Peter Quinn (Postdoc '82-'85), Computational Astrophysicist and Dark Matter Explorer
Some of today's most exciting and impactful work in cosmology and astrophysics is happening in Australia, and Peter Quinn has been central to those developments. A leading researcher in the search for dark matter and in the dynamics of galactic formation, Quinn has led a long and varied career. He currently serves as Executive Director of Research Initiatives at the University of Western Australia, which offers the opportunity to empower the careers of younger scholars and to remain focused on his own research.
In the discussion below, Quinn recounts his undergraduate education at the University of Wollongong south of Sydney, and his graduate work at the Australian National University in Canberra in 1978, where he completed a thesis on colliding galaxies. As a postdoctoral scholar at Caltech, Quinn was co-advised by the "Dream Team" of Kip Thorne and Peter Goldreich, which gave him an early view on LIGO and on Goldreich's foundational investigations of neutron stars. While at Caltech, Quinn began collaborating with Lars Hernquist (now a professor of astrophysics at Harvard), and the two remain collaborators to this day. After Caltech, Quinn held positions at the Space Telescope Science Institute during the intense days planning for the Hubble Telescope, and at the European Southern Observatory in Munich, where he worked on managing the vast amounts of data that astronomical observations were creating. This burgeoning of data led to the concept of a virtual observatory, in which astronomy data is made freely available and open for analysis (and discovery) to all people.
In his return to Australia, Quinn became Executive Director of the International Centre for Radio Astronomy Research, and he became closely involved in Massive Compact Halo Objects (MACHOs), which are a promising dark matter candidate. In reflecting on his career, Quinn describes the ultimate identification of dark matter - for which he is optimistic - as one of the great double wins for the future of science. It will be one of the most significant validations for the Standard Model of particle physics, and understanding what dark matter is will enable the creation of new tools that will unlock yet knew ways to see the universe, and to identify the next great unknowns to explore.
Interview Transcript
DAVID ZIERLER: This is David Zierler, Director of the Caltech Heritage Project. It is Tuesday, April 30, 2024. It is my great pleasure to be here with Prof. Peter Quinn. Peter, wonderful to be with you. Thank you so much for joining me.
PETER QUINN: Pleasure to be here, and great to be part of the project.
ZIERLER: Thank you so much, Peter. To start, would you please tell me your title and institutional affiliation?
QUINN: I am Professor Peter Quinn. I am the Executive Director of Research Initiatives at the University of Western Australia in Perth, Western Australia.
ZIERLER: Does that have more of an administrative component to it?
QUINN: Yes, it does. I guess it will come out during the interview, but academic careers take trajectories which are quite often zig-zaggy. Indeed, doing Big Science these days requires scientists to do lots of different kinds of things, research obviously being fundamental but also running projects, running institutes, working with people and budgets and international activities. These have all been part of my landscape. Right now, I am coming to the end of my time at the University of Western Australia, where I've been involved in research and putting together a major institute, which I was director of for 18 years. Recently I stepped down from that position, and now I'm helping put together a few new institutes at the University and internationally. Building institutes and running institutes and doing science with institutes, has very much been my world for some time.
Radio Astronomy in Australia
ZIERLER: Tell me about the International Center for Radio Astronomy Research.
QUINN: The International Center for Radio Astronomy Research, ICRAR as we call it, was founded in 2009. It was founded in Perth, Western Australia, as a joint venture, a collaboration between the two main universities—University of Western Australia and Curtin University—with significant funding from the state government of Western Australia. Before 2006, I was working for the European Southern Observatory based in Munich. I had been there for 11 or 12 years working on the VLT project, ALMA and various other things, doing research work when I had the time. Then a new opportunity arose that caught my attention. The Square Kilometre Array, a radio telescope, was looking at Australia as a potential site. Australia—Western Australia in particular—looked like an ideal place to build such a telescope for lots of reasons. The Western Australian government offered a professorship to basically come down and help Western Australia be part of this international effort to build the telescope.
At that time, they hadn't finished the competition to decide where the telescope was going to be. I decided to go to Western Australia with two things in my mind. One was to help Australia win the telescope, but also to create a new institute that would help anchor some of the science, some of the engineering, some of the development from the project into Western Australia. My main job was to create this new institute. There wasn't very much astronomy on the west coast of Australia at the time. Over the course of the following 14 or 15 years, we created ICRAR, which is an institute focused on four different things—astronomy and astrophysics obviously; data science; engineering; and education, outreach and the connections to industry. ICRAR was founded around these things. It now has more than 250 staff and graduate students, it is very internationally connected and is doing very well. It's something I'm very proud of. It has become an indispensable part of the SKA project but also part of the landscape of astronomy and science in Australia and internationally.
ZIERLER: Is the observational technique of radio astronomy particularly relevant for your research?
QUINN: That's a funny one, because I absolutely always tell people, "I'm not a radio astronomer." I'm not an optical astronomer. I'm not a theoretical astrophysicist. I was brought up in computational astrophysics, so my grounding was in using computers to solve astrophysical problems by doing simulations. But you find when you get involved with big problems in astronomy that you have to be a little bit agnostic about the kind of telescope that you get married to, at some level, because quite often problems require information from all kinds of telescopes (including computers). So, I've done a little bit of radio astronomy. I've done a little bit of optical astronomy, theoretical astronomy and computational. I've done all of these bits because they are all part of the big picture of trying to solve a fundamental problem. Dark matter, for example, requires all of these telescopes and all of these skills to focus in on that one challenge. Radio astronomy was certainly something I had an interest in, and I saw the potential in Australia to contribute to be building the SKA and giving a home to this telescope. SKA will a significant step forward for astronomy —it's a factor of 3,000 times more capable than anything we've ever built. When you do that sort of step forward, you're going to find some amazing things that you didn't even think about. You're going to solve some problems that you would love to solve.
ZIERLER: Your long affiliation with the European Southern Observatory, I wonder if you have unique perspective to share on the European effort to build an ELT, an Extremely Large Telescope, in Chile.
QUINN: The European Southern Observatory, based in Munich, is an international, intergovernmental body, 15 countries, mostly European. It was designed to help European astronomers by giving them the telescopes and the instrumentation they needed to do forefront science. ESO was founded at a time in the 1960s when European astronomy was falling well behind some of the efforts in the U.S., with the Palomar telescope and plans to build things like Keck, et cetera. The European efforts needed to be upgraded. In 1995, I was in Australia, working on a dark matter project and becoming familiar with how important it was to build facilities which were capable of doing great science. I was invited to go to ESO to work there on the design and the development of the ESO Very Large Telescope (VLT), that would lead eventually the ELT project.
ESO is a great organization. It has a combination of skills which is unlike any other organization in the world. It has the scientists, the engineers, the money and 60+ years' experience in building billion-euro-plus facilities. The natural aspiration after building the VLT was to consider a very large, single-aperture telescope, potentially the largest single-aperture telescope you could ever build. I have absolute confidence in them as an organization that they've got the people and skills to do it. They have built large facilities multiple times before, and I think if anybody in the world has a shot of pulling this off—particularly with the complications of the multimirror technology that they're using, I think ESO is the place to do it.
ZIERLER: Administratively and scientifically, where do you put the ELT in relation to the two major ELT projects in the United States—the TMT proposed for Hawaii and the GMT, which would be right next to the European effort in Chile?
QUINN: It's clear there have been challenges for both of the American efforts in terms of how they move forward—the issues with the sites, the issues with the funding. It's tragic in some sense, because it has slowed down these international efforts. It would be fantastic to have an ELT-class facility in the North and one in the South. But I think where we're headed right now is the European effort is on track to have first light probably in around 2028 or so. That's potentially five-plus years, maybe more, in advance of an American ELT, just because of the unfortunate delays that have occurred. However, major telescopes traditionally are international capabilities. They're rarely "owned" by any one country. They do international science. It's one sky out there. We're all doing the same problems. While one is certainly better than zero, two is even better, so I hope the American effort does get back on track and they can contribute. The US led effort now has a chance to optimize the design to complement the European telescope as well. It would be highly desirable if both facilities were very supplementary and complementary in what they can do.
ZIERLER: Are you still chair of the International Space Center?
QUINN: There are two new institutes that are being founded at the University of Western Australia. One is called the International Space Center, which I am the chair of the board of. The other is called the University of Western Australia Data Institute. I'm the chair of the board of that as well. Those are the two new institutes that I've been helping get off the launchpad, so to speak, after I finished with my ICRAR responsibilities.
ZIERLER: Tell me about the mission and goals of each of them.
QUINN: The Australian Space Agency, Australia's equivalent of NASA at some level, was created in 2018. This resulted from a government decision to make Australia a "player" in space, that would develop a space industry and contribute to space exploration and space science on multiple fronts. So, there was an opportunity to galvanize, in various ways, the Australian space-related expertise. We had a look around at the University of Western Australia, and we discovered there was a significant body of work which was certainly space work. It was in multiple different places around campus that were not connected up in any shape or form. There was work going on in laser communications to LEO, propulsion systems, energy sources, space medical issues, designing space habitats, growing plants in space and writing laws that are appropriate to space. All these things were happening around the campus, involving over 150 staff and students.
What we decided was to put a wrapper around this and christen it as the International Space Center at UWA, connect the parts and provide new funding so that there was cross-fertilization between the research groups enabling new joint research projects. We could also present the collective of skills as a contribution towards a pan-Australian space industry of some sense. It's working extremely well. There are a number of new research opportunities that have been identified which are the cross-product of all the parts. There are also some brand-new activities which have come from thinking from the members as well. It's a productive way to form an institute once you have a well-defined opportunity like the creation of the Space Agency.
Dark Matter in Historical Perspective
ZIERLER: Let's now move to your research. Of course you're talking to us from Johns Hopkins. Is this an opportunity to get away a little bit from all of the administration and just to focus on the calculations?
QUINN: It very much is, and I welcome it. I want to keep the research "gray matter" alive. I've been passionate since the beginning of my career about dark matter, about how dark matter might influence galaxies and the universe on the whole. The cold dark matter theory of the universe has been incredibly successful at explaining many of the features of the universe. It's embarrassing, in a sense, that as astronomers and astrophysicists, we don't know what this material is that is so important to the physics of the universe. Since the early 1980s I have been modeling with computers how the dark matter influences the behavior of galaxies. Could we tell something about dark matter just by looking at galaxies and the way they're structured? Could there also be ways of looking for the dark matter directly, in the lab or at a telescope?
