Sean Solomon (BS '66), Geophysicist and Planetary Scientist
April 22, 2022
Sean Solomon's research career spans from Earth-based seismology to studying the relationship of the interiors and surfaces of Earth-like planets. He was introduced to geophysics as an undergraduate at Caltech and remembers the Seismo Lab when it was housed off-campus in a mansion. Solomon went on to complete a PhD at MIT on seismic attenuation, and he stayed on to join the faculty at MIT. He then moved to become director of the Department of Terrestrial Magnetism of the Carnegie Institution of Washington before taking up his most recent role as director of the Lamont-Doherty Earth Observatory and a chaired professor of Earth and Planetary Science at Columbia University.
Solomon's key research projects include serving as a Principal Investigator for NASA's Astrobiology Institute, as a member of the Magellan Project Science Team and the Mars Orbiter Laser Altimeter Team, and as Principal Investigator for NASA's MESSENGER mission—the first spacecraft to orbit Mercury. Solomon's numerous honors include the National Medal of Science, the G. K. Gilbert Award from the Geological Society of America, and the Harry H. Hess Medal from the American Geophysical Union. He has received the Distinguished Alumni Award from Caltech, and he was elected to the National Academy of Sciences in 2000.
Interview Transcript
DAVID ZIERLER: This is David Zierler, Director of the Caltech Heritage Project. It's Friday, April 22, 2022. I am delighted to be here with Dr. Sean C. Solomon. Sean, it's great to be with you. Thank you for joining me today.
SEAN SOLOMON: Happy to have this conversation.
ZIERLER: To start, would you tell me your title and institutional affiliation?
SOLOMON: I have almost no titles at the moment. [Laugh] I used to have many. Up until 2020, I was Director of the Lamont-Doherty Earth Observatory and a chaired Professor of Earth and Planetary Science at Columbia University.
ZIERLER: Are you enjoying a full retirement now? Are you emeritus? Are you involved institutionally at all?
SOLOMON: I still have an institutional appointment that allows me to do research. Columbia and Lamont kindly allow me to use an office and the Columbia libraries, and I can do that virtually. I keep my hand in research and advising, but I don't have any administrative responsibilities, I don't teach formal classes, and most of my collaborations are remote as well.
ZIERLER: During your time as director of the Observatory, how able were you to stay close to the science? Were you able to keep up an active research agenda yourself? Or that was simply too demanding?
SOLOMON: No, I was running a spacecraft mission at the time, and that was also intensive. I was the principal investigator for NASA's MESSENGER mission, the first spacecraft to orbit the planet Mercury. That had been launched in 2004. It took a very tortuous path through the inner solar system in order to use planetary flybys to slow its speed relative to its ultimate target planet, so it didn't go into orbit until March of 2011. I moved from the Carnegie Institution of Washington where I was, until 2011, director of the Department of Terrestrial Magnetism there, to Columbia in July of 2012. It was in the middle of the orbital operations for the MESSENGER mission. I was juggling both tasks at the same time. I was doing my own science largely through a splendid team of scientists working on the data from the orbital phase of the MESSENGER mission. At the same time, I was learning all the science that goes on at Lamont and helping direct that institution.
ZIERLER: When you became director in 2012, what were the big issues facing the Observatory? What was most important for you to accomplish?
SOLOMON: My biggest goal, really, was to maintain, and if possible, enhance, the scientific excellence of the Observatory. Despite my mix of backgrounds in Earth and planetary science, Lamont is pretty squarely Earth-focused. That was not always the case, but it has been for several decades. And they're really a premiere organization across more aspects of Earth science than most university departments can afford to do, even Caltech, simply because of its size, and the large number of faculty, and the ability to make appointments limited only by resources. Resources was the second concern I had, and I spent a lot of time interfacing with the university, dealing with fundraising, and endeavoring to work on strengthening the financial stability of Lamont.
ZIERLER: Were you able to accomplish that by the time you retired? What kind of financial shape is the Observatory in now?
SOLOMON: Near the time of my retirement, Lamont became part of a new entity at Columbia called the School of Climate, Earth, and Society. That's very much a work in progress and was when I stepped down. Lamont may well be on a long path toward greater financial stability as an embedded institution in the scientific heart of Columbia's Climate School. But that Climate School is still nascent – fundraising, recruiting, and trying to define itself. I think we have to watch for some years to see how successful that experiment will be.
Earth and Planetary Science at Columbia
ZIERLER: Some questions about the Observatory in historical perspective. Prior to you becoming director, what were some of the big research areas of the Observatory, and to what extent did that change during your time as director?
SOLOMON: Lamont as an Earth science observatory goes back to 1949, when Maurice Ewing, then a faculty member at Columbia, took advantage of a gift of the Lamont family estate to the university. He said it would be a wonderful site to build what they then called a geological observatory, which became an Earth observatory. Ewing was a physicist and very interested in seismology, the structure of the Earth, the nature of the seafloor, and oceanic crust. He brought with him, as one of his almost brand-new PhDs, Frank Press, who became a staff member at Lamont before Caltech hired him away to bring him to direct the Seismological Laboratory in 1957, I believe. Lamont, early on, was a really strong player in the development of global seismology, making use particularly of surface waves, but more generally of quantitative methods, the power of the computer, to understand large earthquakes and the structure of the Earth. In 1953, I believe, Ewing acquired Lamont's first ship, so it became a seagoing oceanographic institution that carried out any kind of measurement they could make in marine geology and geophysics. Almost everything was new. What was on the seafloor? How old were the ocean basins? What was the thickness of the crust in the oceans? What was the relationship between the crust beneath the oceans and the crust beneath the continents?
The seagoing work, seismology, and other aspects of geophysics were the original strengths of Lamont, all guided by Ewing and a small, very dedicated staff. Lamont was very instrumental in forwarding what became known as the plate tectonics revolution in the 1960s, the concept of rigid plates moving in steady relative motion, accounting for most of the world's earthquakes, volcanoes, and eruptions of fresh magma on the Earth's surface or seafloor. While those basic ideas came from elsewhere, Lamont, by virtue of its years of collecting marine data, had archives of deep-sea cores, magnetic anomaly profiles, and seismic data, so that when these concepts were first being put forward in the early 60s, it was scientists at Lamont who could go to these huge libraries of marine and seismic data and test the ideas that became the underpinnings of plate tectonics. The observatory was a real hotbed of global geophysical research and synthesis in the late 1960s.
ZIERLER: Some overall questions as they relate to your research. First, when did you start thinking about planetary science beyond Earth? Was that right from the beginning when you were a graduate student, or that came later on?
SOLOMON: It came when I was an undergraduate. Caltech, as you may know, was at the forefront of exploration of the planets, the earliest spacecraft missions, through the Jet Propulsion Laboratory, which they managed and still do, but also through the interests of faculty members. And I realized early on that there were key faculty members with whom I interacted who were interested in planetary science and asking questions about other planets. And some of them were involved in planetary missions. I will never forget the Mariner 4 mission, which was the first spacecraft to visit Mars and successfully return data, because that probe flew by Mars in the summer of 1965, and it was the first time we'd had any close-up images, outside of a telescope, of the surface of another planetary body other than the Moon. Several Caltech faculty – Bob Leighton, who had taught me freshman physics, Bob Sharp, who had taught me Geology 1 and was instrumental in my switching majors to geophysics midway through my undergraduate career, Bruce Murray, whom I was going to know better after that summer, Gerry Neugebauer, who taught some lectures in an astronomy course I took – they were all central to the first imaging experiment on an interplanetary spacecraft.
The imager was very primitive by modern standards. It was a vidicon system, and the probe didn't have the communications system permitted by modern technologies. The data rate was very, very low. But Caltech had set up televisions on the campus that summer, and as the images were beamed back to Earth by the Mariner 4 spacecraft, you could go to several places on campus and see each image develop one line at a time on a television screen. Eventually, if you had the patience, you'd get a view of the surface of Mars, the first close-up view that humanity ever obtained. I was fascinated, watching those images returned. There weren't very many at all. It was a flyby mission with a low-data-rate communication system. I believe there were maybe 20 images in all. Either due to poor luck or the vagaries of celestial dynamics, all of the images came from one particular portion of Mars that was heavily cratered and was not representative of the entire planet, but looked lunar-like in the high density of impact craters, which gave rise to the view that persisted for a number of years – until the first orbiting spacecraft was successfully sent to Mars – that Mars was an ancient planet without any modern geological processes going on today.
I took courses in the physics of planetary interiors from Don Anderson and Bob Kovach, and I was deeply impressed that planetary science, just during my tenure as an undergraduate, was becoming a discipline, a legitimate field of study to which serious scholars from the fields of geology and geophysics were turning a portion of their research time.
ZIERLER: I wasn't prepared to go all the way back to Caltech because I had no idea you were thinking along these lines then, but while we're there, we'll stay in the narrative. Was JPL relevant at this point at all? Were they yet a player in planetary science?
SOLOMON: They certainly were in terms of delivery of spacecraft and development of experiments. Less so in terms of scientific personnel. This is 1965. In 1965, there were almost no planetary scientists. It wasn't a discipline. It was before Apollo. A few scientists had sent experiments on robotic spacecraft to the Moon, and JPL had launched Mariner 2, which successfully flew by Venus in 1962. But there was a Mariner 1 that failed, and a Mariner 3 that failed. The early robotic exploration of the Moon was also not nearly as successful as we think of the space program today. The number of people who turned a large fraction of their attention to the planets was still quite small in 1965. Lunar science, largely through Apollo, which came a few years later, and planetary science, really entrained scientists from physics, geology, chemistry, and other fields, as there were opportunities to look at a new object and turn our eyes away from the Earth.
