Peter Armentrout (PhD '80), Thermochemist, Mass Spectroscopist, and Research Leader in Transition Metals
As an undergraduate at Case Western Reserve University, Peter Armentrout became interested in ion-molecule chemistry, and a meeting with Jack Beauchamp, who struck Armentrout as both a great person and a great scientist, made Caltech an easy choice for graduate school. For his graduate research, Armentrout focused on the thermodynamics of uranium chemistry, which required him to build an ion beam apparatus. Between the instrument building and the fact that this was brand-new research in chemistry, Armentrout's time at Caltech was exhilarating. As a postdoctoral scholar at Bell Labs, Armentrout was fortunate to continue his work at one of the world's premier institutions dedicated to basic research. Working with Robert Fruend, Armentrout investigated the role of electrons in the ionization of metastable atoms and molecules. At the metastable timescale, the atoms and molecules are sufficiently stable to be studied but do not remain in a given state permanently.
After Bell Labs, Armentrout began his professorial career at UC Berkeley, where he focused on transition metals, identified as such because they are the transitional elements between and below the alkaline and the alkaline earth elements on the left hand of the periodic table, and the main group elements carbon, nitrogen on the right of the periodic table. In his move to the University of Utah, Armentrout continued in transition metal research and branched out to a broad range of research pursuits, both fundamental and applied in nature, which include the gas phase of solvated ions, organometallic chemistry, environmental and battery chemistry, and the computational application of first principles to provide theoretical guidance to experimental results.
Armentrout's many honors and recognitions include an NSF Presidential Young Investigator Award, an Alfred P. Sloan Research Fellowship, the Buck-Whitney Award from the American Chemical Society Eastern New York Section, the Biemann Medal from the American Society of Mass Spectrometry, and the Governor's Medal for Science and Technology Award from the State of Utah in 2010. In 2018, Armentrout was named the Henry Eyring Presidential Endowed Chair of Chemistry, an honor which connects Armentrout's record of scientific excellence with a leading member of Utah's Department of Chemistry from its foundational years.
In the discussion below, readers will benefit from Armentrout's precise and accessible explanations of the science, the choices he has made on which problems are worth pursuing, and his reflections on the importance of mentorship and teaching - with Armentrout on both the receiving and giving end. And an important theme emerges throughout: a key ingredient for scientific success is persistence, albeit in the context of the laboratory or among one's peers. And when buttressed by genuine intellectual curiosity and an intuition to separate the signal from the noise, the results can be both powerful and rewarding.
Interview Transcript
DAVID ZIERLER: This is David Zierler, Director of the Caltech Heritage Project. It's Tuesday, March 26th, 2024. It's my great pleasure to be here with Professor Peter Armentrout. Peter, it's wonderful to be with you. Thank you for joining me today.
PETER ARMENTROUT: It's a pleasure to join you, yes, indeed.
ZIERLER: Peter, to start, would you please tell me your title and institutional affiliation?
ARMENTROUT: I'm presently at the University of Utah. I'm a Distinguished Professor and the Henry Eyring Presidential Endowed Chair in Chemistry.
ZIERLER: Now, the name, the honor of the named chair, what does that signify?
ARMENTROUT: Henry Eyring was a towering figure in chemistry. He should've won the Nobel Prize, never did, but he basically invented transition state theory, which in some form or another is still used by physical scientists and chemists today.
ZIERLER: Peter, tell me a little bit about the interplay of theory and experiment in your research.
ARMENTROUT: I'm fundamentally an experimentalist, but we have endeavored to incorporate synergistic theory in virtually everything that we do. I'm not a theoretician, which means that I don't develop new theories, but we're avid and fairly proficient users of the theoretical packages that have been developed by others.
ZIERLER: Does that include computation? Have you become increasingly reliant on computational models?
ARMENTROUT: "Reliant" isn't the right word. We do use computational models a lot. Back when I started, we didn't because they weren't available. It's more convenient to use them and probably more accurate and, because of the power that those computations have now, it makes the insight, I think, that much sharper. But I wouldn't say we're reliant on them beyond that those comparisons then end up being fairly insightful.
ZIERLER: Are you working in data sets that are large enough where machine learning and artificial intelligence is becoming increasingly likely as a research tool?
ARMENTROUT: We haven't gone down that road yet. I have been approached by others about the possibility of doing something along those lines. Our data sets, for the most part, are not so large that they really require that kind of approach at this point.
ZIERLER: Peter, are there any aspects of your research that we might consider translational, or is this exclusively a basic science operation that you run?
ARMENTROUT: It is largely a basic science operation, but there are places where I think in the not too distant future, one can anticipate that some of the things that we do will have direct applications. There's at least one project for the Air Force that potentially could turn around and be utilized almost immediately.
Where Energy Meets Metals in Chemistry
ZIERLER: Peter, let's go now into some more specific questions. Of course, thermochemistry is central to your research. I wonder if you could define thermochemistry and why it's so interesting to you.
ARMENTROUT: Thermochemistry is what we do and what I'm known for. Thermochemistry is measuring how much energy it takes to do a chemical reaction. It tells you, for instance, how much energy you get when you digest food, and turn it into energy for your body. It tells you how much energy you get when you burn a gallon of gasoline. It tells you how much energy it takes when you burn a candle.
ZIERLER: As this relates to metallic chemistry, I wonder if you can explain that.
ARMENTROUT: The kind of chemistry that involves metals is that most people have probably been exposed to is, for instance, the catalytic converter on their car. There you have a fairly expensive metal—which is why the catalytic converters get stolen now and again—that catalyzes a particular process that removes the toxic species from the car exhaust. Those metals are able to do that, whereas other kinds of chemicals are not. There's increasingly a reliance on using metal-based systems to catalyze interesting chemical reactions that are industrially important.
ZIERLER: Mentioning catalytic converters, what do you see as the impact of your research on environmental science?
ARMENTROUT: One of the things that we have looked at there is understanding, for instance, how metals interact then with water in particular, so aquifers. If you're interested in removing metals from an aquifer that are dangerous then some of the information we supply would be potentially useful for at least understanding what that process is. By the same token, anytime you're delivering trace metals into your body, they usually come in in aqueous media surrounded by water molecules. But when they get to where you want them, then all the water molecules have to go away. Again, understanding the thermodynamics of that process and the replacement of what's solvating the metal is potentially then useful in understanding that process and potentially controlling it.
ZIERLER: Peter, there's so much interesting research happening at the interface of chemistry and biology. What are some of your contributions in that regard?
ARMENTROUT: We're presently looking at the thermochemistry associated with small biological molecules, so typically just small peptides which are chunks of proteins, and understanding how they interact with protons or with abundant metal salts like sodium or potassium. We've looked at various processes then that do have implications for aging and for the onset of neurodegenerative diseases.
ZIERLER: Peter, tell me about your work studying nonadiabatic effects.
ARMENTROUT: Nonadiabatic effects are interesting. What we mean by that is that adiabatic effects are usually how most chemistry occurs, that you follow the lowest energy pathway. As a consequence, if you understand what the thermochemistry of your reactants and products are, then you understand a lot about what that chemical process is. Nonadiabatic effects then are when something a little bit different happens, and the system goes off in a direction that is a little bit unexpected. Partly because we look at the energy dependence of chemical reactions over a much wider range than has typically been done in the past, we see on a regular basis what I would call nonadiabatic effects. I wouldn't say that we understand it all that well, and that's in part because they haven't been looked at in great detail under many circumstances.
ZIERLER: Peter, I want to learn about your research group. Let's start with the graduate students and postdocs. What kinds of careers do they go onto when they leave your group? What's the rough proportion who go on into academic positions, and to industry, or governments and National Labs?
ARMENTROUT: I'd say about a quarter of my students have gone out to academics, which is either R1 institutions but also a number of teaching colleges and universities. I'd say about a quarter have gone into positions at National Laboratories and various scientific endeavors like that. At least another quarter are still gainfully employed in industry, doing chemical types of projects. A quarter have probably gone into industry and have moved on to other kinds of things. I have a couple of real estate agents, and they seem to be doing just fine too.
ZIERLER: Peter, for you, what are the institutional connections or collaborations beyond the University of Utah that are most important, both in industry and in academia?