When I left America in 1989 and went back to Australia for a short period, I was involved in a project called MACHO, which was a search for dark matter in a particular form. Dark matter could be all sorts of things. It could be rocks. It could be dead stars. It could be planets. All these things, when you put them out in space, don't shine so they're potential dark matter objects. The MACHO project was a particular project to look for dark matter in this form of large macroscopic things, from the size of a planet or the Moon, to maybe something that's multiple times the size of the Sun. The way you do that is kind of interesting. If dark objects pass between you—the observer—and a distant field of stars, then occasionally the dark matter object will bend light due to its gravity as described by Einstein. That bending is called microlensing of light and for a brief period of time, it amplifies the brightness of the background star you can see.
You can ask yourself the question, what's the probability that you will see one of these lensing events? If the entire Milky Way dark matter halo was made of these dark objects, what's the probability that if you looked at a particular star, you'd see it go up and go down in brightness over some period, whether it's hours or minutes or days, due to microlensing? The answer is, it's about one in millions. In other words, you have to look at millions of stars before there's a chance of order one of seeing one of these events. The MACHO project was a joint effort between Mt. Stromlo Observatory in Australia, the University of California Berkeley and Lawrence Livermore Labs. We built a special telescope and a special camera that looked at a nearby galaxy to us, the Large Magellanic Clouds. With our MACHO camera we could monitors millions of stars in the LMC once a night, or once a minute, or once a year, and then measure its brightness, looking for signs of these microlensing variations.
You have to have a lot of patience and perseverance to do this project. We took data over a period of about ten years. We had data on about 20 million individual stars consisting of their individual brightness histories over time. We then searched all these histories, or light curves, and this is where data intensive astronomy comes in and were I first got "the bug" for doing astronomy this way. In a relatively short time we found an excellent candidate for a microlensing event. It was exactly the sort of variation we were expecting. We were all overjoyed and the discovery appeared on the front cover of Nature. We were all very happy! However, we did not find the number of events we were expecting. After the statistics were all done, assuming all the dark matter in the Milky Way was in the form of MACHOs, we would have expected hundreds of these events, and we found a few tens of them.
That's a bit of a disappointment, but disappointment like this often highly a different direction to take. In the world of dark matter research, a lot of people started thinking that maybe the dark matter isn't these big things; maybe it's small things. Maybe it's particles. There was then a big push to start a major search—and this is now 20+ years ago—for what they call WIMPs, weakly interacting massive particles. These particles are possibly about the mass of a proton. When they bang into atoms or crystals or cubes of ice, and they give off signals which you might be able to detect. So, people have been looking for WIMPs for 20 years or so involving many experiments around the world. Unfortunately, the results so far are "zero". That's also disappointing. It's kind of like the "searching under the lamp post" story. We had this lamp post which was the MACHOs. We had another lamp post which was the WIMPs. We've searched under those and no "keys" were found, so we have to continue finding more lamp posts and see what we can find.
Based on the MACHO and WIMP searches so far, maybe the next lamp post will be found if we keep following this line downward in dark matter "particle" mass. The particle which is next on this list is a thing called an axion. An axion is a very interesting particle. It's thought to have a rest mass energy between one millionth and one thousandth of an electron volt (the rest mass energy of a proton is around 1 billion electron volts). They are predicted to exist because of a particular problem in the Standard Model of Particle Physics which is an incredibly successful theory.
The original version of the Standard Model could not explain an effect called CP (charge and parity) violation. It was proposed that you could solve this if there was this particle called an axion. This axion is a direct prediction of a new Standard Model that solves the CP issue. So, there's a good physics reason for this particle called an axion to exist that is nothing to do with dark matter. When you look at the predicted properties of these axions and ask where they were made and how many of them are there, they turn out to also be ideal dark matter particles. You could in fact have all the dark matter in the universe made of axions. Terrific, that's wonderful, so let's go look for axions. The problem with that is of course they are weakly interacting, just like WIMPs, so it's very hard for them to interact with normal matter or give off any kind of radiation that we could see. You have to fool them, you have to trick them into decaying in some sense and giving off some radiation.
There are experiments around the world which have basically big electromagnetic cavities that are a few meters long, inside of which is a very strong magnetic field and a high vacuum. When an axion encounters a very strong magnetic field, there's a finite chance it will decay into a photon, a real photon you can see. The wavelength of those photons is in the millimeter wave range. If you've got a very sensitive microwave radio detector in this cavity, there's a chance you can find one of these axions decaying. There are axion dark matter search experiments going on at the University of Washington (ADMX is one of the very big ones) as well as in Europe, Australia and Korea. The problem with the searches so far is that these cavities can only detect one possible mass of an axion. They're tuned to one particular frequency. An axion could be anywhere from a millionth of an electron volt to a thousandth. You have to build lots of cavities, or you have to figure out how to tune the cavity. That search has been going on, and it's doing really well, but it's going quite slowly.
My thought was, maybe there's a chance for an axion signal from the sky, from the universe. An axion resonant cavity in the lab has got a very high magnetic field and a relatively small volume. The universe has got a very small magnetic field but a very large volume. It's the product of those two things that gives you the probability, in some sense, of the axion decaying. I was interested in looking at whether we could see axions buzzing around the Milky Way that would interact with the magnetic field of the Milky Way, or objects in the Milky Way that had magnetic fields, and give off signals that we could detect with radio telescopes. If the axion mass is about a millionth of an electron volt, the frequency comes out in the radio at hundreds of megahertz, so it's ideal for radio telescopes. If large radio telescopes like the SKA could point in the right direction and listen for long enough, they could potentially find some of these axion radio signals.
A student of mine and I went through all the math and the theory of predicting what the signal would be like and how strong it would be. It turns out to be very weak in typical radio astronomy terms. You'll need the SKA to have any chance whatsoever of detecting these radio emissions. It would be extremely pleasing if we did an experiment on the sky that showed you there was an axion, and in the lab you also found the same axion mass. It would be a beautiful verification that indeed this dark matter is axions and we've solved two problems at once - the CP problem and the dark matter problem. But, we may also not find axions, and where do we go from there is an interesting question.
ZIERLER: Peter, in all of this long research and searching for dark matter, have we winnowed the possibilities? In other words, is your focus on axions well-grounded in the idea that there's a lot of candidates that it certainly is not, and that's why axions are so promising?
QUINN: Yeah, exactly. I think, as I said, it's the lamp post story. WIMPs provide a lamp post. MACHOs provide a lamp post. Axions I think provide a different lamp post. We're just crossing them out one by one and that's all we can really do. We can theorize until forever about what possibly could be there, but unless we've got some way of actually shining a light and searching, it's very hard. I think if we get through the story and the axions themselves of the kind that solve the two problems are not there, we are then starting to get into more speculative territory about where the dark matter could be. Some people have thought about changing the laws of gravity to fix the dark matter problem. I personally am not an advocate of that. I think we should still keep searching for the lamp posts. Some people like the idea that there are very, very, very small particles, 10-20 electron volts, and these have interesting properties that may also be relevant to the dark matter problem and may have some manifestations that can even be observed. So there's still some territory out there to explore, but we're working our way through this parameter space and carving out very large pieces of it, I think.
ZIERLER: Of course the other big mystery here is dark energy. I wonder if that compounds the problem of dark matter—in other words, the mystery of dark energy makes understanding dark matter all the harder. Or do you see them separately?
QUINN: I think they are separate problems. Einstein had a term in his equations, which if we accept it as a given component of the universe, it works very well as a pressure-like term in the cosmological equations. What is causing it is another question, and I think it's a separate issue from the dark matter question. The dark matter question is fairly well posed and there's a lot of observational evidence for dark matter. From the way galaxies move and rotate, through gravitation lensing, through the cosmic microwave background, there's all sorts of good evidence for dark matter. I think we don't yet have as much data on the dark energy problem as we would like.
Now, just a few weeks ago DESI, the Dark Energy Survey Instrument from America, produced an extremely interesting result which said that there was potentially some evidence that dark energy is evolving as a function of cosmic time. That, we didn't know. Einstein's term does not vary with time so this new claim is extremely interesting. These are the sorts of things we need to understand to start getting some lamp posts for the dark energy, because we don't know it well enough. If we can figure out what it's doing to galaxies better, or how it's changing with time, then I think we can start progressing our knowledge on dark energy.
ZIERLER: The provocative notion of changing the laws of gravity, are you referring to the effort to merge quantum mechanics and general relativity, to quantize gravity, as it were?
QUINN: That may end up with some interesting results, but no, there has been a suggestion by several people that you can change Newton's laws in a very simple way. Instead of being one over r squared, it becomes one over r squared with another term, which changes the strength of gravity as a function of the size of the acceleration due to gravity. In other words, the weaker gravity gets, the more it doesn't behave like one over r squared. If you just make this change to the equations, you can fit some of the observational data on the way galaxies rotate quite well. So, it's just hypothesized as a modification to Newtonian gravity. Some people have tried to justify it by saying it is consistent with general relativity. I think the consensus is that it is not. Yes, you can mess with the gravity acceleration law but the motivation for that, at least for me, is not well posed. I'd rather go look for lamp posts and data and see how things play out —assuming Mr. Newton was right in the first place.
From Wollongong to Canberra
ZIERLER: Let's go back now and establish some personal history. Tell me about your undergraduate experience and if astrophysics and astronomy was always part of your agenda.
QUINN: As a kid, I was always interested in science. I thought science was just tremendous. When I say science, I mean test tubes, experiments and the like, that was science for me as a primary school kid. I had a couple of really good teachers in primary school and high school who fueled that scientific "spark". I was fortunate to grow up at a time in the 1960s and the early 1970s when science education was highly valued. I was a Sputnik-era child. I was 14 when Armstrong landed on the Moon. It was a time when science was at the forefront of some of the thinking of educators who determined what was taught in schools. We had very specific courses on physics and chemistry and biology, and that fueled in me a scientific passion. By the time I got to the end of high school, I was pretty convinced I wanted to go to university and do science, and in particular do physics. I did an undergraduate degree at the University of Wollongong. For those who don't know where Wollongong is, it's about 50 miles south of Sydney on the eastern coast of Australia and had good university with a strong physics department. I completed my undergraduate degree in physics and mathematics in 1977. I had convinced myself that physics was all about subatomic particles at that time, and I was going to go off and do a PhD in Stanford or somewhere similar.