ZIERLER: Being from Los Angeles and being interested in science, was Caltech the be-all and end-all from you? Or did you think about places farther from home?
SOLOMON: I went to a public high school in Los Angeles. I still marvel at my naivete at the time. It was uncommon in the early 60s – I graduated high school in 1962 – to visit colleges to which one applied. There must've been people who did that, but I did not. I didn't have the resources to visit very many schools. Of course, nowadays, it's almost unheard of for a high school student to go to a college they haven't visited. But Caltech was very well-known to me, and I only applied to two other schools. I applied to MIT, and I applied to Stanford. Stanford in 1962 wasn't quite the Stanford of today, and I regarded it as my safety. They would be amused by that now. [Laugh] In any event, I got into all three. But when I graduated high school, I was 16. Caltech was much closer to home and had a stellar reputation as the Southern California institution of scientific and technical prowess. I wasn't quite ready for the culture shock of moving to the East Coast, so Caltech was my choice.
ZIERLER: What was the game plan? What did you want to study when you arrived on campus?
SOLOMON: Well, like 95% of my class, I had in mind majoring in physics.
ZIERLER: Did you know about figures like Feynman and Gell-Mann even before you got to Caltech?
SOLOMON: I may have heard of Gell-Mann. I don't think I'd heard of Feynman before I set foot at Caltech. My level of physics was pretty limited, unlike a lot of my classmates at Caltech. I didn't take high-level physics classes or a summer school in physics. I just didn't have the exposure. I just took one high school physics class and read Scientific American, so my understanding of physics was pretty much at the lay level, other than the high school class. It was clear, once I arrived at Caltech, how outstanding the faculty were in all fields, including physics. But the physics department did not wish to have 95% of the class major in physics, so they took it as part of their mission to discourage those who were not at the very top of the class in physics. I was taking freshman physics the year after Feynman taught it. We followed his lecture notes. As I mentioned, Bob Leighton gave almost all of those lectures, trying to teach Feynman physics but not being Feynman. He did a fine job.
But I did get some exposure to what it was like to take physics from Feynman in my sophomore year because he, the year before, had taught Physics 2 to the sophomore class one year ahead of me, and he decided he didn't do as good a job as he wished on the quantum mechanics piece, which was the third quarter, so he taught it again to our class. For one quarter, my class got the tremendous gift of hearing Feynman himself give lectures in quantum mechanics. But it was very challenging because while his lectures were fascinating, they didn't bear any relationship to the problem sets or tests, and particularly freshman year, I started out at a big disadvantage in physics background compared with some of my classmates, and I didn't score that well in freshman physics. I did better in sophomore physics and much better by the time I was a junior. But at the beginning, the difference in backgrounds was more important than later. I was one of those discouraged from majoring in physics by the end of my freshman year, and we had to declare a major for sophomore year.
The only scientific classes I'd had were physics, chemistry, and mathematics. I did well in mathematics. For lack of anything better, I majored in mathematics for my second year. But I found that pure mathematics was pretty dry. While I could get good grades, I couldn't see myself doing that for my entire career. There were three elective classes my sophomore year that had once been required of all students in the sophomore year – one was Geology 1, one was Biology 1, one was Astronomy 1 – but they were electives by the time my class got there. Nonetheless, word of mouth was that the geology class was excellent and that one should take it. And more than half of my class did take it as an elective. Bob Sharp gave most of the lectures. He had a few guest lecturers from other parts of what became GPS. But most of the lectures were his, and he was so good and engaging. There were a couple short field trips that Clarence Allen led. It was just a wonderful class. Also, by word of mouth, the second quarter of my sophomore year, I took geophysics, which was Ge 2 and was then taught by Stewart Smith. It was a sophomore-level look at the physics of the Earth – seismology and other physical aspects of the Earth and its interior. It was more quantitative than the geology class, and I liked that. I was quite interested in the application of physics to Earth science. But that was really my first exposure to geophysics, that class. Stewart Smith was OK as an instructor. He wasn't a Bob Sharp in terms of a charismatic lecturer who had his audience at the edge of their seats, like Bob did. But he still taught a very good class.
By the end of the third quarter, I decided I would like to switch majors from mathematics, which I was finding dry, to geophysics. I went to see Bob Sharp in the springtime of '64. I went into his office. I guess he got a lot of visits from students looking to change their majors. He was very polite, but he asked me what my grade point average was at one point, expecting that to be, I guess, a criterion as to how interested he would be in encouraging me to change. I gave him my grade point average, which was pretty high, notwithstanding a few of the freshman physics grades, and his interest perked up. He said, "Oh, let me see what I can do."
ZIERLER: To clarify, it was a high GPA, but not high enough to stay in physics?
SOLOMON: My grades were high in almost everything but physics. I was a B physicist as a freshman, and that doesn't cut it. Or didn't at Caltech. I got As in physics, as I said, by the time I got to be taking classes as a junior. As a first step toward testing geophysics, I asked Bob if he would help me to find a summer job in geophysics – because I lived within driving distance – that I could use to learn more about the field. He said he would do that. And within a few days, I got a call from Stewart Smith, from whom I'd taken Ge 2. He said, "I heard about you from Bob Sharp. I remember you from Ge 2. How would you like to spend the summer working on the spectral analysis of earthquakes?" I had no idea what the spectral analysis of earthquakes was about. But I wasn't going to say that, and I wasn't going to say no. [Laugh] I signed up immediately. That became the first of three successive summer jobs I had working for Stew at Caltech's Seismo Lab. By the end of the summer, I was thoroughly convinced that geophysics was a much more interesting field, and I formally switched, as I'd promised Bob Sharp, my major to geophysics as of that fall.
Geophysics at Caltech
ZIERLER: Once you understood the project, what were you looking at?
SOLOMON: Stewart was very kind because I had no computer programming background. We used that first summer to teach me how to program a computer. I learned Fortran, I learned how to punch computer cards on a key punch machine, and how to submit jobs to the central Caltech computer center, which required driving onto the main campus and handing over a deck of computer punch cards to a computer operator, then either hanging around or coming back later to get the output of the computer job, once they had run through the queue and gotten to the job. Once I had gained programming confidence with simple tasks, the particular assignment I had from Stewart Smith was to take advantage of a new algorithm that had been developed by a couple of scientists at IBM and Princeton to calculate Fourier transforms much more efficiently than the traditional means. That became known as the fast Fourier transform. He had code for that particular algorithm and wanted to adapt it to looking at normal modes and other long-period seismic waves. I'm not sure if he ever used my code, but he delegated to me the task of writing software that made use of this new algorithm for doing spectral analysis, doing Fourier transforms. It was a great experience for me. I got to meet a lot more of the seismologists at Caltech. I was able to teach myself Fortran programming, and I started learning about research and geophysics.
ZIERLER: Was this at the mansion? You were off campus for this work?
SOLOMON: For the summer, yes. They kindly gave me, a rising junior, a desk in one of the rooms with graduate student desks in the upper Seismo Lab, the Donnelly Lab. The room was a converted living room; it must've been, just as a guess, 50 or 60 feet in the long direction, and it had 10 or 12 Steelcase-type desks. Most of them were occupied by graduate students in seismology. My first summer, I was rubbing shoulders with Francis Wu, Leon Teng, Lane Johnson, Bruce Julian, John McGinley, and Larry Turnbull, all of whom were there doing graduate work. I'd taken one class in geophysics and was learning how to program a computer, but they were still friendly and supportive to somebody as junior as I. I made some great friends. It almost felt like what it might be like to be a grad student at Caltech at the time.
ZIERLER: Even as an undergraduate, did you have a sense of what some of the big debates were in seismology and geophysics at that point? For example, what were people saying about plate tectonics?
SOLOMON: Caltech kind of got left behind in the plate tectonics revolution. For instance, J. Tuzo Wilson's paper on transform faults and his recognition that the San Andreas Fault was a transform fault were both published in Nature in 1965. But his idea really wasn't grabbed by the Caltech faculty. I overheard some of the geologists talking about Wilson, and they didn't have a very high opinion of him. I guess he had been a source of many earlier ideas, and only some of them turned out to be true. But he was a very creative guy, and the transform fault hypothesis was key to the development of the plate tectonics theory. I think Lamont was better suited to take up these ideas – it was a competitor with Caltech in seismology at the time. We were always reading papers by Lamont seismologists. Frank Press, of course, who was director of the Seismo Lab when I was working there, had gotten his PhD and first job at Lamont and so knew Lamont very well. He was a very competitive scientist and wanted Caltech to be at the peak of its field, and he regarded Lamont as chief competition. But I think because they didn't have a tradition in marine geology and geophysics, and they weren't sitting on all the data that proved key to demonstrating the validity of seafloor spreading as well as the earthquake data that validated the transform fault hypothesis, Caltech did not participate in the key studies. All that came along in '67 and '68, just after I left. I was at MIT, where Frank Press had moved a year or two earlier to build up another powerhouse department. There were only a few institutions in the earliest phases of plate tectonics that were leading the new ideas: Lamont, Cambridge, Princeton, a few others. Caltech missed that one.
ZIERLER: Did you have a chance to interact with Frank Press at all?
SOLOMON: Interact is too strong. [Laugh] No, I didn't have regular conversations with him.
ZIERLER: Was he imposing? Was that just not an option as an undergraduate?