ARMENTROUT: For me personally, right now I have an active collaboration at Radboud University in the Netherlands. For about the past 15 years, I've been traveling there to work at a free electron laser facility, where we take infrared spectra of some of the molecules that we're interested in. I have two collaborators there working on fairly distinct projects. I take students with me. We usually go—except for COVID, where we had a bit of a lapse—we usually go twice a year for a couple of weeks, and collect an update to keep as busy for the rest of the year. That's been very valuable. Then I've developed a couple of collaborations with other people, mainly in Europe, as it turns out. Partly, I think that's because chemical physics is mainly done in physics departments in Europe, and it's mainly done in chemistry departments in the United States. The overlap that I have very often is with physics departments in Europe, which has worked out well.
Bespoke Instrumentation in the Lab
ZIERLER: I'd like to engage you in some questions about instrumentation and technology. As a preface to that, what have you learned about technological advances as a driver to discovery? How important is that versus having an idea and then developing a technology that's responsive to that idea?
ARMENTROUT: What we do, you cannot do well using commercial instrumentation. One of the things I learned at Caltech, working with Jack Beauchamp, was I built my first instrument that enabled us to look at some things that at the time he was interested in, and then as time went on, I became fascinated by. At that time, there was also a paper that was published that had new technology that used radio frequency electric fields in a very profitable way. I told myself that if I ever had the chance to do so, I would try to implement that in any instrument that I built. We've got two of these now. They're called guided ion beam tandem mass spectrometers. They're still fairly unique. There are a couple of other people that have them in the world, but there aren't that many. As I say, it's the gold standard then if you want to look at the kinetic energy dependence of ion-molecule reactions and, as a consequence, use that to measure thermodynamic information. That has turned out to be really a gold mine of information, but also then it's turned into what most people would appreciate is the gold standard for getting some of the information that we get.
ZIERLER: Now, the idea that you simply can't use products off of the shelf, is that because industry is not looking at the kinds of things that are important to you? Are you operating at sensitivities that are simply not relevant in industry? What's the disconnect there?
ARMENTROUT: The disconnect is really that what most of the instruments that do the kind of thing that we do are designed for is analytical purposes as opposed to physical. People are interested in just determining what's there, and maybe approximately how much of it, and separating it from a mixture of things. But the aspect of digging deep into what those materials are, what their structures are, what the thermodynamics are is not something that everyone is interested in. Lots of people are interested in those things and would happily do it if they could buy an instrument that would do it. But there's still not enough of those people that it makes sense for somebody to put together an instrument designed expressly for that purpose.
The Centrality of Mass Spectrometry
ZIERLER: Let's now go on to the techniques itself. We could start obviously at the broadest level, mass spectrometry. Why is that so important for what you do?
ARMENTROUT: Mass spectrometry is the ability to weigh individual molecules, determine their mass, so you determine their mass-to-charge ratio. But, at least for small molecules, you can determine what the charge is pretty readily. That tells you pretty much what the molecule is. It acts as a very efficient way of determining what you've got, which is then why it's used extensively in analytical chemistry. That in and of itself, mass spectrometry then enables you to just really determine what you're looking at, and how much of it you've got, and our sensitivity then is fairly high.
ZIERLER: As a technique, of course, mass spectrometry has been around for quite a while. Are there still advances, are there still marginal gains to be made technologically with this technique?
ARMENTROUT: Absolutely. There was a new mass spectrometer that came out a decade or a decade and a half ago called an Orbitrap that has really extremely high mass resolution. But it's a much more compact instrument than its competitor, which is a Fourier transform ion cyclotron resonance mass spectrometer. That also has extremely high resolution but is a much more cumbersome apparatus because it involves a very high magnetic field. There's always something new around the block in terms of sensitivity, in terms of resolution, in terms of how quantitative you can be. Mass spectrometry, I think, is one of still the fastest growing analytical techniques. It's used by a tremendous number of physical chemists to look at molecules that they're interested in, even if they're not looking at ion chemistry like we do.
ZIERLER: Another spectroscopic technique that you use, of course, is laser spectroscopy. What is laser spectroscopy good for?
ARMENTROUT: What we do is measure infrared spectra. In the infrared region, that's where molecules vibrate. You can determine then the frequencies at which they vibrate. That tells you something about how strong the bonds are, how massive the particles that are vibrating are. That acts as a fingerprint for what the structure is. You can, even for isomers, distinguish isomers by looking at their infrared spectra.
ZIERLER: Of course, there are beams that you use as well, ion beams and molecular beams. I wonder if you can explain both.
ARMENTROUT: We don't use molecular beams so much anymore. We only use ion beams. But in both cases, that's what it is, it's a collimated line, if you will, of either the ion or the molecule. Those can be created in a couple of different ways. Ions, it's very easy to generate them. For that matter, all mass spectrometers have an ion beam in them because what you've done is created the ions at one place, and then you're accelerating them through a mass selection device, a magnetic field, electric field, or a combination thereof. That ion beam then is the thing that's transporting the ions, and then eventually it hits a detector. The ion beam just moves the ions through the apparatus. In our hands, we use it in a much more quantitative way, which is the guided iron beam aspect of this, so that we focus more on what happens in between the mass spectrometers and not what happens inside the mass spectrometers.
ZIERLER: Peter, with all of these techniques, are there specific safety considerations to consider for the lab?
ARMENTROUT: We use high voltages, and so you need to be careful that those are shielded properly. We use compressed gases, and those need to be handled in a safe manner so that you're not spraying stuff all over the place. Occasionally toxic chemicals, fortunately for us not too often and too many of those. Beyond that, our laboratory is a reasonably safe environment because we're not exposed to the chemicals. They're always inside of a vacuum chamber, and then the little bits that are left over are pumped somewhere else.
ZIERLER: In creating and maintaining all of these instruments, do you have engineers on staff? Is there an expectation that the postdocs and graduate students should really be good with their hands?
ARMENTROUT: It definitely helps to be good with your hands, so if you don't have at least one student or postdoc that can do that, you're probably in trouble. We do all our own maintenance. There are machine shops and some minor maintenance for electricity and things like that that we can call on. But for the most part, we do all of our own electrical work, plumbing, and the construction of these things.
ZIERLER: Now, for the lab as a whole, are you operating strictly in a classical or a Newtonian environment? Are there quantum mechanical aspects to your work?
ARMENTROUT: The computations that you mentioned earlier, the kinds of things where quantum mechanics then comes into play and, for that matter, understanding the chemistry and the nonadiabatic effects, much of that is a quantum problem. We have a heavy dose of classical mechanics going on, but the quantum mechanics is a good part of a lot of the chemistry that we look at, especially with the metals.
ZIERLER: Peter, if we could zoom out at the institutional level, the Department of Chemistry at the University of Utah, historically, it's such a strong department. Do you have a sense of how it became such a source of excellence?
ARMENTROUT: That dates us back to Henry Eyring again, which is of course why the name sticks. Henry was a very influential figure in chemistry, and he was at Princeton. He was recruited out here partly because he was Mormon. He became the first dean of the graduate school at the University of Utah. But a lot of his work on transition state theory was done here as well. He started the graduate school here, was a leading figure then in science, and basically built the chemistry department up from certainly vestigial beginnings to what it is. He was certainly a large part of what it is today.
ZIERLER: As a recruitment tool, being situated in such a beautiful part of the world, is that really an asset to get top-rate scholars to join the department?
ARMENTROUT: We find that when we are able to get graduate students to visit here, and faculty for that matter, that, yes, our prospects for recruitment go up by quite a bit.
ZIERLER: For you personally, do you take advantage of the great outdoors?
ARMENTROUT: I grew up in Ohio. I've lived on both the East and the West Coast. I love it here, partly because, for instance, less than half a mile from here I can get on mountain trails and go for a run. I can do that within a mile of where I work. I get out almost every day I can.
Chemistry at Case Western
ZIERLER: Peter, let's go back now, and establish some personal history. Were you always focused on chemistry? Was that your interest as an undergraduate at Case Western Reserve?
ARMENTROUT: I was interested in chemistry fairly early on. I was interested in science for a long period of time. I had the classic chemistry kit at home that I never followed any of the directions for. I had very good chemistry and physics teachers then in high school, and so they certainly didn't dissuade me. I was certainly on the cusp of going either way. When I started as an undergraduate at Case Western Reserve, I was a double major in math and chemistry.
ZIERLER: Now, growing up in Ohio, did it just make a lot of sense that there was an excellent school right in your backyard?
ARMENTROUT: I certainly thought a little bit about where I wanted to go to school. But Case had a very good reputation in the technical areas, and so that eventually is where I decided to go.
ZIERLER: Were there real research opportunities as an undergraduate for you at Case? Did you get to work in labs?
