April 6, 2022
The lithosphere - comprising Earth's outermost rocky crust and the top portion of the upper mantle - has been the object of Seth Stein's study for his entire career. Through innovative applications of space geodesy, marine geophysics, and seismology, Stein has investigated the nature of plate boundary processes and deformation. More specialized projects derived from this research include earthquake hazard mitigation and developing mapping techniques and policies to minimize destruction and to protect life.
Stein arrived at the Seismo Lab in 1976 after completing his undergraduate degree in Earth and Planetary Sciences at MIT. His thesis, completed under the direction of Hiroo Kanamori, examined seismic vibrations and waves, the spreading of mid-ocean ridges, and an analysis of the Indian Ocean as a plate motion system. After a postdoctoral appointment at Stanford, Stein joined the faculty at Northwestern in 1979. Stein is the recipient of the James B. Macelwane Medal from the American Geophysical Union, the George P. Woollard Award from the Geological Society of America, the Stephan Mueller Medal from the European Geosciences Union, and the Price Medal from the Royal Astronomical Society.
Interview Transcript
DAVID ZIERLER: This is David Zierler, Director of the Caltech Heritage Project. It's Wednesday, April 6, 2022. I am delighted to be here with Professor Seth Stein. Seth, great to be with you. Thank you for joining me today.
SETH STEIN: My pleasure.
ZIERLER: To start, would you tell me your title and affiliation?
STEIN: I'm Seth Stein, Deering Professor of Geological Sciences at Northwestern University, and I'm also an Associate of the University's Institute for Policy Research.
ZIERLER: How big a program does Northwestern have in seismology and geophysics?
STEIN: It oscillates depending on the hiring. We've had two of our four geophysicists retire in the last two years, and we're bringing new people on board. I have about five PhD students working with me, then Emile Okal has had a series of good ones. We've had four faculty, two have retired, then another new person has come in place. About four to six, depending on how you define it. By Caltech standards, one would say probably six because at Caltech the area of study of the physics of the Earth's interior through experimental mineralogy, laboratory experiments and theory, is housed in Seismo Lab.
ZIERLER: In your overall research agenda, of course, there's seismology, there's planetary science, there's geophysics. What are you at the end of the day if you had to identify as one?
STEIN: I would say more than anything else, I'm an earthquake seismologist. But I and coworkers have done things like develop the standard models used to describe the rates of plate motion. If you read, "The such-and-such plate is moving at such and such speed," those will be our numbers. But I came into that from the standpoint of seismology. We also do a lot of work on earthquake hazards. You've probably seen these earthquake hazard maps of California. We have a major effort to understand how well those maps actually work in California and worldwide and how to improve them. We basically study things that are directly related to earthquakes, things that are related to plate motions and how they cause earthquakes, and things that are related to the consequences of earthquakes for society, hence my Institute for Policy Research tie.
All About the Lithosphere
ZIERLER: Where does the lithosphere play into your research?
STEIN: Everything we do is the lithosphere, which is the outer solid shell of the Earth. My interest has always been in that part of the planet. I do not have direct research with the deeper interior.
ZIERLER: What are some of the ongoing debates about the lithosphere, and what is some of the settled science?
STEIN: Here's the problem. The lithosphere is something which cannot be uniquely defined. It's the outer shell of the Earth, and we can define that in three ways. We can define it in terms of its mechanical properties, which is probably the best. It's the rigid outer shell that floats above the weaker asthenosphere, the softer shell. Second, it can be defined chemically because it's chemically distinct from the rest. Third, it's thermally different from the rest because it's the cold outer layer of the Earth where temperatures cool. All those things are closely related entities, but depending on how we define the lithosphere, it has different properties and different thicknesses. The existence of this entity is settled science. Plate motions are settled science, much more settled than when I was in school because with space-based geodesy, we can actually measure the motions of plates very accurately. We know plate tectonics is very settled. We know what the lithosphere does is essentially settled science.
I would say the unsettled science questions include when the lithosphere formed and started acting the way it does today. We've been looking back about a billion years to big structures in the Midwest, like the stuff you see behind me on the image there. We don't know when the lithosphere formed and started acting the way it does. We don't know how mechanically strong it is, and that makes a big difference in terms of earthquake physics. From that, we in turn don't understand what controls how often large earthquakes happen. We have a fairly large effort in that here in terms of how the energy is stored in the lithosphere. What we don't understand at the deepest level, you might say, is why is it of our three neighboring planets, Earth, Mars, Venus–you'll sometimes hear that called the Three Bears paradox - Earth is just right, Venus is too hot, Mars is too cold.
When I got into this business, in 1971 when I hired on with the seismology group at MIT as an undergrad, we had not yet imaged the surface of Venus, and we were all convinced it would look and behave very much like Earth. We also figured Mars would probably behave just like Earth, because plate tectonics is the theory of how the lithosphere works. It turns out Mars and Venus–Venus is almost identical in size to the Earth; Mars is a bit smaller–do not behave the same way. And we do not either understand very well how they're behaving, although we know it's not like on Earth, we know they don't have the kind of plate tectonics, and we don't know why they don't behave that way. It not like quantum mechanics, which presumably works on other planets. But if you go to Mars or Venus, plate tectonics doesn't work. That's where we are.
ZIERLER: What role does geodesy play from space in your work?
STEIN: Incredibly. I was director of UNAVCO, the National Scientific Space Geodesy Consortium, for a couple of years. At Northwestern, one of our group's biggest efforts was to build a global plate motion model describing plate motions over the past few million years geologically, and to compare it with the space-based geodetic data. We got involved fairly early on with the development of space-based geodesy. Strictly speaking, the subject goes back to the ancient Greeks - Eratosthenes and Alexandria. But modern space-based geodesy, mostly GPS, is recent. We've used that in a couple of ways. It lets us look at plate motions taking place today, not over millions of years, but seeing that the Atlantic is 20 millimeters wider than it was last year. It lets us look at deformation within the lithosphere. We can see the lithosphere storing up energy for earthquakes, then releasing it. One of our big efforts about 15 - 20 years ago was to look at places in the continents and see if energy was being stored up for future earthquakes. To our amazement, it turns out, at least in the area of the New Madrid Seismic Zone in the central U.S. that has had big earthquakes, there's no sign of any energy being stored up there. That's led us to look at a whole new way of thinking about how earthquakes and plate interiors work. The answer about how important geodesy is, essentially, totally. [Laugh]
ZIERLER: How do you separate out in your research geophysics in a marine context and geophysics in a terrestrial context?