At the time that I was doing the fourth year of my undergraduate work, there were a couple of astronomy postdoctoral fellows in the Physics Department at the university. They convinced me that Australia, as a country, had always done really well in astronomy. That's perhaps something to do with the great view of the universe we have in the Southern Hemisphere. They also instilled in me a sense of how much undiscovered "land" there was in astronomy. Physics as a science had hundreds of people working on individual big accelerators projects, whereas in astronomy there were still some fundamental problems, like dark matter, where individuals and relatively modest amounts of equipment could make a big difference to our understanding. That really excited me. As well as being interested in the physics, the opportunity to contribute in a significant way was also very exciting.
I started a PhD at the Australian National University in Canberra in 1978. Mount Stromlo Observatory, the Research School of Astronomy and Astrophysics at ANU, is where I was based for four years. I did a PhD in Astronomy under the supervision of Professor Ken Freeman, one of the founding fathers of dark matter astronomy. Ken was one of the main people that really brought to our attention what dark matter does to a galaxy like the Milky Way. I developed a PhD topic on colliding galaxies that would use of a lot of computer simulations. When I was an undergraduate, I also developed a passion for computers. There was nothing quite so satisfying as writing a program, getting it to running and having it work something out for you. It was just a tremendous "buzz"!
I wanted to study what happens when galaxies collide. At that time, there was a very strong belief that when galaxies collide, they can stick together and form a new galaxy, i.e. galaxies can merge. We thought that was potentially one of the ways that galaxies evolve. We know the universe is full of elliptical galaxies and spiral galaxies, and maybe when the spiral galaxies collide, they form ellipticals. So, there was perhaps an evolutionary story here that connected the two types of galaxies together, and maybe collisions were an important part of that story. At that time, there hadn't been any self-consistent, nonlinear modelling of galaxies merging. I was one of the first people who looked in detail at models of merging galaxies—and found some interesting results and I was very happy with it.
ZIERLER: What were the results?
QUINN: So galaxies do stick together, which is of course nice. And, the big piles of stars that are left after the collision look very much like elliptical galaxies—so, tick, there's a consistent story. But what came out of this was something unexpected. When you collide galaxies together sometimes you can form, around the big pile of stars, extended structures (made of stars) that are very, very thin and produce very sharp edges when you look at them in projection on the sky. They look almost like eggshells surrounding the galaxy. At the same time as I saw these "shells" in the models, some observational astronomers who were taking photographs of galaxies, at the Anglo-Australian Observatories, also saw some of these very sharp and very faint features around the galaxies. They originally said, "These obviously are artifacts of our photographic techniques." because when you look at the sky, you don't very often see razor-sharp things like these shells. Just by happenstance we crossed paths, and I said, this is great, because I think they could be the evidence that the galaxy in the middle was formed through some type of collision. I finished my thesis in 1982 on several aspects of colliding galaxies include the results on shells. This caused a lot of interest in the astronomy community because it was hard evidence that galaxies did have a history of mergers.
Then I asked "Where am I going from here?". I knew I needed to apply for a postdoc but where? The Director of the Observatory at the time, Prof. Alex Rodgers said go for "the best places in the world .. Princeton and Caltech and Cambridge". I was fortunate to receive offers from both Princeton and Caltech and I was told, "Under pain of death, you should go to Caltech." [laughs] There were several strong connections between some of the staff at Mount Stromlo and Caltech, and the work being done at Mount Wilson and Palomar. So, I packed my bags and headed to Caltech.
Computers and Galactic Mergers
ZIERLER: I want to ask one question before we move on to your time at Caltech. Your work on disk galaxy mergers, is it specific to disk galaxies? In other words, are there other kinds of galaxies that merge in a similar way?
QUINN: Yes, that was the focus of work I subsequently developed in the following years. Galaxies often have smaller satellites galaxies that orbit them. The Milky Way has two satellites, the Large and the Small Magellanic Clouds. These satellite galaxies that orbit big galaxies also eventually spiral in and merge with the big galaxy and have all sorts of consequences for the big galaxies. For example, the Milky Way is a disk of stars and gas, but it also has a pile of stars in the middle called a bulge. Where did the pile of stars in the middle come from? We think one of the strong contributors is smaller galaxies which spiral in from the outside and end up in the middle. On their way down, they shred themselves and leave debris all through the halo of the Milky Way. Today this is a big industry with people looking for and finding all these streams of stars that are in the Milky Way halo and are evidence of a merger of a satellite galaxy with the Milky Way. So, not only is the big-galaxy/big-galaxy-colliding story important, but also little-galaxy/big-galaxy-collisions and mergers play an important role in the galaxy evolution story.
ZIERLER: I know this is going to sound like a long time ago, but exactly how were computers useful for this research?
QUINN: You need to make a realistic model of a galaxy like the Milky Way to study how it might evolve by itself in isolation as well as in a merger of two galaxes. You need to somehow represent hundreds of millions of stars which are moving around under the force of Mr. Newton's one-over-r-squared gravity. So you put down particles to represent the stars, hopefully lots and lots of particles (at that time only thousands were possible) and tell them all that they have to move according to the forces of gravity generated by each "star"—in other words, a self-gravitating model, so every single particle exerts a force on every other particle. Using Newton's laws of motion and using a numerical integration method to solve the equations, you can figure out how each particle moves forward in time. It must satisfy some criteria, but it mimics the behavior of a real galaxy using a computer. Then you make two galaxies and you let the computer follow what happens as the two fall together, as the potential changes with time in a very non-liner way and finally comes to some stable and static result (the merged pile of stars in the middle).
It's the evolution of nonequilibrium-to-equilibrium dynamical systems (galaxy encounters and mergers) that computers are very good at. We had a modest computer in the basement of the observatory at Mount Stromlo but it was good enough to do some of these models. Over time, computers have become more and more performant, and of course you can now do models of the whole universe with galaxies of the same size (particle number) as I used as the individual merging galaxies.
ZIERLER: Your advisor who suggested that you look at Caltech, was there a particular professor he had in mind for you to work with?
QUINN: I don't think so. I think it was recognized that Caltech was obviously a center of excellence for many things, in particular astronomy through Palomar and the Astronomy Department and Mount Wilson, but also theoretical astrophysics through what was happening with Kip Thorne and Roger Blandford. For an astrophysicist and an astronomer of my sort who was sitting on the fence a little bit between the theoretical/computational world and the observational world, it was an ideal choice.
Between Theory and Experiment at Caltech
ZIERLER: What were your impressions when you first arrived in Pasadena?
QUINN: Scared. [laughs] It was very intimidating. I often tell this story now to graduate students of mine. When you grow up in a particular academic environment, like Australia for example, the way students are educated is by instruction of course, but also by giving them their head, letting them go, and maybe guiding them along. Some need more guidance than others, but you guide them along the path. It's normally a very nurturing environment and experience. Caltech was—different. Caltech was more of an environment where knowledge was won, by combat, almost. You went into debates—I'm not going to call them arguments; constructive dialogue I think is the right word—with your colleagues, all the time. I think when I first arrived, the very first day, somebody asked me the question you asked—"What have you been doing?" I explained about galaxy collisions and shells. The very first reaction was, "Doesn't sound right!" From that point forward, it was a conversation about why I was right, and all the assumptions I had made, rightly or wrongly. It's learning by dissection, argument and reassembling. It's not a skill you necessarily learn as a PhD student outside of America, and it was something I had to learn.
Once I got over the fear and the shock, it was incredibly rewarding because it taught me to be self-critical in more ways than I had been, but also to be able to speak and have a dialogue with everybody around on multiply subjects. If you don't understand something, you ask. You go to the roots of the problem and work your way up. So, the time at Caltech was very, very formative for me, because I learned a better way of being a scientist. All of my scientific interests I think grew out of my time at Caltech. There was a group of postdocs there at that time who were an incredible bunch of individuals, doing all sorts of different things (none of them working on galaxies like I was). For whatever reasons, we got along as a group incredibly well, talking "science" with each other, and earning respect from each other.
Through those interactions, new interests grew. Interest in satellite galaxies grew out of conversations with Jeremy Goodman, who was basically a mathematician. It was a transformative time for me, as I said, in terms of the way I regarded the way science is done, and how to do good science, and paths into new problems. I was in Bridge Annex, which was the Theoretical Astrophysics Group. That was a little group that sat between physics and astronomy. I was in exactly the right place because you'd find people flying through the room from the physics side and people from the astronomy side. In fact, I was very fortunate—in my office was one of Richard Feynman's students, so he would come and visit his student and walk past my desk maybe once a week. Occasionally I'd pluck up the courage to say, "Hi."
ZIERLER: [laughs]
QUINN: Peter Goldreich and Kip Thorne were my two formal bosses, but the postdoctoral family that was there at the time—Craig Hogan, Wojciech Zurek, Jim Applegate, John Hawley, Bram Achterberg, Ramesh Narayan and Jeremy Goodman became leaders in their own right in various ways—we just happened to be, as I said, an eclectic mix of people who got along very well.
ZIERLER: As you say, operating in both worlds—on the experimental side, on the theoretical side—did that really work well as far as Caltech's research culture was concerned?
QUINN: I think so. In the group that I was in, in particular—in the Theoretical Astrophysics Group—there was a lot of interest in observational research and what the data was telling them. Gravitational lenses are a good example. Strange arc-like structures were seen in images of clusters of galaxies taken at Palomar and elsewhere. When it was realized these could be gravitational lensing, the Theoretical Astrophysics Group was one of the first groups to write down the theory on gravitational lenses, because we could see the data, and we had the experience in the theory of gravity to provide the explanation. It was an extremely good thing to have that group in the middle that did connect the physics and the astronomy in a very natural way. One story I'll tell you, which is sort of funny—when Feynman walked by one day, I showed him a picture of a galaxy with the shells around it, and he said, "Nobody shows me something interesting anymore," and he walked off. A good example of bridge building!
ZIERLER: [laughs]
QUINN: Clearly he was interested in the data and interested in the galaxies. That's where we were. We were in the middle. We were working with and talking to the astronomers in Robinson and Downs, and all the other places where there were observational astronomers on campus. I think we were doing a good job of listening to what was being discovered and trying to think about ways of solving the problems.
ZIERLER: Were you able to take advantage of Caltech's observational capabilities?