ARMENTROUT: When I was a junior, I joined an inorganic laboratory, where I was set to work washing glassware. I found it fairly mind-numbing, frankly. The next year, I had the opportunity, and met with Professor Rob Dunbar. He let me start doing some research, and that was my entrée into ion chemistry, so that's where I got started with that. He also introduced me, when I was a senior, to the person that would eventually be my PhD advisor, Jack Beauchamp from Caltech. Rob made a point of having us meet. It turned out they were graduate students together at Stanford, and so I got to meet Jack for the first time then. I was drawn to that field then for quite some time.
ZIERLER: Peter, on the social side, being an undergraduate at the tail end of the Vietnam War, was Case Western Reserve a political kind of campus? Were there protests and things like that?
ARMENTROUT: Not to the level that it was at a lot of other places. But it was certainly the case that I remember vividly watching the draft on TV, wondering where my number would come up.
ZIERLER: But, of course, that ended halfway through. That wasn't a concern for you when you graduated?
ARMENTROUT: That wasn't a concern when I graduated, but I did go through the draft at least twice while I was in college.
ZIERLER: Tell me about meeting Jack Beauchamp. What was that like?
ARMENTROUT: Jack's a really affable guy. I remember in particular we were coming back from lunch, and he and I were talking. He was asking me questions to get a feel for whether I was worth spending any time on, I think. I remember thinking, you know, being challenged by the questions that he was asking. But apparently I did OK.
ZIERLER: Did you consider anywhere else besides Caltech for graduate school, or meeting Jack and having that connection was all you needed?
ARMENTROUT: No. I considered other places. I ended up applying to six different schools, all really good ones. I ended up visiting Berkeley, Stanford, and Caltech. But I certainly had the best personal connection at Caltech. The other places, I hadn't known anybody. Jack went out of his way then during my visit to make me feel comfortable. He took me, a good old Midwest boy, to a sushi restaurant, where I had raw fish for the first time.
ZIERLER: [laugh]
ARMENTROUT: He thought that was amusing.
ZIERLER: What were your impressions of Pasadena when you first arrived?
ARMENTROUT: Because Caltech is where it is, and it's this closed off, I didn't get much of a sense for everything else around it at the time. Caltech is just this community unto itself. After a while, you learn that there are the gardens in Pasadena, and lots of other things going on. But at the time, I don't remember. I was thinking, wow, it's a nice community, and I can see the mountains.
ZIERLER: What was the Beauchamp lab focused on when you joined?
ARMENTROUT: The exciting thing going on in the Beauchamp lab was infrared spectroscopy, which I was not a part of. I had this other project that was being funded by the Department of Energy to look at uranium chemistry, and so that's where we built up this ion beam apparatus. That was all done from scratch. Jack wasn't devoting a lot of money into this particular project, so we scrounged a magnet from another laboratory on campus. I figured out how to mate it to the little chamber that we had that had a little quadrupole mass filter on it. I developed a new novel source then for generating a fairly clean source of uranium cations.
ZIERLER: Was the uranium research, did it have a national security component to it?
ARMENTROUT: We did not need a clearance or anything like that. It certainly was related, at least in those days, it was related to understanding the chemistry for isotope separation.
ZIERLER: Was it your interest? You said that this was not a major focus of the Beauchamp lab. Was this your interest to get involved in this?
ARMENTROUT: No, not really. I initially worked on the ICR. Most people in the Beauchamp lab did, I mean, that's what Jack was known for, was ion cyclotron resonance mass spectrometry. I did a couple of projects on that, to get my feet wet. Jack was interested in having somebody follow up and revise this other apparatus to do different things than what it was capable of doing when it was first built.
Uranium and Isotope Separation at Caltech
ZIERLER: What was the state of the art in terms of thermodynamic states as it related to this uranium research?
ARMENTROUT: Back then, there really wasn't a lot of thermodynamic information, certainly in the gas phase on small molecules that had any actinides in them. Virtually anything that we did in those early days was brand new information.
ZIERLER: What explains that? What was the hole in the collective knowledge or the technological limitations?
ARMENTROUT: Most thermodynamics, I mean, most thermodynamic information that's available for things is obtained by burning stuff. You just take a molecule, and you burn it, and you see how much energy is released, and you determine what the products are. All of organic chemistry, that's fundamentally what's done. As you start doing this with metals, you end up with a lot more difficulties. Also, those aren't very bond-specific, so you're measuring all the bonds simultaneously. This was what was different about our approach. It's not like people hadn't looked at the chemistry, but there hadn't been good techniques to look at the thermodynamics of those species.
ZIERLER: Was this not something that would've been considered during, for example, the Manhattan Project?
ARMENTROUT: The Manhattan Project, having just watched Oppenheimer a couple of weeks ago, that was really focused on one thing. Understanding all the underlying chemistry and what might happen once you released a bunch of uranium into the atmosphere, that wasn't information that was needed then.
ZIERLER: Did you ever have any direct interface with the DOE? Did you get a sense of why they were interested in this research?
ARMENTROUT: It was isotope separation, so that was the main focus back then. These days, because we still have DOE funding, we're still looking at uranium and thorium chemistry in my laboratory. The focus now is really on maintaining the expertise that this country has in actinide chemistry at some level, and expanding that to understand what might go on with transuranic elements. At the time, 30–40 years ago, uranium and plutonium were probably all that you really needed to worry about.
ZIERLER: What was so important about isotope separation at that time, and specifically for the DOE?
ARMENTROUT: If you want to build a bomb, you need uranium-235, and that's a minor isotope of uranium, so it's mainly uranium-238. You need enriched uranium, and that means you've got to separate the isotopes—these things have virtually identical chemical properties because all they vary by is how many neutrons are in the nucleus. The chemistry is the same, so you needed to come up with techniques that allowed you to separate them, one isotope, 235, from the main isotope, 238. Mass spectrometry is one way to do that. It's not a very efficient way of doing it. But understanding the chemistry is potentially a way of applying that in other areas where you could then do more efficient isotope separation.
ZIERLER: Was there also a civilian nuclear energy component to this?
ARMENTROUT: There must have been. I wasn't interacting with that directly. But the DOE evolved out of the Nuclear Regulatory Agency. I may be forgetting exactly what the name was. DOE started to exist about the time I was a graduate student.
ZIERLER: I'm thinking of course of Three Mile Island. Did that register with you at the time?
ARMENTROUT: Sure. Anytime you have a nuclear accident, that draws a little bit of attention. One of the other reasons that these days I think you do want to understand the chemistry of actinides better is that if you have a nuclear accident, you're exposing these materials to the atmosphere. Reactions can occur, and they can occur under extreme conditions. It's not like it's all going to be done at room temperature if you have a nuclear explosion. That kind of chemistry then is potentially valuable. Understanding that chemistry is potentially valuable for understanding nuclear cleanup, nuclear waste, nuclear separations, and building safer nuclear plants.
ZIERLER: I wonder if you could talk about working with the uranium ions. Where did you get the samples from, and how did you store them safely?
ARMENTROUT: At the time, Jack assured me that it was safe. We were working with depleted uranium, which helps. That means that it's already undergone this process where you've removed a lot of the uranium-235, which is the most radioactive part of uranium. Uranium-238 is radioactive, but it has a half-life of something like 10 to the 9th years. I do not know where Jack got the sample. We had this chunk of stuff. We had a couple of uranium salts as well. Jack assured me that it was no more radioactive than the potassium in the paint in the walls—and that fundamentally is true. These days, we do keep it under lock and key so that nobody else can get to it though.
ZIERLER: If you could walk me through the experiment, what did the apparatus look like? How did you work with it?
ARMENTROUT: The apparatus at that time is, I mean, what these devices are is you have a place where you can generate the ions. I developed a source that used a process known as surface ionization. All that is, is you take a filament, not unlike the one in a light bulb, and the reason it glows is that it's really hot. We did that with a rhenium filament. If you then expose that to a sample that has some uranium in it, everything falls apart, and the uranium will boil off. There's a probability that it'll boil off as an ion. There's a finite probability that you can expose this hot filament to a uranium sample, and you'll get uranium ions coming off of it, and they'll be atomic ions. You don't even have to do any separation at that point. But once you get that, you'd have a couple of electric lenses that take the ions, accelerate them. I added the first mass spectrometer, which is a magnetic sector instrument, so that just you put the ions in a magnetic field, and they undergo a circular motion. Depending on how much energy they have and how strong the field is, you can pick out one species, one mass-to-charge ratio that it'll focus through the rest of the apparatus. That comes out of the magnetic field, and then you focus that into a little region where you have a neutral reactant. The ions, the uranium ions, and the neutral react in this little chamber, chemistry occurs, and then you draw the ions out, and shove them into a second mass spectrometer, which was a quadrupole mass filter. That uses radio frequency and electric fields to separate mass-to-charge ratios. Finally, you have a detector. You slam the ions using a high electric field into a surface that creates electrons. You use an electron multiplier then to make sure that you can see that.