SOLOMON: He was very busy. He was running the lab, directing the theses of almost all the grad students at the time. He led the now longstanding tradition of the coffee breaks at the Seismo Lab that happened every morning and afternoon and participated in them. When he was around they were strongly restricted to scientific topics. Everything was very businesslike, even though we were in cramped quarters, and the water heaters could be reached if you extended your elbow too far. Still, they were marvelous discussions. But as an undergraduate who had had one class in geophysics, all I could do was listen and marvel. I was learning by osmosis and not feeling that I could contribute very much original at that stage. But I went to Frank for advice. I went to him to tell him I was changing majors, and a year later, I went to him to talk about what graduate schools I should apply to. He was helpful in both cases.
ZIERLER: Besides Press, who else was considered a mentor to you at Caltech?
SOLOMON: I ended up working three summers for Stewart Smith, so I certainly have to count him. I count Don Anderson, from whom I took a couple of classes as a junior. Even though I didn't do any research under Don's guidance, his personality and presence were strongly felt at the Seismo Lab. This is long before he himself was director, but he was clearly a rising star under Frank in Frank's last days. I got good advice from Jerry Wasserburg. I took a mineralogy class from him late in my undergraduate career. I was supposed to take it as a sophomore, and I took it as a junior. But I got to know him and valued his advice. Those, plus Bob Sharp, whom I continued to rely on as a source of very levelheaded advice, round out the list.
ZIERLER: I asked about some of the great debates, and you said that actually plate tectonics was not one of them, so what were? What were the faculty members working on intensively when you were an undergraduate?
SOLOMON: I probably don't have a full picture of what everybody was working on because there weren't easy routes for learning about the research of every faculty member for an undergraduate. In seismology, I could gather, from published literature and what discussions I could listen to in the summers, what some of the seismologists were working on. And I would say that the principal discussions were on Earth structure, the growing recognition that the Earth has a three-dimensional structure that extends quite far beneath the surface, which came out of surface wave seismology, the development of new analytical tools to get more and more information out of seismograms, and the application of computers. I got to the Seismo Lab after the very first papers reporting observation of Earth's free oscillations or normal modes that Press and Smith were both involved in along with Hugo Benioff. And there was always work on seismic sources, extracting information about large earthquakes, and discriminating earthquakes from underground nuclear explosions, because that question was an important source of funding for seismology in that era. And the earthquake hazard problem in California, most generally, because Caltech had already, by that point, a long history of operating a seismological network in Southern California, trying to understand the earthquake hazards. I was not present for any of the significant earthquakes in Southern California. They came along a little later, like San Fernando and others after that.
ZIERLER: Either by the people who were still around who would come to visit the Lab or just from the culture of the place, did you feel connected at all to the Seismo Lab's foundational days?
SOLOMON: I didn't know exactly what the foundations were because that went back to the 20s and the Carnegie Institution, which is another place I spent a lot of time. But I felt that Frank Press's era at the Seismo Lab was pervasive in everything there. I rattled off earlier a few of the grad students with whom I shared office space, but some of his PhDs hung around for a year or two and were also present. Press was sending them off to become faculty members at most of the top Earth science institutions around the country. While I was there, Nafi Toksöz, who went off to MIT, Shelton Alexander, who went off to Penn State, David Harkrider, who went off to Brown, Ari Ben-Menahem, who went back to Israel, and others who had recently completed their PhDs under Frank were also around. Charles Archambeau went off to Colorado after spending some time on the Caltech faculty. You got to see the growth of a whole school of seismology that Frank had initiated at Caltech. He was then sending his disciples out to the great centers of learning across the world.
ZIERLER: What was your sense of how Frank translated his own area of expertise into what the Seismo Lab should be doing institutionally?
SOLOMON: I think Frank, from his graduate student days, had a pretty inclusive grasp of seismology. He developed instruments, he worked on seismic theory, he worked on seismic data. And he was voracious in applying new ideas to the studies of earthquake sources and Earth structure. A lot of new ways of looking at the Earth through seismology came out of that group. But he was involved in some broader debates that were going on in the Division of Geological Sciences. And he lost one. I think that was a factor in his eventual move to MIT. In the 1960s – I got a lot of this information later rather than seeing it as an undergraduate – the Caltech geologists were debating how much emphasis they should place through new hires on two promising fields. One was planetary science, but the other was ocean science and marine geology and geophysics in particular. Of course, Frank, as a product of Lamont, knew the power of those last fields and had argued that Caltech should grow in that discipline and hire enough faculty who worked in that area to be a powerhouse in those fields. But I understand that the faculty decided they couldn't do both and that the proximity of Jet Propulsion Laboratory, the novelty of planetary science, and the opportunities presented by spacecraft missions – maybe colored by the professional interests of some of the then current faculty – led them to choose planetary science over oceanography as a field to emphasize. That wasn't so much a scientific debate as an institutional debate.
ZIERLER: Nowadays, we take for granted how data flows over the World Wide Web. What was the flow of information for the Seismo Lab? Where was it coming from, how did it get to the Lab, how was it analyzed?
SOLOMON: The field of seismology was changing rapidly in the mid-1960s because the US government, motivated by a desire to understand the signatures of underground nuclear explosions and be in a position to monitor explosions by any party around the globe, was developing a new generation of global seismic stations. That became the World-Wide Standardized Seismograph Network. They were pre-digital, but they were standard instruments, observatory-class, that went around the world. At the time I was starting at the Seismo Lab in 1964, that network was still a work in progress. Caltech by and large was making use of data they'd collected through networks in Southern California, instruments like Benioff's strain-meters, specialty instruments at really high resolution but demanding observatory-class conditions.
A few instruments of really high caliber could make a huge difference when a big earthquake came along, and the Earth started ringing globally. Having a few really high-quality instruments anywhere offered the opportunity for large advances. But I think Caltech was also at the edge of the scientific frontier in terms of pushing both theory and the development of efficient computer codes for solving some of the difficult problems numerically that couldn't be solved analytically and making use of the power of computation to advance seismology. The combination of novel instrumentation and emplacement of instrumental networks featuring a variety of forms of measurement, the development of computational tools to take advantage of those data, and the application to a huge host of global problems – they had the full sweep of approaches. That Frank Press school of seismology I referred to really became a leading source of insight into the power of seismology to advance our understanding.
ZIERLER: Although in later decades data would flow freely among and between institutions, when you were an undergraduate, was the data at the Seismo Lab proprietary? Was it not shared freely? Was it something where outside scholars would have to come and visit to look at the data?
SOLOMON: I think the answer was that it depended on which data and which scholar were at issue. There were gentlemanly – to use a non-gender-neutral term but one that was appropriate back then – agreements among seismology institutions to share data, and one could seek data collected by another seismic group if you wrote to them, or had a collaborator, or some other means. You didn't have to visit them in some cases, but in some cases you did. And a lot of the data stayed within national borders. The fact that the field is so global did not mean that all data were equally accessible. But that has largely evolved so that now there are global data centers and global standards for data formatting, for documenting data types. It's much more transparent to the student of today than it was in the early 1960s when you were pretty much stuck with the data your institution collected and what your advisor might be able to acquire from other institutions.
Apollo and Planetary Science
ZIERLER: To go back to this amazing idea that it was as an undergraduate when you first started to think about planetary science beyond Earth, in the days before Apollo, how much of this was simply scientific projection? Before there was the technology and capacity to do this, how carefully could people think about conducting planetary science beyond Earth?
SOLOMON: Well, there are multiple levels to answer that question. Before there were spacecraft, there were a handful of planetary scientists, who were a mix of theoreticians and astronomers. The astronomers could view all the solar system planets, so we knew a little bit about the atmospheres of major planets. Percival Lowell drew maps of Mars acquired through a telescope. They weren't very good, but the concept of mapping was there. The run-up to the Apollo missions that involved lunar landings, 11 through 17, involved a lot of people turning attention to the Earth's moon. Of course, telescopic observations of the Moon produced pretty high-resolution images, and prior to Apollo, we had sent spacecraft to orbit the Moon. The Lunar Orbiter series had taken global images, and spacecraft had landed on the Moon: the Surveyor series did some chemical analyses and measured some physical properties of the surface. Apollo was preceded by enough robotic exploration that we had some idea what we'd find when we got there. But the flip side of your question is that every time we study a new planet in detail, be it through a flyby mission, the first orbiter mission, or the first time we get to the surface of those planets that have surfaces or send an atmospheric probe to those planets that don't, there are always surprises. We don't know enough about all of the planets on the basis of astronomical information or extrapolation from what we know about our own planet.
Over the evolution of the space program, the fact that even the rocky planets are so different from each other came as a surprise. We knew about the Moon, we knew about the Earth, but the fact that Mars, Venus, and Mercury are not like either the Earth or Moon but instead each have their own distinct personalities took multiple spacecraft missions for us to deepen our understanding enough to reach that conclusion. Going back to 1965, there was literature on planetary science, particularly theories about planetary interiors and some theories about geological processes on planetary surfaces that had been based on limited observations like the bulk density of a planet, some information on the atmosphere, or what the atmosphere might do to the surface if there was wind. You could extrapolate what we learned about the Earth in those limited senses. But really, every mission that saw a new body, provided a new extent of coverage, or used a new instrument to record a new type of observation or improved resolution brought new surprises.
ZIERLER: In these early days, I'm curious what the optimism was about the research of planetary science as a two-way street. In other words, taking what we know about Earth and applying that to other planets, and vice versa.