QUINN: Not at the time. I was really deeply into the computational world. Even though I went to Mount Stromlo, I wasn't trained as an observer. I knew what a telescope was and which end to look in, but [laughs] that's about it. It was something that grew over time. When I left Caltech, various opportunities I started to develop a little bit more expertise in some of these areas.
ZIERLER: Tell me about meeting Lars Hernquist and the nature of your collaborations.
QUINN: Lars was a graduate student, so he was doing work for his PhD. He was under Roger Blandford, who was one of the faculty members in the Theoretical Astrophysics Group. Lars was working on mathematical modeling of neutron stars. These are objects which are left after the death of a big star. Lars was working on the mathematics of the way these neutron stars radiate. It was work that I could never do. Lars shared the desk across from me. Occasionally he would ask me what I'm doing, and I'd say, "Well, I'm working on galaxies, and collisions of galaxies, and these funny dynamical features and what it means for galaxy evolution."
I think he got the bug and decided that while the neutron stars were interesting, maybe they are not a long-term interest. We worked a little bit together towards the end of his graduate time on doing some more detailed models of the shell galaxy production. What are these shells—how do you describe them mathematically, dynamically and physically? We did some very tailor-made modeling to understand the shells in much more detail than I understood before. Hernquist and Quinn produced two or three papers, which were then well cited, because they really laid out clearly the physics of shells and the collisions. We discovered that one of the interesting things about shell galaxies is you could also use them to tell you something. In particular one of the things that fascinated me was that the spacings of the shells actually tell you about the shape of the potential of the galaxy. That potential of course is mostly dark matter, when you get out to where the shells are. So, you had a handle on the shape of the dark matter from the shells. We didn't really appreciate that until Lars and I started to work it out in detail. We had another handle on dark matter thanks to the shells, which was great.
Early Perspective on LIGO
ZIERLER: What was your funding when you were at Caltech?
QUINN: I was a postdoctoral fellowship jointly funded with a grant from Kip Thorne and Peter Goldreich. It wasn't one of the named fellowships. It was a hybrid fellowship from Peter and Kip, which was fantastic.
ZIERLER: What was the value of having access to those giants in their fields?
QUINN: Amazing. One of the nice things about the Theoretical Astrophysics Group, which I've tried to build in every single institute I've ever been involved in, is the so-called "interaction room". There was a room in the middle of the offices where there was a continuously percolating coffee pot and an old couch and a couple of big blackboards. Any time of the day, you would always find people in there talking. Quite often, Kip and many of his colleagues and students working on gravity waves were in there talking, and I would just go and sit and listen as they discussed their aspirations to build a gravity wave detector to detect these things. It was truly impressive. We had Stephen Hawking there one day. Unfortunately, at the time, he couldn't speak very well at all. He had a young graduate student who could interpret in various ways for him. In terms of meetings with transformational people, the Bridge Annex interaction room certainly the place to be.
ZIERLER: You got the sense that it was very early days but that this was the origins of LIGO? People were talking about what would become LIGO?
QUINN: Absolutely. There was laboratory work going on with isolation of masses and various other things. Ron Drever and the guys were there, and Kip Thorne of course with his students and postdocs. They were talking about how you would build an interferometric gravity wave detector as well as what you would actually detect. There was a lot of very good people coming through there predicting the waveforms that would result if two neutron stars collide or two black holes collide. What would you look for with a gravity wave telescope? – the waveforms, duration, and things like that.
ZIERLER: I think he would have been provost at the time, but do you recall ever having any interaction with Robbie Vogt?
QUINN: He was, I think, provost, and no, I didn't, at the time. He was way in the clouds above where I was in Bridge Annex.
ZIERLER: When it was time to move on from Caltech, did you specifically want to stay in the United States? Were you looking for either a faculty or another postdoc opportunity in the States?
QUINN: Yes, very much so. I was really buoyed up, enthused, by what I had done at Caltech. One of the postdoctoral people at Caltech was a guy called Wojciech Zurek who left Caltech as well and went to Los Alamos Labs. He was encouraging me to come and spend some time in the Theoretical Astrophysics Group at Los Alamos, in particular because they had access to very large computers. We were going to do astrophysics on some of these very large computers and migrate from single-galaxy modeling to cosmological modeling. We were doing some of the very first cosmological modeling using some of those resources. I worked with Wojciech, and so I wanted to stay in the States, so I was looking for postdoctoral opportunities to do that.
I was also fascinated by what was happening with space astronomy and astronomy instrumentation in general. I ended up taking a postdoctoral appointment at the Space Telescope Science Institute in Baltimore, Maryland, where I was in the academic affairs department. It was a purely academic position—I could do my own research—but I was there to again sit on the fence a bit, between the theoretical and computational world, and see what was happening about planning for the Hubble Space Telescope. What are the telescope targets and what is the science the telescope is going to do? That was also a fantastic time to meet people. At that time, before Hubble was launched, the Space Telescope Science Institute was like the hub of the world. Everybody wanted to visit. Everybody wanted to come and talk. You didn't have to travel anywhere; you'd just sit in your office and all these people would walk by your door. Allan Sandage was a frequent visitor. At the same time, Lars had taken up a postdoctoral position at Princeton University, so we were both on the same coast, and we did a lot of work together at the Pittsburgh Superconducting Center to do some of the first detailed work on galaxy satellite collisions.
So, I was keen to stay in the States to continue my work with Wojciech, continue my work with Lars and to be part of the Hubble excitement. That had other consequences as well. One of the things I didn't tell you about Caltech being a transformational experience is I found my wife in Los Angeles. We got married before I left Caltech and then we went to Baltimore. Our daughter was born in Baltimore and now lives in San Francisco. So, the bonding to the U.S. was quite well and truly affirmed. However, as an Australian, there was a limit to how much time I could spend in America on the kinds of visa that I had. At the end of that Space Telescope period, I would probably have to return to Australia.
ZIERLER: What was it like on launch day for Hubble?
QUINN: I wasn't actually there on launch day. I left very briefly before that time. I was there when all the proposals were submitted, the proposal submission day. There were FedEx trucks backed up for miles and miles and miles. The excitement was fantastic, absolutely fantastic. Then just seeing the potential of this telescope—it was an extremely exciting time.
ZIERLER: Do you have any unique perspective on the discovery of the mirror flaw in the telescope?
QUINN: That was discovered after I left, but I knew a lot of the people who were working on the solution. There were some extremely talented people at the Institute. Again it's similar to an ESO-like organization. It had the people who were great astronomers, great technicians, great computational and instrumentation people. I was not surprised that they came up with an elegant solution quickly. If anybody could have done it, they were the people to do it. I had a lot of confidence in those people that they would find a solution.
Early Days of Supercomputing for Astrophysics
ZIERLER: Between your work at Los Alamos and the Pittsburgh Supercomputing Center, what was the state of the art in 1980s in supercomputation? What did it allow you to do more efficiently and what did it allow you to do, period? What did it simply make possible?
QUINN: It was a huge step forward in terms of the numerical resolution we could achieve. When I was doing models in my thesis work, the galaxies I was dealing with had maybe a few thousand particles in them. That was about as much as the computers of that day could really tolerate. When we went to the supercomputers, you were now talking about hundreds of thousands of particles, so a hundred times more resolution in terms of what you could resolve in the dynamics and the physics. The supercomputers of the 1980s and 1990s that were at Pittsburgh and Los Alamos—the very early Cray computers, the Cray-1, Cray-2, Cray X-MP—were very good at doing hundreds of thousands if not millions of particles. That opened immediately the possibility of modelling a small section of the cosmos itself in which you might find 50 galaxies or so. You had enough particles to individually resolve the mechanics and dynamics of those galaxies, but you were doing it in a cosmological volume. That was extremely exciting, because we had the chance then—this is in the 1980s and 1990s—to do cosmology modeling in a nonlinear sense, to actually see gravity do the work. We could then go from a linear universe of very small density fluctuations at high redshifts to these very highly nonlinear structures like the galaxies we see around us today. I think the ability to go from individual galaxies to cosmology is what happened at the time that I was in the U.S.
ZIERLER: Angular momentum is one of the great mysteries. It's such a fascinating topic. I wonder if you could explain your work in angular momentum and how it relates to galactic accretion.
QUINN: Angular momentum is a wonderful thing. It's interesting to consider how it affects the dynamics of galaxies colliding. Suppose we have a collision which involves a disk of stars like the Milky Way, and another little galaxy which is buzzing around that galaxy. If this satellite galaxy is going in the same direction as the stars are moving, they then share the same angular momentum vector direction. The relative velocity is very small of stars in the galaxy and the satellite is then small. In other words, if you and I are going in the same direction, the difference in velocity between you and me is quite small. That means that effectively the force that the little satellite exerts on the stars in the disk acts over a longer period of time, because it's going slowly as far as the stars are concerned. You have a bigger force, a bigger consequence, a bigger tidal force exerted if everybody is going in the same direction.
If the opposite is true—if the little satellite is going in the opposite direction, its angular momentum vector is opposite, then the relative velocity of the stars in the galaxy and the small satellite is very high. It turns out that the consequences of this, the forces that act, go like that, one over the relative velocity squared. If the velocity difference is small, you get a very big force. If the velocity difference is very large, one over a large number squared is very small. This is the angular momentum dependent accretion, the Quinn-Goodman effect (developed at Caltech with Jeremy Goodman) that galaxy accretion/merging processes do know about the sense of motion of the various components, and that's why angular momentum is important.
ZIERLER: Does this get us closer to the fundamental question of where the momentum originates?
QUINN: That's a good question. We also can ask questions about angular momentum in the cosmology models as well. Take the cosmic volume today, run it backwards for some time, look at the places where galaxies we know are going to form, and ask how much angular momentum in those places. Protogalaxies are not spherical, they've got lumps and bumps—and when you've got two objects with lumps and bumps, they tend to torque each other, i.e. provide angular momentum to each other. That process was enough, in the early universe, to give these big dark matter halos that form around galaxies enough angular momentum content to make the high angular momentum galaxies we see inside today. I think the tidal torquing story of where the angular momentum comes from is a consistent and approximately correct story.