STEIN: I don't. Part of that is twofold. One of them is, my wife comes from a marine geophysics background. I met her at Caltech. We're pretty sure she was either the first, second, or third woman to get a bachelor's degree in geophysics from Caltech. We work very heavily with marine data. We've developed what are now standard models for how the depth of the ocean increases with the age of the seafloor, and that's actually not as arcane as it sounds because that reflects the cooling of the plates as they're formed at the mid-ocean ridges. That's why they're ridges, they're high, they're hot. As the plate moves away, it cools. We've developed a way of characterizing that cooling which researchers use heavily. We recently had a large effort to look at passive margins. Those are continental margins like the East Coast, when a continent splits apart. A lot of the history is buried in the rocks under the continental margin, so we have a fairly large effort looking at that, trying to account for the volumes of rocks that are produced there, and in turn, about the temperature structure. This is a very important topic for how continents break up. It's also important, of course, for the generation of oil and gas deposits on continental slopes, but we don't do that part.
ZIERLER: Earthquake detection and mitigation. First, on the policy side, what are the relevant agencies, and what levels in government are most important for earthquake mitigation?
STEIN: There's complex interaction, as always in the US, between the federal, state, and local governments. In some abstract sense, FEMA is in charge of the federal mitigation component, but the federal government does not have the power to prescribe building codes for seismic resistant construction. It makes recommendations to an outfit called the Building Seismic Safety Commission, but that, in turn, is propagated out to states and local governments, and they decide what standards they will apply for earthquake-resistant construction. There are two challenges in that. One is to try to decide how often large earthquakes will happen, where they'll happen, about how often they'll happen, and how much shaking they'll do. We're involved in that side. The other part, which is equally important, that we're not involved in, is designing structures and buildings. Structures are things like dams, tunnels. If people don't live in it, it's a structure, not a building. But the earthquake engineers are very interested in how you build things to stand up to earthquakes. Essentially, building codes are adopted at the state level. At that point, some states, like California, have very strong entities within the state government that do it. California's one of the better ones because of the earthquake hazard. The California Geological Survey has a lot of expertise. There's also a lot of expertise in the academic world. On the research side, there's expertise in the U.S. Geological Survey. The states have regulatory responsibilities, and they also have, in some cases, research entities. It's kind of complicated.
ZIERLER: Onto the science part. For earthquake mitigation, if we can imagine that the gold standard at some point might be, and you'll tell me if this is even possible, the way the meteorologists can tell us ten days out, "There's a hurricane brewing in the Atlantic, and it might become a real problem." There's a tremendous amount of lead time. The worst-case scenario is, we have no warning whatsoever, there's a big shake, and there's tremendous damage and lives lost. Where are we right now in seismology and earthquake mitigation on that scale for early warning?
STEIN: We can make forecasts on the scale of probably no better than 100 years, that this place, based on plate motions and earthquake history, has energy that has to be released and is more prone to have earthquakes. We're putting a lot of effort into trying to do better at that. That's what I've been doing the rest of today, working on a manuscript. But we still don't know how to do that well, and we have a fairly strong suspicion that we may not be able to do much better because we believe that these are chaotic systems. Let's start at the San Andreas Fault. Suppose centimeter-sized pieces of the San Andreas Fault move a little every day. One out of every ten of those moves a tiny bit more. Of those, one out of ten moves somewhat more. The Earth doesn't know which of these is going to grow into an earthquake. Now, to be fair, the meteorologists have the same thing. They cannot tell you, "This patch of the Atlantic Ocean is going to give rise to a hurricane."
What they can tell you is that "Under these temperature conditions, there's a reasonable change that something within there will start." Remember, hurricane forecasting is not forecasting the development of a hurricane, it starts when the hurricane forms and starts to move and then the models make pretty good predictions about which way it's going to go, what it's going to do, how big it's going to get. But they can't predict the occurrence of it. In fact, there's an interesting analysis. They can't tell you why there are, for example, 20 named storms a year in the Atlantic as opposed to 2 or 200. They have no idea why that is. We can make very, very long-term earthquake forecasts basically in terms of the energies involved. California's a more dangerous place than Utah, which in turn is more dangerous than Tennessee, which in turn is more dangerous than Chicago. We can do that. And that's not quite as useless as it sounds because buildings have long lifetimes. When engineers design a building, their goal is to make sure that over the useful life of the building, it won't collapse. We've had a spectacular series of failures at efforts to predict earthquakes on any short-term basis.
Then, we have another thing, which is very much like what they do with the hurricanes, earthquake early warning. There, the earthquake has happened, and they're warning you that seismic waves, which they know exist, are on the way. Tsunami warning is a longer timescale version of the same thing. You know that tsunami is on the way, and it will arrive in Honolulu two hours and 24 minutes from now.
In contrast, we believe that the nonlinear chaos theory aspect of earthquake faulting probably will prevent us from doing any short-term predictions, and that's based on the hypothesis that if the Earth doesn't know when the next earthquake's going to happen, it can't tell us. A huge amount of effort has been put into looking at whether anything changes an hour or two or a day before the earthquake. So far, nothing has been observed. That would lead one to believe that this probably can't be done. Of course there is Arthur Clarke's law about things not being impossible. But no one I know can see any reason to believe that this is possible, which is not the same thing, of course.
ZIERLER: This fascinating notion, whether the Earth itself knows about an earthquake. What's the mainstream thinking right now in the seismology community?
STEIN: The Earth almost certainly doesn't know. If nothing unusual happens before an earthquake, it's because there's nothing in the Earth that's any different from any other day. The day the next San Francisco earthquake happens, the question is, was anything unusual going on in the faults the day before? One hypothesis is, no, nothing unusual was going on in that fault that hadn't been going on 20 years earlier or 20 years from now, and there's nothing different about which of these tiny perturbations happen by a series of implausible events to grow into a big earthquake. If there's nothing unusual, the Earth can't tell us.
ZIERLER: This idea that, for example, in California, we're due for the big one based on some idea that these are cyclical, that does not resonate with you?
STEIN: It does, but the uncertainties are colossal and not usually discussed. This is one of the projects we're working on. If you actually look at the uncertainties, they can be factors of two or three. The recurrence interval for earthquakes on a piece of fault is something like 200 years plus or minus 150 years. If it's been 200 years, OK. If the Hayward Fault started fracturing now as we speak, I wouldn't be the least bit surprised. If it doesn't happen in my lifetime, that wouldn't surprise me either. The variability is so enormous, and the models we have are lousy because they're very unrealistic. They don't account for why we get this huge variability. The standard deviation is usually about two-thirds, at least, of the mean.
ZIERLER: The metaphor of, for example, a spring being wound, and it's just wound so tightly that at some point it's going to blow, we don't have the means to measure that pressure to have predictive ability to figure out when that might happen?