SOLOMON: That question was asked a lot, in part as a motivation for continued exploration of our neighboring planets. Why should we be spending hundreds of millions of dollars to go to Mars? Would we learn something about our own planet that we can't learn by using those funds here? I don't think that's the most important question to motivate exploration of our solar system. I think it's most critical to place the Earth in the context of a complicated star with a planetary system that has evolved through formation, through interaction of many bodies, and through what we now believe to be planetary orbits that may have changed markedly over the history of the solar system. There may have been more planets than we now have, and every planet is distinct, and yet the processes that formed all of our planetary neighbors are common with those that formed the planet on which we live. I think a more compelling argument is that understanding our environment and the planetary system in which Earth formed and evolved helps us to put our planet and the processes that govern the changes to our planet into a much broader and deeper context that gives us an improved understanding of how our planet works.
Because the same processes that produced us also produced our planetary neighbors. We're still wrestling with the question of whether life independently evolved on any other body in the solar system. That would be exciting to know one way or the other. But just from the point of view of planetary processes independent of life, although life has markedly altered our planet, studying deeply the consequences of a different mix of starting conditions and boundary conditions on planetary processes gives us lessons into how special our own planet is and how to understand the way our planet works.
One example that's commonly used is the importance of impacts in the history of Earth's surface conditions, a topic not widely appreciated prior to the Apollo program. If you go to the astronomical literature prior to Apollo, there was considerable debate going back a century or more as to what fraction of the craters on the Moon were produced by the impact of objects that collided with the Moon versus interior processes like volcanic explosions. Serious geologists debated that question for a long time. Even once impact craters were discovered on the Earth, it took scientists like Gene Shoemaker, Ed Chao, and others to marshal the evidence for an impact origin for those terrestrial craters. Then, lunar exploration showed us the history of that impact bombardment on the Moon, a satellite of the Earth in close proximity to us in space relative to the rest of the solar system.
That work immediately led to the realization that the Earth must've been intensely bombarded early in its history and that substantive impacts occurred throughout geologic history. Then, it took the Alvarez hypothesis in 1980 to link an impact not yet discovered with the extinction event at the end of the Cretaceous and the die-off of the dinosaurs. That idea really built on the understanding of impact phenomena from the Apollo program and the Moon. The signature of an extraterrestrial source as the impactor in the case of the Chicxulub crater, which was eventually discovered off Yucatan, was recognized on the basis of deposits collected worldwide by the Alvarez group. That was an example where it took not only a lot of careful work on the Earth but also the planetary perspective to understand the role of impacts in shaping our own planet and even contributing to major episodes in the evolution of life.
ZIERLER: You wouldn't have had any way to compare it at the time, but as an undergraduate, when you were developing these interests, would that have been possible at any other university in the United States? Or was there something uniquely far-reaching and advanced happening at Caltech that allowed you to develop these interests?
SOLOMON: I think I may have had some intrinsic interest in the planets as well as the Earth. Caltech exposed me to a lot of exciting things that were going on in solar system exploration in the 1960s because of the missions being run out of JPL, because of the faculty members who had taught me classes and were working on spacecraft missions. But had I been an undergraduate at Columbia, for instance, maybe a bit entrained at Lamont, and with a different set of faculty, I would likely have still been intrigued by Earth and even planetary science. We sometimes forget that when the Apollo program went to the Moon, not only did the astronauts take images and return samples, they left on the lunar surface a large number of geophysical experiments: a seismic network, heat flow probes, magnetic instruments, gravity instruments, and others. A lot of those were built at Lamont. The seismic system that went to the Moon was led by a Lamont scientist, and Frank Press had contributed to it when he was at Lamont. Less so when he was at Caltech, but he still was a co-investigator on the Passive Seismic Experiment on Apollo from his early investment in that opportunity. There were even seismometers put on some of the earliest robotic spacecraft sent to the Moon to crash on the surface. The very first ones didn't get anywhere close to the Moon. This was the Ranger series. The last three of the nine did impact the Moon as designed, took a bunch of images, and collected some other data. But I don't think any of the seismic instruments by then were aboard or worked.
ZIERLER: Based on all of these interests, what kinds of graduate programs were you focused on?
SOLOMON: There were no graduate programs in planetary science, so I was focused on programs in geophysics. I applied to five graduate schools with the advice of my mentors, particularly Don and Frank. I applied to Caltech, not expecting to stay, but knowing it to be an outstanding institution for working in geophysics, and recognizing that the faculty encouraged its undergraduates to go elsewhere. Then, I applied to Scripps, Princeton, Lamont, and MIT.
ZIERLER: Why did MIT win out?
SOLOMON: It was really the promise of Frank Press. I knew MIT to be an outstanding institution overall. I knew that he had been recruited from Caltech and given carte blanche to put together a first-rate department, had the resources to do that, and was beginning to hire outstanding people, some of whom were former students of his at Caltech. I remembered that I'd once applied to MIT for my undergraduate degree and elected to do Caltech instead, so this was kind of a second try for MIT.
ZIERLER: Who ultimately was your thesis advisor at MIT?
SOLOMON: Nafi Toksöz. A seismologist, Caltech PhD, and Frank Press student. He had been hired by MIT, one of the last hires at MIT before Frank himself was recruited. He didn't come with Frank, he came ahead of Frank, but Frank kept him on the faculty, promoted him, and nurtured his career further, and Nafi did very well.
ZIERLER: What was he working on when you connected with him?
SOLOMON: He was carrying on a lot of the studies that he'd started at Caltech. He worked on some of the earliest work on the three-dimensional structure of the Earth, he made use of surface waves, but he was also interested in earthquake sources. In the time I was at MIT, he also got quite interested in seismology applied to oil, mineral, and gas exploration. I didn't share that interest, but Nafi grew a large group in that area as well.
ZIERLER: In the late 1960s in Cambridge, were you political at all? Were you involved in any of the political tumult that was happening at that time?
SOLOMON: I was not very political. I was at MIT, and MIT was not very political. MIT students worked hard. Yes, there were a few marches – I remember one in 1970 I participated in. But we were much more focused on research. Following the politics, but not actively political.
ZIERLER: What was the process for putting your thesis research together?
SOLOMON: It kind of grew out of some projects I'd worked on with Nafi on seismic attenuation, a variety of studies of surface wave and body wave attenuation and the three-dimensional structure of attenuation in the Earth that came out of those studies. It started out as one paper and evolved with add-ons that grew to thesis level.
ZIERLER: Were there discussions about you joining the faculty even while you were a graduate student?
SOLOMON: No, not serious ones. I did start to interview for faculty jobs in my last year as a grad student, and in two cases, a research job. I got a National Science Foundation postdoctoral fellowship to keep working at MIT, and it was early in that post-doc that MIT made me a faculty offer.
Seismology and Venus at MIT
ZIERLER: What were some of the key conclusions of your thesis research?
SOLOMON: I was trying to understand the variation of seismic attenuation in the upper mantle and its effect on seismic amplitudes, and the frequency dependence of that amplitude, and to relate those variations to the tectonics going on at the surface, particularly to the differences between stable continental interiors and actively deforming continental interiors and oceanic plate boundary regions. It wasn't a formal 3D inversion of attenuation structure. Other seismologists came along much later and did that. But my work constituted early steps toward trying to understand the connection between attenuation and higher mantle temperatures and partial melting, and how those phenomena vary with tectonic setting in the Earth. Although I was on a fellowship my entire time in graduate school at MIT, a lot of the research costs were borne by a large contract that MIT was awarded, much like Caltech had been awarded, by the Air Force Office of Scientific Research. Such contracts were motivated by the desire to understand everything about seismic wave propagation as a tool in nuclear test detection and monitoring. It was the Defense Department that really nurtured the whole field of global seismology in the 1960s, driven by that defense issue. But they were quite enlightened, the defense agencies that supported seismology, to fund almost any kind of seismology that might contribute to that general question.
ZIERLER: You mentioned the DoD. Where was Lincoln Labs in all of this?
SOLOMON: Well, Lincoln Lab is a big DoD-funded laboratory that is still managed by MIT, is well off campus, and so survived a lot of the student unrest in the 70s that pressured MIT to walk away from research requiring security clearances, which MIT finally banned from their campus. MIT also divested then from a nearby laboratory they managed called the Draper Laboratory, which worked on guidance systems that involved a lot of classified work. But I think because the Lincoln Laboratory is 15 miles from the MIT campus, the students never quite pushed to have that divestment happen, so that link is still there. There was a branch of Lincoln Laboratory on the MIT campus for a number of years, a small group in seismology that was very much focused on understanding wave propagation in the Earth and its application to characterizing seismic sources. They hired some of the MIT seismology PhDs, and they provided another group of colleagues on the campus who participated regularly in scientific discussions, seminars, and some collaborations.
ZIERLER: When you joined the faculty, was it the same department in which you were a graduate student?
SOLOMON: Yes.
ZIERLER: And did your research change at all as a result of becoming a professor? Was it a continuation, at least in the early years, of what you were doing in graduate school?