Forefront Research in Thermodynamic States
ZIERLER: Peter, going back to the relative dearth of literature on thermodynamic states at the time, given that lack of knowledge, what kind of theoretical or intellectual bounds did you put on the experiment to know if it was working or to provide guidance on what reliable data would've looked like?
ARMENTROUT: There are a couple of benchmarks that we could look at, at that point. For instance, what we measure is a reaction probability for this particular reaction. We do that as a function of how much energy the ions have. One of the things that happens for a really simple reaction is the following. Typically, what we wanted to look at, because we wanted to measure thermochemistry, is that we would look at endothermic reactions; reactions that you need to supply energy in order for them to occur. Then by measuring the onset, the energy onset for that reaction, that would be the difference between the bond you were making and the bond that you were breaking. By measuring that difference, if we knew what the bond we were breaking was, and that was typically a stable, neutral molecule that the thermochemistry would be known for, then we could measure now uranium bound to a nitrogen atom, or uranium bound to a hydrogen atom, or uranium bound to a sulfur or whatever. But the other thing that happens at higher energies is that you form this molecule, and then it falls apart. Where it starts to fall apart is the thermochemistry of the neutral that you started with, so you know that, and you know where it should fall apart.
Sure enough, if we looked, we would find that this cross-section would peak right where it was supposed to peak. Beyond that, nobody had looked at any of these things before, so we had no idea fundamentally what it should look like. We didn't know where this onset would be. We didn't know how big the cross section should be, although you know approximately what the atomic sizes are, and so you know then there are limitations from just how big they are, whether or not they'll hit each other. But beyond that, we didn't have a lot of information. For that matter, theory at that point wasn't developed to the point where it was very much help.
ZIERLER: Peter, what was Jack's style like as a thesis advisor? Did you work closely with him? Was he mostly hands-off?
ARMENTROUT: He was largely hands-off, except when it came time to, you know, if there were things, issues going on in the laboratory, or things that I wanted to develop, he was always available to talk things over. When it got time then to write up results, he would always take time to go through those. I have a wonderful picture of Jack and I sitting on a bench outside of Noyes laboratory. We were going through my first paper at the time. It was a nice spring day, so we figured we'd do it outside. The campus photographer happened to come along, and took our picture. But that was his style. He let students do what they could do, and then provide support when they needed it.
ZIERLER: Did you recognize or did Jack encourage you to publish, given how important this research, how new this research was?
ARMENTROUT: I don't know of any researcher that doesn't think publishing is probably a decent idea because, ultimately, that figures back into whether or not you get the next grant. Also, it's how you get the attention of your peers. I never felt pressured, per se. But it was always, for me, the way you showed what you had done was by publishing it, and getting it out there. For me, writing was never a real issue. That was never a stumbling block for me.
ZIERLER: I meant that question more in the vein of, you know, it's relatively rare where a graduate student who's working on something that's truly brand new to the field, that this is new science, more so than coming up with an experiment which might argue on the margins with an established literature.
ARMENTROUT: I'm not sure that I appreciated at the time how novel what we were doing was. I know that Jack knew how novel what we were doing was because there had been other experiments like this that had been done, just not on actinide chemistry.
ZIERLER: Beyond Jack's lab, were there other labs, other chemistry labs at Caltech that you worked with?
ARMENTROUT: For the most part, no. I was familiar with them because my wife also worked there, and I had a couple of good friends working in other laboratories as well. I seriously contemplated working for Peter Dervan when I first came to Caltech. But working in ion chemistry and working with Jack ended up being too strong a pull. But, no, I didn't. The one other laboratory that we did work with was Bill Goddard's theoretical group. That was a place where I really got a good grounding in doing computations, although not nearly at the same kind of level because there weren't the package codes that are available now. But I became well-versed in doing high-level theory because of my interactions with Goddard.
ZIERLER: Peter, looking back, what was the research culture like at Caltech? Did it feel competitive or intense? Was it laid-back?
ARMENTROUT: I would never say that Caltech is laid-back in any field, particularly. But it wasn't competitive. Again, in my case, I built this instrument. I got to use it. I ended up training a couple of other people on how to use it as I was finishing up, and so it went on and continued for quite some time. But I never felt competitive about what was going on because I had my own project. I think there were others in the group where there was more competition, where they were all using the same instrumentation.
ZIERLER: Besides Jack, who else was on your thesis committee?
ARMENTROUT: John Bercaw. Let's see, I'm blanking.
ZIERLER: Bill Goddard?
ARMENTROUT: Bill must have been on it. Yeah, I think Bill Goddard was on the committee too, now that I think about it, now that you suggest that. I don't think Aron Kuppermann was. He would've been one of the other people. I'm not getting a vision.
ZIERLER: Anything memorable from the oral defense? Any good spirited conversations about the science?
ARMENTROUT: Oh yes. [laugh] Apparently this particular story has lasted well beyond my time there. But at the time, we had to write three proposals, and then defend those. One of mine had to do with isotope separation for uranium. There was some thought. My committee decided that I didn't appreciate hyperfine splitting, which is because the nuclei for different isotopes are slightly different, it turns out that the spectroscopy changes a little bit. What I proposed would probably be messed up by this. They wanted to get me to think about this, and so they wanted to start by just asking me to write down the Schrödinger equation. When they did that, these are stressful events, and the Schrödinger equation was not occurring to me very rapidly. I said, "Relativistic or non-relativistic?" just to buy some time. They said, "Whichever you like."
There's fundamentally no difference in writing down the two things; it just means that the operator is slightly different. It bought me no time, and it put me in a position where I was sounding like I should be able to come up with either the relativistic or the non-relativistic version of the Schrödinger equation, and I couldn't do either. They drug me through this whole thing, and we finally got to the point where, yes, I was appreciating the fact that the Born-Oppenheimer approximation may not be totally satisfactory in this particular case. But the idea that somebody would, in order to buy time, ask the question, "Relativistic or non-relativistic?" apparently has taken on a life of its own. I had a postdoc that joined my group many years later, maybe 15 years later, and she had heard this story.
Bell Labs as the Temple of Basic Science
ZIERLER: Peter, we talked about some of your graduate students going on to industry. Back when you were at Caltech, was almost everybody exclusively on an academic track? Were you ever interested in going into industry?
ARMENTROUT: I certainly looked. I did a postdoc in industry, so I went to Bell Laboratories, and was offered a position there. I was offered a position at a couple of industrial places coming out of Jack's lab: Bell Labs and GE and Kodak for that matter. I certainly considered it. I would not say that everybody in Jack's group was on an academic track. Certainly, he has had a number of students that have gone on to academic careers, but that wasn't a given. I don't know that it can be for virtually anybody, but there may be some exceptions.
ZIERLER: It's so funny to think about working in industry when you go to Bell Labs because the joke is it's more academic than academia sometimes.
ARMENTROUT: At the time, I joined it just before divestiture, so it was still in its heyday, substantially. It underwent a massive reorganization and changed shortly after I left. But at the time, it was the Bell Labs of that era.
ZIERLER: Now, what was the connecting point between Caltech and Bell Labs? Did Jack know someone? Were they a leader in the kind of chemistry you wanted to pursue?
ARMENTROUT: No. I went to Bell Labs. I interviewed two places there. I interviewed in I think they called it their Section 2, which was the more applied industrial part, as well as then Section 1, which was the more academic-oriented. There was an experiment there that intrigued me, which was a means of taking an ion beam, efficiently neutralizing it to generate a neutral beam that was a hypersonic beam, so you could accelerate the neutrals. My thought was, could I possibly do something like what I had done at Caltech with ions but do it with neutrals? Look at the kinetic energy dependence of neutral-neutral chemistry, and then you would use ionization techniques in order to detect the products. But the reactions would be taking place at elevated kinetic energies between neutral molecules. I wanted to go and learn whether or not that was feasible to do that using this particular technique.
ZIERLER: Was Robert Freund, was he one of the pioneers of this research?
ARMENTROUT: Yeah, he was. He was the one that had built the apparatus at the time, and built up the expertise to do this particular experiment.
ZIERLER: Was this brand new science to you? Had you been exposed to any of this at Caltech?