STEIN: Wrong and right. We do have the ability. Geodesy lets us measure the strain accumulating on a fault very well, and we know it by now. And we know when the last one was. But the system is very, very far from periodic. We see the strain accumulating. But if we just look at the history of earthquakes on the San Andreas Fault, for example, the variability is so enormous over time that you can get probabilities that vary greatly from any calculation. We've put a lot of effort in trying to improve that. We have a model that we hope is better that tries to look at this in a more realistic way by actually looking at that strain accumulation. It seems like a lot of this variability is baked into the system. There's a high stochastic element to it. You cannot describe this without accepting the fact that the distribution of times between earthquakes is governed by some sort of probability density function, and the spread in there is really big. It's not that the argument is false, the strain is accumulating, the spring is loading, but it's highly variable.
ZIERLER: Just as a snapshot in time, what are you working on currently?
STEIN: At the moment, we're doing a couple of things. One of them is trying to understand why the earthquake hazard maps for California over-predict the amount of shaking we've observed over time. We have a project called CHIMP, the California Historical Intensity Mapping Project, where we've looked at 160-some years of shaking data all over California and compiled it, then we've compared that to the hazard maps that are used. Those maps systematically over-predict the amount of shaking that's been observed, and we've seen the same phenomenon in Italy, France, Japan, Nepal, etc. We know that the hazard maps we're using now are too high. We're trying to understand why that is and how to improve those.
Second, we're trying to build better models of earthquake recurrence, to try to include the fact that earthquakes are not uniformly spaced in time but will sort of cluster for a while then un-cluster. That is actually a Seismo Lab discovery, by Kerry Sieh starting at Pallett Creek. That's what drove our thinking on recurrence being highly variable. We've built models that have that property. Right now, when you hear statements like, "We're overdue for an earthquake," those are based on the idea that after one earthquake, all the strain on the fault is released, then it starts rising again. We can do a somewhat better job by recognizing that not all the strain is released, and we call that LTFM for long-term fault memory.
Third, we also have a fairly large effort to understand how continents rift, and a lot of that has been looking at the big rifts in the center of the US that failed to split up North America, so they're snapshots of what happens as a continent tries to split. We've been looking at that in a lot of detail, then comparing the results to offshore regions.
The fourth big project is looking at huge compilations of earthquakes around the world. Our simplest view of earthquakes- they are all slip on a fault. When you look at the results of the seismic analysis, we're seeing things that are a lot more complicated than that. We call the slip motion double-couple motion, and the other one's called non-double-couple motion. We have a huge effort involved in trying to understand if that's real, or an artifact of the math that's used to produce these solutions. We have a pretty strong suspicion that it's an artifact of the math. We've looked at data from three global seismic networks and four regional seismic networks, then we're doing numerical simulations to understand how these artifacts come about.
From MIT to Caltech
ZIERLER: Let's go back and build up the historical narrative. What years were you at MIT as an undergraduate?
STEIN: '71 to '75.
ZIERLER: And were you interested in seismology and geophysics at that point?
STEIN: Yes. I came there planning to do applied math. The MIT freshman experience is similar to the Caltech one. You take physics, which is like high school, only harder, math, which is like high school, only harder, chemistry, which is like high school, only harder. For another course, I took geology, and I was absolutely fascinated by it. This was the very early days of plate tectonics. So in the first class, I walked in the door and was taught about plate tectonics. Five years previously, I wouldn't have been. I was absolutely fascinated by the stuff. I hired on that summer with the group working with the data from the seismometers in the Apollo missions. Apollo was still ongoing, there was still one mission left to go, 17. But at the time, we also thought there would be 18, 19, and 20. These were other planned missions, which were canceled to provide more money for Vietnam. I hired on with the seismology group and started working there summer of my freshman year, and I worked with them all the way through college. Then, I had an absolutely fantastic seismology class from one of the great modern seismologists, Keiiti Aki, Aki was one of the three or four top seismologists of the previous century. I was influenced by him and his class, then of course, Kanamori's class at Caltech. I also got very interested in plate tectonics, plate motions, and thermal evolution of the lithosphere.
ZIERLER: Did MIT have an analog to the Seismo Lab? Was there a separate institute?
STEIN: No, they didn't. It was just part of a department. In terms of number of warm bodies, it was roughly comparable, but it wasn't broken out as a separate entity. The building was a 22-story tower, and seismology was "the fifth floor." [Laugh] It had no formal existence beyond that. The overspill I was in was in one of the old rad lab buildings, building 24, where the lunar seismology team was housed.
ZIERLER: When it was time to think about graduate school, was the Seismo Lab at Caltech the place to go? Was that the advice you go?
STEIN: No, I would say I looked at three places, each of which had strengths. One of was to stay at MIT. My advisor wanted me to stay. The graduate admissions director was very nice, he'd been the chief scientist of NASA, and had this very thoughtful conversation with me. He said, "You're at the top of our list, but you should go somewhere else because you'll grow more as a scientist." I took that to heart. I thought it was entirely sensible. The two other places I looked at were Stanford and Caltech. They each had strengths. Stanford was better at plate tectonics. Caltech, at the time, was not that good at it. Stanford also had the absolutely superb exploration seismology, which is the kind of seismology you use to look for oil and gas, or geologic questions in the lithosphere. Each of those groups had different personifications. The Stanford one was personified by Allan Cox, who invented the paleomagnetic time scale we use to date, changes in the earth's magnetic field that we used to develop plate motions, and John Claerbout, who's the world's greatest exploration seismologist, another absolutely brilliant guy.
At Caltech, it was clear to me, if I went there, I would want to work with Kanamori. I don't recall particularly why I chose one over the other. I was equally torn by both and found both utterly fascinating. I didn't flip a coin, but I could've. I had a Hertz Fellowship, so I wasn't dependent on any of their funding. Both places made me nice offers, and the Hertz Fellowship was such a good deal in those days that you'd turn down the NSF Fellowship to take the Hertz. They've switched that around now, but in those days, you did. I don't know particularly why I made the choice I did. I did a Stanford post-doc later on and liked Stanford very much, too. And I was drawn, like a lot of East Coasters, by the adventure of going to the West Coast, which in those days was much more of a schlep and less common.
ZIERLER: When you got to Pasadena, how separated were you from campus? How much was your research world at the Seismo Lab itself?
STEIN: Just as I was in the first plate tectonics generation, I was in the first generation that was not in the old off-campus lab. I'd never even been in the old lab. I'd heard these mystic stories about it, but I was never there.
ZIERLER: You were already on campus at that point.
STEIN: I walked into South Mudd, and that was where I was.
ZIERLER: Did you have an appreciation from the older generation of the decisions leading to abandoning the separate house and bringing it on campus?
STEIN: No, I did not. It was established. I knew some things about the new building. If you go into the faculty-side offices, the wall of the building is that thick, and it's a shear wall that holds up the whole building. Short of bombing the place, it wouldn't come down because they didn't want the bad press of having the Seismo Lab destroyed in an earthquake. And that was personally done by Clarence Allen, who personally supervised the plans, I've heard. All this took place before I got there. This was one of the things that made me stand out from some of the other people is that I was never in that culture of the old lab. Just hadn't ever been there. Never been in the building and knew nothing about it. [Laugh]
ZIERLER: What was the process of determining who a thesis advisor would be for you?