SOLOMON: Yes, but I did a couple things as a graduate student that didn't make it into my thesis but helped define research directions that I continued to follow. One of my early mentors at MIT was Shawn Biehler, a Caltech alumnus and Frank Press student who had gone to MIT. Biehler was getting into marine geophysics, particularly marine gravity. And he didn't have too much difficulty recruiting me to go on an oceanographic cruise after my first graduate year to the Southwest Pacific to shepherd a big gravimeter that was onboard one of the Scripps Institution ships. I spent about ten weeks at sea trying to keep that gravimeter working, and I later analyzed the data from that instrument to understand variations in crustal structure and their relation to plate tectonic processes in the area to the northeast of Australia. It became a paper that was completely independent of my thesis. Also, with Nafi, I got entrained in an effort with another block grant from NASA that Frank Press had secured toward understanding the Moon ahead of the Apollo landings. Frank assembled a team that put geophysicists, geologists, and geochemists together to think about the Moon and make some predictions for what information the Apollo experiments might provide. I got involved with Nafi on thinking about the interior of the Moon, what its structure might be, and how we would learn more about the structure from the seismic experiments.
ZIERLER: To return to this earlier theme about the reality of doing planetary science beyond Earth, when did that start to become a reality technologically? When was your sense that this was actually something that we could pursue?
SOLOMON: People were pursuing it, of course, even while I was an undergraduate. The biggest demonstration that solar system exploration could advance science was provided by the Apollo lunar landings. The first three lunar landings happened while I was a grad student, and NASA very wisely recruited a large number of scientists to work on Apollo experiments and to develop laboratories that could analyze lunar samples when they were returned. This happened at Caltech but around the country and the world as well. By the time the first lunar landing had been completed, the data had come back from the experiments on the surface, and the samples had come back to Earth to be distributed to terrestrial laboratories, there was a large community of people who, at least for a time, were lunar scientists. They weren't trained as lunar scientists; they didn't get PhDs in lunar science; there was no such field. They were chemists, physicists, geologists, and geophysicists who had turned their attention to the Moon because it was an important problem, because there was promise of exciting new data, and because NASA made the investment in labs, instruments, and people. In many ways, the modern era of solar system exploration was born through the genesis process that the Apollo program provided.
ZIERLER: When you were at MIT, was anybody talking about astrobiology? Or that was still too far afield?
SOLOMON: That term – literally meaning the biology of stars and space – has caught on but isn't quite the right term. It was invented by NASA in the late 1990s. If you're asking whether people were thinking about the possibility of life on other worlds, yes. But it was known to be a challenge. NASA probably put the most serious investment in pursuing that question early on into the first landers they sent to Mars. Those were the Viking landers. The first one landed on the 4th of July 1976, the second shortly thereafter. There were orbiters that also operated at the same time, so there were four spacecraft successfully sent to Mars through the Viking program. The major goal of the Viking landers was life detection. But we didn't know that much about the environment of the Martian surface, and we didn't know that much about how to design experiments that could search for life in settings that either were a little different from those on Earth or were challenged by surface conditions.
The Viking experiments were deemed to be a negative result for life on Mars, and that outcome set back Mars exploration for 20 years. But the roots of the Viking program go back several years before the actual mission. By the end of the Apollo program, the planetary community was thinking about astrobiology as applied to Mars and persuaded NASA to fly a mission with two landers and two orbiters directed at that broad question. But Viking was developed ahead of many advances in biological understanding and instrumentation that we now realize are needed to answer those difficult and subtle questions on a body different from the Earth. Of course, astrobiology is now targeting not only Mars, but other so-called ocean worlds in the outer solar system as candidates for hosting habitable environments, places like Enceladus, Europa, Titan, and other bodies with subsurface oceans. It took several generations of robotic spacecraft missions to even develop the understanding of those worlds to the point where we could point to evidence that there are subsurface oceans, and those might be good places to look for prebiotic conditions or even life.
ZIERLER: How did you get involved with the Magellan Project Science Team?
SOLOMON: I was helped by two aspects of my career. One, I was already showing some interest in the planet Venus through publications, and I was on a scientific advisory committee at the National Research Council convened in the mid-1970s to recommend a strategy for exploration of the inner planets in the decade to follow. I was on that committee because of Jerry Wasserburg, who chaired that committee and made sure that the committee included a healthy number of junior scientists who might be around long enough to see some of the recommendations come to fruition. I was one of the people he recruited who were around age 30 at the time, which was pretty young to be on an advisory committee in those days at that level. The development of the Magellan mission, which evolved through several iterations and started out with other names, was centered around a radar system to image the Venus surface.
As you know, Venus is covered by a global cloud layer that blocks almost all visible and even near-infrared wavelengths from the surface from reaching orbital elevations. A conventional imaging system would thus be unable to image the surface, although we now know there are some narrow atmospheric windows in the near-infrared. The idea was to send a radar imaging system because the long wavelengths of radar could penetrate the global cloud cover. It was my good fortune that one of the leading radar scientists who was sought for advice on that mission and ended up being the principal investigator was my MIT colleague, Gordon Pettengill. We'd had many conversations about Venus, and he was a good physicist and astronomer, but I knew he needed somebody with a little more geological expertise, which led to a number of our conversations about not only the need for that kind of imaging, but what you would do once you obtained those images, what that meant in terms of motivation for flying the mission, and the scientific requirements that put on the imaging system and on our measurement objectives. Those conversations led to his inviting me to be part of one of the early proposals to form a science team for a radar imaging experiment on the first iteration of that mission, which then was called the Venus Orbiting Imaging Radar, or VOIR. That mission concept had other instruments, then grew to become too expensive, was canceled, and then was reborn as a radar-only mission known as the Venus Radar Mapper. That name was viewed as boring, so it was changed within a single federal funding cycle to Magellan.
ZIERLER: What were some of the scientific objectives of the mission in the context of what you were working on? What was compelling as it related to your research at that time?
SOLOMON: The very basic question, which is still with us, is rooted in the fact that Venus is the planet in our solar system most like the Earth in terms of bulk properties: mass, radius, bulk density, and presumably composition. It has a very different atmosphere and climate, but it's an Earth-mass planet, the only Earth-mass planet around our star other than our own. It has this hellishly different climate. The question that the radar imaging was designed to address was how the geological evolution of Venus differs from or is similar to that of Earth. And there were hints from earlier radar data. The first radar data from Venus came from Earth-based radar, but it was really limited in resolution. Then, the first spacecraft to do radar imaging of Venus were two Soviet missions, Venera 15 and 16, which helped provide additional political motivation for the Magellan mission when it came to seeking approval for that. Both the Earth-based work and the Soviet missions demonstrated the ability of a radar system to produce images, and we saw that there were lots of features on the Venus surface. The idea was to obtain a global image that would give us a view of the tectonic and volcanic processes that had operated on Venus to compare with those of the Earth. One of the issues that was still debated as Magellan was being assembled and getting ready for launch was whether Venus had some version of plate tectonics. An Earth-like planet, with an Earth-like heat budget: Did it operate like Earth in terms of tectonic plates that moved relative to one another? We saw hints in the Venera data of mountain belts on Venus, and we understood that similar mountain belts on Earth are the products of plate collisions. How did those mountain belts form on Venus? The literature prior to Magellan was quite wide-ranging on the question of whether plate tectonics once operated or is still occurring on Venus. We were really looking with this mission to see if Earth's neighbor and most similar sister planet is Earth-like in its volcanic and tectonic evolution.
ZIERLER: What do we know today?
SOLOMON: We still don't know why the planets look so different. But the judgment from Magellan was that no, there's no modern plate tectonics, there's no continuous network of plates delineated by plate boundaries of the sort with which we're familiar on Earth. But there are features that have strong Earth analogues such as rift zones, volcanoes, and mountain belts. There are also huge expanses of volcanic plains. We don't have a very good idea of the segment of geological history represented by the rocks on Venus because we have no samples. Even the use of impact craters to date planetary surfaces, which is common on airless bodies in the inner solar system, doesn't work very well on Venus because the atmosphere has screened most impactors, thereby preventing the formation of impact craters smaller in diameter than several kilometers. The geological history of Venus is still debated, as is whether there was an era when the climate was different and maybe the tectonics were different, that is, a time in Venus's history when water was stable at the surface and the climate conditions were much more Earth-like.
Climate models now suggest that an interval of Earth-like conditions could've been several billion years long, or such an interval could've been missed entirely in the history of the planet, depending on the starting conditions. Was there an episode when Venus looked Earth-like in its tectonics as well, and are the mountain belts the remnants of that, and some other intermediate form of global tectonic evolution has replaced plate tectonics? These are some of the questions driving the next generation of Venus missions. There are three that were recently approved by NASA and the European Space Agency that are looking to acquire higher-resolution images, high-resolution topographic data, and some geochemical remote sensing of some of the areas viewed as probably old terrain to address those questions.
ZIERLER: Did Magellan play a role in your decision to take a new position at the Carnegie Institution in Washington?
SOLOMON: No, not really. I think Magellan probably helped raise my profile among some planetary scientists and astronomers. Maybe it helped lead to my being offered that position. Frankly, I was persuaded, really, by being recruited by a very charismatic institutional president, who got me to think about leaving MIT, which was not a priority, just by virtue of the power of her personality and the support she evidently gave to her department directors. That was Maxine Singer, a highly regarded biochemist and molecular biologist.
ZIERLER: What was compelling to you about the science in this new opportunity?
SOLOMON: I think it was that the directors at Carnegie are given a lot of autonomy, that there were opportunities coming up for hiring staff members, and that the institutional support for the scientific staff, for post-docs, and for instrumentation was very, very strong. Although the institution is small, and the departments are small in comparison to the largest departments at universities, the amount of institutional funds going into each department per faculty equivalent is as high as at any university. Or at least they were when I was looking at making that change. In addition to the availability of resources and the opportunity to set new directions, there was a good match in that particular department between the people already there and my own scientific interests.