The more interesting question is, if you have this dark matter halo which has got a certain amount of angular momentum now in it, how does it get transferred into the stars we actually see? How did that all happen? The simple picture is that the gas was originally all spread through the halo and had the same kind of angular momentum per unit mass as the halo did. Gas can do something that dark matter can't. Gas can lose energy. It can radiate. So, the gas shrinks. As it shrinks, it conserves its angular momentum, it starts to spin up, and spins like the galaxies we see today. There's another consistent story here about the angular momentum of stars, and the angular momentum of dark matter, in both the nonlinear universe and linear universe. All that came out through the modeling advancements in the 1980s and 1990s.
MACHO and Return to Australia
ZIERLER: Did you want to specifically come back to Australia? Did you want to go home, as it were, for a faculty appointment?
QUINN: I think it was, to be honest with you, more personal reasons than science reasons. Both my wife's and my families were getting quite old, and we felt like we'd like to spend some time back in Australia. Our sojourn to Australia after the Space Telescope was motivated a bit by that. But it turned out, very shortly after I arrived back in Mount Stromlo, that this opportunity arose for the dark matter MACHO project. That also set me on a new course of dark matter work, which was sitting on the fence between optical astronomy, dark matter theory, gravitational microlensing theory, and the modeling. It was also an important time becaus it really instilled in me how powerful it is when you combine the right instrument with the right detector, the right computers and the right software to solve a physics problem. MACHO put all those parts together to solve a physics problem. It wasn't just any old telescope with any old camera, with any old software; it was a purpose-built, bespoke system to solve the dark matter problem. Being able to make bespoke systems consisting of computers, hardware, theory, models, software, whatever, to solve a particular physics problem, is a very important modern paradigm. MACHO proved the success of that paradigm.
An interesting coincidence in some sense here is that when I was at Space Telescope, my boss was Riccardo Giacconi, the director of the Space Telescope Science Institute. Riccardo eventually moved from Space Telescope to ESO where he was the Director General. I remember from conversations, that Riccardo also liked this paradigm of being able to connect data, telescopes, software, people, theory, into something to solve a problem. I think he saw Hubble as not just a telescope in orbit but part of a bigger system to solve a problem. So, we did MACHO, we were successful, and then one morning the phone rings and it's Riccardo. He says, "Peter, would you like to come to Europe, to Munich? I want to build one of these end-to-end science-solving machines and I'd like you to help me." That was an interesting opportunity.
ZIERLER: To clarify, when you got to ANU, was the MACHO project already up and running, or you went to ANU to launch it?
QUINN: MACHO was launched after I arrived a Stromlo following discussions with Ken Freeman. Ken was aware of the work of Bohdan Paczyński, who pointed out that microlensing might be a way of detecting dark matter. Ken and I said, "Maybe we could actually build something that would do a Paczyński experiment". We got talking with Alex Rodgers, the director of Stromlo, and he had connections to Berkeley and to Livermore Labs, who were also interested in potentially building something like this. I ended up running the Australian leg of the team, so I was responsible for basically all the things that happened on Stromlo, which was the telescope and the software systems, observing and some of the analysis. We started the project, built it, ran it, got the results. Big experience. A really important experience in my career. Riccardo I guess saw that as well.
ZIERLER: There's a nuance here. You make the cover of Nature. It's a very big deal. This is the first microlensing evidence of baryonic dark matter. How then do we not get from this discovery to understanding what dark matter is?
QUINN: I'll say it in a blunt way—MACHO was a failure, basically [laughs] in the sense that we didn't find the dark matter. We found some dark things whose statistics tell us it cannot be all of the dark matter. It's an absolutely valid piece of dark matter, for sure, but not all of it. If you've only got ten percent, there's something else out there, not just this MACHO stuff. MACHO was an experiment that had to be done. We proved we could find these things. If the halo was indeed 100 percent MACHO, then we would have found it. We didn't. We found ten percent. That tells us that we had to go find the other lamp posts. MACHO also told us a lot about other things (variable stars for example) and how to do science with these bespoke end-to-end systems.
ZIERLER: If MACHO had been more successful, do you think you would have been less likely to join the ESO?
QUINN: It's a good question. I think probably no. I think I would have been looking for how to build the next big MACHO experiment, maybe working in Andromeda galaxy. We knew the technique worked so let's push it to its limits. I wouldn't have been happy just sitting on what we had done. I would have pushed it.
ZIERLER: Did you take a leave of absence of ANU, or did you resign your position to go to Munich?
QUINN: I resigned my position. My position at Stromlo was not a faculty position; it was a five-year fellowship. ESO was a long-term faculty position so it was a very attractive offer. But it meant uprooting a young family to the other side of the world, and potentially taking a big risk. I think—again this is a story I like telling graduate students—that you occasionally have to take right-angle turns in your career. I'm going to be really honest—there were people at Stromlo at the time that said that I was mad. "Why would you give up an astronomy research position to go off and help this crazy Italian guy build telescopes? You're not going to be able to do your research work anymore; why should you go?" There was this in one ear. In the other ear was, "I will learn things I would never have learned if I stayed in Australia. I will do things I would never do if I stayed here."
ESO was a very big challenge and a very big opportunity. In the end of the day, the opportunity was too big to pass up. Yes, it did mean I did not do as much research. I was not a pure researcher anymore by any stretch of the imagination. But why I tell graduate students about this is because doing research in this century quite often involves being involved with big projects of various kinds, like LIGO or the SKA. When you're involved with big projects, you have to learn skills, very different skills. Not just your astronomy skills; you must learn how to deal with people, talk to people, manage people, budgets, planning, contractors, industries and governments. You need to learn a new toolkit that you should carry in your scientist bag, because doing science needs these things as much as it does calculus. I rationalize my decision—not that I regret it in any way—but I had the opportunity to learn an enormous set of skills I would never have learned if I stayed where I was.
The Big Data Challenge in Astronomy
ZIERLER: In joining the VLT, what were the big challenges in data management?
QUINN: The VLT was designed to break the paradigm of ground-based astronomy. Traditionally, as astronomers, you apply for telescope time on a telescope somewhere, like Palomar. You put a science case in, some panel of experts looks at your science case and says, "Yes, you're good. You can have three nights, April the 1st, 2nd, and 3rd, next year." So, you walk up to the telescope April 1st, 2nd, and 3rd next year—and it's cloudy. Astronomy traditionally had been a science where you'd take chances. You're at the mercy of the weather and the mercy of the instrument working correctly. At the mercy of trying to figure out how to analyze the data and how you calibrate the data. Riccardo was very keen to change that paradigm. We didn't want astronomers going through that traditional process anymore where you would apply for time, get some nights, go to a mountaintop somewhere or other, and sit there and look at the clouds.
What we wanted to do was to basically go through the scientific adjudication process—"you're an astronomer, great project, should be done"—and maybe we give you a hundred hours. Then we get you to describe what you want done in each one of those hours. Then you give the responsibility to the observatory, to the people in the observatory, to execute each one of those hours at exactly the right time to give you the best result. You take out the weather from the equation. You don't have to have the weather be a problem anymore. If you write a good science proposal and you say you want a hundred hours of clear nights with no Moon, you will get a hundred hours of clear nights and no Moon, independent of whether the sky is cloudy or not on any date. We'll just give it to you whenever it occurs. So, it was optimizing the use of the telescope for weather.
The other thing we did was we would not let you touch the instrument. You obviously weren't going to the telescope anymore so you didn't have to travel all around the world, but also you couldn't touch anything, because the instruments were designed, built, operated, and calibrated for you by the observatory. The observatory maintained the configuration control. You weren't allowed to go in there and turn a screw and change something. You were guaranteed that the data that you got was the right data, that it could be calibrated, and it was calibrated for you. The observatory took on the responsibility for quality assurance and quality control of the data. In other words, the data is the right data that you asked for.
This was initially "hated", by astronomers. Astronomers will tell you—my colleagues at Stromlo and elsewhere in the world—that astronomy is all about going to mountaintops and having a good time and turning knobs on instruments and trying things out. We were implementing a very different way of doing astronomy. Some people said it was going to limit creativity, and how could you possibly know how to observe this object when I'm the world's expert on this object? In the end of the day, it turned out that more than 80 percent of the data that people wanted could be done in exactly this way. Maybe you needed 20 percent for weird objects where you didn't know what was going to happen and people did have to go to the telescope, but 80 percent of the time you could do it in this more scheduled, programmed, queue-observing kind of mode, as they call it, where you can guarantee the quality of what's delivered.
Hubble is exactly like this. As an astronomer, you don't go and visit Hubble and turn screws of course. It's sitting up there in orbit—you specify the data you want, the commands go up to orbit, somebody examines the data, processes and quality checks the data and sends you back a table, link or a CD or whatever it happens to be. That model is easy to do in space because there's no weather in space and nothing changes much in space. On the ground you have weather and things can change or even break. So, with the VLT we were moving the space paradigm to the ground paradigm and changing the way astronomy was done.
Dealing with the data flow—from defining the data you wanted to take, scheduling at the right time, perfecting the calibration, processing the data with the calibration, putting it into an archive where people can find it again and do something else with it—all that chain of data was very new to astronomy on the ground when I walked in the door at ESO. Riccardo's vision was, let's do it like space. Let's make VLT a very efficient telescope. VLT has proven, time and time again that it's probably the most efficient telescope in the world. Its efficiency is more than 80 percent in terms of how much time it spends taking data from the sky. The VLT has produced an archive of data which is all calibrated and quality controlled. There's four times more data coming out of that archive than going in, because people are taking it away and doing more science with it, because they know what it is. It's a guaranteed quality controlled product. This amplification of science - improvement of efficiency, changing the way astronomy is done—was my job description, day one.
ZIERLER: The legacy of VLT is really incredible in that regard, that it was set up to break this paradigm, and in fact it did.
QUINN: It did.
ZIERLER: What's the benefit to astronomy?
QUINN: You'll find now that pretty much all the major telescopes in the world are doing exactly the same thing. The Gemini project, the TMTs and the ELTs of this world, the SKAs, the NRAOs, ALMA—many telescopes around the world have been bitten by this bug to some extent. They now do exactly this end-to-end process that we did. It did have a big effect. People saw the benefit. There was this one observational astronomer who was working, in fact, on gravitational arcs in clusters, and sources embedded in the gravitational arcs. He needed 100 hours of 0.1 arcsecond seeing. To get that naturally from a really good site like Paranal in Chile, it would have taken you probably five years, maybe ten, if just went on random nights throughout the year. He got it in one year, because of the way we scheduled the high-priority science. So, it is a way of doing good science well, and it does suit the way science is done for a large number of the science cases.