STEIN: In my case, I simply decided. Since I was completely funded by the Hertz Foundation, it didn't make any difference. I didn't need money from anybody and didn't need any direct involvement from anybody. I had a sort of unique experience, which I very much valued. I did not have a thesis supervisor. I just worked on what I wanted. I certainly had a thesis advisor in that I talked to Hiroo Kanamori very, very frequently, and he was incredibly giving of his time given that I wasn't working for him. I never wrote any papers with him, but I learned a lot from him, and I would go in and talk to him all the time. I was in a somewhat anomalous situation. I had my own funding, wasn't under the control of anybody, and as a result, set a record for the minimum PhD time there, although I think another person also got out in three years. I could just work on whatever I wanted. My thesis had three different parts on wildly different topics because they were just things that interested me. I'm really very grateful to Kanamori for the amount of time he was willing to just talk about arcane questions. I would also run what I was doing by him, but he never had any direct control over it.
ZIERLER: What was Kanamori doing at that point? What was his research on?
STEIN: Until slightly before that time, mostly what people used in seismograms was the times the waves arrived and sometimes the polarity, this way or that way. Kanamori was among the leaders in trying to use the full waveform, to actually match the wiggles using more sophisticated models of the Earth and more sophisticated models of the earthquake source. There were two people like that. These two people who pretty much made the lab on the earthquake side, I'll leave out Don Anderson's role in deep earth structure, were two waveform modelers of enormous distinction, Don Helmberger, who worked on body waves, and Kanamori, who worked on surface waves. Surface waves are generated by bigger earthquakes, and Hiroo was also interested in their tectonic meaning, which made him very unusual at the lab. He was really interested in what these earthquakes told you about the subduction process. Of course, that came from having grown up and been educated in Japan. He really had enormous breadth of knowledge and interest.
There used to be a joke, I think it was by Bob Geller, that we were supposed to come up with some topic and force Kanamori to admit he didn't know anything about it. I don't think we ever succeeded. Bob, at one time, talked to him about the generation of the geomagnetic field in the core, and Hiroo started offering his views as to how this worked. [Laugh] He was interested both in earthquakes as a physical phenomenon and the development of new technologies to study them. And those have become very, very standard. He had a wonderful combination of different kinds of skills, the ability to work with and see things in seismograms, the ability to conceptualize the underlying physics and math, and the ability to conceptualize the geology. The ideal seismologist would have all three of these attributes. Most good ones have one, a few have two. He's probably the only one I've known who had all three.
ZIERLER: What were some of the big debates when you first arrived at the Seismo Lab? What were the schools of thought that were debating the big issues?
STEIN: The big question was whether earthquakes could be predicted. It wasn't that much of a debate in the lab, and as near as I could tell, most of the people really didn't think it was possible, but there was so much money floating around, they took it. I remember asking Hiroo, "What do you think the probability of successful earthquake prediction is?" He gave me this big grin and said, "Zero." A lot of them thought it was silly and wasn't going to work, but there was a huge national push. The geo community had gotten the attention of the federal government, which poured money into this. Huge amounts of money were sloshing around, so they took some. Scientifically, one of the biggest questions to me was one where Hiroo made a very profound observation. If you added up the amount of slip in big earthquakes in Japan–and by then, we already had reasonable estimates of plate motion–you could tell that the earthquakes were only soaking up a small fraction of the motion.
The question is, did that mean that you were simply overdue for large earthquakes, or did it mean that a lot of the motion on the faults did not come out in earthquakes, called aseismic slip? Hiroo was very involved in that and got it right. A question I'm not sure he got right was the question of whether there are differences in mechanical behavior between earthquakes in the continents and those that are not, plate boundary earthquakes versus plate interior earthquakes. There, I don't think he and Don Anderson got it right. As near as I can tell, there's actually no difference. Based on some fairly marginal data, they concluded that you could actually see differences in the amount of stress released. I did some analyses and concluded that there wasn't. I was never able to convince Hiroo of this, but I did a bunch of simulations showing that you couldn't tell the difference, and those actually became figures in my seismology book. I'm not complaining about it. We've now confirmed this with "big data" approaches some of my people are doing here. With thousands of earthquakes, you can do stuff like that.
For the earthquake prediction question, a related one is whether there are changes in Earth's physical properties before earthquakes. Is the Earth telling us there's an earthquake coming? The group at Columbia University at Lamont thought they'd observed such changes. Kanamori did a very elegant experiment because you don't know when the earthquake happened, a lot of what appear to be velocity changes can simply result from errors in timing and location. Kanamori came up with this idea of recording quarry blasts, so you know where it happened and when it happened. Takes out that uncertainty. When he took that out, all the velocity changes went away. I've always thought that was a particularly elegant experiment.
A related question was about the San Andreas Fault. We thought we knew the rate of motion between the Pacific and North American plates. If you look at the amount of motion that accumulates on the San Andreas Fault, that's about 36 millimeters a year, whereas at the time, we thought incorrectly that the opening rate of the Gulf of California, which is the same plate boundary, was 50 millimeters a year, so about 15 millimeters a year of that motion was missing. At the time I was at Caltech, there was a lot of discussion about this, and it was termed the San Andreas discrepancy. We looked into this once I got to Northwestern, and it turned out most of that motion wasn't missing, it was just never there in the first place.
Another big question, which is still unresolved, that was drawing a lot of interest at the time, is called the San Andreas paradox. From what we thought we knew about the strength of rocks, there ought to be enough friction on the fault to generate high heat flow anomalies. That meant either that the rocks were a lot weaker, or that it was being lubricated by water flow, so you'd sort of expect the San Andreas Fault to look like Yellowstone or something. This San Andreas paradox was a heavily researched question. Are there strong faults and weak faults, or is there something basically wrong with the paradigm? And that gets into an overall question we still don't understand, how strong the Earth's faults are relative to earthquakes. We can measure the strength of rocks in the laboratory, and that's very strong. The standard unit is bars, one atmosphere of pressure is a bar. The strength of rock is kilobars. The stress changes in earthquakes seem to be on the orders of ten bars. The question of how strong the lithosphere was then and is today a big question.
ZIERLER: Was the Seismo Lab considered an intellectual center? In other words, would senior scholars come there for seminars to present research and things like that?