Hot Spots and Mercury at Carnegie
ZIERLER: What were some of the major research projects happening at the time you joined?
SOLOMON: In seismology, it was early in the field of portable broadband array seismology: the use of temporary networks of sophisticated broadband seismic stations to address problems in upper-mantle structure and, in some cases, earthquake sources. Carnegie had invested early in those instruments. There was a national pool of such instruments, but they were still limited in number, and there was a long lead time to requesting them for experiments. The Carnegie scientists had the bright idea to purchase a set of instruments that did not depend on getting in a queue for the national pool, so their scientists could do some of the earliest work with portable instruments and skim some of the scientific cream from those experiments. At the same time, the geochemistry labs at Carnegie were state-of-the-art in terms of isotopic chemistry and addressing problems ranging from Earth history to cosmochemistry and the department's astronomers were individually strong in their areas of work. It looked like a good group on which to build, with new appointments possible because some retirements were in the works, and with new instruments to keep that group at the forefront.
ZIERLER: How much of a professor's life do you retain at Carnegie? Were you teaching, mentoring students?
SOLOMON: Neither. But I'm speaking to the Carnegie departments that were in Washington, D.C. The institution, when I got there, had five departments. One was on the campus of Stanford, one was on the campus of Johns Hopkins, two were in DC, and one was in Pasadena. The departments at Hopkins and Stanford had students in their labs, and many of the staff had courtesy faculty appointments at the university. Those are much more like university faculty. The departments in Washington, D.C., in contrast, were not near any university, had no students, and had no instructional teaching roles. Instead, they had a very vigorous post-doctoral program that brought in a large number each year of very bright PhDs to work in the labs, the field experiments, and other projects. Post-docs are usually independent scientists, but they're also young colleagues who interact strongly with their scientific next-door neighbors. Having a fresh new group of post-docs every year really plays some of the role of having a graduate student body.
ZIERLER: How much did your own scientific research change as a result of joining Carnegie?
SOLOMON: My basic scientific directions, which at that point were pretty broad, didn't change markedly. But I did get involved in portable seismic experiments that I wouldn't have done probably if I'd stayed at MIT. And most of those were focused on so-called hot spots, trying to understand the deep structure of the Earth's mantle underlying those hot spots, using seismic imaging, and taking advantage of broadband instruments deployed for a year or two to gather those kinds of data. And that position at Carnegie made it easy for me, a few years after I got there, to take on the lead role in a spacecraft mission. And that was the MESSENGER mission to Mercury. I think that worked out naturally for me because the implementing organization was not the Jet Propulsion Laboratory, which was across the country from me, but rather the Johns Hopkins University Applied Physics Laboratory. MESSENGER was one of the first deep space missions they attempted to do.
ZIERLER: Do you have a sense of why MESSENGER started at APL?
SOLOMON: Yeah, I've got a very good sense. In the early 1990s, we were coming out of a long dry spell in planetary science. NASA had launched two big missions, Galileo and Magellan, in 1989. But those had been what they call hangar queens for several years, because NASA had no launches of any mission to a planetary object for an 11-year period between Pioneer Venus and those two launches in 1989 as a result of some poor decisions and the Challenger accident. The poor decisions involved NASA announcing that they would no longer use expendable launch vehicles, rockets, to launch spacecraft missions. They decided they were going to launch all deep space missions with the Space Shuttle, really, for political reasons, to give further justification to the Space Shuttle program. But it meant that NASA stopped acquiring expendable launch vehicles and designed all their missions to be launched from the Shuttle, from Earth orbit. The Shuttle is a big bus, and the cargo bay in the shuttle that carries the spacecraft to Earth orbit is big. To take advantage of the big cargo bay, NASA was able to build large, complex spacecraft missions like Galileo and Magellan because they fit in the cargo bay. But those were very expensive. To build a big spacecraft, you've got to pay a lot of money. That means you don't get to fly very many of them in a given time interval. You're putting your bets on infrequent large, complex missions.
On top of that, in 1986, we had the Challenger accident. The Space Shuttle was grounded, and there were no launches until the Space Shuttle was re-certified for flight. Missions that would have launched in the next few years, like Magellan and Galileo, had to wait until the Shuttle was operational again. All of that was so frustrating that the community made a variety of efforts to try to find new ways of developing less expensive missions that weren't reliant entirely on the Space Shuttle, which could be grounded on the basis of a single accident, however tragic. The Mars Observer mission came out of that concept as the first in what was supposed to be a series of spacecraft that built on a common platform design and would be developed by JPL. NASA headquarters also launched the Discovery program of competed mission concepts that were limited only by mission cost, development time, and launch vehicle, and enabled with much higher frequency the launch of smaller targeted missions. In particular, that Discovery program opened the door for the Johns Hopkins University Applied Physics Laboratory to get into deep space.
They'd had a long and distinguished history of Earth-oriented missions funded largely by the Navy and other Defense Department agencies and had outstanding engineers and a solid track record of successful missions. But they hadn't done anything in deep space. With the launch of the Discovery program, a deal was cut that allowed the first two missions under the Discovery program to be developed, one at JPL and one at APL. Those were the Near Earth Asteroid Rendezvous mission in the case of APL and Mars Pathfinder – a Mars lander that included the first small Mars rover – for JPL. All the missions after that were competed. With a foot in the door with the Near Earth Asteroid Rendezvous mission, APL wanted to be in a position to compete for planetary missions, and they submitted proposals in response to each Discovery RFP. By the time I got talking with them in 1996, at which point I'd been at Carnegie four years, they thought they knew how to do a Mercury orbiter and wanted to propose such a mission. There were other groups that also wanted to propose Mercury orbiters, including several at JPL. It took us two tries, but we honed the science and the technical aspects of the mission to lower the risk, so the second time we proposed it, our mission concept was selected for flight. It was the first truly planetary target for a mission by APL, and for a variety of reasons, it ruffled a few feathers at JPL.
ZIERLER: Just a counterfactual, if it did end up taking place at JPL, would you not have gotten involved, most likely?
SOLOMON: I don't know. I could go to APL one day a week, which I did during the peak development phase, and it was a 30-minute car ride each way. Whereas if I'd had to go to JPL, I'd have had to get on a cross-country airline flight each time I wanted to go. I couldn't do that weekly. I don't think I could play the same role and keep a position doing some administrative work and some other science as part of my job as a director at Carnegie. I think it would've been hard. It was not only a good geographical connection, but APL, in contrast with JPL, was small, focused, and sufficiently informal that most everybody knew one another. It was a good environment for tackling as a group a mission with some challenging technical problems.
ZIERLER: What were the science objectives of MESSENGER? What was most interesting to you?
SOLOMON: There was a long list, which I helped develop. And interestingly, they weren't all that different from the list that we wrote two decades earlier as part of that decadal strategy group that I sat on in the mid-1970s; we'd had a whole chapter devoted to Mercury. But in the 1970s, the inner planet trajectories that made use of multiple flybys of Venus and Mercury to permit a spacecraft launched on a conventional propulsion system to be inserted into orbit around Mercury had not been discovered. Those were not discovered until the 1980s at JPL by a mission design expert. But the science objectives were unchanged because no spacecraft had visited Mercury since 1975, the last flyby of the Mariner 10 mission.
We wanted to know what Mercury was made of, because there were no chemical measurements by Mariner 10. We wanted to know the global geology, because only half the surface had been seen by Mariner 10. We wanted to understand why Mercury had a magnetic field, which was discovered by Mariner 10. Conventional wisdom was, because the planet was so small, it shouldn't have one. It should've lost the heat that drives the generation of a magnetic field, as occurs on Earth.
We wanted to know what the polar deposits consist of. They were postulated to be water ice. They were discovered by Earth-based radar in the 90s, and they had unusual radar characteristics, which is how they were discovered, that were best matched by water ice. But we had no geochemical measurements. It was only a hypothesis that these deposits were located in polar cold traps that were cold because they were in permanent shadow because Mercury's spin axis is almost perpendicular to its orbital plane. Also, in the time since Mariner 10, we'd discovered a lot of new constituents of Mercury's very tenuous atmosphere that must be derived from the surface and by surface processes, so we wanted to understand the dynamics of atmosphere generation.
Because Mercury has a magnetic field, it has a magnetosphere – the volume of space dominated by the planetary field that stands off the solar wind most of the time. But Mercury's internal field is much weaker than Earth's and Mercury's magnetosphere is consequently much smaller, and the interactions between that magnetosphere and the solar wind and the interplanetary field were big unknowns that we wanted to study, too. In addition, we sought to learn about the planet's internal structure. There was a very clever theory that had been worked out by Stan Peale at Santa Barbara that if we could measure some of the geodetic parameters of Mercury and the long-wavelength gravity field, we could determine the size of Mercury's core. We knew Mercury had a large core for its size because of the high bulk density. And we suspected that the core was the source of the magnetic field, but we didn't know.
There was a list of six major questions, and those questions dictated the payload. Then, we fought to keep every instrument operational, and ready to be launched at the right time, and low enough in mass to fit in the payload. There were two big challenges for MESSENGER. The first was keeping the dry mass of the spacecraft low, because we knew we had to fly more than half the launch mass as propellant for all of the flyby trajectories it would have to do and for orbit insertion. The second was to address the high temperatures the spacecraft would face because it would be much closer to the Sun than any mission to Earth or Venus and because of the surface of Mercury itself. We had to deal with solar heat, as much as 11 times that at 1 AU, and heating from Mercury's dayside. We had solar arrays that would work at high temperatures, and we kept as much of the rest of the spacecraft as possible in shadow with a sunshade. We also had to be careful with our orbit so that we didn't see the highest surface temperatures at closest approach. It was a wonderful example of how a bunch of individual experts clever in their fields could together come up with a solution in a very complicated trade space that managed to deliver a spacecraft that operated successfully in a harsh environment for four years and returned as much science as possible.