Virtual Observatories and Citizen Science
ZIERLER: Peter, the concept of a virtual observatory, does this come directly out of this paradigm-shifting effort?
QUINN: It very much did. I went to ESO in 1995, and we had the first light of the first VLT unit telescope in 1998. By 2002, all four VLT unit telescopes were working, data was flowing, and we were building a data archive. I think because of this paradigm, because we were filling up the archive with really well understood data, well described data, at least that opened up the opportunity for there to be interoperable data. In other words, this data could talk to potentially other data because you could describe this data really well. If we could describe the other data very well, we could connect the dots. The ESO archive has optical and infrared data—if we had an X-ray archive or a radio archive, and the data in those archives had some sort of translation layer or interface that could talk to ESO data, we could start doing multi-wavelength astronomy and multi-messenger astronomy at some level.
Around 2002, I had a long conversation with several colleagues around the world. This included Alex Szalay, who is here at Johns Hopkins, Bob Hanisch, who was at the Space Telescope Science Institute at the time and Andy Lawrence at the University of Edinburgh. We decided to form an alliance of similar minds to say, "Is it possible to join data and make data interoperable on a global scale for astronomy?" This is just astronomy data we're talking about. What are the things astronomers want? What sort of data do they have? Is it possible to make it interoperable? So, we formed the International Virtual Observatory Alliance that now extended to astronomers in more than 15 countries. We decided to make working groups to start thinking about this problem.
We modeled ourselves on W3C - the Worldwide Web Consortium. That was a way of making sure the web continues to work. In other words, the web that we all love works because there are certain standards in place. HTML, URLs—these are all things that didn't happen by magic. They happened because somebody invented them and maintained them as a standard for the internet. The internet works because there are these standards that W3C maintains. So, we decided our business was going to be about standards. In other words, what's the standard for publishing (making available to everyone) astronomy data? I want to publish a piece of spectroscopy or a piece of image data. It doesn't matter whether I'm an optical telescope or a radio telescope. I've got an image, or I've got a table of numbers, or I've got a spectrum; what is a standard that would describe a spectrum, an image, that would allow my image to talk to an image from somebody else's archive? These are called data access layers, DALs. They're basically standards for the way you, the data holder, put a certain sort of skin over your data, which allows other people to see it, and you can publish it into this Virtual Observatory.
The Virtual Observatory became all the interconnected archives that adhered to a set of standards. Just like all the websites in the world that adhere to HTML standards can talk to each other through the web, all the archives in the world that adhere to the data access layer standards for spectra, images, tables, could talk to each other. Over the last 15 years or so we have put in place IVOA standards for access to images, spectra, time series and alert events. IVOA exists today as a standards organization like W3C through the good will of the international community. If you are building a new archive these days in astronomy, you would certainly want your collection to be visible through IVOA standards to the rest of the world. IVOA gives you that window to expose your content to other people's content, and for you to see their content.
ZIERLER: What were some of the motivations, what were some of the pleasures in making astronomy more accessible, given the fact that, with the rise of astroinformatics, databases, it's out there for everybody to contribute and to learn from?
QUINN: I think for me it was the connection that was made possible between different wavelengths and different parts of the spectrum. One of the things we've learned from having different kinds of telescopes, and telescopes in space and telescopes on the ground—stars and galaxies and the universe as a whole has a very complicated spectral energy distribution. It's not just a black-body curve. There are lumps and bumps and lines. It's telling us about the objects which are contributing the energy or absorbing the energy from the universe around us. If you want to study this process of evolution of stars, of galaxies, of the universe, you need a range of wavelength data. You have to be able to connect the wavelengths together. For me it was really pleasing to see some of the very first papers that came out that joined together all of this data. Simon Driver and his group who are based at ICRAR, joined together many of these different spectral regions, both space and ground, into one coherent picture of the energy budget of the universe. That was only possible because of IVOA standards. You couldn't have talked to the data had you not had those standards.
ZIERLER: I asked about equity for observation time. In what ways does the creation of a virtual observatory democratize the data? If it doesn't democratize the observing time itself, how does it make astronomy more democratic, more of a community worldwide effort?
QUINN: It does, very much so. The Riccardo vision, the end-to-end story, ended in an archive. You fill up an archive with data that you really trust and know. The idea was always that you would expose this archive of data to the world. In other words, when a person is given telescope time, usually they have the right to that data for a limited period for their own use. In other words, there may be 12 months or 18 months, as a proprietary period, where their team and themselves own sole access to that. After those periods expire, then that data should become public property. Public money paid for it in the first place, so it's public property in some sense. If I'm an astronomer the idea was, "I'd like to find all the blue galaxies in the Southern Sky, " I've got to know where all the data on blue galaxies resides. When they took the data, did they do a good job? Did they calibrate it properly? What were the restrictions of their instruments?
Creating archives is all about creating content for a question exactly like that. I could be an astronomer in a small country in Africa and I could still access these archives internationally and make queries on its content. One of the queries the Virtual Observatory created and supported was called a cone search. A cone search basically says, "Give me everything you know about that particular piece of the sky." You're carving out a little cone in that particular part of the sky; "Give me everything. Tell me what you know." It'll tell you all the stars, all the galaxies, all the different wavelengths that have been observed, the time domain data if there's any, et cetera, et cetera. In other words, it's a very democratizing thing because you can ask some pretty fundamental questions. It can find asteroids, find quasars, whatever it happens to be. It's a very democratizing thing, because it does give everybody access.
And it multiples the return. All of these peak facilities like VLT cost a huge amount of public money, billions of dollars. You should do everything you possibly can to multiply, to enhance the return on that investment. These archives are probably the most straightforward way of doing it because you give the chance for that data to be used multiple times for multiple projects. As I said, the average these days is four times the data going out than going in. In some archives, it's much more than that. The Sloan archive is an ideal example. The Sloan project here in the United States I think has produced more than 20,000 research papers. It was one archive. It's the value and the merit of having a well-defined archive, with well understood content, exposed in the right way to the rest of the world.
ZIERLER: Peter, tell me about the Premier's Fellowship, and if that's what pulled you back to Australia.
QUINN: I was at ESO in 2005. The VLT is operational and has been doing great. ALMA is well advanced in planning and construction. ELT is still the dream down the road. I had been obviously well and truly bitten by the bug of the big machine picture of doing science. I discovered that Australia was being considered for the SKA project, the SKA radio telescope. You don't need high mountains, and Australia doesn't have any, but what you do need is a lot of isolation and quiet, radio quietness, and Australia has huge amounts of that. So, there was a real chance that Australia could be the home of the SKA project. I was really keen to be part of that, to be part of another large, international effort, particularly because it was going to be in Australia, a chance after 27 years of around-around, to go back and basically try to implement, in Australia, some of the ideas that I had collected along my travels. The SKA was a great chance to be back in the Australian community but also to bring this SKA project to a reality.
ZIERLER: I wonder if you could provide some institutional history for astronomy and astrophysics research at the University of Western Australia.
QUINN: The University of Western Australia was the first large university in Western Australia. It's over 100 years old. There was a little observatory in Perth run by the State Government, and it did some observations in the late 1800s, early 1900s but astronomy was never a big subject in Western Australia. Not until the Australian National University was founded in the 1950s was there a national research capacity in astronomy and astrophysics. Before then, there was very little research astronomy. The Commonwealth Scientific and Industrial Research Organization, CSIRO, was a government-funded organization created in the 1950s, like Bell Labs at some level, where things got designed and built, and science was done. There were some very clever people in CSIRO who were also in the grassroots level after the Second World War in radio astronomy. So, most of the astronomy was elsewhere. There was nothing much in Western Australia. When I arrived in 2006, there was a couple of people in the Physics Department who had some astronomy interests. There was certainly an awareness that radio astronomy could be an important thing, and there were some plans of perhaps deploying some test equipment up into the desert, but there really wasn't much. We went from ten people in 2006 to now where one third of the astronomy community in Australia lives on the west coast. If you add ICRAR, CSIRO, and the SKA communities that are arriving there's probably about 600 people or so. It's big.
ICRAR and the Square Kilometre Array
ZIERLER: When you arrived in Western Australia, was ICRAR already in planning mode?
QUINN: No. As a new Premier's Fellow, the government of the day basically said, "Look, we would like to have the SKA in Western Australia, so anything you can do to help win it would be great." They also said – "Oh, and by the way, make sure the benefits of that thing stay in the state, that we don't just end up having all the benefits go to other places." I scratched my head for about a year trying to figure out the best thing to do to achieve both of those things. Eventually I decided the best thing to do was to create a new institute that was a bespoke organization that would be in the long term indispensable to the SKA project. The SKA project would see the fact that ICRAR exists as an important element of deciding to put the SKA in Australia, part of the SKA in Australia, but also that the institute would have local people interacting with local people that would deliver students and education and STEM outcomes to Western Australia.
The institute—not classical academic, but bespoke institute, kind of mini ESO—was going to be an institute that had astrophysics obviously, the data science, and the engineering, and the education and the outreach capacity that would be essential to weld this institute into the project, and in the long term provide that benefit to the state. ICRAR was my idea that began to take form around 2007. In 2008 we asked the state government to buy into this. The premiere of the state at the time, Alan Carpenter, was very supportive and in 2009, with the two universities—University of Western Australia and Curtin University—and a lot of backing from the state government, we started ICRAR.
ZIERLER: What was the consideration for bringing Curtin University on board?
QUINN: Curtin University already had one or two people who were keen on radio astronomy, particularly radio astronomy technology. We had a skill set there that was very well tuned particularly to some of the engineering aspects of the SKA.
ZIERLER: Of course Australia is very isolated geographically. Was one of the strategic objectives of ICRAR and putting the Square Kilometre Array—to get it going—that Australia generally would be more of a destination for international astronomers?
QUINN: Absolutely. We put "international" in the title of ICRAR for exactly that reason. We wanted it to be an international research and development center. We wanted to make it a place where people would focus their energies to do astronomy and astrophysics, particularly in the early phases of the SKA, to design and build the telescope, to figure out how to use it, to figure out all the algorithmics and the data management, all the nuts and bolts of actually doing science with the SKA.
ZIERLER: Was there a local supercomputing capacity that you were able to tap into, or was building that part of the objective?