STEIN: I think so. Caltech has the Fairchild Scholar program. Two Fairchild Scholars had a lot of influence on me. One was Kevin Burke, who was one of the earlier discoverers in plate tectonics of the continents. The first paper I wrote at Caltech came out of some discussions in his class, and I said, "If Africa is fixed with respect to the mantle," which was his argument, "then all the ridges around it would have to move away from Africa." I had this idea that we'd be able to identify results of this in magnetic anomalies, so I wrote a paper about that. Also, Hiroo Kanamori was part of this network of senior Japanese geophysicists. One was Seiya Uyeda, one of the founders of marine heat flow, who became a pretty good friend. They were all together at the University of Tokyo. Uyeda came to us, and I learned a lot from him. We had other Fairchild Scholars visiting, and we had various Kanamori associates from Japan visit us. Yoshio Fukao, a post-doc then, would go on to become the director of the Earthquake Research Institute and one of the best seismologists in Japan. A bunch of the other Kanamori students came over.
Instruments and Computers at the Seismo Lab
ZIERLER: What were the instruments at the Seismo Lab that might've served as a magnet to outside researchers?
STEIN: There were two kinds of things. There were experimental high-end seismometers out at the old lab. They kept that facility. Those did not really draw outside researchers. Also, as part of the Cold War, the US government established programs to monitor Soviet nuclear tests. One of the things that came out of that was the World-Wide Standardized Seismographic Network. Prior to the 1960s, no seismometers were standardized worldwide. Caltech had one of the few collections in the country of all the data collected from that network. It was on microfilm, but it filled up a large room on the first floor of South Mudd, and was what drew me. I could study earthquakes in the Indian Ocean from the stations around the Indian Ocean, stations in Pakistan, India. All that was on the first floor, and you could just walk in and use it. There was also a large archive of Southern California local seismograms that I never worked with.
ZIERLER: What roles did computers play in those years at the lab?
STEIN: At the time, Caltech was one of maybe three or four places in the country that had full collections of the World-Wide Standardized Seismographic Network. Computers were important, but even more important was the analysis software. At the time, we were still running stuff on punch cards. You'd submit your punch cards, come back two hours later, see if it ran. The outcome came out in pen plotters, where mechanical pen would move around on a plotter. [Laugh] Data was stored on disks, nine-track tapes that were about the size of large frisbees and had to be mounted at the computer center.
That part was pretty primitive by modern standards. The computing power on campus was less than an iPhone or something. What was really important was that particularly between Kanamori's group and Helmberger's group, they were developing programs, waveform-modeling codes. Again, pre-internet, you just didn't share programs around the world. These were boxes of punch cards. If you wanted them, you had to make your own copy of this stack of punch cards. You had no easy way of transferring that information to anyone else. You just didn't exchange software in those days.
In fact, to give you an idea how funny this was, my office had file cabinets, and on top of them were boxes and boxes of punch cards. One day, I came in, and there were punch cards all over the floor. My whole career at MIT, we stored boxes of punch cards with rubber bands around them, and that was considered a semi-permanent storage method. [Laugh] In Pasadena, the smog was attacking the rubber bands, so after about a year of exposure to California smog–they broke. With punch cards, if there was the most minor thing wrong with your program, you'd find out about it three hours later and have to go back and do it over again, submit this box, do something else, then come back. In my second to last year, they got the first of what were called mini computers, the size of a file cabinet or two. Far less power than even an early Mac. But it was the first one you didn't need an account for, so you'd use those all around the clock. I saw a lot of sunrises over the San Gabriel Mountains when I was in grad school because you'd work at night if the computer was free. [Laugh]
ZIERLER: What were the topics that ultimately became your thesis research?
STEIN: Threefold. One, was the one closest to Kanamori, and is kind of arcane. The Earth's longest vibrations can be viewed as standing waves or normal modes of a solid object. I was interested in how to describe their generation by very, very large earthquakes. This involves some fairly heavy-duty math, which fortunately, someone had done for us because you could crib it from the quantum mechanics literature. Bob Geller and I were able to describe seismograms from the great Chilean earthquake of 1960 and the great Alaskan earthquake of 1964. That enabled us to say a fair bit about the earthquake sources. At the time, those two earthquakes had been studied over and over again. Remember, this was 1976, and those earthquakes were in the 60s. We didn't have a huge earthquake that big, almost magnitude 9, until 2004, when we had the giant Sumatra earthquake. Then, Emile Okal and I exhumed all this old stuff on punch cards and found a lot of neat things about the earthquake that we hadn't known before, but that came later.
The second part was to look at mid-ocean ridges and how they spread. When you learn beginning geology, you're told that it's always symmetric, the same amount of crust is added to either side of the ridge. That's actually not true, and there are pretty substantial differences in some places. I spent a lot of time documenting that phenomenon, then trying to understand how that relates to the way the ridge works. Then, I tried to understand the following. When you look at a mid-ocean ridge, like the Mid-Atlantic Ridge, you'll see ridge segments that are offset by transform faults. Those are at right angles. The question is, why does it develop that particular pattern? What is it in the energetics of the system that does that?
Two of the three projects were very un-Seismo Lab sorts of topics because they were heavily plate tectonic at a time when they didn't do it. But since the Hertz Foundation said I could do whatever I wanted, they were quite happy with it.
The third part was to try to understand how the Indian Ocean worked as a plate motion system. Emile Okal and I studied earthquakes some people in the middle of the Indian Ocean, and in retrospect, it turned out that India is really split into two plates, India and Australia. We studied a lot of earthquakes from the Indian Ocean. We were just pulling that data. That was what made it so great. We went down to the first floor, went to a drawer, and pulled it out. Go to Pakistan on this day at this time, pull out the seismograms, and look at them.
ZIERLER: Was there a lot of field work for your research thesis? Or it was analyzing data?
STEIN: Some people had field projects. Mine had absolutely none.
ZIERLER: Where was the data coming in for you?
STEIN: We were benefitting from all these people around the world who were running the seismometers and sending all this data to the National Geophysical Data Center in Boulder. But I didn't actually do any field work on it. Other people had field projects, but of the three of us who worked most closely together, Bob Geller, Emile Okal, and I, none had field projects. I helped out on some other people's field projects just for fun, but I didn't personally have any field projects.
ZIERLER: What were some of the principal conclusions in your thesis research?
STEIN: Most important is, that the Indian Plate was splitting up along the middle. Later, at Northwestern, we were able to show that India and Australia were distinct plates. I didn't get that right in my thesis, but Doug Wiens, one of my PhD students, got that right when we followed up on it here. Second, we concluded that there were systematic patterns as to which side of a spreading center spread faster. We tried to attribute that to the motion of the ridge over the mantle. That idea keeps getting rediscovered every ten years, then people say it hasn't held up. The pervasiveness of the patterns is real. I still can't think of a better explanation, but I would say it's not conclusive. But we had the advantage that we were the first to both point this out and document it, and that was kind of gratifying.