ZIERLER: The 2011 to 2015 timeframe, was that the timeframe that was initially thought to be a reality?
SOLOMON: Initially, we proposed a single Earth year of orbital observations. The mission was designed and budgeted for launch, plus cruise, plus orbit insertion, plus one year of operations. We hoped that everything would be healthy by the end of that year, that there would still be enough propellant on board so that we could manage attitude control and orbit control – and that required propellant – and that all of our electronics and subsystems would still be healthy, and that was the case. We proposed to NASA a second year, and we argued that a long list of new questions generated by the first year of observations and a different observational strategy for the orbital campaign would give us new science. They agreed and extended the mission.
Then, in the second year, we realized we had some more life left, and the Sun was going through a different phase of the solar cycle. Our mission was also evolving because the orbit was changing. It was always in an eccentric orbit to help the spacecraft radiate heat at the highest altitudes that it'd absorbed at the lowest altitudes. The complicated effect on that orbit of being close to the Sun was that the orbital parameters changed, and the sign of those changes changed during the four years of the mission. We knew that during the second two years we would get much closer to the planet than we did during the first two years at closest approach. As long as we had propellant, we could keep the spacecraft off the planet, even at closest approach. We asked for a second and final – we promised – extension of the mission for years three and four and got approval for that.
ZIERLER: In the mid to late 1990s, how much of a time commitment was it when you were the president of the AGU?
SOLOMON: It was probably a day a week. I was induced to stand as a candidate for president-elect, which involves a two-year term that precedes the similar term as president, by a nominating committee chaired by Don Anderson, and he specifically argued that it would be good to have an AGU president who was in the Washington, D.C., area. His committee also recruited a second candidate, and we ran against each other. The other candidate was at Johns Hopkins, which is still in greater D.C., but not as close as I was. I was a ten-minute drive from AGU headquarters. As an aside, the reason Don wanted an AGU president from Washington was that he was wary of the long-term executive director at AGU and wanted an AGU president who would be present enough of the time to look carefully over the shoulder of the executive director and keep the organization guided by the best interests of its scientific members. That's how I came to stand as a candidate for president-elect. But my term as president was an intensive two years. You have to recruit all of the members of AGU committees, for instance, and you've got to think broadly about the issues that are important to the Earth and space science community.
For AGU the particular concerns are dominated by publications and meetings, but they extend to outreach, education, political communication, and many other secondary activities. I didn't have to address the biggest challenges that AGU faced a few years later. My term was a little bit ahead of the move to all-electronic publications, for instance, which was a big headache. But we were already seeing some challenges to the meetings, so there were serious issues. By and large it was a fun experience and one that enabled me to know better many of my colleagues, particularly in fields somewhat distant from those in which I worked every day. I think getting to know the community was one of the best aspects of my experience.
NASA and Astrobiology
ZIERLER: I asked about astrobiology at MIT as a way to foreshadow when you became PI for the NASA Astrobiology Institute. First, in what ways had the field matured that made it more official at this point in the late 1990s?
SOLOMON: The maturing, I think, was more evolutionary. Certainly, biology was advancing in leaps and bounds, including microbiology and an understanding of biosignatures. NASA was again looking to Mars more intensively, motivated in part by the erroneous claim that evidence of life had been found in a Martian meteorite. But that work really energized the community into a very healthy debate about whether that scientific conclusion was correct, and if not, what it would take to answer it in the affirmative in the future. A lot of very important work was done to argue against that assertion that the McKay group had made in the mid-1990s. I think a lot of the NASA focus on Mars and the realization that the interface between biology and geological and planetary processes was not heavily populated by people who could easily cross back and forth led NASA to create some programs with some new names and invest some money in getting teams together to address broad questions.
Carnegie was a great place to tackle some of those questions because, just on one campus, we had planetary scientists, geologists, organic and inorganic geochemists, a microbiology group, and even astronomers discovering extrasolar planets and wondering what those bodies say about planetary processes. It was easy to put together a very broad group to pool the intellectual threads of the group effort. That field of astrobiology, which grew out of the NASA investment in the late 1990s, took a while to bear fruit because bringing all these experts together from these different fields meant that there had to be time devoted to learning the foreign language of each other's field. An astronomer could not easily talk to a microbiologist. There were too many technical terms that were second nature to one and completely opaque to the other. It took a number of meetings and some patience on the part of presenters and discussionists to reach the stage where astrobiology became a real discipline. And along the way, students and post-docs got entrained by that process. And, as is usual, they became much more expert at crossing those disciplinary boundaries than any of their senior mentors.
ZIERLER: The high-profile failures in the Mars missions at JPL in the late 1990s, I wonder how closely you followed that and if it affected your day-to-day at all.
SOLOMON: Yes. Of course, any planetary scientist follows the news of any planetary mission, and failures are not welcome to anybody in the field. That was the heyday of so-called "Faster, cheaper, better," the mantra of NASA Administrator Dan Goldin. And the failures convinced NASA headquarters and others that maybe you can't have faster, cheaper, and better all at once, you've got to pick two. First Mars Climate Orbiter and then Mars Polar Lander failed in quick succession for different reasons, which was quite embarrassing. For me personally, it meant the mission I was leading, although still in the development stage, suddenly got a lot more attention from NASA managers. Sometimes attention is good, sometimes it is not. We had to jump through a lot more hoops, prepare for a lot more reviews.
There was another planetary mission that failed a couple of years later, an APL mission called the COmet Nucleus TOUR (CONTOUR). CONTOUR was designed to conduct successive flybys of multiple comets. Early in that mission, after launch, they turned on the propulsion system, and the spacecraft exploded. Because that was an APL mission, we were asked a lot of questions as to what heritage from that mission had been applied to MESSENGER, and there was another set of reviews we had to go through. It was a very stressful period, particularly since some of these reviews were conducted by people who had no prior knowledge of our mission. Not only did we have to answer all the probing questions, we had to educate them about what we were doing and had done. Failures somewhere in the planetary program make work for all other parts of the program for a while.
ZIERLER: Did it improve the mission? Were there positive aspects of the increased attention?
SOLOMON: Sometimes. Most often not. It depended on the review. The best reviewers for our mission were technically savvy reviewers who had followed the mission from the beginning, met with us with some regularity, and pointed us to any ideas we'd overlooked, or asked us to justify the decisions we'd made, which was also helpful. The least helpful reviews were the ones where headquarters assembled administrators and others who have been managers for a long period of time, not line engineers. Those guys made decisions frequently because that was their job. But they didn't have the depth of knowledge of our mission or even of all of the possible engineering solutions to problems. Those reviews were almost worthless, but we had to do them because of the gravitas of the reviewers.
ZIERLER: Of course, you're the recipient of many awards and honors, more than we can cover here. Two, I want to talk about in detail. The Distinguished Alumni Award from Caltech in 2006. Tell me what that was like and if it provided an opportunity to reflect on what your Caltech education did for your career?
SOLOMON: It did. I was very honored to get that, even in advance of the MESSENGER mission producing data from Mercury. I think I was nominated by a couple of my former faculty members. It gave me a chance to go back to the campus and enjoy a ceremony in Beckman Auditorium – which had been constructed while I was an undergraduate – and got me a lunch with David Baltimore and Alice Huang. [Laugh] It was all great fun. It was the only alumni weekend that I've ever attended at Caltech. But of course, I had a special reason for going to that one. Caltech made me feel very welcomed.
ZIERLER: Later on, when you won the National Medal of Science, did you get a chance to meet President Obama?
SOLOMON: I did. We didn't have long discussions, but those of us receiving medals were invited to the White House for an entire morning. Initially, we got a tour of the White House. We were each allowed three guests, no more. Then, there was a photo opportunity with the President. He would come into the Green Room, and we would each get our chance to have photographs. He and I had a very brief exchange of remarks at that time. My only other conversation was when he actually gave me the medal in the East Room ceremony, and we exchanged one-sentence compliments to each other.
ZIERLER: Tell me about the decision to join the faculty at Columbia.
SOLOMON: That took a while to make. I had stepped down as the director of the department at Carnegie. I'd been in that position for 19 years. Carnegie had a rule that people in administrative positions could not continue in those positions after they turned 65. I had turned 65 in 2010, so in July of 2011, I had to step down as director, which I was very happy to do, being in the middle of a spacecraft mission. I thought I would be content just doing research as a member of the research staff there, but I got a few calls about the search for a director of Lamont. I knew very well the previous director because we had been collaborators for many years in marine geophysics. That was Mike Purdy, whose career at the Woods Hole Oceanographic Institution pretty much coincided with my time on the faculty at MIT. We did many marine seismology cruises as co-investigators, and we co-advised a number of PhD students in the joint PhD program at MIT and WHOI.
Mike had been director of Lamont for ten or more years, so I knew that position well, and I'd been on an external advisory committee to the Columbia Earth Institute, of which Lamont was a part. I got to know Jeff Sachs, who then was the director of the Earth Institute, through that process. As they were looking for a new director, I had conversations with Jeff about what I thought they should be looking for in terms of a new director without thinking about myself as a candidate. Jeff said, "Yeah, that is what I should be looking for. You should talk to the search committee and tell them your thoughts." I said, "OK, I'll talk to them." That got me an invitation to New York, and I met with the search committee.