QUINN: One of the skills you learn when you get into big science projects is communicating with governments. The state government of WA had access to the site we were going to use, and the federal government were funding Australia's participation in the project. The federal government wanted to make sure they did everything possible to win a piece of the telescope for Australia, and so did the Western Australia government, so they were mutually aligned. What things could we do to make Western Australian science, and Western Australia in general, look like a very attractive location? We effectively convinced both governments to lay 800 kilometers of fiberoptic cable and build a supercomputer center.
Then the federal government said, "Here's a bunch of money to build a supercomputer center." The supercomputer center was going to be a potential landing pad for a future SKA computer, but in the short term a site which would also do science with what we call precursor telescopes. That's the other thing the Australian government funded. They actually built a small radio array on the site called the Australian SKA Pathfinder to get used to being on the site, to see what the science of the site was like, to put some new technology in the field. Even that precursor telescope needed some supercomputing to do the data analysis. The government's investment in the precursor telescopes, in the supercomputer center, in the fiberoptics, were all meant to enhance Australia's position as a potential place for the SKA.
The Benefits of Distributed Computing
ZIERLER: Where does distributed computing come into play here?
QUINN: Good question. The world has oscillated on this question a bit. The idea of cloud computing, where "clouds" or resources are not local, became a reality in the early 2000s, and it's very much a reality for many people these days. There's a lot of the computing resources that can do jobs for you in the cloud, particularly if you've got a job which is episodic. Maybe you need, for the next 30 hours, a big supercomputer to do this problem, and then after that you don't want it anymore—you don't want to build a building and pay the power bills on a supercomputer if you just want 30 hours' worth of work. That's where the cloud and cloud resources have become extremely valuable and extremely useful.
In the case of the SKA, the computing is a little different. The computer is very much part of the telescope. In the past the concept was there's a telescope that produces some data, and it comes to you, and you do something to it. To do the basic functions of the SKA telescope, observing the sky and producing raw data, you need a supercomputer to be embedded in the telescope. In other words, this is a different kind of model of supercomputing. It's not batch oriented, cloud oriented supercomputing where your use is episodic; a supercomputer embedded in the telescope is 24/7 supercomputing. It has to be there and the supercomputer has to work. If the supercomputer stops, the telescope stops. It is fundamentally part of the telescope. That was a bit of a change of paradigm for how astronomy regarded supercomputing.
Once that embedded supercomputing and then the telescope produces the raw data, you need to process it. Processing that data is a very big task. The amount of data the SKA produces is measured in exabytes. Not petabytes; exabytes. We recently ran some tests on the SKA processing with the Summit supercomputer at Oak Ridge Laboratories. Summit was the world's largest computer until fairly recently, and ICRAR has a research relationship with them. We ran some tests on Summit to synthesize six hours' worth of data from the telescope being processed. We used the entire Summit machine. We demonstrated that the SKA does need super-supercomputer.
The question you're asking is interesting, whether you can cloudify or diversify some of that. The answer is probably not, because you have to dissect the problem into two parts - a processing part and a data part. Processing, once you've got the data in a form where the processor can deal with it, is straight forward; the processor can do it. But the data is so large—we aren't as good at data storage and data movement as we are at data processing. Data processing is relatively cheap - what's expensive still is storage and networks. We know we have to probably keep big vats of data, lakes of data close to the supercomputing, and then once you carve out a piece of the data, you can send it to a cloud processing units to do something with it and bring it back again. You don't want to try to send the entire lake of data anywhere in the world because it's just too expensive and too big. In other words, you can't cloudify the entire problem. You have to do it in a hybrid way, probably.
ZIERLER: Was the SKA always conceptualized as a truly multinational project, or did that develop gradually over time?
QUINN: In the 1990s it was realized what the cost of the SKA would require it to be international. The probably cost was in the billions of dollars and potentially billions of dollars to just build the computing system to do the science, with tens of millions of dollars a year to pay the power bill to run the supercomputers. It became a project which was beyond the scale of any individual national science program. The case for it being international was obvious I think from the get-go.
So, it became international, and then the question becomes, what kind of international? Is this a treaty? Is it an intergovernmental organization? ESO was a potential model being an intergovernmental organization. ESO exists because of a treaty that is signed at the highest level of government between governments. That means that it has certain privileges and assurances. For example, sending equipment from A to B, you don't have to pay certain kinds of taxes. Employment contracts become easier. So, there are some advantages surrounding intergovernmental organizations for doing a project which is intrinsically international. The easier you can send people and things around the world, the better.
ZIERLER: The siting in both Australia and South Africa, I imagine that there's some specific value in being located in the Southern Hemisphere?
QUINN: Certainly there was an attraction to the Southern Hemisphere in terms of what you can see well from the Southern Hemisphere. The Southern Hemisphere has the Magellanic Clouds, our nearest major galaxies as well as the center of the Milky Way galaxy. We also have some views orthogonal to the plane of the Milky Way into deep cosmological space. We have other big optical telescopes, the VLT and ALMA radio telescope in the Southern Hemisphere, and the complementarity between radio, millimeter, and optical infrared was extremely attractive as well.
ZIERLER: We talked about this sort of at the margins, but let's get into a little more specifics. I'm curious, as the collaboration grew—as the technology, the instrumentation, the computation, all of the international partners—what was the impact of that on the original science objectives? Have they changed over the past two decades?
QUINN: I think the fundamental science objectives have stayed the same. The focal point of the science is basically the epoch of reionization—looking at the earliest signals of hydrogen gas from the very first stars in the universe—that has driven the science case. To be able to do panoramic surveys of the sky to get literally billions of galaxies in the radio. What does that mean? That means billions of black holes. Because every one of those galaxies is powered by a central engine which emits radio waves which is likely to be a black hole.
The fundamental science case and agenda have stayed the same but the path towards that point has evolved. The SKA was always imagined in two phases: phase one, which was roughly 20 percent of the array, and then a full build out in phase two. Right now we've just started last December phase one constructing. The project is struggling with the realities of building phase one because of the economics of the world we live in today. Inflation, obviously the pandemic and its impact on prices and people's movement, the cost of aluminum, all have significant impacts. It will be a challenge to deliver the capabilities of phase one by the end of this decade. Phase two I think has probably drifted off some distance until we prove the success of phase one.
ZIERLER: When you decided to step down from the leadership role at ICRAR, how did that change your research? Did it actually have a beneficial aspect of allowing you more bandwidth to do the research?
QUINN: It didn't change my research. My research interest was still in dark matter. I was doing some work on axion dark matter when I was still executive director of ICRAR because I had a particularly talented student, Katharine Kelley. It allowed me more time to think about what I wanted to do, for sure. It allowed me to come over to the US for a month or so a year and think about dark matter. It also allowed me some time to think about other things like the International Space Center and the Data Institute. I don't intend to sit on the porch, so to speak.
ZIERLER: We'll bring the story right up to the present. What is the current state of play with the Square Kilometre Array and what are your best hopes for it?
QUINN: We started construction before Christmas. I'm talking about the Australian construction. The South African construction is going very well as well. We are going to build 512 pads of antennae in the desert. Each one of those pads is about 100 meters across and has 256 Christmas-tree-like antennae. So, 512 pads each with 256, is about 130,000 antennae. We imagine that we'll have probably a quarter of it made in two years' time and then maybe the whole thing by four years' time or so. The nice thing about building a radio telescope array is you can do it in bits. With this array, probably when we get maybe a dozen or so pads out there in the desert, we'll start doing the very first serious science-like tests. Full operational science verification will probably be three to four years away.
ZIERLER: Now that we've worked right up to the present, for the last part of our talk I'd like to ask a few retrospective questions about your career, and then we'll end looking to the future. Given all the focus on building observatories and institutions, we haven't really talked about your career as a mentor, as a teacher to undergraduates, as a mentor to graduate and postgraduate students. What has been most meaningful to you in terms of the classes you teach and the people that you've trained?
QUINN: Unfortunately, given my career path, I haven't actually taught that much. I like teaching very much, and I consider it a bit of a failure that I haven't taught more. I've taught a couple of graduate courses and I love it. In this next period, I'm hoping that teaching and mentoring will be part of what I do next.
In the training of the PhD students, I've had probably had about 15 or so students over the years. Not a huge number, mostly because I felt very much like I always wanted to give the students a lot of my attention when they needed it. In my functional roles quite often that wasn't possible, because I traveled a lot and did things. So, I looked for the students that I thought I could work well with who were really self-motivated and I could guide constructively. I've been very happy in having about 15 or so students who were really self-motivated in various ways and that really did very well. A lot of them have gone off into non-astronomical careers. That is, again, in astronomy these days, not unusual. We produce far more young astronomers than we've got tenure-track astronomy jobs. They often go off into some really interesting career paths. Basically you're taught how to solve hard problems. All sorts of people want to solve hard problems, in particular if the hard problem involves computers and data. I have two people who are very high up in Google who have come through me, which is very nice. You train them with a skill set that can be applied in multiple dimensions. It has been very satisfying to see those young people succeed in multiple dimensions. I think that's why part of my message was, "Do not be averse to having a career which has got zigzags."
Tracking Discovery and Computational Power
ZIERLER: You grasped early on, all the way back to the 1960s, the value of computers in science generally, in astronomy specifically. Has the explosive growth in computational power matched the explosion of discovery in astronomy and astrophysics? Do they basically track in your mind?
QUINN: In a funny way. There's two ways to look at this. You could build a bigger computer. You can build a better simulation and you get more physics knowledge, more physics insights. I think where the computing and the algorithms and the software has helped most is in the observational side. We've had some telescopes which have produced incredibly rich datasets. I'm talking about Planck, for example. Planck has produced some beautiful data and very complicated data, and data that really needed new algorithms, new data processing, new concepts even, for describing the data in some sense. I think more so perhaps than just the raw computing power, the growth of algorithmics and the understanding of how to do data science, has improved and grown and supported breakthroughs in science. I think the results of Planck owe a lot to a better understanding of applying statistical rigor, of building new techniques for multiple dimensions of data and to designing new descriptions of data. I think it's our stepwise growth in understanding how to use computers, how to use that potential, that has really aided science as well. That will be probably the case for some time. It's our use of the computing resources that has improve our ability to compute. It's not the computer that does it; it's us. Our ability to compute is two parts. It's the computer raw power plus what we tell it to do.