ARMENTROUT: There are very few experiments like this, and I don't know of any of them right now, because I concluded after my postdoc that this was not going to work—
ZIERLER: [laugh]
ARMENTROUT: —that it was a waste of time. But it was good to learn that then, as opposed to trying to do it while I was trying to establish an academic career.
ZIERLER: What was it like when you arrived at Bell Labs? It was still, as you mentioned, the temple of basic science. What was that like for you?
ARMENTROUT: It was an interesting thing because you'd go to lunch, and people were talking, and it was this very active, stimulating environment where lots of things were going on. There were a couple of laboratories nearby where I developed good friendships with people that I still know today. It was just a very active place.
ZIERLER: We use the term, of course, interdisciplinary or multidisciplinary today. Were the chemists siloed or not at Bell Labs? Could you work with the physicists if you wanted to?
ARMENTROUT: Yeah, absolutely, there were really no walls there for what you could do or wanted to do. I talked to quite a few people doing a large number of different things there.
ZIERLER: Now, the term metastable as it applies to atoms and molecules, what is meta? What does that mean there?
ARMENTROUT: It just means that it has a lifetime associated with it that's long enough that you can see it, but it really is not in a state that will last forever.
ZIERLER: Now, of course, when we say that, the temple of basic science, there was no requirement that the research should be responsive to the bottom line of Bell as a corporation. Was there any commercial benefit realized or not for this work?
ARMENTROUT: For what I did, absolutely none. For that matter, for a lot of the labs nearby, that was not really what was going on in those laboratories. They were just doing good science. A lot of the people then that I interacted with most strongly moved to academia shortly after divestiture.
ZIERLER: Now, how big was Freund's group? Was everybody there involved in this research?
ARMENTROUT: Freund's group was very small. It was me and one other guy.
ZIERLER: That's the standard model for Bell, right, small operations?
ARMENTROUT: Virtually everybody there had a postdoc, maybe two, and no more than that.
ZIERLER: Now, the divestiture, as you mentioned, was right on the horizon. Did those rumblings begin? Did you feel any of that while you were there?
ARMENTROUT: I don't remember exactly, but I ended up rooming with a guy that was then in the more applied areas, and so he was involved in packaging. It's not like Bell Laboratories didn't do anything that served telephone customers. A very large part of the company was this Section 2 part, where they did, you know, it was all sorts of things then that really were commercially appropriate and commercially viable and aiding things. But Section 1 where the fundamental research was done, that's what developed the transistor. It's hard to imagine. It would've been developed eventually, but it wouldn't have been developed when it was without this really fundamentally part of Bell Laboratories. That's certainly why they wanted to keep it going because they were hoping they'd end up with a new thing just like that. But that wasn't the requirement for anybody. The whole idea was to get really good people in there, and let them do what they thought was interesting.
ZIERLER: Did you give any thought to becoming a staff scientist, to making a career at Bell Labs before you would know about the monopoly?
ARMENTROUT: As I say, when I first went there, I was offered a position as a staff scientist. But after I finished with the postdoc, I had a better understanding of what it was that I really wanted to do or wanted to try. At that point, I think I could have easily gone and become a staff scientist there if I'd wanted to. But that isn't what I was interested in.
ZIERLER: Now, to go back, I want to make sure I understand the comment. Did you not see the work that you were doing as viable scientifically in the long term?
ARMENTROUT: No. Scientifically, it was fine. It was good science. The metastable thing that you mentioned, that remains, as far as I know, the only time anybody has measured the electron ionization cross section of a metastable anything. I don't think that nets a lot of people a lot of information, but it's an interesting niche thing to have done. It's good science, but it's certainly not mainstream. It was a very difficult experiment, but we managed to pull it off.
ZIERLER: Were you recruited to Berkeley? Did you go out on the job market relatively early in your postdoctoral assignment?
ARMENTROUT: I was there at Bell Labs for a year and a half. Was I recruited by Berkeley? There were a couple of us at the time, other people that I didn't know at the time but have become friends since then, they were going to the major chemistry departments. Wisconsin, Illinois, Berkeley, all had job openings for physical chemists. A number of us went to each of those different places.
Transition Metals at Berkeley
ZIERLER: Tell me about building up your lab at Berkeley. What was most important for you?
ARMENTROUT: Most important was, I think, really getting good students, and giving them the opportunity, the same opportunities that I had had when I was a graduate student. I had a pretty good idea of what I wanted to do. When I first got there, I spent a long time at the drawing board, just drawing up things that I wanted the machine shop to make for me. I got a laboratory from somebody that had done something similar to what I had done, Bruce Mahan, and so I could use a lot of his equipment. In retrospect, that was really a cost-saving measure on Berkeley's part. They could give me this laboratory that didn't cost them anything, and keep the expenses down. It worked out fine. I got done what I wanted to do. I still have some of that equipment, and it still operates today, including one of my favorite pieces, in fact, a little magnet that was hand-wound by Yuan Lee when he was a graduate student working for Bruce Mahan. Yuan then later went on to win the Nobel Prize in chemistry and was a colleague at Berkeley, for that matter.
ZIERLER: What were the most important sources of funding to get your lab up and running at Berkeley?
ARMENTROUT: NSF primary, but then I also got, fairly early on, I got some Air Force funding as well, really designed to look at plasma chemistry in general.
ZIERLER: Now your focus at this point on transition metals, a transition between what and what?
ARMENTROUT: They're called transition metals because over on the left-hand side of the periodic table, you have the alkaline and the alkaline earth elements. Then over on the right-hand side, you get into the main group elements: carbon, nitrogen. But as you go down the periodic table, then they turn into metals. The transition metals is this big bunch of stuff in between those two things. They're transition metals because you start occupying instead of just s- and p-orbitals and electrons, you start putting electrons into d-orbitals. D-orbitals then give transition metals and metals in general the flexibility to do the catalytic chemistry that we talked about earlier.
ZIERLER: What were some of the big questions you were pursuing with transition medals?
ARMENTROUT: One of the key things I was interested in, and nobody else really could get a handle on, is whether or not different electronic states of those metals would affect their chemistry. We found fairly early on that they did. But one of the key things that I focused on was understanding then how changing the electronic configuration, what orbitals the electrons were in, did that change the chemistry? Because if you could do that and understand that, then you could conceive of directing the chemistry in ways that you might like to, for industrial purposes.
ZIERLER: This might require a really technical answer, but I wonder if you can explain how you change the configuration. How do you perturb the electrons?
ARMENTROUT: You can do that in a couple of different ways. What we did was just change the temperature at which we generated the ions because, as you increase then the energy available when you do the ionization, you can begin to populate states that are excited that need more energy. If you do that in a controlled fashion, then you can systematically change whether or not you've just got the ground state or whether or not you're populating excited states and, for that matter, how high you populate them. We developed three sources that we could use interchangeably that would enable us to vary that over a really fairly wide range.
ZIERLER: Now, as a matter of techniques and instrumentation, I wonder if your time at the Beauchamp lab or at Bell Labs was more relevant?
ARMENTROUT: Fundamentally, my time at the Beauchamp lab was more relevant. I certainly learned additional tricks and tools when I was at Bell Laboratories, but fundamentally that experiment was designed to look at neutral-neutral interactions, and then detect the products via ionizing them afterwards. Whereas ion chemistry was the name of the game in the Beauchamp laboratory, and fundamentally what I had decided then, you know, I would continue to do.
ZIERLER: Did you enjoy your time at Berkeley?
ARMENTROUT: Berkeley is an interesting place. It's a very big department. I would say that Berkeley is a department that is full of itself, so it's very proud of what it does. I had the either good fortune or misfortune, depending on how you want to look at it, that Yuan Lee, my colleague at the time, won the Nobel Prize while I was there. What that did was set expectations for physical chemists in the department at a fairly sky-high level. The question when my tenure came up, for instance, was, would I win a Nobel Prize or not? It was contended by at least one of my colleagues that I might win the Priestley Medal but probably not win a Nobel Prize. Take that for what it's worth. Does that mean that you don't deserve to be there anymore? Apparently they decided it meant that.
ZIERLER: Tell me—
ARMENTROUT: I got a very good start. I had very good students, and I achieved basically everything that I wanted to achieve while I was there.
The Excellence of Utah Chemistry
ZIERLER: Were you aware of Utah's reputation in chemistry? Was that a very positive transition for you?
ARMENTROUT: It was a transition for me in some respects. In terms of the stature of the department, Berkeley is generally viewed as being a better department. It's bigger in part, and it certainly has more people that are well known, I would say, in general, again, partly because it's a bigger department. I certainly knew that Utah was a good department. But I also found then that the colleagues that I had there were much better colleagues for me than what I had at Berkeley, for that matter. I also got students that were nearly the same quality. In the end it, was a lateral move for me and a positive in terms of my interactions with colleagues.