The other one was this ability to describe an effect like the way in physics, if you have atomic spectra, and you put a magnetic field on it, one of the lines splits. In fluid mechanics, if you spin a pan of water, waves go around one way faster than the other way, depending on whether you're with or without the spin. Bob Geller and I figured out how to do the same analysis for the Earth. Why does this matter? Because the very largest earthquakes, Chile, Sumatra, Tohoku, put energy into the very longest-period bands of the Earth, a period of about 52 minutes, and from that, we could study properties of the earthquakes. Years later, after the 2004 Sumatra earthquake Emile Okal and I redid this and got a lot of attention, we were in the New York Times and everything. We showed that the Sumatra earthquake happened on a much, much bigger fault than was previously thought when the earthquake happened.
ZIERLER: Who was on your committee?
STEIN: Hiroo was the advisor. I'm pretty sure Don Anderson was on it. I don't remember who else. Committee structure was not a big deal there. It was the primary advisor, and the other people were just sort of there. I remember some interesting comments from Don Anderson. We got a very nice write-up about this Indian Ocean discovery, News & Views in Nature, talking about a major discovery that changed our ways of thinking about the Indian Ocean. I showed that to Anderson, and Don gave me an Anderson sort of grin and goes, "That's very nice, but that's what we expect to see from all our students." I was willing to take him at face value. [Laugh]
ZIERLER: In what ways did the research you pursued at Caltech set you on the path for your subsequent career?
STEIN: Totally. Basically, I had far more projects than I could do then. Most of these projects continued more or less indefinitely with students at Northwestern. The long-period seismology mode stuff, I've done since. We've been interested in mid-ocean ridges ever since, done a lot of stuff on that. We built plate-motion models, and that was derivative from there. The Indian Ocean, this idea of how plates deform internally, led to our global plate motion models. All these projects, you could think of five or ten years of stuff to do, and those in turn provided another five or ten years of stuff to do. I would say the only part of what I do that is not directly derivative from my Caltech time is the work we do in earthquake hazards. At the time, there was more of a separation between earthquake seismology, which was done for basic science, and engineering seismology, which was done for applications. When I was at Caltech, I had no involvement with the earthquake engineers. Later on, I got interested in that, too. In retrospect, I should've taken advantage of the earthquake engineers, but I didn't.
ZIERLER: Looking back at your time at the Seismo Lab, were you representing a school of thought that was very much of the Seismo Lab? In other words, you were doing research that was different than had you gone to Stanford for graduate school?
STEIN: It's hard to say. If you were to ask whether a lot of what I was doing was very much in the school of what Kanamori's students did, in the US or Japan, I would say yes. The lab had different groups. There was a very good group in mineral physics, high-pressure experiments that Tom Ahrens was running. Don Anderson was very interested in deep-earth structure, which I've never been involved in. I don't have an instinct for it. I'm from a plate tectonics generation. I have strong opinions and pretty good instincts about how plate tectonics work, but I have no instinct for what the light element in the core is or anything, so I never got involved in that stuff because I had nothing useful to contribute to it. I'd say that many of us who were involved with Kanamori over the years acquired certain attributes. I'm probably the most plate tectonic of that crowd, but that's because I came to the Lab that way. MIT was much stronger in plate tectonics at the time, and I was taught that there, so I came in knowing it. I was probably the only person at the lab then comfortable looking at marine magnetic anomalies, which was because I'd been taught that in undergrad school.
ZIERLER: What's your sense of why MIT was stronger in plate tectonics at that point?
STEIN: Good question. They for some reason bought into it sooner and faster. When I got to Caltech, it was quite far behind in terms of understanding things we knew and routinely talked about regarding plate tectonics at MIT. At the same time, the MIT seismology group at its best was as good at Caltech, but didn't have Caltech's depth and breadth. I think it was basically that Caltech was doing very well at what it was doing, so the incentive to expand into plate tectonics was not great.
Caltech was in a very unusual position then. Here's the way I think about it. These days, seismology is very democratic. Data's available everywhere online. Code is available everywhere online. There's nothing special about being in place A versus place B. There are probably 200 places in the world you could do equally well seismologically now, but that wasn't true then. Very few places had the same amount of data. Almost nobody had the same ability to compute waveforms and so on. Also, the part I wasn't involved in, they were very strong in local California seismology. The third thing, which is kind of subtle, is that the field was very small at the time, and a lot of information just wasn't written down. My big textbook came out of the idea that much important information wasn't actually written down. It might be buried in research papers by people who knew it, but there was no obvious way for students to find it easily. Bob Geller, Emile Okal, and I spent a lot of time agonizing over questions. If we went in and asked Kanamori, Dan Helmberger, or Dave Harkrider, they'd tell you the answer, because they knew it.
ZIERLER: There was almost an oral tradition in the field.
STEIN: Absolutely. One of the things I decided I would do, which took much longer than I thought it would, was to write a book and simply explain all this stuff. Michael Wysession and I wrote the textbook, but that took years. So now, most of the stuff, you can simply look up. I've had senior seismologists, faculty at good programs, come to me and say, "Hey, I've always wondered what they did about earthquake probabilities," or, "How is the attenuation measured in terms of velocity different than that measured in terms of the bulk moduli?" Don Anderson had actually figured that out, but it took us a long time to find it and put it in a book. One of the reviews on Amazon says, "Whenever I have a question in seismology, I can almost always find the answer in the book", but there was no comparable thing then. Some stuff I knew, I had learned from Aki at MIT, and other stuff, I learned from Kanamori at Caltech, but much of that information wasn't distributed.
Caltech was a place where they had the data. They had computers, and it wasn't that the computers were different than anywhere else, but they had software. It wasn't software products you distribute, but something that Hiroo was writing. We had this ludicrous experience, where I found an error in his associated Legendre polynomial routine, which was the heart of everything he was doing. To his credit, he dropped everything to chase down that error. It turned out, it hadn't been activated in any of the applications he had. It was a bug in his code, but none of it affected any of his results at all. [Laugh] But you didn't have the stuff because it wasn't distributed or documented, and lots of the oral lore of seismology about "Why does this work the way it does?" was hard to find. It was a very different time.
ZIERLER: The idea that the Seismo Lab dominated in terms of the data it had, is your sense that that was really baked into the origin of the Seismo Lab, coming from the Carnegie Institute?
STEIN: I don't think so, not directly. I think what happened is, they were very much plugged into the national science establishment, so when the government started distributing these archives of seismic data, only three or four places had the connections–I don't know if they had to pay or not–to get it. Columbia had it at Lamont, and I think MIT did, but I'm not positive. I think Caltech certainly had a data tradition; that's the way I would put it. Another name you'll hear is Hugo Benioff. Benioff was into instruments, building seismometers. I never had any connection to that world. He had retired by the time I got there. They had been operating a network in Southern California, which was Charles Richter's contribution. Gutenberg was much more interested in global seismology. I think they had a very strong tradition of observational seismology, actually working with seismograms. Some people were really good at it, like my colleague, Emile Okal. Of the three of us who were in school together, Emile was by far the best observational seismologist. He has this skill Kanamori has, where he will routinely discover things by looking at seismograms and saying, "I wonder what that is." I don't have that skill, but a number of people do. Richter, of course, was interested in such things. The instrumentation was probably less important than the ability to look at seismograms–I would go to a seismogram to look for an answer to a certain problem. Other people like Kanamori and Emile had this ability to look at a seismogram and say, "I wonder what that is." It's a very specialized skill. I never mastered it.