I will also put a word of caution out there. When Lars and I were doing modeling of galaxies with computers, we wrote all the code ourselves. We wrote it, and we understood why it worked, how it worked, when it was broken, where you could trust it where we could not. One of the success stories of course is that now these codes have become very popular, and they are part of research all over the world, accessible to everybody. You're a graduate student; you can download a really high-performance simulation code, and you can run a simulation and get some interesting results. I don't think there's enough introspection going on. I don't think people are asking hard questions enough about what's inside the "black box". You see students come forward, and not even students but full-fledged researchers come forward, making claims about something they got from a simulation, and you know in your heart of hearts, when you were a young lad, that there was a problem with this stuff. Maybe it has been forgotten, or maybe it's just invisible in inside the black box, hidden behind a lot of smarts. But there's still some intrinsic limitations for the way modeling works. So, I also try and teach the students to be a little bit introspective and a little bit questioning of the results. What does the basic physics tell you should be true? Does what is happening make sense from the basic physics point of view? While big computers and big codes are great for science, stop and think about what they produce.
ZIERLER: The theme that is coming out loud and clear from you here that it's the people that are driving the machines, I presume that means that currently machine learning, artificial intelligence, is not changing the equation, and for as far out as you can see in the future, it won't?
QUINN: It's a very good question. I think there's clearly a role for AI to do some of the things which are currently not done very well by the systems we have as astronomers. The way we classify things, the way we break problems up into parts, the way we explore various kinds of parameter choices—I think this is where artificial intelligence and machine learning will have a big impact. I don't believe it will replace the research, the person in the equation. I think the person in the equation brings some conceptual parts that didn't exist before. Language models are beautiful, and large language models are great, but they're just the sum of what exists. Quite often as a researcher, you come up with something that doesn't exist. You come up with a new idea, a new concept. That's something a machine might eventually learn, but you came up with it. I think people are the critical part of research, but there's certainly a huge opportunity to use the advancements we're seeing right now in making what we do better.
ZIERLER: You have fostered collaboration. You have made scientific discovery possible through collaboration from as small as across the office to across the planet, in international terms. What are some of the big takeaways for you? What have you learned about leadership, about bringing people together, about all of the issues, the cross purposes, the administrative burdens, the financial issues? What have you learned to make it all work?
QUINN: There are a few basic lessons I've learned. I learned some of them from people, like Riccardo, and I learned some of them myself the hard way. I think in terms of doing science and doing big projects in particular, putting together these purpose-built, bespoke entities that help you do science is fundamental. In doing that, it's really important to have a clear impression of what are the things you want to do. I have an engineering friend and he always used to talk about "form following function", that the thing you build is a reflection of what you want to do, not the other way around. That form-follow-function thing is very much instilled in me, that understanding of what it is we want to do. In building ICRAR, what is it we wanted ICRAR to do? We wanted ICRAR to help win the SKA, and we wanted to basically anchor the results, the benefits into Western Australia. That's what we wanted to do. What form should it have, then, to do that? Well, it needs astrophysics, and it needs engineers, and it needs data science, and it needs education, outreach and other things. That form followed the function.
In putting that all parts and skills together into making the right "form," one thing that is really important is to have the parts be complementary rather than conflicting from the start. If you've got two universities—pick any two universities in the world—I'm sure you'll find conflicts of various kinds between them. It's very important to find a way where party A does what party A does well, and party B does what party B does well. A can celebrate it, and B can celebrate it, but they don't collide. A's part and B's part are not the same parts, so that you don't build conflict into your enterprises from the very beginning. You keep your enterprises well defined, well directed, well celebrated. That's the complementariness that you critical to success.
You also need supplementary. In other words, you want to find something that you can create in the middle. This collaboration of two entities is not enough collectively to do the job. You need to make something new. You create something new, and that creation which is new is a creation of the collaboration. It wouldn't have existed unless the collaboration existed. All of a sudden you have something that both can celebrate. We can both celebrate this thing in the middle that we created together, and it didn't exist before. So, supplementary and complementary mindsets are essential to putting these things together from lots of parts. And you'll always be putting these big things together from parts. Complementary and supplementary, that's a really important lesson I learned.
A white piece of paper is the other one. You need a white piece of paper if you're going to build new fit-for-purpose entity. You don't want to be overly restricted. For example, you need to employ seven of those people and three of those and you don't wont caps on the types of people you employ that have nothing to do with your needs. You can only spend money on "tables and not chairs". This sort of stuff is a killer. You will not build the form that follows the function. You want to fit for purpose entity. To do that, a white piece of paper is essential. We were very lucky to be given that by the two universities and the state government, for ICRAR. "You build what you think is needed—here's a bunch of money. Do it." That is so valuable. It's scary, it's completely scary, because you have to start from scratch on everything, but it's also the right thing to do when you're building the correct "form".
A white piece of paper is incredibly important. Complementary and supplementary is very, very important. A sense of timing is also very important. Make sure you do things when they're best done. Sometimes you might not have the money, or the people, or the opportunity, or it just might not be the right time to start something. Or you did something but you forgot to celebrate it, and nobody knew you did it. That's bad, because then nobody knows "why you're there". So, celebrating at the right time, act at the right time. My mentor, Riccardo Giacconi, had a saying—"Early to bed, early to rise, work hard, and advertise."
ZIERLER: [laughs]
QUINN: Never a truer word! Because that advertising, that sense of timing, is extremely important. So—white piece of paper, complementary and supplementary, sense of timing. I'll add one more at the end, and it's to do with people. It's to make sure people understand what it is they need to do to be successful. I've seen so many times people in organizations that are very unhappy. Quite often it is because they don't understand their role in a big picture and what you want of them to be successful, to fulfill their role. In other words, it's just a clear definition of where they fit in the big picture, and what you want them to do to make the big picture happen. It sound simple but it's so often lacking. Because there are many tangential things to do. You have to fill in this form and do that report and who knows what. Many times, as I said, I've had various problems with fairly senior people who are indispensable to the organizations who have gotten into a rut because they've lost track—well, I've failed, because I haven't told them what they need to do properly—but they've also lost the plot because they've lost their picture of it. Big pictures and roles, and a shared awareness of them, are the key part of the story.
Dark Matter for the Double Win
ZIERLER: Finally, last question, looking to the future, we'll end on an optimistic note. Let's say, best case scenario, the axion research plays out. Home run! It is dark matter! What does that mean? What does that mean for our understanding of the universe? What does that mean for astrophysics and astronomy?
QUINN: It would be a double win. It will be a big win for the Standard Model of Particle Physics because that was a big prediction and missing piece of the puzzle. In some sense, it has to be there. If it's not there, the Standard Model is in big trouble. It would be a big win for astrophysics because, one, we'd know what this silly stuff is, but two, we could then start "looking" at it. We could then start measuring the dark matter's properties. If it's there and if it's detectable by the kinds of methods I think could be possible, we could actually measure things like an accurate total dark matter mass, the velocities of the dark matter particles, the mass of the particles and how the dark matter is moving as a part of the galaxy and the universe in general. This will tell us when the axions first emerged as a component of the mass of the universe. We'd have a different window on galaxies, a different window on the universe, because we can make measurements on dark matter we couldn't have made before. Because we've got a tool now, we've got axions, and they decay and make photons we can measure. That will tell us things about dark matter we would never have known without the axion.
ZIERLER: This has been a wonderful conversation. I'm so glad for our time together. Thank you so much.
QUINN: My pleasure.
[END]
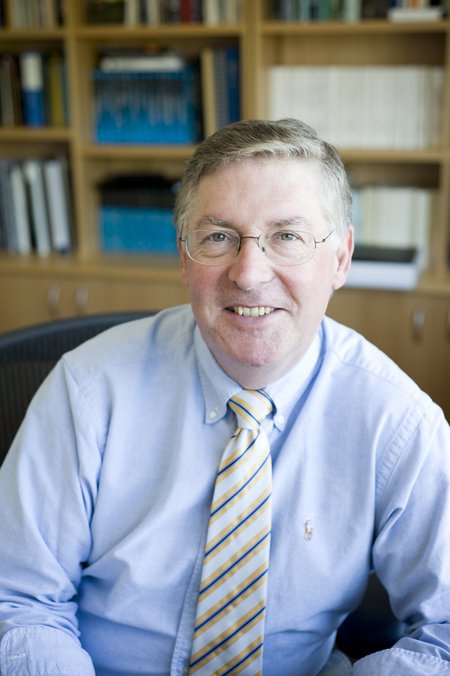
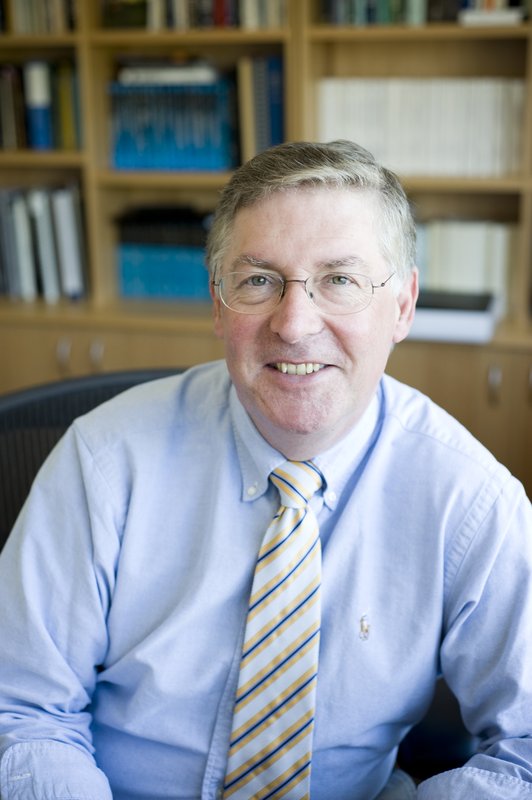
Interview Highlights
- Radio Astronomy in Australia
- Dark Matter in Historical Perspective
- From Wollongong to Canberra
- Computers and Galactic Mergers
- Between Theory and Experiment at Caltech
- Early Perspective on LIGO
- Early Days of Supercomputing for Astrophysics
- MACHO and Return to Australia
- The Big Data Challenge in Astronomy
- Virtual Observatories and Citizen Science
- ICRAR and the Square Kilometre Array
- The Benefits of Distributed Computing
- Tracking Discovery and Computational Power
- Dark Matter for the Double Win
Related Links