ZIERLER: Switching universities, building up a new lab, did that change your science at all? Was there a value in this jolt to your professional trajectory?
ARMENTROUT: I think it made me more determined but, no, I kept doing the, I mean, I was convinced that what we had learned and what we were learning was still very valuable and useful. I think I expanded the kinds of things that I wanted to look at a bit. But the kinds of things that we did and the tools that we used and had developed, I continued along more or less the same path.
ZIERLER: What new research did you take on? We were discussing transition metals at Berkeley. What was new or expanded when you got to Utah?
ARMENTROUT: I had just started looking at metal clusters, so now small chunks of metals, so rather than just the atomic chemistry, looking at cluster chemistry. That really expanded and became more vital. —I rebuilt the instrument, and made it behave and work a lot better than what I had at Berkeley. Then eventually I expanded into biological systems that we talked about a little bit earlier. We've continued to do the transition metal, the atomic chemistry. We've expanded them, you know, to lanthanides and actinides and now into biological systems. We did cluster chemistry for a long period of time, although that operation is now shut down.
ZIERLER: Now, the entrée to biology, what was the opening for you there?
ARMENTROUT: It was a really terrific postdoc as much as anything. She got her PhD at Caltech as well, and is now at Wayne State University. She wanted to look at some biological systems, so we started doing that. Then that's just snowballed.
ZIERLER: Now, the phrase—I want to make sure I heard right—cluster chemistry?
ARMENTROUT: Clusters, yeah.
ZIERLER: What are the clusters?
ARMENTROUT: Clusters are just what it sounds like. If you take a couple of transition metals, and stick them together, you make a cluster of metals. In the field, that's just called cluster chemistry so, in our case, metal cluster chemistry. We would look at how does the chemistry change as you go from an atom to a diatom of the same metal, or three atoms, or we went up to about 19.
ZIERLER: Peter, you've been recognized for your commitment to teaching. What have been some of the classes that you most enjoyed teaching both undergraduates and graduates?
ARMENTROUT: Undergraduates, I really do enjoy teaching thermodynamics and kinetics. It's what I do, and I like to think that I can bring them to life a little bit, perhaps more than many people, because I'm passionate about both of those things. I'm teaching that right now, as a matter of fact. In graduate studies, I've taught quantum, as well, spectroscopy. But my favorite courses are again statistical thermodynamics and kinetics.
ZIERLER: Tell me about being named a Canon Fellow. What did that allow you to do?
ARMENTROUT: I think, as much as anything, it wasn't that much money. But it gave me a little bit of extra cash to spend on a couple of things. I think, as much as anything, it was just an internal recognition that I was doing OK, and that if you've got some sort of a title that you can stick at the end of your letterhead, other people will notice, and that helps things.
ZIERLER: I want to ask about the scholarly community of chemists, the things that are most important to you. Let's start first on the society side. What are the most important scientific societies for you to be a part of?
ARMENTROUT: I've been a long-term member of the American Chemical Society as well as the American Physical Society. I think, in fact, I joined both of those when I was a graduate student, and I value both of those. I'm also a member of the AAAS. These days probably the society that matters the most to me is the American Society of Mass Spectrometry because what I do and the people I know there probably overlap as much with them as anyplace else.
ZIERLER: What's the value of being a member of a society that has such a specific focus to it?
ARMENTROUT: I think fundamentally it's meetings for me, and the idea then that you get to share what you've done, and hear what other people are doing, again, in areas that you're interested in. The societies put on meetings then that draw people together and allow them to interact. It's those interactions that often lead to advances in science.
ZIERLER: On the editorial side, what are the most important journals, first of all, from a service perspective, where you've served as an editor or a reviewer?
ARMENTROUT: I've served as a reviewer for something like 30 or 40 journals, so that doesn't narrow it down a whole heck of a lot.
ZIERLER: [laugh]
ARMENTROUT: The ones that are most important to me are Journal of Physical Chemistry, Journal of Chemical Physics, these days Journal of the American Society of Mass Spectrometry. I've been a long-time editorial board member of the International Journal of Mass Spectrometry. Those are probably the ones that I look to as often as any.
Scintillation for the Air Force
ZIERLER: Peter, we'll bring the story right up to the present. What are you working on these days?
ARMENTROUT: Anything and everything. We've got projects for the Air Force right now that have to do with lanthanide chemistry. Lanthanides are like actinides. Then I've got a project with the Department of Energy Heavy Element Chemistry program to look at actinide chemistry. Our experiments are designed to provide some thermodynamic information that act as benchmarks for theoreticians. The Air Force Project has some reasonably direct applications that they're interested in as well. But all of those things, in both cases, you're talking about elements now that are below the transition metals, so they're sort of transition-transition metals, if you will, because they involve now f-electrons. The science, despite the fact that we've dealt with these things for a long period of time, I'm not sure that we have a really good quantitative understanding of just what f-electrons can do. I'm very interested in the possibility of can we understand better how to move electrons in and out of f-orbitals where we can store the electron density if we want it, but then pull it out if we need it for catalysis of some sort. How easy or how hard is that to do, and how does that change then as I move the electrons around? As I say, from a quantitative point of view, I'm not sure that that's terribly well understood.
On the other end, our biological program has probably increased over the years from where it started. We do a lot of work which is fundamentally related to analytical applications of understanding how to sequence proteins, what their primary sequence is, just which amino acid residues follow one after another. Mass spectrometry is used to do that in many cases. But the mechanisms for how that process works are not necessarily understood very completely. Potentially if they were, then the application of the completeness and the robustness of the identifications of proteins could be improved. Then there are also processes that I mentioned that are interesting from a point of view that they relate to things like aging and neurodegenerative diseases. Can we understand that well enough that perhaps somebody else could develop some sort of a therapy to avoid those kinds of things?
ZIERLER: Now, to go back to the support from the Air Force, is this the Air Force's long-standing interest in supporting fundamental research? Did they see specific applications to what you're working on?
ARMENTROUT: There is a specific application for this particular item.
ZIERLER: But that's something that you might not be able to talk about?
ARMENTROUT: I can tell you a little bit about it. There's a process known as scintillation, which is that radio waves pass through the ionosphere twice as they go up to a GPS satellite and back to someplace else. If you're doing radio wave communication, you don't want scintillation to occur because imperfections in the plasma density then diffract the radio waves and disrupt communication. The Air Force is sufficiently interested in not losing radio wave communication sometimes that they're looking for ways to get rid of these imperfections. One of the ways potentially of doing that is to artificially enhance the plasma in the ionosphere. There are reactions known as chemi-ionization reactions that potentially could do that. A chemi-ionization reaction is a little unusual. It's a chemical reaction that is exothermic enough that it induces spontaneously ionization, and that's relatively rare. But lanthanides can do this under special circumstances. What we've been doing is looking at the thermochemistry associated with that, partly to identify what lanthanide might work best for this particular process.
ZIERLER: Peter, to go back to your interest in understanding storage of electrons, is there an aspect of this that's important for battery research?
ARMENTROUT: I think the simple answer to that is that I don't know enough about battery research to give you a really good answer. But it's that kind of thing, you know, moving electrons around and orbitals, does that change the conductivity, so could one develop then a battery based on lanthanides? That's out of my realm of understanding, I would say, but I wouldn't say that it's out of the realm of possibility.
Applications from Biology to the Environment
ZIERLER: Thinking about the biological side, human health, and therapies, best case scenario, what would be a question that starts with your research that ultimately wonderfully might end up helping people?
ARMENTROUT: From my understanding, the fastest degradation process that proteins undergo is a deamination, which is a loss of ammonia. There's a particular side chain of the amino acid asparagine that undergoes this deamination. It forms a cyclic species, loses ammonia, and the rate that that happens is very dependent on the amino acid next to the asparagine residue. What we've done is begun to look at that particular process for small molecules. But we've at this point developed is an understanding of the thermochemistry, and we've looked at the mechanism for that deamination process. That deamination process has been implicated in, because it's the fastest spontaneous degradation process, it's been implicated in aging, and it's been implicated in the onset of Alzheimer's and Parkinson's. If we understood the mechanism whereby this happens, one can at least potentially envision that you could develop a therapy that would slow the process down because, depending on what that secondary amino acid is, this process can occur daily, or it can extend out to something like 10,000 days. 10,000 days is 30 years. If your proteins are falling apart on a daily basis, that's probably bad for you. But if it takes 30 years, I like to say you can live with that.