ZIERLER: You're obviously several academic generations from the founding, but being at the Seismo Lab, did you feel the influence of Gutenberg, Benioff, and Richter?
STEIN: Yes. You could see that in a couple of places. Each of them had different traditions. The strong interest in Southern California earthquakes, which I never acquired, was clearly Charles Richter's legacy. The deep-Earth structure was very much Gutenberg's, having grown up a seismologist in Germany where there aren't many earthquakes. The strong tradition in math and physics was Gutenberg's legacy, and I came out of a similar sort of tradition at MIT. In those days, one did not do double degrees, but I was one course short of doing a physics degree and one course from a math degree. I knew I wanted to go to grad school in geology, so I didn't get them. But I came with that knowledge, so that came very naturally. When I told Hiroo, "I think we can find the answer for this problem in the quantum mechanics literature," he thought that was perfectly reasonable. We were supposed to take additional math courses, and some of us took it and liked it. There were other people who didn't. But there was still that tradition. There was good and bad to it. I'd say, compared to a lot of other school's students, Caltech students were very reluctant to question faculty, and I got in a lot of trouble when I did. But at the same time, most of the lab faculty were extremely bright and set a good intellectual tone.
What I've tried to do in my group is to maintain that intellectual tone, but without the "Herr professor" side. Caltech was more prone to than I would've liked. But Caltech had a tradition where doing complicated and difficult things was considered normal and healthy. I think that was their strength.
The time I was there is not a replicable time. Once the data, software, and understanding of how to do seismology in the world started flowing around–it's not replicable. Just like you couldn't continue Bell Labs, you couldn't continue the Seismo Lab as it was. Although they've kept the name, it's no longer led by seismologists, as far as I know. You could see that big change. It's a good general geophysics program, but is it better than 20 others? Probably not. It called for very special, new data from the World-Wide Network, real breakthrough advances in waveform analysis of seismograms, etc. A bunch of stuff came together, and those of us who were there at the time had no idea how lucky we were. There were things I didn't like about the place, but at the same time, there's no question that it completely influenced everything I've done ever since.
ZIERLER: It's a great opportunity to be retrospective and have that appreciation.
STEIN: I started thinking about this when I started writing my book about the New Madrid earthquakes. Most of this is discussed in more detail there. But it was really interesting, Emile Okal and I were talking about some of this, and part of it was, interestingly enough, the Sumatra earthquake. I exhumed all these programs on punch cards, and I'd copied them onto tape. I hadn't looked at this code in probably 30 years. And we started talking about the old days, and it was really interesting to think back on that time. There was good and bad, but it really was a situation where a bunch of factors came together, some very special people. Then, there were all these strange contributing factors. One was that both Kanamori and Aki had come to the US because there were a lot of political problems in the University of Tokyo at the time. Senior faculty there either took jobs in the US, such as those two, or like Uyeda, who just loved traveling to other places.
The Seismo Lab was very fortunate. I think something like that, you couldn't really hold together long-term, but then you wouldn't really expect to be able to. This was the period where the US was absolutely still preeminent in world science, and the only group that was comparable in seismology was the Japanese. Now, what we do in the U.S. is good, but there are good people in France, and many other countries.
ZIERLER: That's probably a good thing, this democratizing effect for information.
STEIN: I think so, I think it's a healthy thing for science. When Space Geodesy came online, NASA absolutely required it. NASA has always been very aggressive about requiring this stuff. I was involved in starting this big program called EarthScope, a big NSF project to understand the structure and evolution of North America. NSF, from day one, made it clear all this data would be available in real time online to anyone in the world. It's clearly good for science, but it does mean that the days of three or four top-dog institutions are long since gone. There's nothing you have to be in Pasadena to do that you can't do anywhere else. I think the astronomers, for example, have long since hit this point.
Plate Tectonics in Retrospect
ZIERLER: For the last part of our talk, I'd like to ask some retrospective questions, where you think about your time at the Seismo Lab and what's happened in the field since. I love that in seismology and geophysics, there are orthodoxies that get blown up and orthodoxies that remain over the years. Let's start with some of the real solid propositions or hypotheses when you were a graduate student that have lived up well over the years. What are they?
STEIN: I came in right after the basic plate tectonic paradigm was established. Anyone ten years older than I would've been through that transition. I was just taught it as a freshman. That has held up well. Our basic seismological paradigms in terms of how waves and earthquakes operate were resolved, again, slightly before my generation. I walked in and was taught, "This is how the normal modes work, this is how the double couple faults work." Those have held up. Some things I would say have not held up as well. People wanted to believe that earthquakes were much more regular than they do now. The advent of paleoseismology, where they started digging trenches and building long historic records. showed clearly how very variable earthquakes are. Once you start realizing you're talking about recurrences of 178 years plus or minus 85 years, you say, "Well, the earthquake forecast can't be worth much in those conditions except over very long terms."
That changed with the advent of the paleoseismic data. Another big change was the following. This is more on the hazard side, so I was not thinking about this when I was at the lab. But there was all this talk about "the big one", this big earthquake that would do damage in LA. We didn't really appreciate how ground motion worked. We now know that ground motion is like any other seismic energy, spreads as R-squared on the surface, or R-cubed in the interior, decays incredibly rapidly with distance. We've now realized that the real danger in Los Angeles is not a magnitude 8 out on the San Andreas, which is on the other side of the mountains. The real killers have been sixes in the LA Basin underneath you. We didn't even know that those could happen. I came immediately after the San Fernando earthquake in 1971, and people thought that was a one-off. Then, during the time I was there, essentially no large earthquakes happened in Southern California. Sometime later, around the 80s, we routinely started to have good-sized earthquakes in the LA Basin, like the Northridge earthquake. We realized that the real danger is not the eights far away, but the sixes right underneath you, and that's because ground motion decays so rapidly with distance. That, I think, was not in any way appreciated at the time. It was a very big conceptual change in our worldview.
We also eventually realized that precursors to earthquakes, which all these people were looking for to predict them, don't seem to exist. No one's found any convincing ones. We've concluded that earthquakes are much less regular than anybody thought. We've concluded that a lot of the hazard comes from the moderate-sized earthquakes.
