan has to be wise in diversifying their capabilities, locations. That's one thing. Team up with people around the world but still express goodwill in general.
But this is a very complicated thing because we are not dealing with a rational regime. That's the problem. [laugh] It's like who would have thought that Russia would invade Ukraine? But this invasion of Ukraine, and the world reaction, does make Chinese government pause a little bit. They realize that the world would not necessarily tolerate such behavior. But, at the same time, there's this sanction against them, effectively, the CHIPS Act. Without chips, China can only manufacture lower-level chips, and so these high-tech chips are mostly in the hands of TSMC. More than 90% of the top-notch chips are in the hands of TSMC. US is not going to allow China to get to the technology. It's a tough situation. I cannot predict what would happen down the line. It could be very dangerous. It could be very dangerous. But what can we do? You are asking me what scientists could do. Science is without borders. But, right now, we're even having a hard time having free communications with China now. We have to watch out. We have to be very careful. We don't want to run into trouble with the US government. We also don't want to run into trouble for our Chinese friends with their government. It's very tough right now. I don't know. I actually don't know what would happen in the coming year or two because, right now, the situation is very bad. The open communications, except for if you're doing truly, truly fundamental science, you can still communicate. But anything started becoming sensitive in technology, you have to be very careful. I don't know. I'm sorry I cannot provide a good answer. It's something I'm truly worried about.
My whole life, I have been trying to do things out of good will. I always believe in sciences without borders. I have been serving on all kinds of things all over the world for all different countries. I've done a lot for Taiwanese government, for China also, and for other places, like Japan, Germany, Hong Kong, Singapore, Turkey, and Korea, and all these countries. I do services because science is without borders. That's what I believe but not entirely [laugh] because when you start running into issues of national security, then you have to start worrying about things. It's a tricky situation. In general, science promotes, common aspiration, common goals. We are human beings. We are curious. We want to pursue the truth. There are a lot of things better done together, and then a lot of the issues facing humankind right now, global warming, climate change, all of these things really require people to work together. But there are a lot of geopolitical complications that are beyond what we scientists can do. [laugh]
ZIERLER: Nai-Chang, let's end on a happy note. We'll return to the science. Because your research agenda is so varied, how far can you plan out in terms of what you expect to be working on? In other words, can I ask what you'll think you'll be working on in a year, 5 years, 10 years? How far out can you plan, and how do you build in for the element of surprise as that happens along the way?
YEH: I can predict about what I want to do in a year or three years, maybe, because I'm seeing exciting things, and I'm trying to plan experiments, working towards them. But, usually, then you do something new, and then you learn new things. Then you generate new ideas, and [laugh] then that's the part that's not entirely predictable. I do research almost like a child going into treasure hunting, going into Treasure Island—
ZIERLER: [laugh]
YEH: —and then look for things, and say, "Oh, wow, oh, wow," [laugh] and being surprised constantly. That's pretty much my attitude towards doing research. [laugh] I don't like to be dictated on what I want to do, because you never know. That's what I learned time and again. I always get pleasantly surprised here and there. Then I figure out new directions to go. I can say within one to three years, I can write a one- to three- or maybe up to five-year proposal, because I do have very exciting things that may take up to three to five years to realize, to complete. But beyond that, I would just leave it to make sure I can always be pleasantly surprised.
ZIERLER: That's what keeps it interesting?
YEH: That's right. I don't want to predict, mandate something, because then it's no fun. [laugh] When it's too predictable, it's no fun. [laugh]
ZIERLER: [laugh]
YEH: I want this unpredictable element of surprise. [laugh]
ZIERLER: Nai-Chang, it's been a great pleasure spending this time with you. I want to thank you for sharing with me all of your insight and perspective. This has been a wonderful experience, and a treasure for history. Thank you.
YEH: Thank you, and thank you for giving me this opportunity. It's great to know you too.
[END]
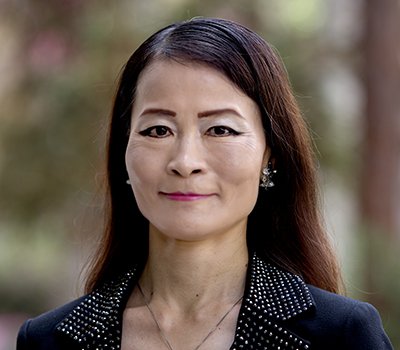
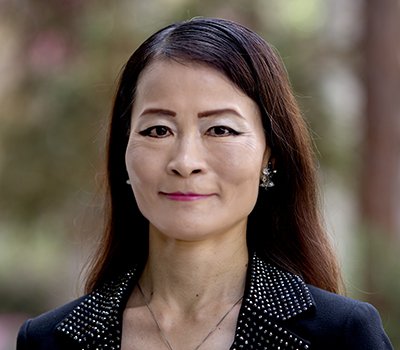
Interview Highlights
- The Matter of Quantum Information
- Nanoscience as Connecting Point
- Crossing the Boundaries of Theory and Experiment
- Trend Toward Applications
- State of the Art in Superconductivity
- Formative Advice to Achieve in Science
- Early Interests in Physics
- From Taipei to Boston
- Learning From the Carbon Queen
- The Wonders of Graphene
- Superconductivity at the Woodstock Conference and IBM
- Recognizing Condensed Matter as Real Physics at Caltech
- The Culture of Support for Junior Faculty
- Vortex Dynamics and Novel Materials
- Demonstrating Universality
- The Impact of Tunneling Spectroscopy
- Physics in Pursuit of Truth and Beauty
- Lattice Distortion and Return to Graphene
- Metrology and NASA
- Scientific Advances in Taiwan
- Quantum Computers Need Nanotechnology Breakthroughs
- Delegating Scientific Applications
- The Legacy of Millie Dresselhaus
- Equal Opportunity in Science as National Policy
- The Moment of Understanding