ZIERLER: That's right. [laugh] Peter, one aspect of your research as it relates to environmental chemistry we haven't talked about is its potential relevance in cleaning up nuclear waste sites. I wonder if you could speak to that.
ARMENTROUT: It's this idea of trying to understand the hydration energies of various ions. If you stick a metal into an aquifer, it tends to ionize, and it's surrounded by water molecules that stabilize it. If you want to extract that, then you've got to extract the metal ion from the water that's surrounding it. There's a balance, there's a thermodynamic balance in what can you use to extract it versus the thermodynamics of it being solvated. What we've done is looked at the thermodynamics of metal ions as they've been hydrated, to try to understand that kind of process.
ZIERLER: Now, of course, in this country, we're in a state of suspended animation with regard to nuclear waste. We don't have everything at Yucca Mountain. There doesn't seem to be a long-term solution. Is this research something that might be responsive to dealing with nuclear waste where it is, and not centrally storing it all in one place for the long term?
ARMENTROUT: I would say that nothing that we have done directly so far addresses that problem directly, but certainly one can imagine that some of the chemistries that we've looked at could have impact on understanding processes that might be developed to do what you're talking about.
ZIERLER: Is this something societally that you're concerned about that we don't have a long-term solution for nuclear waste?
ARMENTROUT: Absolutely. I'm of two minds on this because, honestly, I think that long-term nuclear power is probably a good way to go because it's going to be cleaner than certainly coal- and oil-fired power plants. But we absolutely need to come up with better solutions to the long-term storage. Although potentially what that really means is that you may not want to use uranium. You may want to use thorium reactors, which my limited understanding is, again, can be quite a bit cleaner, and also don't have a nuclear proliferation danger associated with them. There are technologies out there that are potentially useful, but you still have to worry about the radioactivity of these things. Finding solutions then for how to sequester these things safely is an ongoing problem that hopefully some of the things that we do might be useful for.
ZIERLER: Peter, your work in aerosols and atmospheric chemistry, does that get you involved at all in climate change scientists looking at greenhouse gases?
ARMENTROUT: I think it's fair to say that we don't do aerosol chemistry directly. Again, these hydrated systems would pertain to that eventually. But, right now, no, nothing we do, I think, is directly associated with climate change.
ZIERLER: Now, I asked about—
ARMENTROUT: —which I think maybe advantageous, so yes.
ZIERLER: [laugh] I asked about battery research. That was a little far off from your from your research purview. This one might literally be out of this world. But when we get Mars samples back to Earth, I understand that mass spectroscopy is going to be a vital aspect, you know, the kinds of things that we can't study in situ on Mars that we can do back here. Would you try to get involved in that? Do you see yourself having a particular area of expertise to study these samples, and to understand what they're telling us?
ARMENTROUT: I can imagine that if the samples start coming back, and there are interesting elemental concerns associated with metals that some of the expertise that we've developed over the years in how metal chemistry works and what things drive it could potentially be useful there. I don't think that what we do per se is sufficiently on point that I would necessarily call somebody up, and say, "Let me get my hands on this thing."
ZIERLER: For the all-important question of searching for life in these samples, is there a metal chemistry perspective that should be front and center when we ask that question?
ARMENTROUT: Life as we know it is really organic-based chemistry for the most part, and metal chemistry can affect that, and certainly trace elements are biologically relevant. We need a little bit of iron, we need a little bit of cobalt, or nothing works anymore. It's not like metals aren't relevant. But I think when you're looking for signs of vestigial life, the metal chemistry isn't going to be front and center; it's the organic chemistry.
ZIERLER: Although that perspective is decidedly, of course, Earth-centric, isn't it possible that there's a new type of life that might exist elsewhere that we might be open to?
ARMENTROUT: Star Trek's Horta, right—
ZIERLER: [laugh]
ARMENTROUT: —the silicon-based chemistry. It seems unlikely. Carbon is such a versatile element in terms of what it can do that it's unlikely that the range of things that occur in life, which as we know it are really carbon-based, that that can be matched by any other element.
ZIERLER: Peter, on that note, for the last part of our talk, if I may, I'd like to ask a few retrospective questions about your career. Then we can end looking to the future. To go back to your relative lack of knowledge or perspective or even, for example, Jack's about just how new the science was as you were doing it as a graduate student, do you think it was valuable to be naïve in that regard? Would it have been better for you to have a greater understanding of just how new and important this research was at such a formative stage in your career?
ARMENTROUT: I can go back now, and find books that would've told me that what I was trying to do wasn't possible. I think the naïveté was a reasonable component of achieving some of the things that we managed to achieve, and for good reasons. It's not like those things were wrong, it's just that they were incomplete, and now there's a better understanding.
ZIERLER: Is there a lesson there in terms of not being deterred by what the established science tells you? In other words, if you had read all those books that said what you were doing was not going to be going anywhere, is there a value to sticking with your guns, and to having the confidence that you shouldn't listen to the established knowledge?
ARMENTROUT: Absolutely. The trick is always knowing where that fine line is between doing something that people thought was maybe a little unexpected, and just banging your head against the wall for another year or so. But it's definitely the case that breakthroughs don't occur if you're doing what everybody else is doing.
ZIERLER: What has stayed with you from being Jack's student and being a Caltech graduate? What has informed the way you've gone about your science?
ARMENTROUT: I think this was brought home to me recently. Carolyn Bertozzi just won the Priestley Medal for the ACS, one of the things that she is well known for is her style of mentoring. I honestly think that Jack's style is a bit like that. I never felt like and I've never tried to make my students feel like I work for him or that they work for me. We work together to try to make the student learn as much as they can, and progress as much as they can, and to learn as much that we can share with the world as we possibly can. I think that sort of mentoring style all goes back to my time with Jack, and the way he and I interacted and, for that matter, have continued to interact over many years. I like to think that I've been able to do that for my students as well.
ZIERLER: Peter, being at the very tail end of Bell Labs before the divestiture, I wonder what your perspective is. What has the United States lost? What has science lost with Bell's breakup?
ARMENTROUT: Bell's breakup was probably inevitable because, honestly, the Section 1 didn't have anything to do with telephone calls and things like that. It was quite clearly a laboratory that was funded by all of the people to do whatever science these crazy scientists thought was interesting. I think in the end, especially at the beginning of all of this, we benefited from that, from some laboratory somewhere having a bit of an industrial bent to it, being free to explore lots of different things. As I say, that's where the transistor was first developed. I think a lot of mathematical algorithms that we still use today were originally developed there. But it's hard to say that that's a reasonable thing to do. It's valuable as long as everybody was in the dark about it, that it happened, and it was good, and everybody was paying a couple of cents a year to fund this sort of thing. But should they have been paying that? It's not really defensible from that point of view. Have we lost something? Yeah. But we've lost lots of things that are comparable. The innocence of my youth is long gone, for instance.
Lessons in Persistence
ZIERLER: Peter, reflecting on your tenure experience at Berkeley and, as you say, that redoubled your determination, what are the life lessons for assistant professors, and the things that they're going through at this stage in their career? What do you think they could learn from your experience?
ARMENTROUT: I think that not getting tenure is not the end of the world. It all depends on whether or not you do not get tenure because you were ahead of your time, because you weren't appreciated for what it is you had done, or because the mood of the department at the time was just not right. I've known people that have gone both ways, though, people that have turned it into a victory because they've gone on to thrive. I know a number of people like that. I also know people that have just completely disappeared, and it's not because they hadn't been doing good things, they just hadn't been doing quite enough of it, and faced with that obstacle, they gave up at some point or another. But the main point is that I think most students, a lot of beginning professors believe that you've got to get tenure where you are, otherwise, that's the end of the road—and that clearly isn't the case.
ZIERLER: Being in the Department of Chemistry at the University of Utah, after all these years, how has the department changed?
ARMENTROUT: When I first arrived, it was about 20 men, and it had a good reputation. There were a number of really good people there. But it wasn't particularly diverse, although we did have one Black professor, which was fairly unusual for chemistry in general then. The thing that's happened is that it has diversified quite a bit. We have one of the higher percentages of women on the faculty now across the nation. Things have become more multidisciplinary, so less sectored into sort of the classical disciplines. We certainly have ventured more into materials and biological chemistry than ever before. The infrastructure has improved over the years, I'd say.
ZIERLER: The diversification of the department, both from the human side and the scientific side, either from a service perspective or in your role as a senior member of the faculty, where have you played a positive role in those developments?