Another big thing is that before space geodesy, we didn't know how steady plate motions were. We couldn't really say, "If we have 35 millimeters here on the San Andreas, and we have 50 millimeters in the Gulf of California, could it just be that the last 1,000 years, the fault's been moving slowly?" At the time, we had no way of assessing that hypothesis. When we got space-based geodesy, we could say, "Oh, no, the plates are moving on average at the same speeds now as they have over the past three million years." The steadiness of plate motions, I think, is really one of the major discoveries. I wouldn't say it disproved anything because we had absolutely no idea whether they were steady or not when I was in school. I'd say this was the biggest discovery on the tectonic side that we've had.
Then, of course, there were advanced imaging techniques that came just from the proliferation of digital data, that you could do so much more with that. Also, I was brought up on the idea that there were huge earthquakes in the center of the country, and it was functioning like a plate boundary, only moving slowly. Now, we know that's not true. Basically, you'll have one fault that'll work for a while then switch off, another fault will kick in, which we call episodic, clustered, and migrating. That was something we didn't have a clue of at the time. Our basic paradigms on the earthquake side have held up relatively well, but that's because by the time I came into it we were riding on the shoulders of giants. [Laugh] A huge amount of stuff was discovered in the 60s, the decade before I started studying geology.
ZIERLER: Last question, either from your time at the Seismo Lab or not, looking at all of the seismological events you have studied in the interim, what has surprised you the most, and what has been most useful in convincing you that earlier-held notions, either by you or the giants whose shoulders you've stood on, have stood the test of time over the years?
STEIN: Or the other way. I would say one of the more interesting things when I was in school was the idea that–and this was one of the rare cases where Kanamori turned out to be wrong–how big an earthquake you'd get at a subduction zone was related to the speed at which the plate was being stuffed under and the age of the plate that controls the temperature structure. Hiroo said, based on this, that certain subduction zones wouldn't have giant earthquakes. We all sort of accepted that, that in the velocity and age space, there was a whole province that included a lot of subduction zones, that wouldn't get these giant earthquakes. And these giant earthquakes are what generate the tsunamis that kill thousands of people, tens of thousands in some case. There's a famous graph on this that Hiroo made, which turned out to be completely wrong. We had the Sumatra earthquake and thought "wow, it's not supposed to have this kind of earthquakes." Then, we realized, "Oh, it must just be that we hadn't seen them in the record because the record wasn't long enough." I would say the single biggest thing that changed our worldview was the Sumatra earthquake. Now, I think, most of us would lean toward the view that you can have earthquakes that size at any subduction zone that's long enough to accommodate a long fault.
With the Earth, you have to expect the unexpected because our history is so short, and our observations are so limited, that we're always in this situation of, "OK, we have not seen the Earth do such-and-such. Does that mean that the Earth cannot do such a thing, or does it mean that we just haven't seen it?" You'll hear this debate now, "What's the longest possible piece of the San Andreas that could rupture?" Because that will contain the size of the largest earthquake you can get on the San Andreas. We have never had a wall-to-wall rupture, starting out where the fault goes out to sea at Point Reyes going all the way down to the Imperial Valley. We've never seen that. It's three times bigger than we've ever seen. Does this mean that this cannot happen for some reason related to the physics of the fault and the strength of various regions? Or does it mean we just haven't seen it? This issue shows up so many times, and it's very difficult to work our way around.
ZIERLER: That was true when you were a graduate student, and it's true now?
STEIN: Oh, yeah. We dedicated the seismology book to the future generations of seismologists. I've generated about two generations - I've had PhD students who have had PhD students. There are still a lot of very fun things to do, which is what makes geophysics exciting One of the reasons I never had any real interest in physics is that you take physics classes and they tell you all this known stuff. It's interesting, but I had most of a physics major, and I never once heard a discussion of anything they didn't know that would be interesting to study, whereas in geology, there was a long list of things that we don't really know.
ZIERLER: That's interesting because it's not as if physicists do know everything. Maybe that's just a cultural issue.
STEIN: They do a very good job of trying to hide that in their teaching, whereas I've got juniors in class this quarter to whom I'll say, "We're not really sure why this is." When we wrote the seismology book, I wrote a lot of parts that said, "No one really knows why this is," and I used that to motivate PhD students to work with me on stuff. I'd say, "This is a really cool problem, and nobody knows the answer to it." That was the thing I found attractive about geophysics, and I would credit several of my teachers over the years. In the context of Caltech, it would be Kanamori, who was always very good about admitting the things that weren't known. I had an interesting discussion with him about the following question. What causes these really deep earthquakes, at depths of 600 or 700 kilometers? It's a very interesting problem, and he told me a profound thing. He said, "Can you think of anything to do about it? I've always been interested in this problem, too, but I can't think of anything interesting to do about it. If you can come up with something useful, let's talk about it." I was impressed and I've always adopted that mannerism. If a student's interested in some question, I'll say, "What can we do to advance our understanding of this?"
ZIERLER: There was low-hanging fruit then, and there remains low-hanging fruit in the field now.
STEIN: Yeah, and I think that's something that's very important. The other thing I think we have compared to physics is, we don't operate with what we see as fundamental laws. We're aware that a lot of what we're dealing with are basically empiricisms, like thinking we won't have giant earthquakes on a particular subduction zone. There's a line in my natural hazards book that goes, "Nature bats last." [Laugh]
By the way, if you come across fun adages in my seismology book, many are Kanamori-isms. The way the Japanese language works, things tend to come out sounding very Zen and Yoda-ish. Depending on whose work he was complaining about at the time, he would often say, "So-And-So's very smart, but perhaps the Earth is not as smart as they are." And he fell victim to this, too, we all do. But he was showing healthy skepticism that it was not a given that the Earth would behave according to our preconceptions. I remember, in class he said something like, "The inner core, assuming it exists, is said to have the following properties." I don't think he was saying that it didn't exist, he was just saying he wasn't absolutely convinced that the evidence it did exist was so compelling that he necessarily bought into it.
To me, that's one of the nicest parts of doing geophysics, that there are still enormous amounts of stuff out there. A very bright junior faculty member I know is trying to figure out what she should work on. She's got great skills, an office and a computer and so now what's she going to do for the rest of her life, in particular the next six years to get tenure? I said, "You can pick almost any randomly selected three or four basic beliefs in geophysics and start looking at them hard, and I think you'll determine that the evidence for at least one of them isn't very convincing."
ZIERLER: On that note, this has been a great conversation. I'm so happy we were able to do this. I'd like to thank you so much.
[END]
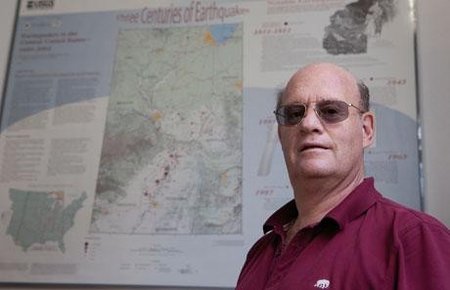