ZIERLER: As director, did that give you opportunity to work with students and assume more of the professorial life that you left at MIT?
SOLOMON: That opportunity might have been there, but I did not take advantage of it. As director, I really devoted myself to campus issues: staff recruitment, making sure that our junior staff got their promotions in a timely way, and financial stability. And I was also running a spacecraft mission at the time. I did not do any classroom teaching or take on any students. I did recruit some post-docs who helped me with mission work.
ZIERLER: Institutionally, how important was climate change and sustainability for the Observatory when you became director?
SOLOMON: Very important, both of them. Well after the time that Lamont became famous for its work in marine geophysics and seismology, they had broadened into other fields. But particularly with Columbia's hiring of Mark Cane in the early 1980s, it set Columbia on a path of outstanding climate science. Mark and his group developed the first climate models that could predict El Niño events in the mid-1980s and realized the importance of that capability to the point that he made actual forecasts publicly within a scientific context. The stress of those forecasts helped build the field and the group. Then, ensuing recruitments of other climate, atmospheric, and ocean scientists made Lamont a real powerhouse in climate science. Sustainability was really a working theme of the Earth Institute and still is, but is a little more applied than the basic climate science. It involves resources and pollution as much as understanding the way the Earth is changing.
ZIERLER: Of course, the work never ends. For your successor when you decided to step down, what are some of the big issues facing the Observatory now?
SOLOMON: The financial one is still there. I didn't solve that one to any degree of completeness. The other one is the one I mentioned earlier, that Columbia has embarked on the development of a new academic school devoted to climate, its impacts, and the amelioration of climate change effects. That enterprise is intended to be broadly cross-disciplinary, anchored in the expertise in climate science at Lamont and extending to all relevant aspects of human existence on the planet, including social justice, architecture, urban planning, agriculture, and everything else you can think of that's related to our environment and how that environment is changing.
ZIERLER: How do you see the Observatory contributing to these extraordinarily complex questions about climate change and how humanity is affecting it?
SOLOMON: I think they're broad opportunities. The challenge will be to maintain the strength of the core scientific expertise that resides at Lamont, while at the same time providing the links and facilitating the communication that enables the science to inform the mitigations, the adaptation ideas, the ways that society, both regionally and globally, will be meeting the challenges, not all of which are understood, posed by our changing climate and changing environment.
ZIERLER: To wrap up, I'd like to ask a retrospective question, then we'll end looking to the future. To go back to when you were an undergraduate at Caltech in that formative moment where you were given an opportunity that far exceeded your knowledge at the time, what did you learn at Caltech in its approach to the science and education that has stayed with you ever since and that put you on a path that allowed you to achieve all that you have?
SOLOMON: I think scientific research in any field is one of learning new ways of answering questions. Caltech was very good at teaching students and me how to figure out ways to answer questions and to be unafraid of bringing in new fields and disciplines, learning new ways of looking at different sides of a problem. In a way, planetary science, as an example, is much broader than most of the disciplines of Earth science because when you encounter an entire planet, you find yourself looking at all of the phenomena that are going on. If we ever find a planet with its own biology, that will be triply true. But you can't go to a new planet without understanding geology, geophysics, atmospheric science, space physics, the evolution of our star, the way planetary systems form, and the interaction of all of these components. A lot of the traditional fields of Earth science permit our scientists to stay fairly narrow within the comfort zone of a single discipline. But planetary science, by its very nature, has no such comfort zones. If you want to address challenges at planets, you have to embrace everything that's going on in the physics, chemistry, and history. I think the field as well as the institution taught me to be comfortable learning lots of new things along the path of my research career. It started me on that path and gave me a good push. I've enjoyed applying those lessons for many years.
ZIERLER: Finally, now that you've shed yourself of administrative responsibilities and can focus only on what's interesting to you, for as long as you want to remain active, what are the big areas of research you want to take on?
SOLOMON: I'm still very interested in our solar system. I'm still very interested in the evolution of our planet and what we're doing to it. But I feel less comfortable leading research toward understanding climate change and environmental change. I want to stay active in the exploration of the solar system with new spacecraft missions. Particularly, I'm still very interested in answering some of those old questions about Venus. Venus is the planet in our neighborhood that offers the most to inform us about how planets like our own work.
ZIERLER: More so than Mars?
SOLOMON: More so than Mars. Mars is only a tenth of an Earth mass, lost its atmosphere very early, and lost its magnetic field very early. It has many parallels, but not as many as Venus. Venus is harder to study than Mars, it's harder to land on the surface, it's harder to survive very long on the surface, and it's a much bigger object to try to get one's arms around than Mars. But Venus may have had three or four billion years of history that were much more Earth-like than what we see today. We don't know.
ZIERLER: Including life, perhaps.
SOLOMON: Including life, perhaps. That's right. I think it's got more to offer. I know there are some missions in the works that I'm following closely. I'm involved in planning some other mission concepts down the road.
ZIERLER: Given that the decadal just came out, are you satisfied with what it says about Venus?
SOLOMON: I think so. The flagship missions it's identified are well defended; I have no argument with either a Uranus Orbiter and Probe or an Enceladus Orbiter and Lander. I think those are great missions: Uranus, because it's a type of planet that we haven't ever sent an orbiter to, an ice giant; and Enceladus, because of all the candidate ocean worlds, it's the only one where we can sample what probably is the ocean itself coming out of the plumes at the South Pole. But there are opportunities to go to Venus beyond the missions that were just approved last year in the competed mission lines at NASA. I think I see a big uptick in interest in Venus as a possible abode for past life, but mostly as an object lesson in a different outcome for an otherwise Earth-like planet.
ZIERLER: On that note, it's been a great pleasure spending this time with you. I'm so glad we were able to do this, to capture your perspective and insight over the course of your career, and to get the stories from when you were an undergraduate at Caltech. I'd like to thank you so much.
SOLOMON: Appreciate it, David. Thank you.
[END]
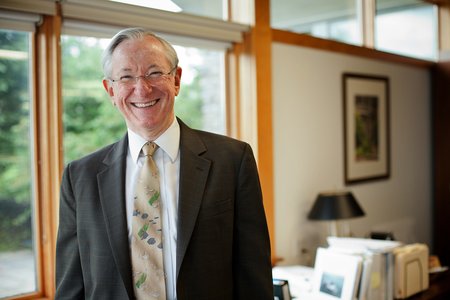
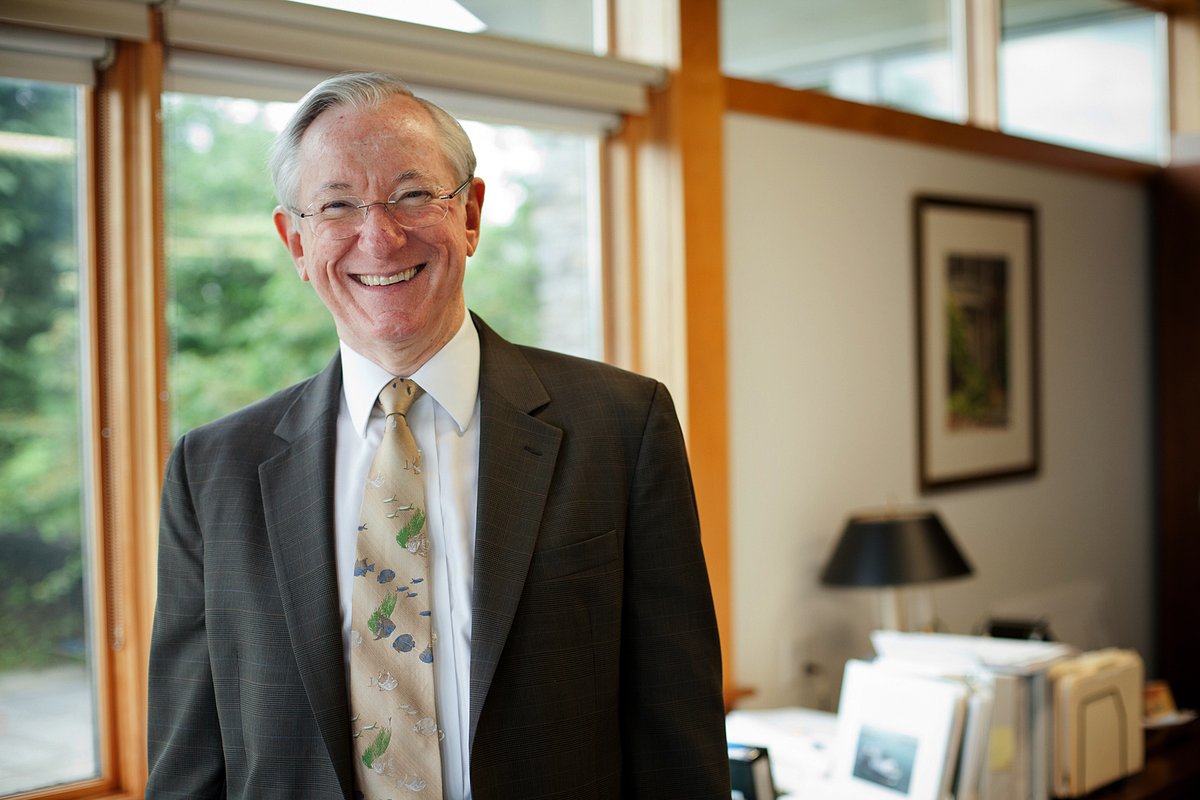