ARMENTROUT: I was chair for six and a half years, and championed a couple of diversity things. We had the first parental leave policy on campus that I established. We built one building for NMR, exclusively for NMR. A proposal that I helped develop toward the end of my time as chair eventually turned into another half of a building that now has enabled the department to continue to grow and provide more modern space. It's been both infrastructure in terms of buildings but also the mood of the department and how we approach things, I think, I contributed to when I was chair, and then subsequently as just a general member of the department.
ZIERLER: Peter, if I can ask you about the knowns and unknowns in your research. Of course, the science never stops. There's always more discovery. But I wonder what sticks out in your memory perhaps that's most satisfying, a research question that you feel you've most fully resolved?
ARMENTROUT: One of the projects I've been interested in for a very long period of time has been methane activation. Methane is when you go by an oil field, and you see these flames, and they're just burning off the methane, which is a real pity because methane is a really energy-rich thing. But it's found at the top of these oil pockets, and there's no good way of turning methane into other things. It's gaseous, so it's hard to transport because you need to liquefy it, and that's hard to do. There's no good storage techniques. What you'd really like to do is be able to turn it into what remains energy-rich, something like methanol. The problem is that the first step in the oxidation of methane to methanol is the hardest step, and all the subsequent steps are really easy. Once you turn methane into methanol, you turn it into CO2 right away. What you'd really like to find is some sort of a catalyst that enables you to selectively activate methane, and turn it into methanol or something like that. I've been looking at interactions of metals of all sorts with methane, throughout my career. My first one was uranium plus methane, back at Caltech. But we've looked at virtually the entire periodic table of transition metals reacting with methane, to try to understand that particular process. Some metals, some fairly heavy transition metals activate methane spontaneously at room temperature. But as you go up in the periodic table, they stop doing that. The question was always why, and what have we produced when we activated the methane? Recently we've done experiments in the Netherlands, where spectroscopically we've identified the structures of -- basically across the periodic table, up and down and side to side, -- what the species are that you form if you activate methane by an atomic transition metal. It's satisfying because it's been a project done that was largely initiated—the first person that found out that the heavy metals would activate it was Jack with a subsequent graduate student, Karl Irikura, some 30 years ago. Really just in the last couple of years, we identified what the species that they first saw that many years ago, what are they really.
ZIERLER: On the flip side of that question, the unknowns, you emphasized in particular some of the current work you're doing, how little is understood about really basic aspects of chemistry. What do you see particularly for the graduate students, the postdocs in your group? What are the most fertile areas of research to explore in the areas that you work in?
ARMENTROUT: We've seen a lot of effects having to do with what happens when reactions occur at higher energies, and that's equivalent to higher temperatures as well. We've seen some interesting effects that are not completely understood. There's a lot that can be done there on all sorts of different fronts to better understand that sort of thing. By the same token, I also have a postdoc that has been with me now for six months, and he's pushing us into larger and larger peptides. There are interesting things that happen that change from the small peptides, certain chemical reactions can occur, certain transformations occur very readily, and then those stop and turn into something else when you get to the larger ones. The reasons for that and why the mechanisms change have not been fully appreciated. But we have a tool that should enable us to begin to look at that. There are just so many molecules that you can look at that nobody knows anything about. There's enough to keep a couple hundred people busy for a long time if they wanted to do that sort of thing.
ZIERLER: Peter, on that note, we can end looking to the future, and to go back to this idea of technology as a driver of discovery versus grinding it out in the lab, and always refining the experiments. For however long you want to remain active—it sounds like your lab is going as strong as ever—how will you use those to push forward the discovery? What's most important to you in that regard?
ARMENTROUT: We are in fact building right now. One of the things that I've become interested in is there's a tool known as ion mobility that allows you to separate species that are the same mass. They're the same molecule, but they could come in a different shape. They're just folded differently. You can also separate different electronic states of atomic metal ions this way. We're putting together one of these devices. We have a vestigial one already in place. But we're putting one together that'll have higher resolution that should enable us to do some brand new experiments that nobody else can do, both for biological molecules and for metal chemistry. I'm really excited to see where that's going to go because I think we can do some really pointed experiments that'll give us good quantitative information that, again, nobody else is situated to touch.
ZIERLER: I can't help but note there's a certain circularity or a parallel to your own experiences as a graduate student at Caltech in this regard.
ARMENTROUT: Yeah, absolutely, I think that's right. What goes around, comes around.
ZIERLER: [laugh] Peter, this has been a great conversation. I want to thank you so much for spending the time with me.
ARMENTROUT: I've enjoyed it as well.
[END]
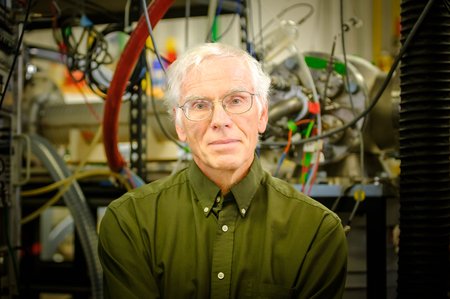
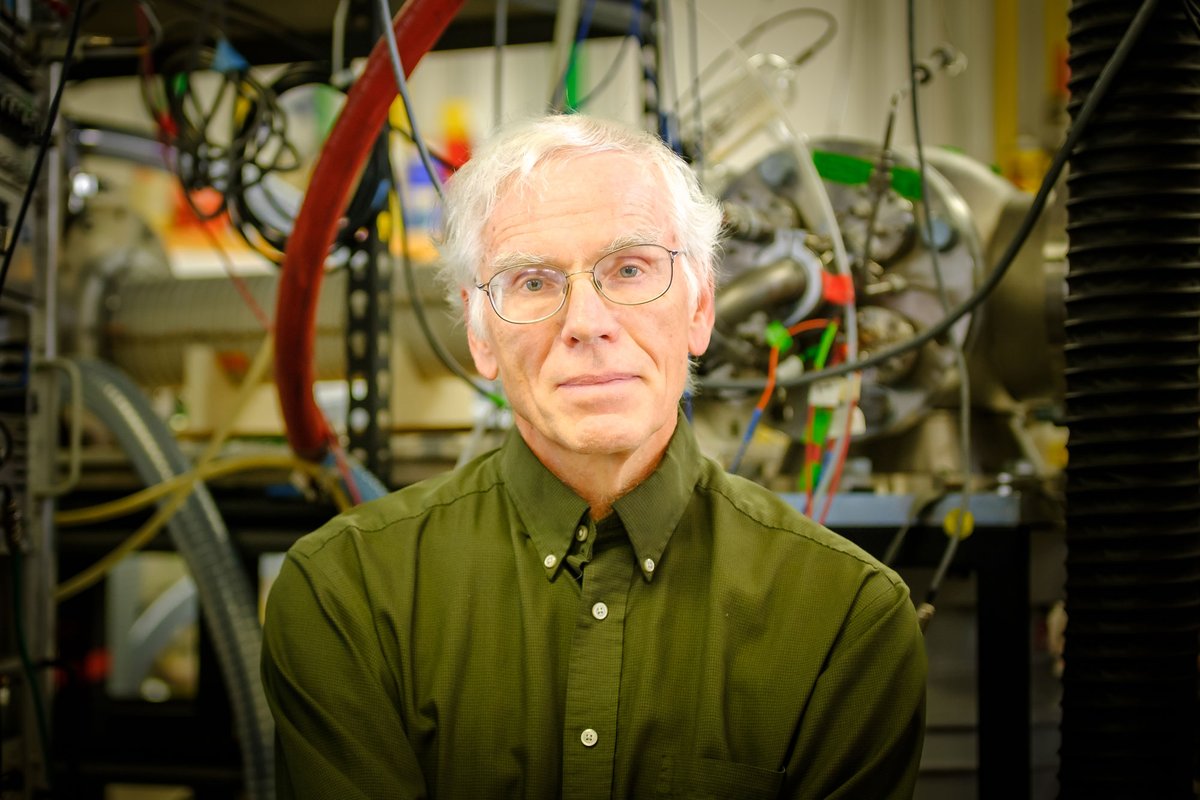
Interview Highlights
- Where Energy Meets Metals in Chemistry
- Bespoke Instrumentation in the Lab
- The Centrality of Mass Spectrometry
- Chemistry at Case Western
- Uranium and Isotope Separation at Caltech
- Forefront Research in Thermodynamic States
- Bell Labs as the Temple of Basic Science
- Transition Metals at Berkeley
- The Excellence of Utah Chemistry
- Scintillation for the Air Force
- Applications from Biology to the Environment
- Lessons in Persistence